Abstract
The fluorescence kinetics of C-Phycocyanin in the monomeric, trimeric, and hexameric aggregation states has been measured as a function of the emission wavelength with picosecond resolution using the single-photon timing technique. All the decay curves measured at the various emission wavelengths were analyzed simultaneously by a global data analysis procedure. A sum of four exponentials was required to fit the data for the monomers and trimers. Only in the case of the hexamers, a three-exponential model function proved to be nearly sufficient to describe the experimental decays. The lifetime of those fluorescence components reflecting energy transfer decreased with increasing aggregation. This is due to the increased number of efficient acceptor molecules next to a donor in the higher aggregates. In all aggregates the shortest-lived component, ranging from 50 ps for monomer to 10 ps for hexamers, is observed as a decay term (positive amplitude) at short emission wavelength. At long emission wavelength it turns into a rise term (negative amplitude). The lifetime of a second ps-component ranges from 200 ps for monomers to 50 ps for hexamers. The long-lived (ns) fluorescence is inhomogeneous in monomers and trimers, showing two lifetimes of ∼0.6 and 1.3 ns. The latter one carries the larger amplitude. The amplitudes of the kinetic components in the fluorescence decays are presented as time-resolved component spectra. A theoretical model has been derived to rationalize the observed fluorescence kinetics. Using symmetry arguments, it is shown that the fluorescence kinetics of C-Phycocyanin is expected to be characterized by three exponential kinetic components, independent of the aggregation state. An analytical expression is derived, which allows us to gain a detailed understanding of the origin of the different kinetic components and their associated time-resolved spectra. Numerical calculations of time-resolved spectra are compared with the experimental data.
Full text
PDF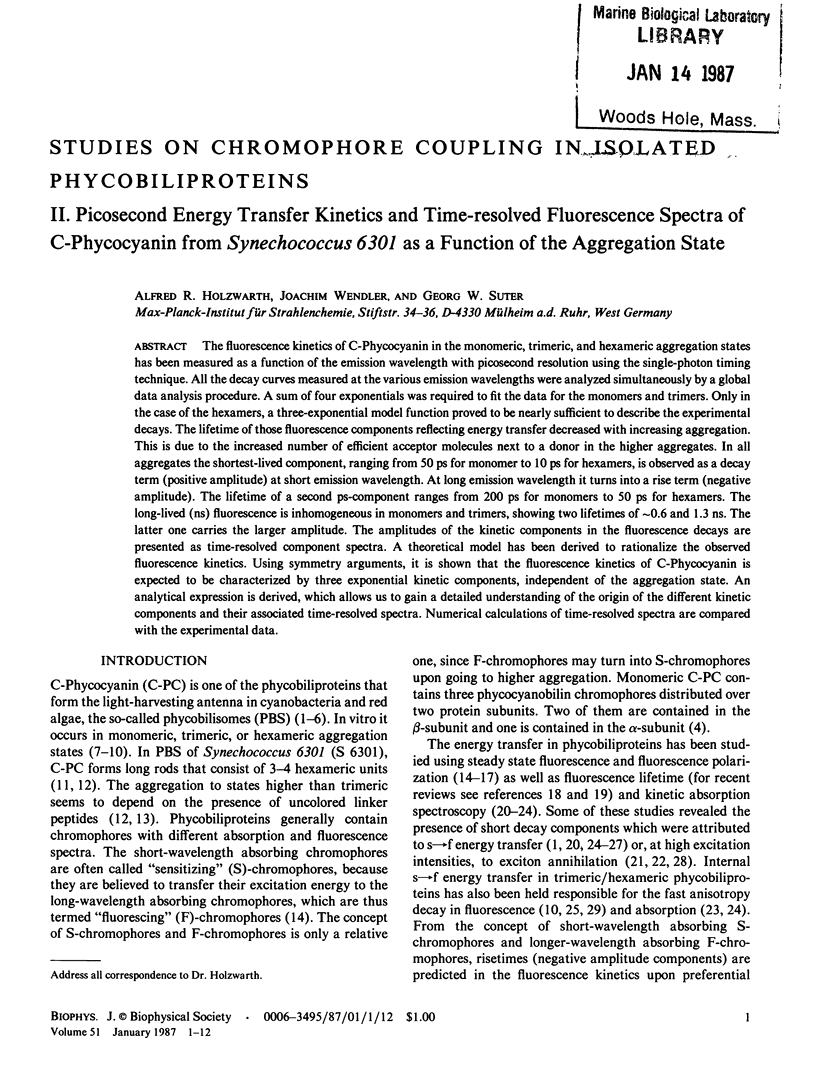
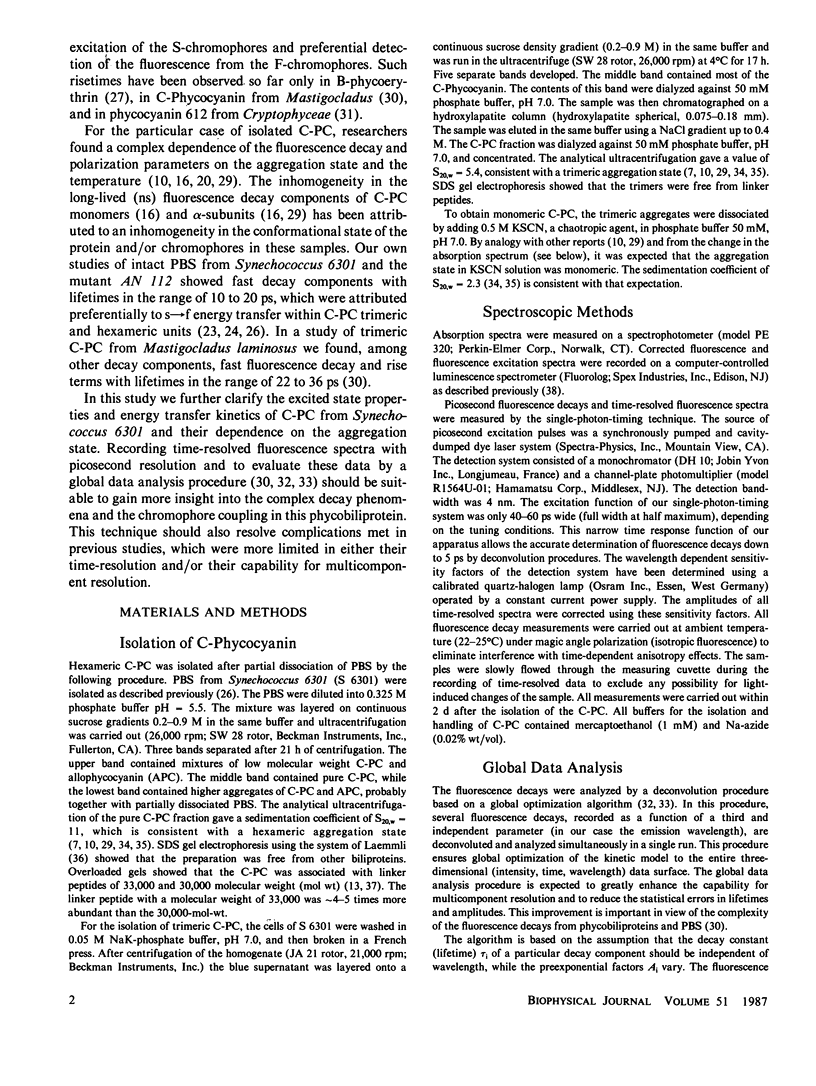
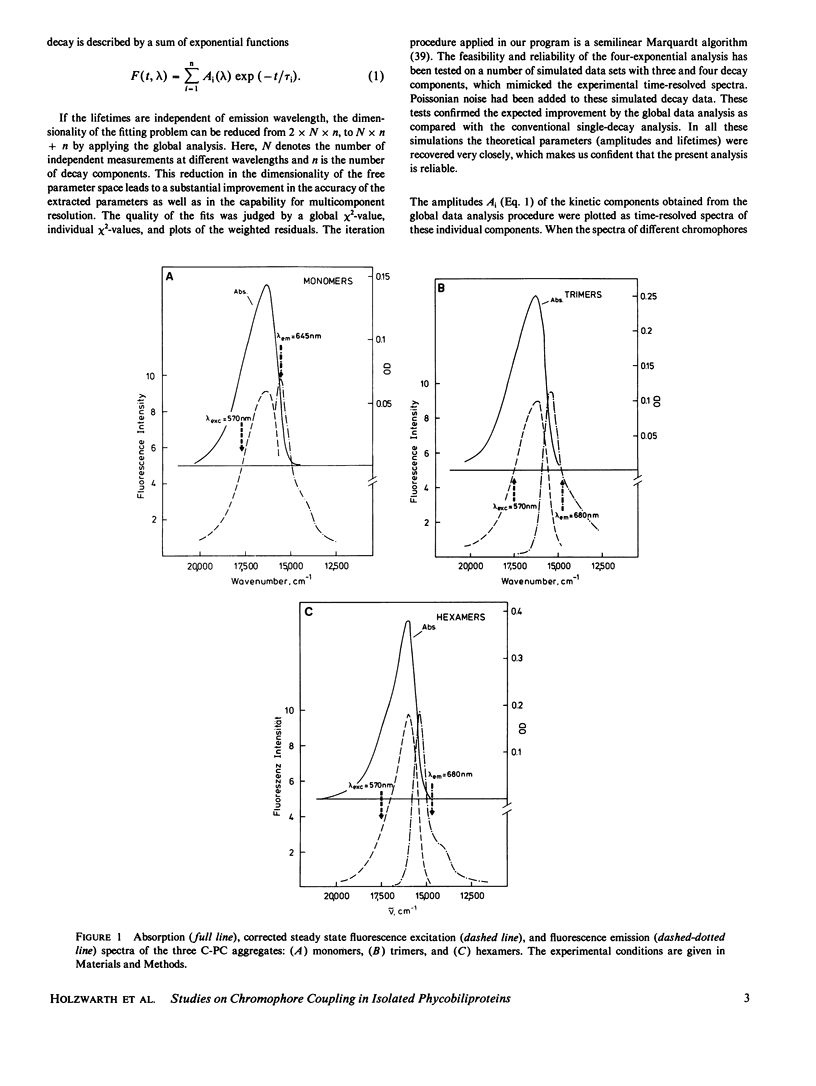
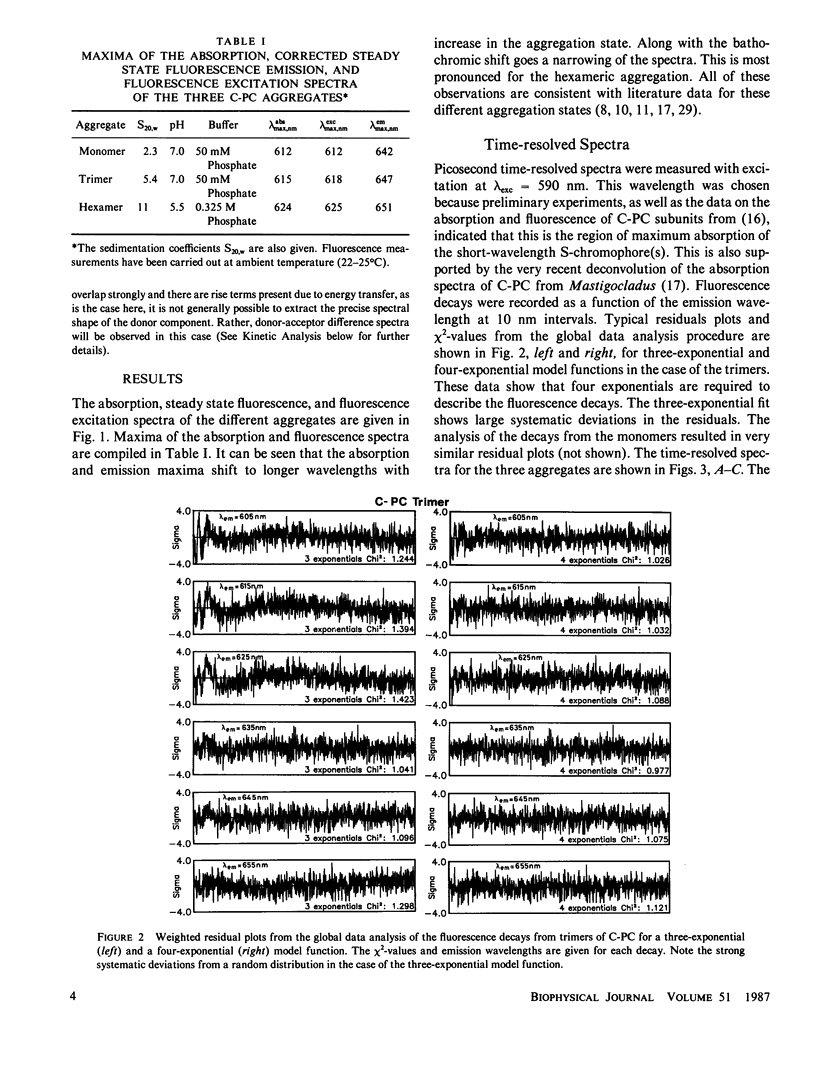
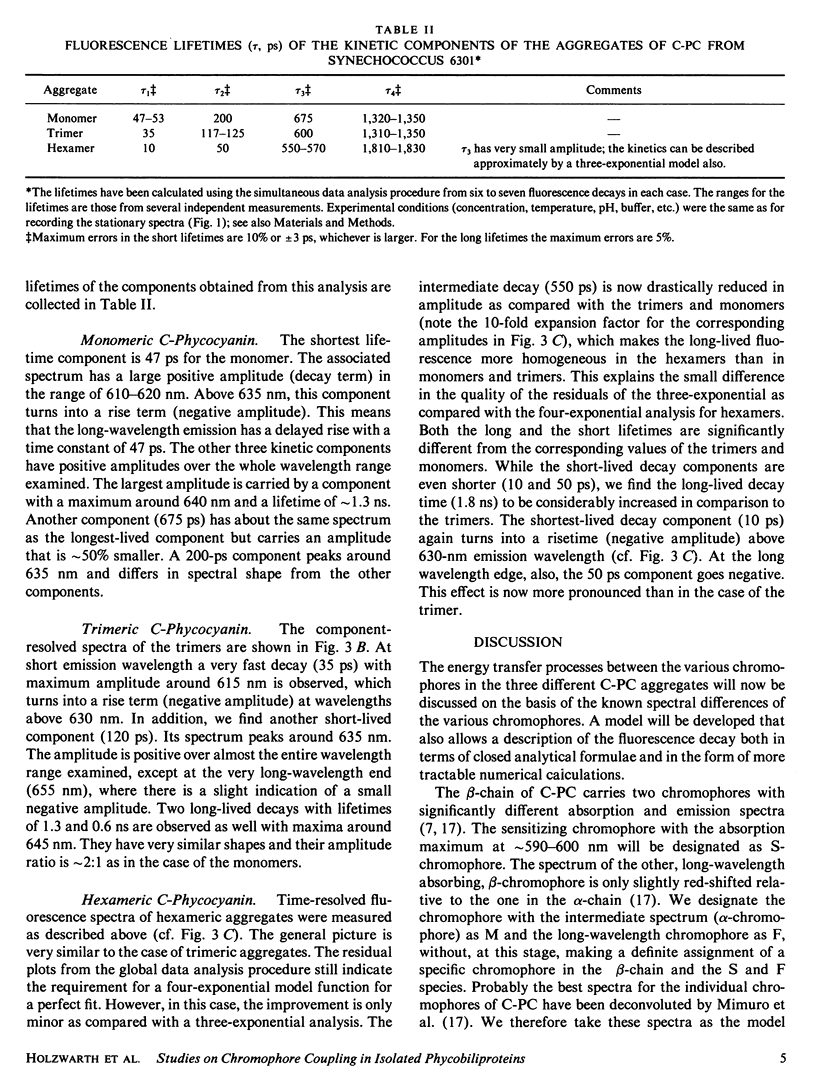
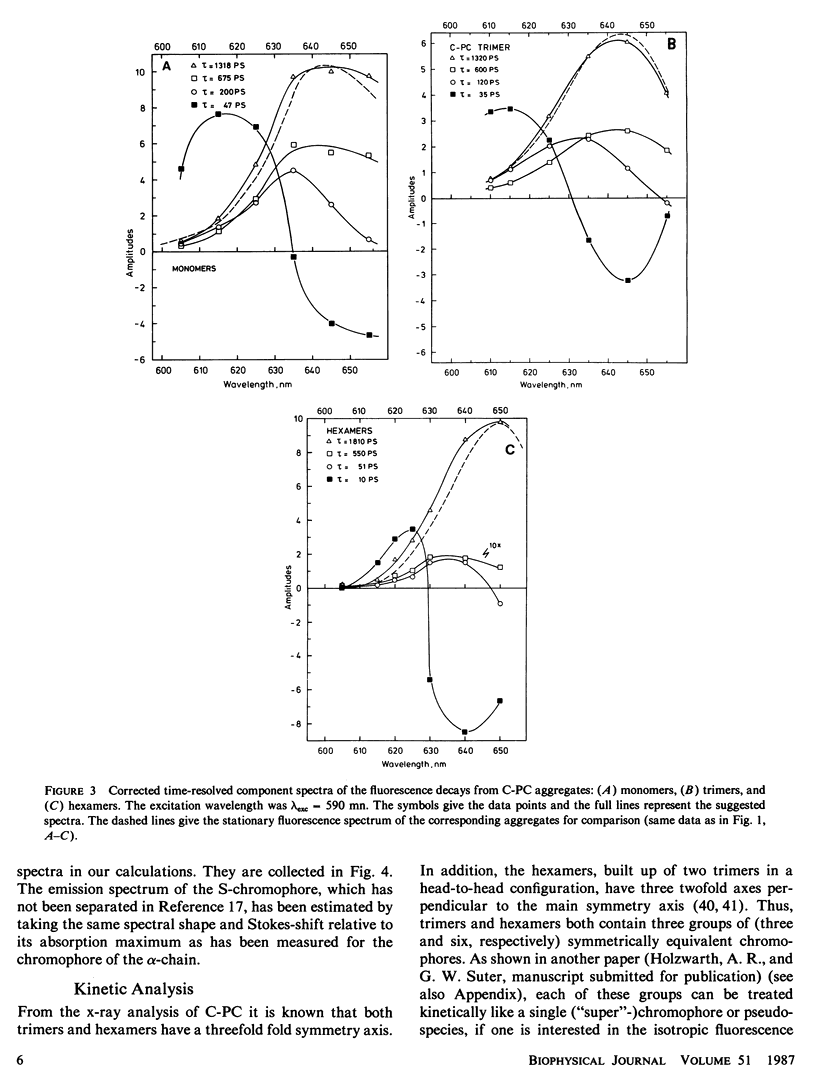
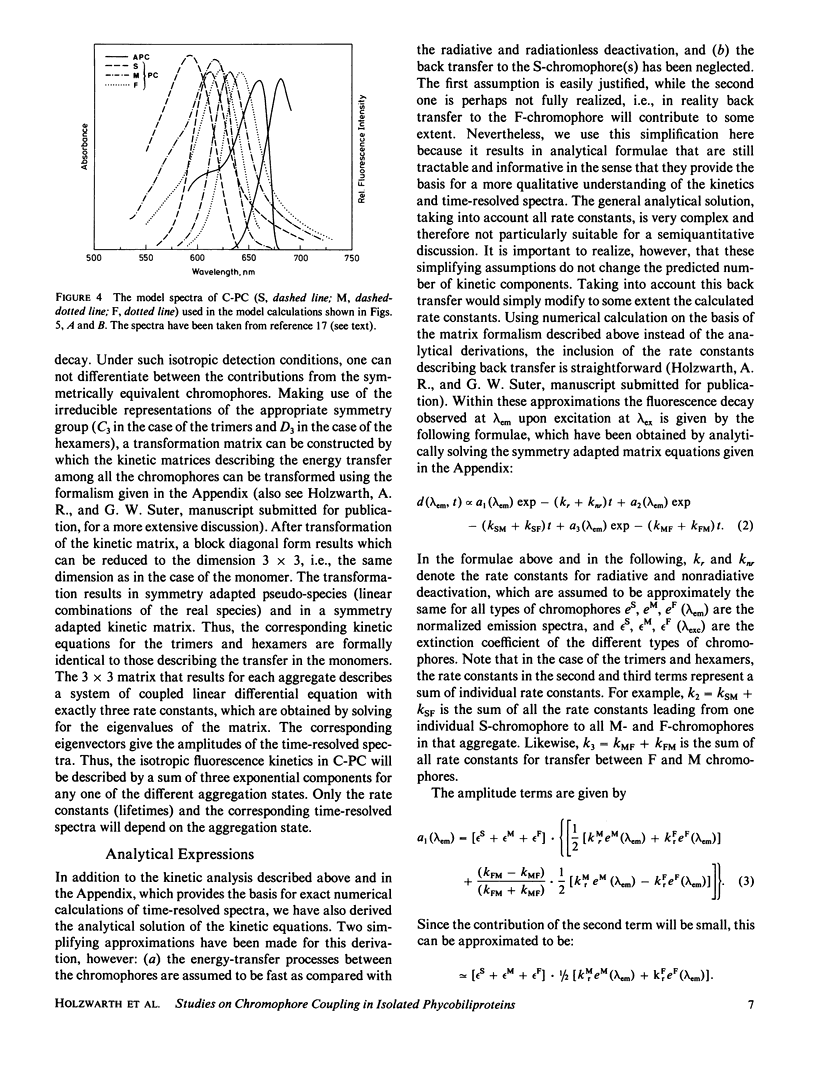
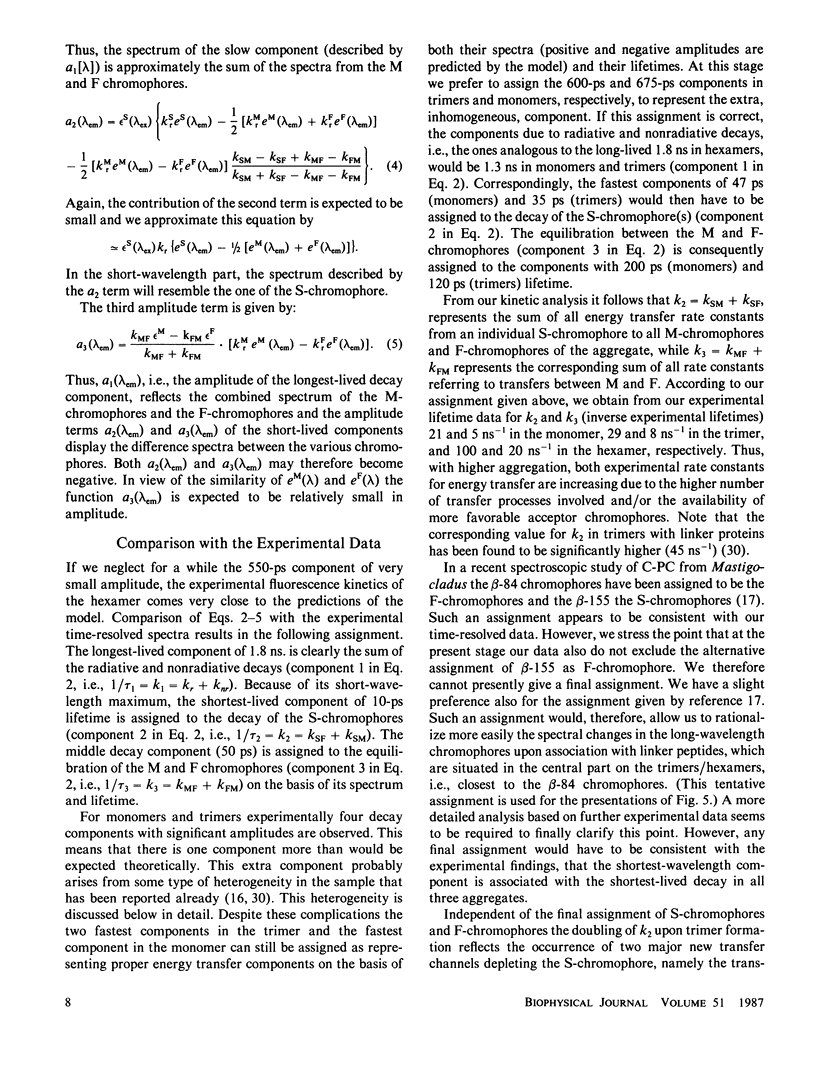
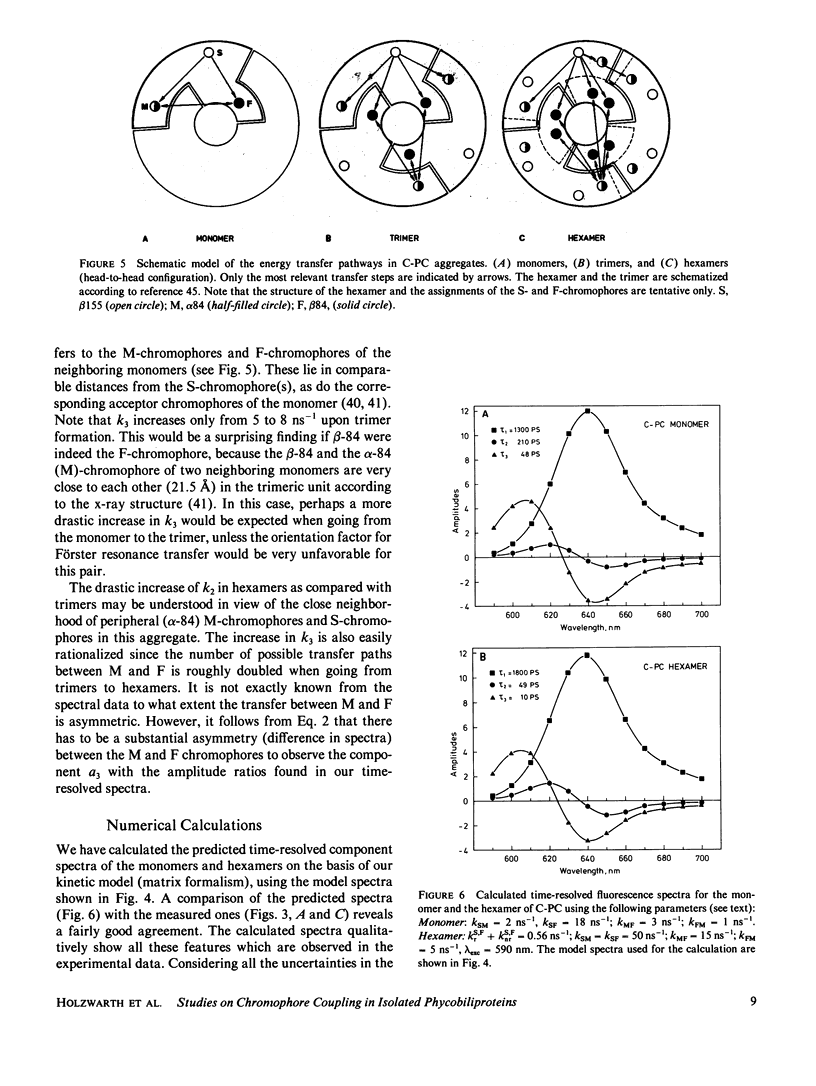
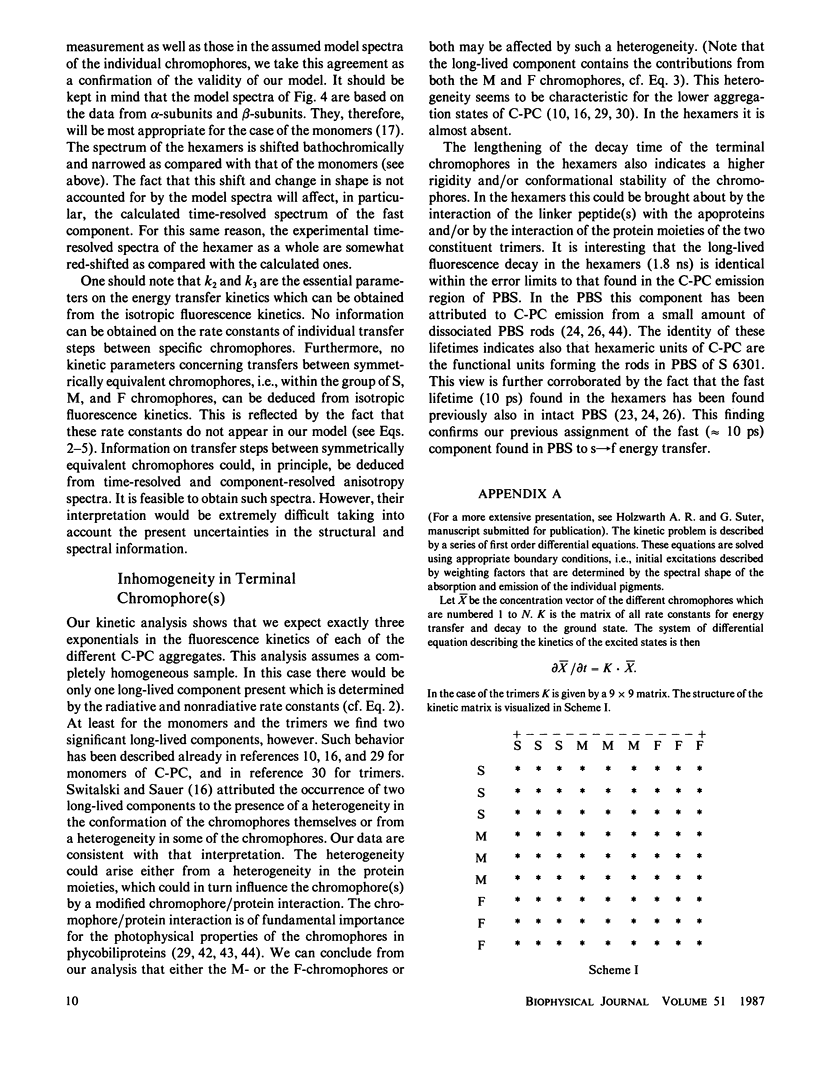
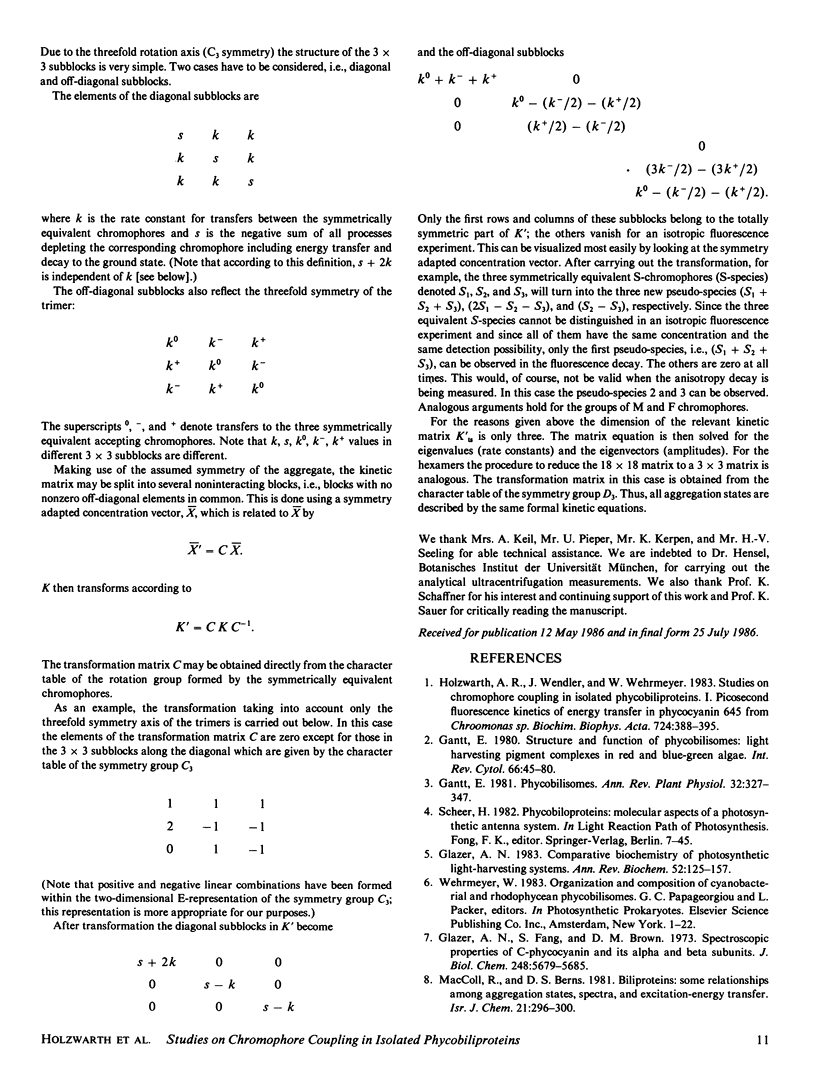
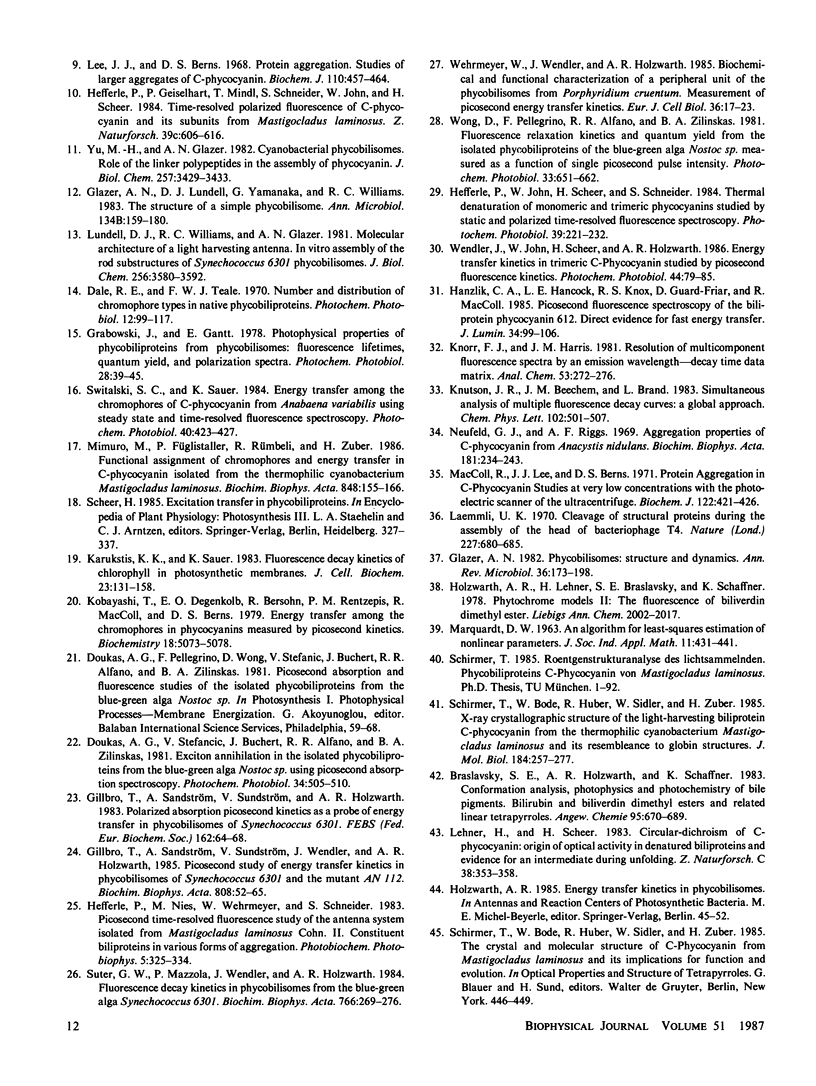
Selected References
These references are in PubMed. This may not be the complete list of references from this article.
- Dale R. E., Teale F. W. Number and distribution of chromophore types in native phycobiliproteins. Photochem Photobiol. 1970 Aug;12(2):99–117. doi: 10.1111/j.1751-1097.1970.tb06044.x. [DOI] [PubMed] [Google Scholar]
- Glazer A. N. Comparative biochemistry of photosynthetic light-harvesting systems. Annu Rev Biochem. 1983;52:125–157. doi: 10.1146/annurev.bi.52.070183.001013. [DOI] [PubMed] [Google Scholar]
- Glazer A. N., Fang S., Brown D. M. Spectroscopic properties of C-phycocyanin and of its alpha and beta subunits. J Biol Chem. 1973 Aug 25;248(16):5679–5685. [PubMed] [Google Scholar]
- Glazer A. N., Lundell D. J., Yamanaka G., Williams R. C. The structure of a "simple" phycobilisome. Ann Microbiol (Paris) 1983 Jul-Aug;134B(1):159–180. doi: 10.1016/s0769-2609(83)80103-3. [DOI] [PubMed] [Google Scholar]
- Glazer A. N. Phycobilisomes: structure and dynamics. Annu Rev Microbiol. 1982;36:173–198. doi: 10.1146/annurev.mi.36.100182.001133. [DOI] [PubMed] [Google Scholar]
- Karukstis K. K., Sauer K. Fluorescence decay kinetics of chlorophyll in photosynthetic membranes. J Cell Biochem. 1983;23(1-4):131–158. doi: 10.1002/jcb.240230112. [DOI] [PubMed] [Google Scholar]
- Kobayashi T., Degenkolb E. O., Bersohn R., Rentzepis P. M., MacColl R., Berns D. S. Energy transfer among the chromophores in phycocyanins measured by picosecond kinetics. Biochemistry. 1979 Nov 13;18(23):5073–5078. doi: 10.1021/bi00590a008. [DOI] [PubMed] [Google Scholar]
- Laemmli U. K. Cleavage of structural proteins during the assembly of the head of bacteriophage T4. Nature. 1970 Aug 15;227(5259):680–685. doi: 10.1038/227680a0. [DOI] [PubMed] [Google Scholar]
- Lee J. J., Berns D. S. Protein aggregation. Studies of larger aggregates of C-phycocyanin. Biochem J. 1968 Dec;110(3):457–464. doi: 10.1042/bj1100457. [DOI] [PMC free article] [PubMed] [Google Scholar]
- Lundell D. J., Williams R. C., Glazer A. N. Molecular architecture of a light-harvesting antenna. In vitro assembly of the rod substructures of Synechococcus 6301 phycobilisomes. J Biol Chem. 1981 Apr 10;256(7):3580–3592. [PubMed] [Google Scholar]
- MacColl R., Lee J. J., Berns D. S. Protein aggregation in C-phycocyanin. Studies at very low concentrations with the photoelectric scanner of the ultracentrifuge. Biochem J. 1971 May;122(4):421–426. doi: 10.1042/bj1220421. [DOI] [PMC free article] [PubMed] [Google Scholar]
- Neufeld G. J., Riggs A. F. Aggregation properties of C-Phycocyanin from Anacystis nidulans. Biochim Biophys Acta. 1969 May;181(1):234–243. doi: 10.1016/0005-2795(69)90246-3. [DOI] [PubMed] [Google Scholar]
- Scheer J. K. Effect of placement in the order of competition on scores of Nebraska high school students. Res Q. 1973 Mar;44(1):79–85. [PubMed] [Google Scholar]
- Schirmer T., Bode W., Huber R., Sidler W., Zuber H. X-ray crystallographic structure of the light-harvesting biliprotein C-phycocyanin from the thermophilic cyanobacterium Mastigocladus laminosus and its resemblance to globin structures. J Mol Biol. 1985 Jul 20;184(2):257–277. doi: 10.1016/0022-2836(85)90379-1. [DOI] [PubMed] [Google Scholar]
- Yu M. H., Glazer A. N. Cyanobacterial phycobilisomes. Role of the linker polypeptides in the assembly of phycocyanin. J Biol Chem. 1982 Apr 10;257(7):3429–3433. [PubMed] [Google Scholar]