Abstract
The effects of disopyramide (Norpace) and 14 closely related structural analogues on the Na current of voltage clamped squid axons were examined to determine which physico-chemical properties and which changes in the structure of the Norpace molecule can alter the nature of its sodium channel blocking actions. Conventional voltage clamp technique for internally perfused giant axons was used. Axons were exposed to 100 microM concentrations via the internal perfusion solution, and the actions of the 15 analogues to produce resting and use-dependent block of Na current were assessed. The roles of Na ions and the activation and inactivation processes in the development of and recovery from use-dependent block of Na current induced by the Norpace analogues were also examined. The results indicate that for both mono-tertiary and bis-tertiary amines the potency to produce use-dependent block was proportional to molecular weight, whereas the correlation between potency to produce resting block and molecular weight was significant only for bis-tertiary amines. The mono- were more potent than the bis-compounds. However, comparisons between compounds having similar molecular weights and/or pKa values indicate that other factors also can influence blocking potency. For compounds within each homologous mono- or bis-tertiary amine series, hydrophobicity as estimated from log P values (P = octanol/water partition coefficient) was found to influence the potency to produce use dependent block of Na current. Use-dependent block was extant in axons internally exposed to pronase to remove the inactivation process, which indicates that inactivation is not an obligate condition for development of use-dependent block of Na current. An important role for the activation process in the development of use-dependent block of Na current is suggested by the finding that, in general, the voltage dependence of Na current activation paralleled that of use-dependent block. However, the potential dependence of use-dependent block produced by less hydrophobic but not by more hydrophobic compounds was shifted in the hyperpolarizing direction by removing Na+ from the external solution. Compounds with intermediate hydrophobicities altered the time course of Na current during its activating and inactivating phases. This finding can be explained by the kinetics of association and dissociation of drug molecules with channel receptor sites during the development and relaxation of use-dependent block rather than by postulating any major effect of drug to alter channel gating kinetics. In summary, a comprehensive study of the structure-activity relationship of the Norpace molecule was achieved and the implications of the findings with respect to several factors believed to influence drug potency for resting and use-dependent block of the Na current in squid axon are examined and discussed.
Full text
PDF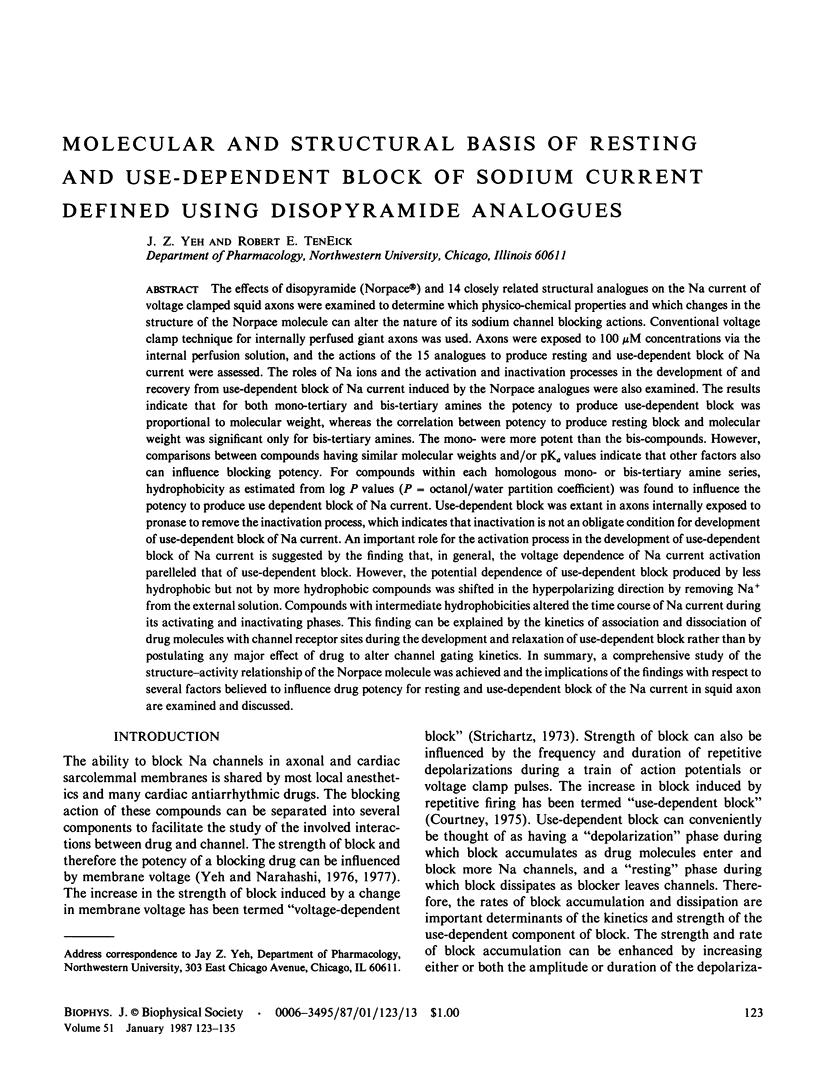
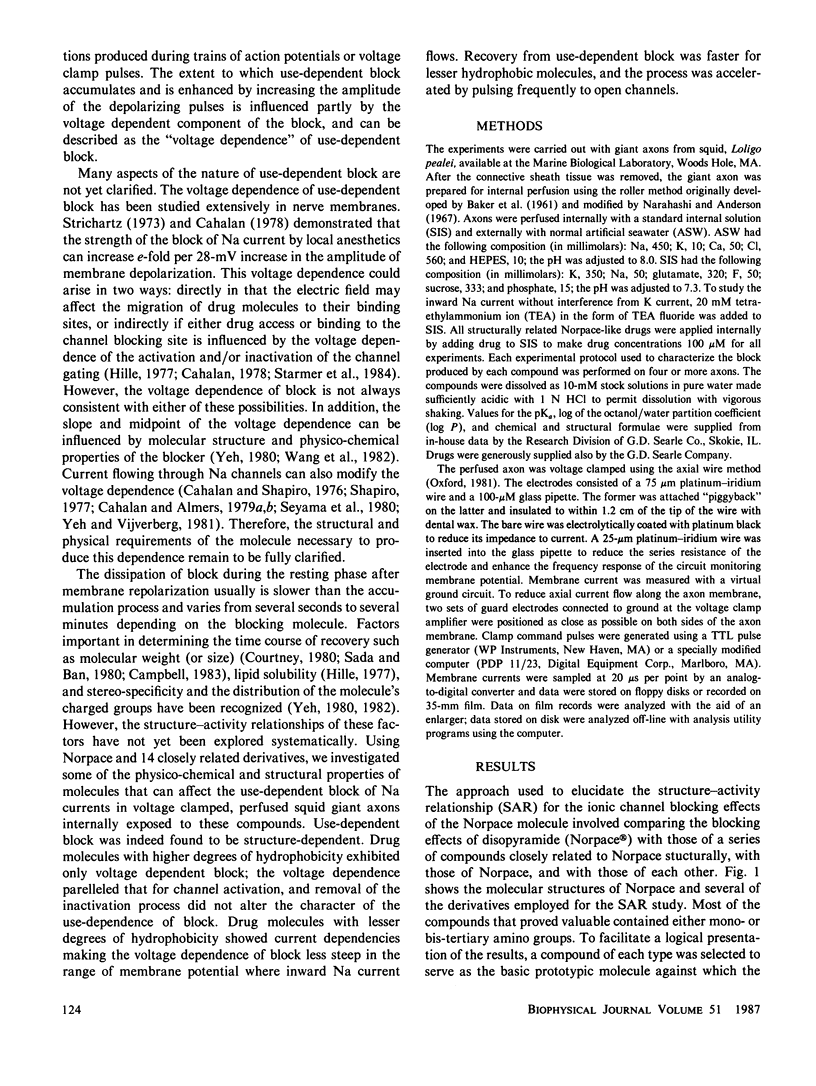
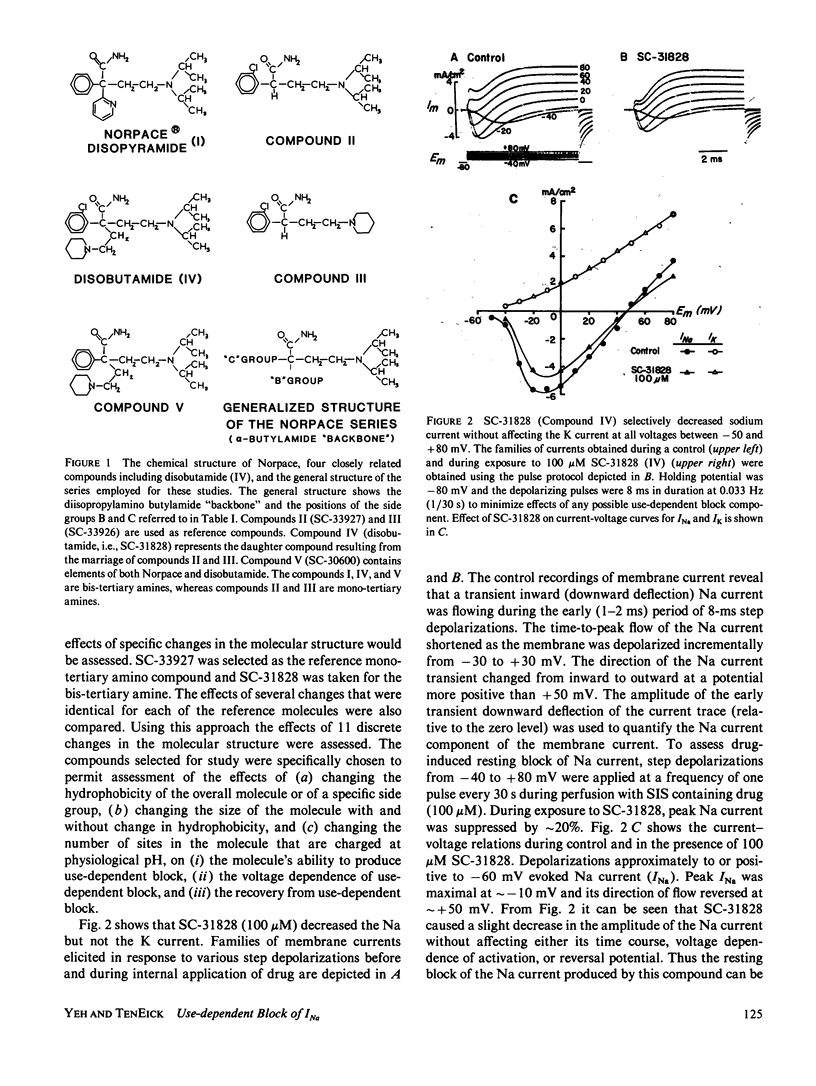
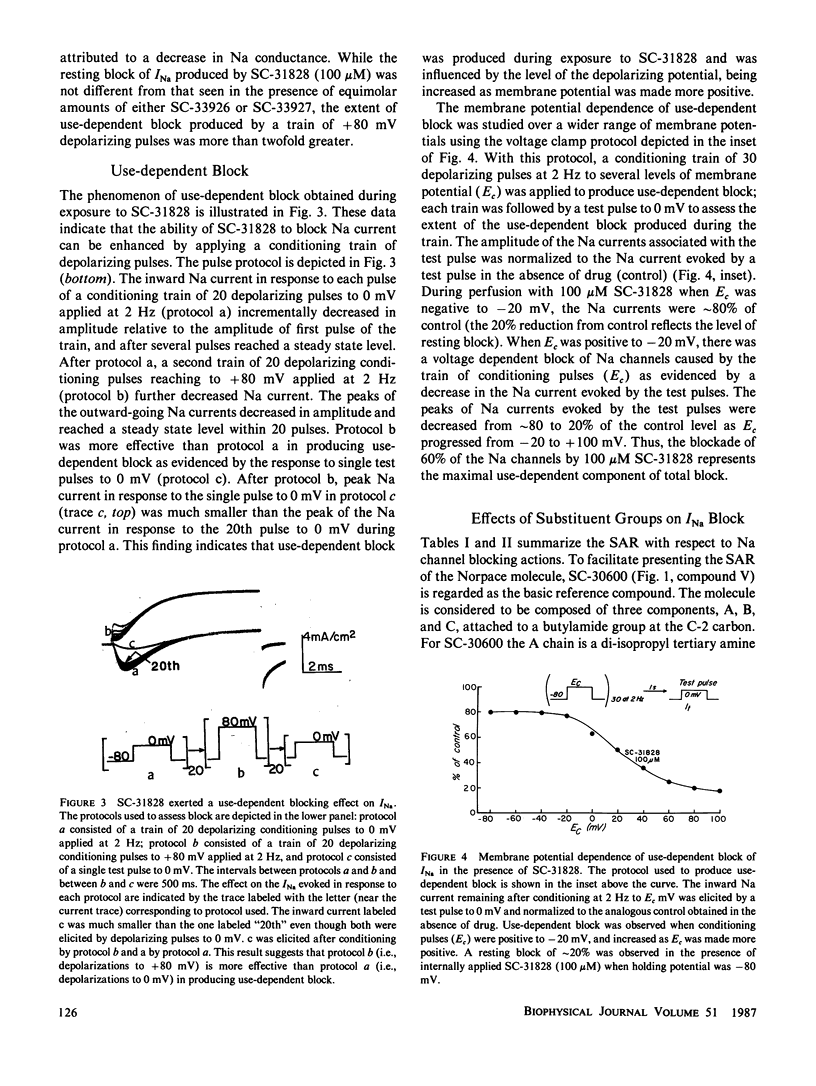
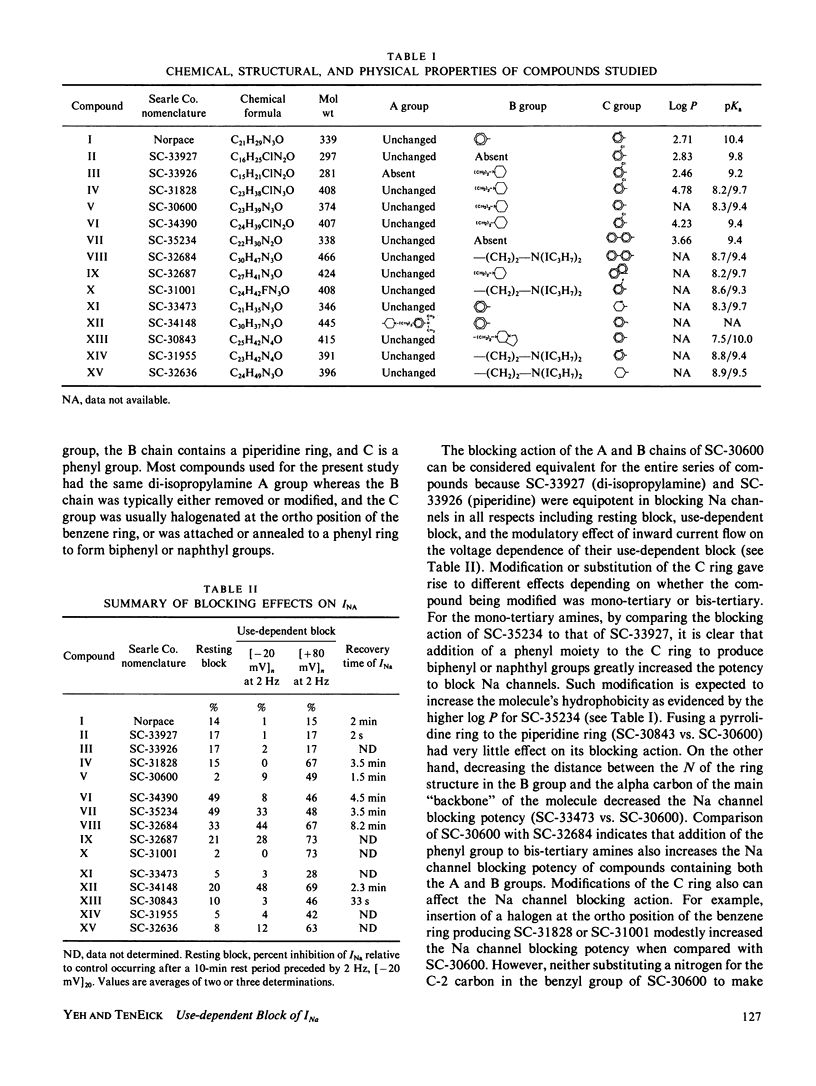
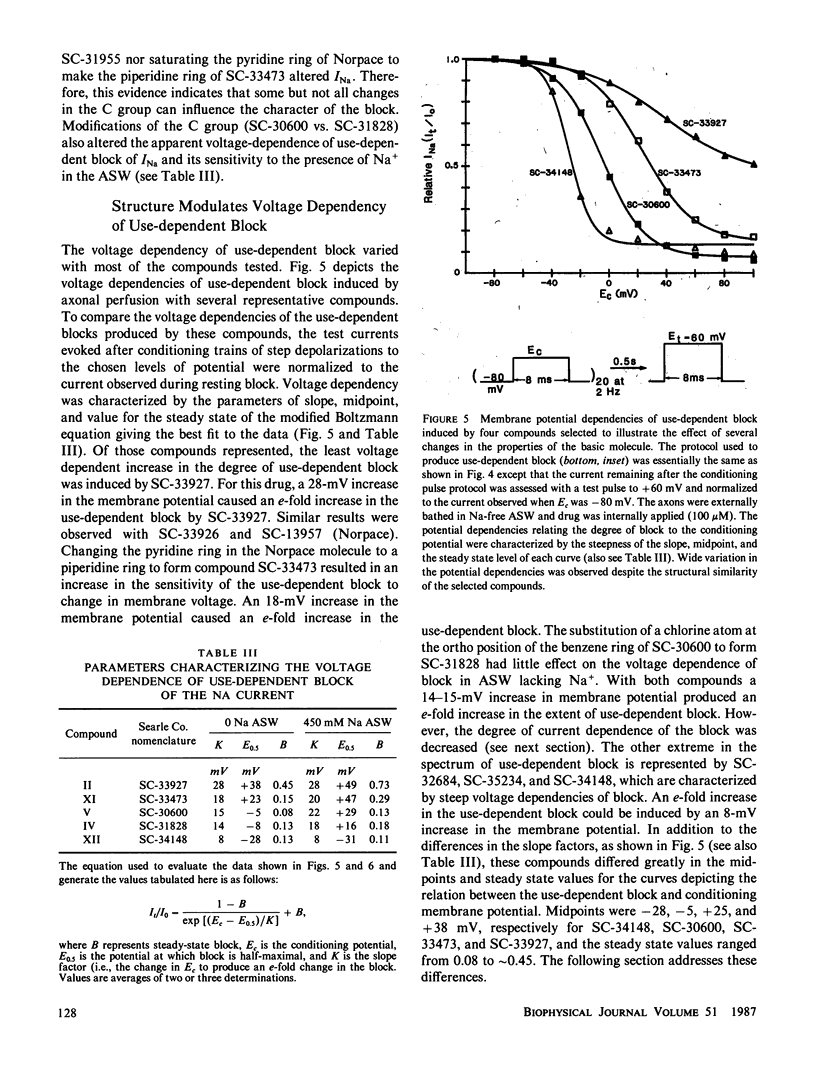
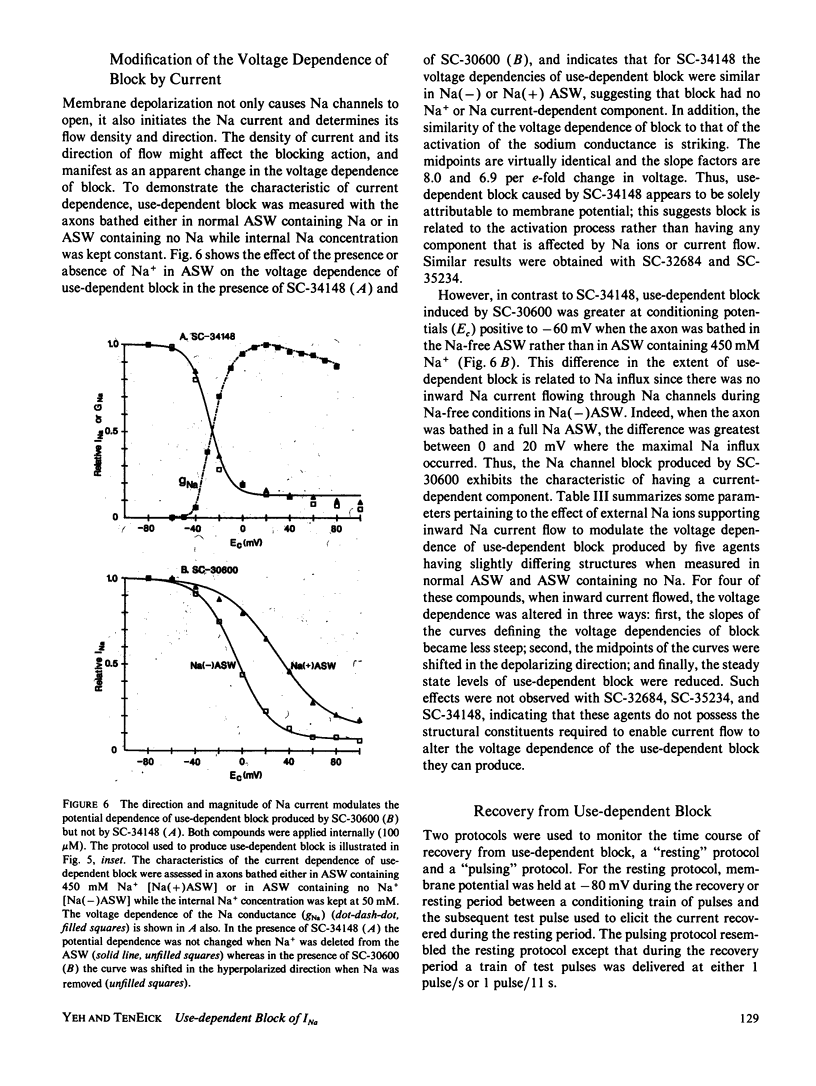
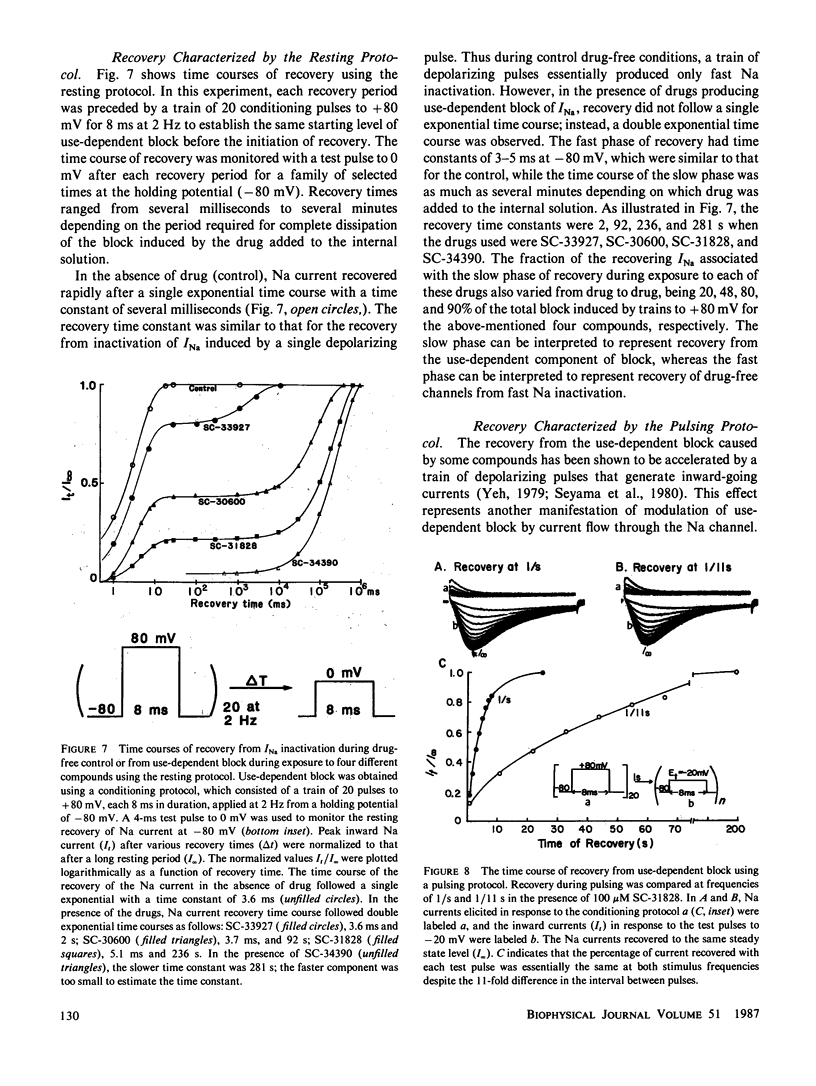
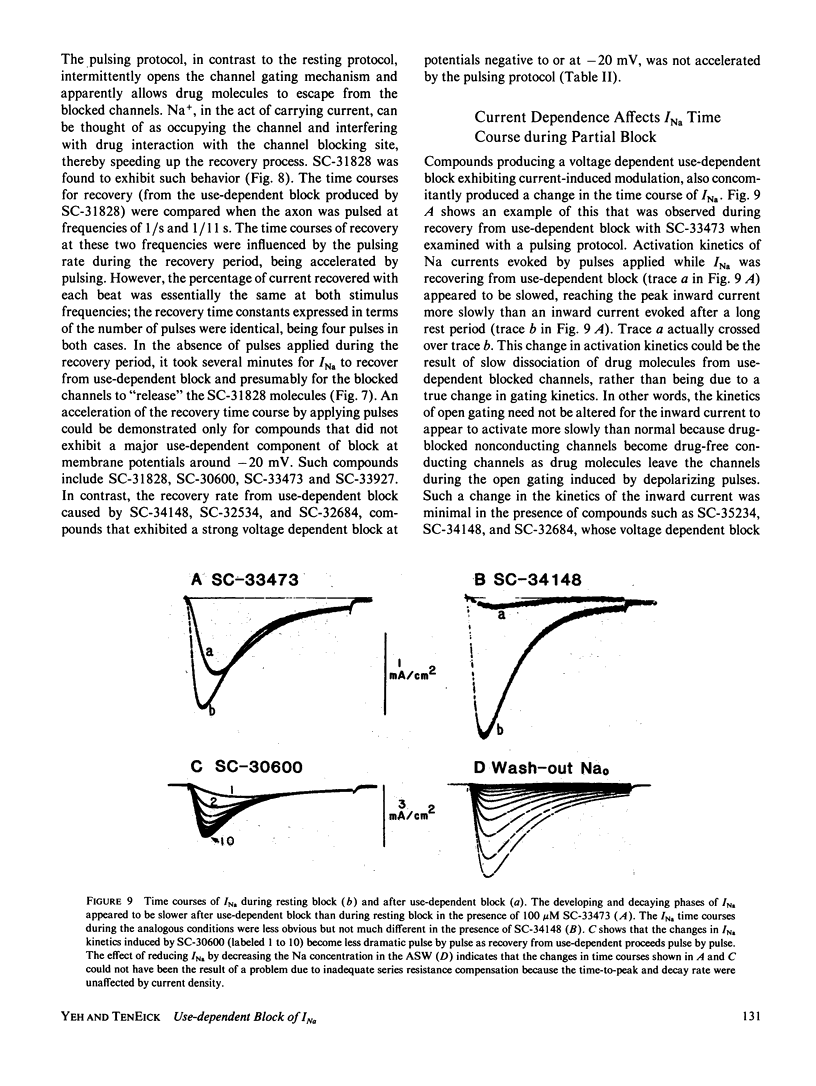
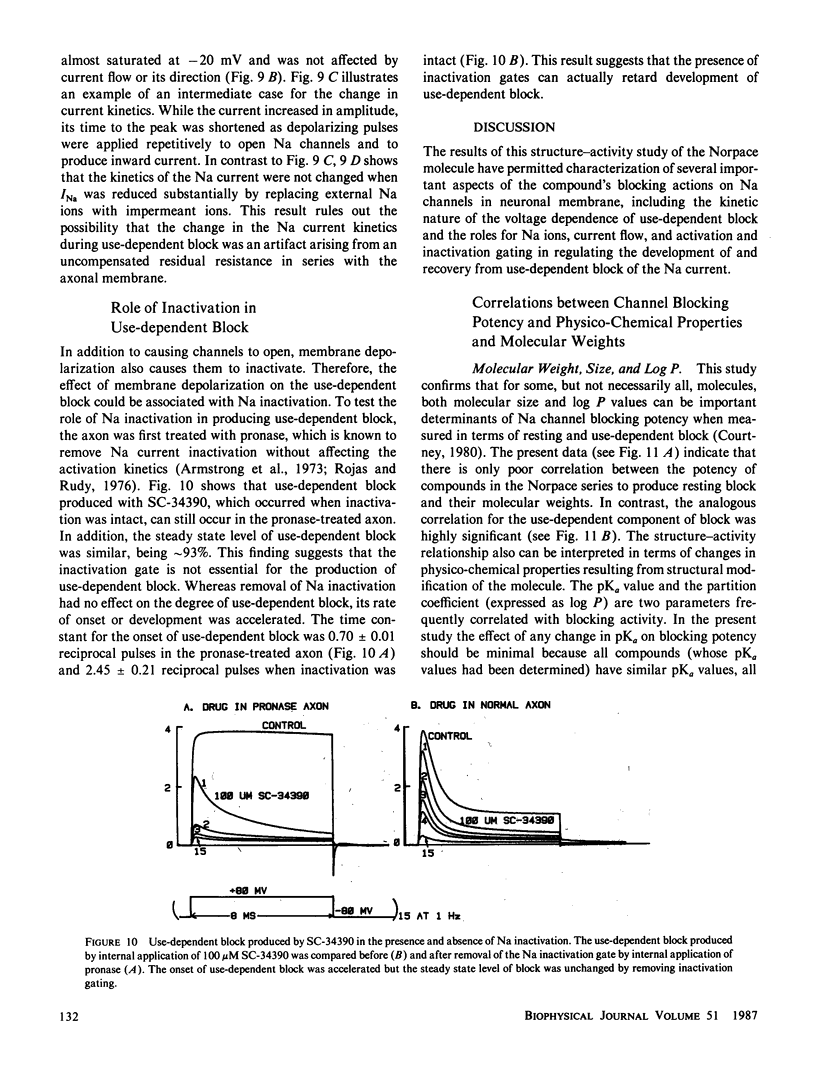
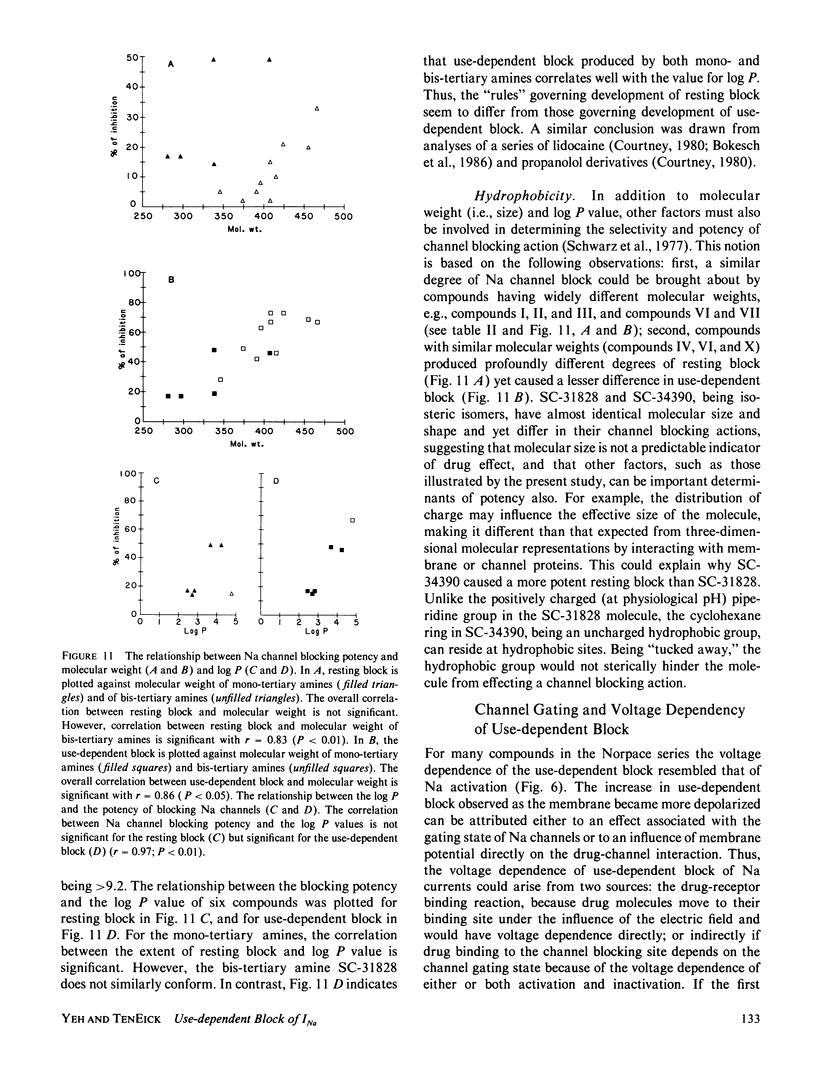
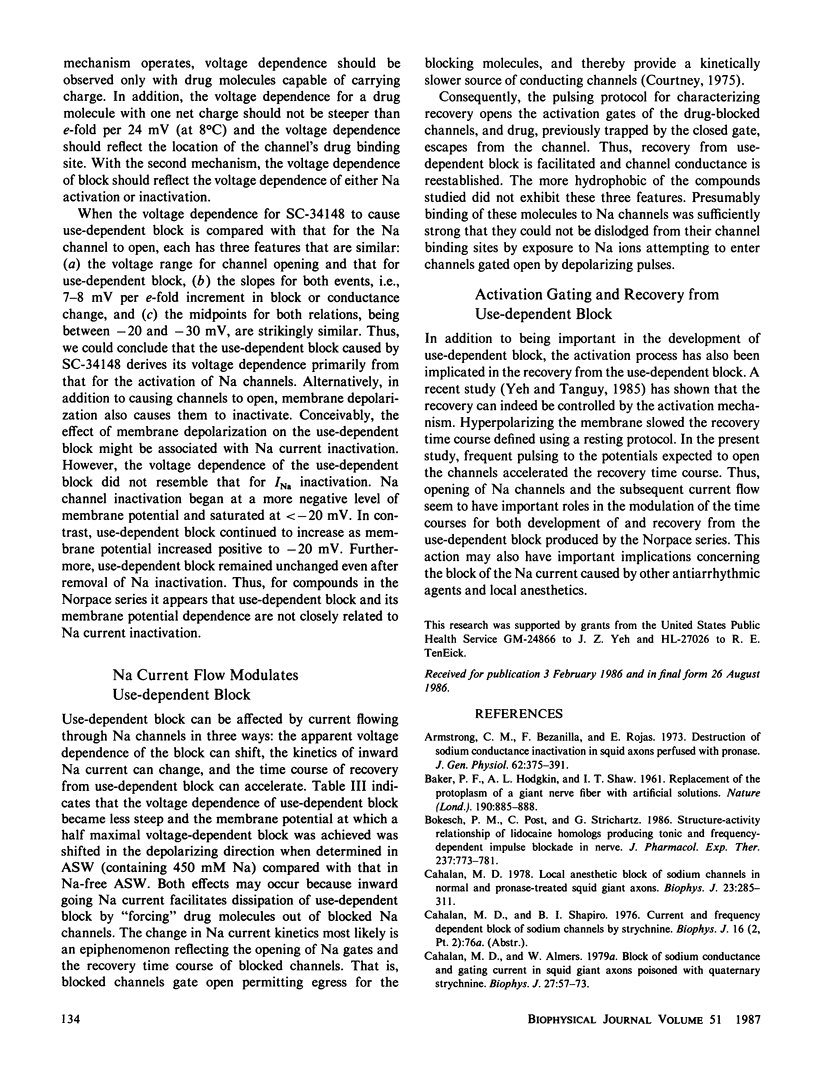
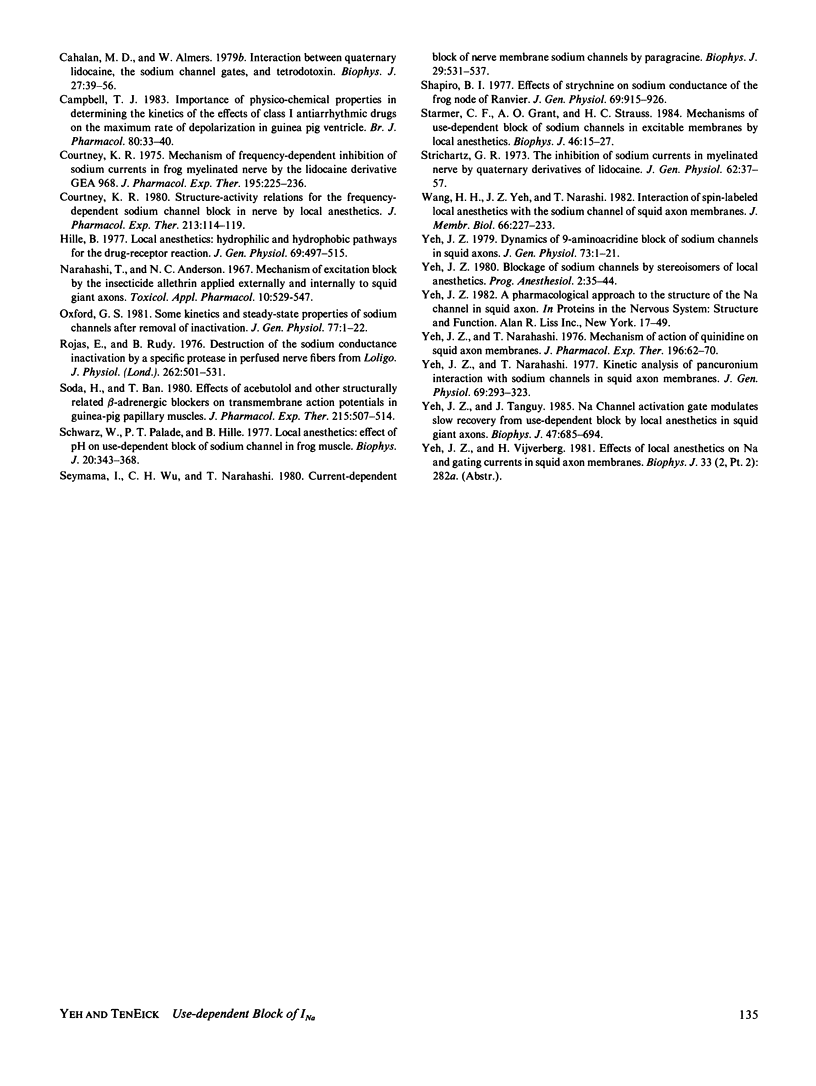
Selected References
These references are in PubMed. This may not be the complete list of references from this article.
- Armstrong C. M., Bezanilla F., Rojas E. Destruction of sodium conductance inactivation in squid axons perfused with pronase. J Gen Physiol. 1973 Oct;62(4):375–391. doi: 10.1085/jgp.62.4.375. [DOI] [PMC free article] [PubMed] [Google Scholar]
- BAKER P. F., HODGKIN A. L., SHAW T. I. Replacement of the protoplasm of a giant nerve fibre with artificial solutions. Nature. 1961 Jun 3;190:885–887. doi: 10.1038/190885a0. [DOI] [PubMed] [Google Scholar]
- Bokesch P. M., Post C., Strichartz G. Structure-activity relationship of lidocaine homologs producing tonic and frequency-dependent impulse blockade in nerve. J Pharmacol Exp Ther. 1986 Jun;237(3):773–781. [PubMed] [Google Scholar]
- Cahalan M. D., Almers W. Block of sodium conductance and gating current in squid giant axons poisoned with quaternary strychnine. Biophys J. 1979 Jul;27(1):57–73. doi: 10.1016/S0006-3495(79)85202-9. [DOI] [PMC free article] [PubMed] [Google Scholar]
- Cahalan M. D., Almers W. Interactions between quaternary lidocaine, the sodium channel gates, and tetrodotoxin. Biophys J. 1979 Jul;27(1):39–55. doi: 10.1016/S0006-3495(79)85201-7. [DOI] [PMC free article] [PubMed] [Google Scholar]
- Cahalan M. D. Local anesthetic block of sodium channels in normal and pronase-treated squid giant axons. Biophys J. 1978 Aug;23(2):285–311. doi: 10.1016/S0006-3495(78)85449-6. [DOI] [PMC free article] [PubMed] [Google Scholar]
- Campbell T. J. Importance of physico-chemical properties in determining the kinetics of the effects of Class I antiarrhythmic drugs on maximum rate of depolarization in guinea-pig ventricle. Br J Pharmacol. 1983 Sep;80(1):33–40. doi: 10.1111/j.1476-5381.1983.tb11046.x. [DOI] [PMC free article] [PubMed] [Google Scholar]
- Courtney K. R. Mechanism of frequency-dependent inhibition of sodium currents in frog myelinated nerve by the lidocaine derivative GEA. J Pharmacol Exp Ther. 1975 Nov;195(2):225–236. [PubMed] [Google Scholar]
- Courtney K. R. Structure-activity relations for frequency-dependent sodium channel block in nerve by local anesthetics. J Pharmacol Exp Ther. 1980 Apr;213(1):114–119. [PubMed] [Google Scholar]
- Hille B. Local anesthetics: hydrophilic and hydrophobic pathways for the drug-receptor reaction. J Gen Physiol. 1977 Apr;69(4):497–515. doi: 10.1085/jgp.69.4.497. [DOI] [PMC free article] [PubMed] [Google Scholar]
- Narahashi T., Anderson N. C. Mechanism of excitation block by the insecticide allethrin applied externally and internally to squid giant axons. Toxicol Appl Pharmacol. 1967 May;10(3):529–547. doi: 10.1016/0041-008x(67)90092-0. [DOI] [PubMed] [Google Scholar]
- Oxford G. S. Some kinetic and steady-state properties of sodium channels after removal of inactivation. J Gen Physiol. 1981 Jan;77(1):1–22. doi: 10.1085/jgp.77.1.1. [DOI] [PMC free article] [PubMed] [Google Scholar]
- Rojas E., Rudy B. Destruction of the sodium conductance inactivation by a specific protease in perfused nerve fibres from Loligo. J Physiol. 1976 Nov;262(2):501–531. doi: 10.1113/jphysiol.1976.sp011608. [DOI] [PMC free article] [PubMed] [Google Scholar]
- Sada H., Ban T. Effects of acebutolol and other structurally related beta adrenergic blockers on transmembrane action potential in guinea-pig papillary muscles. J Pharmacol Exp Ther. 1980 Nov;215(2):507–514. [PubMed] [Google Scholar]
- Schwarz W., Palade P. T., Hille B. Local anesthetics. Effect of pH on use-dependent block of sodium channels in frog muscle. Biophys J. 1977 Dec;20(3):343–368. doi: 10.1016/S0006-3495(77)85554-9. [DOI] [PMC free article] [PubMed] [Google Scholar]
- Seyama I., Wu C. H., Narahashi T. Current-dependent block of nerve membrane sodium channels by paragracine. Biophys J. 1980 Mar;29(3):531–537. doi: 10.1016/S0006-3495(80)85151-4. [DOI] [PMC free article] [PubMed] [Google Scholar]
- Shapiro B. I. Effects of strychnine on the sodium conductance of the frog node of Ranvier. J Gen Physiol. 1977 Jun;69(6):915–926. doi: 10.1085/jgp.69.6.915. [DOI] [PMC free article] [PubMed] [Google Scholar]
- Starmer C. F., Grant A. O., Strauss H. C. Mechanisms of use-dependent block of sodium channels in excitable membranes by local anesthetics. Biophys J. 1984 Jul;46(1):15–27. doi: 10.1016/S0006-3495(84)83994-6. [DOI] [PMC free article] [PubMed] [Google Scholar]
- Strichartz G. R. The inhibition of sodium currents in myelinated nerve by quaternary derivatives of lidocaine. J Gen Physiol. 1973 Jul;62(1):37–57. doi: 10.1085/jgp.62.1.37. [DOI] [PMC free article] [PubMed] [Google Scholar]
- Wang H. H., Yeh J. Z., Narahashi T. Interaction of spin-labeled local anesthetics with the sodium channel of squid axon membranes. J Membr Biol. 1982;66(3):227–233. doi: 10.1007/BF01868497. [DOI] [PubMed] [Google Scholar]
- Yeh J. Z. A pharmacological approach to the structure of the Na channel in squid axon. Prog Clin Biol Res. 1982;79:17–49. [PubMed] [Google Scholar]
- Yeh J. Z. Dynamics of 9-aminoacridine block of sodium channels in squid axons. J Gen Physiol. 1979 Jan;73(1):1–21. doi: 10.1085/jgp.73.1.1. [DOI] [PMC free article] [PubMed] [Google Scholar]
- Yeh J. Z., Narahashi T. Kinetic analysis of pancuronium interaction with sodium channels in squid axon membranes. J Gen Physiol. 1977 Mar;69(3):293–323. doi: 10.1085/jgp.69.3.293. [DOI] [PMC free article] [PubMed] [Google Scholar]
- Yeh J. Z., Narahashi T. Mechanism of action of quinidine on squid axon membranes. J Pharmacol Exp Ther. 1976 Jan;196(1):62–70. [PubMed] [Google Scholar]
- Yeh J. Z., Tanguy J. Na channel activation gate modulates slow recovery from use-dependent block by local anesthetics in squid giant axons. Biophys J. 1985 May;47(5):685–694. doi: 10.1016/S0006-3495(85)83965-5. [DOI] [PMC free article] [PubMed] [Google Scholar]