Abstract
Small, random length changes were applied to bundles of intact fibers from rat and mouse extensor digitorum longus (EDL) and soleus muscles, while they were being tetanically stimulated. With increasing frequency of length changes, EDL muscle stiffness (tension change per unit change in length) increased, then decreased and increased again. The decrease was not seen in the soleus muscles. The EDL frequency-response could be well fitted by three exponential components with apparent rate constants of approximately 25, 150, and 500 s-1 at 20 degrees C. All rate constants increased steadily with temperature and for each 10 degrees C increase in temperature, the rates in the mouse EDL increased by a factor (Q10) between 1.8 and 2.4. With tetanic stimulation, force increased nearly exponentially to a steady level with a rate constant of 24 s-1 at 20 degrees C in mouse EDL muscles, and a Q10 of 2.4. These values correspond closely to the lowest frequency rate constant measured with length perturbations, which suggests that this process may limit the rate of rise of force in intact muscle fibers. During fatigue the high frequency and intermediate frequency rate constants declined, but the low frequency rate constant remained unchanged. These results are discussed in relation to current biochemical models for cross-bridge cycling.
Full text
PDF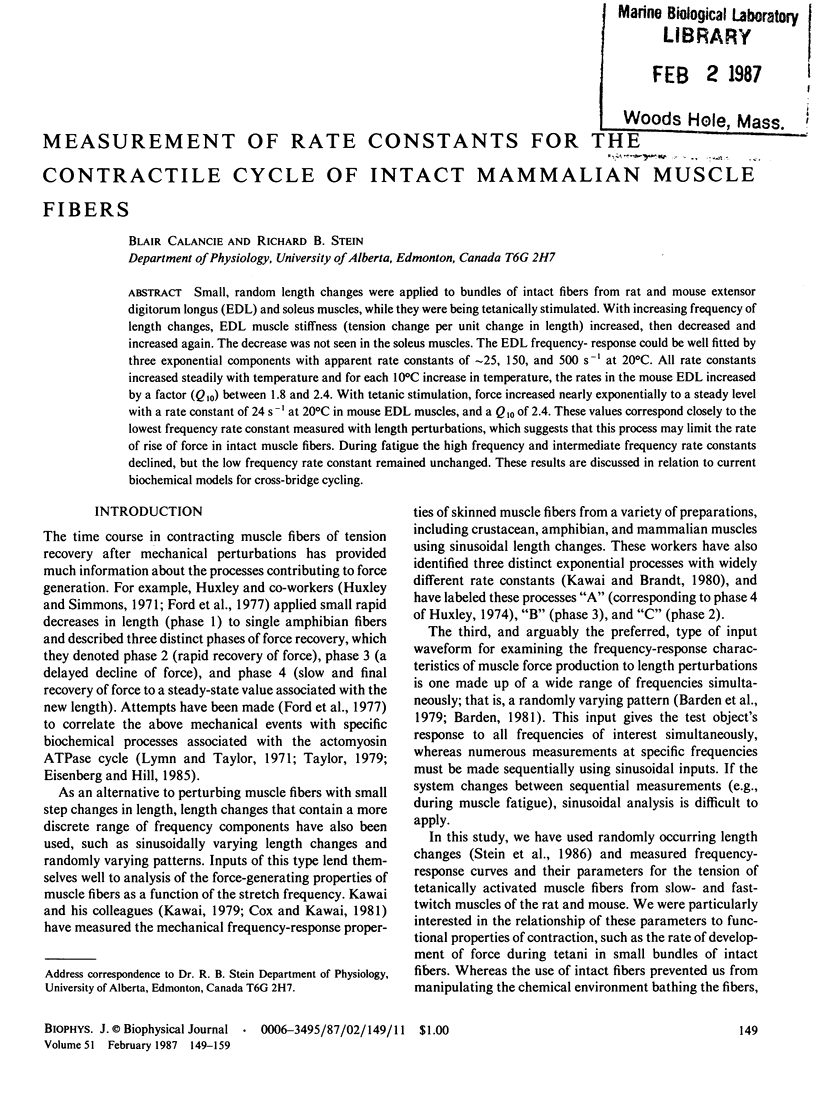
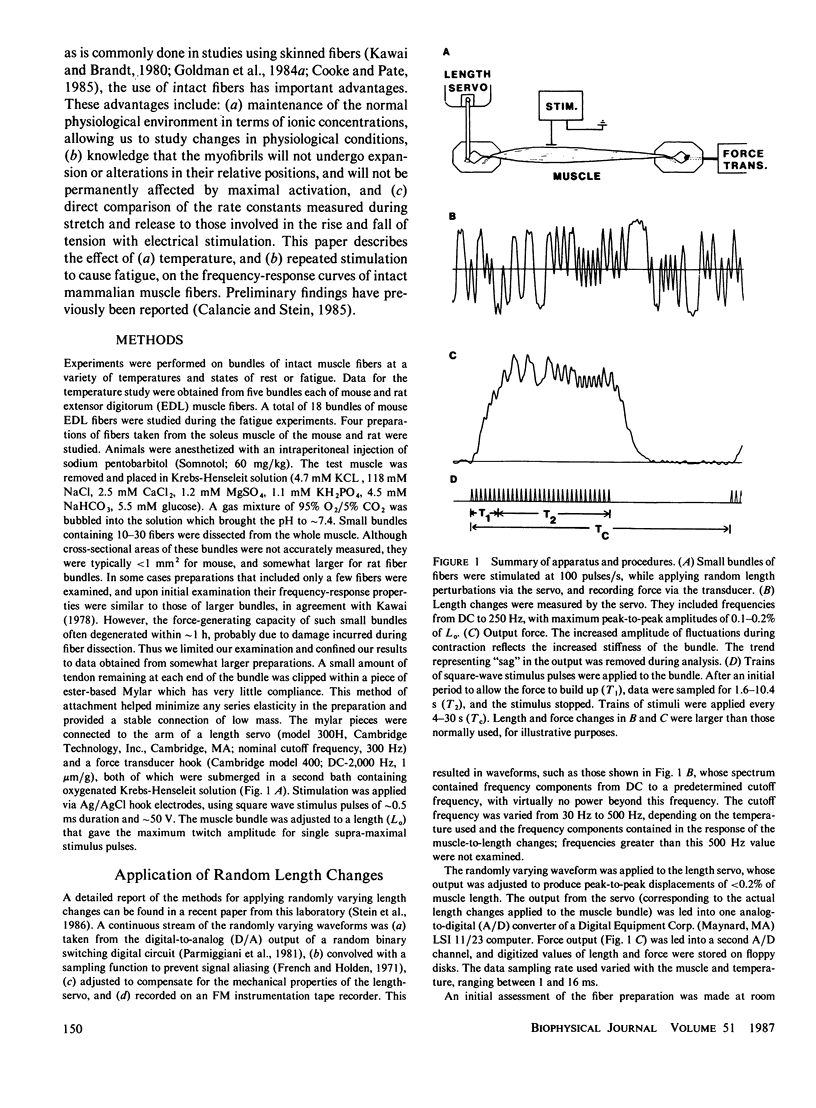
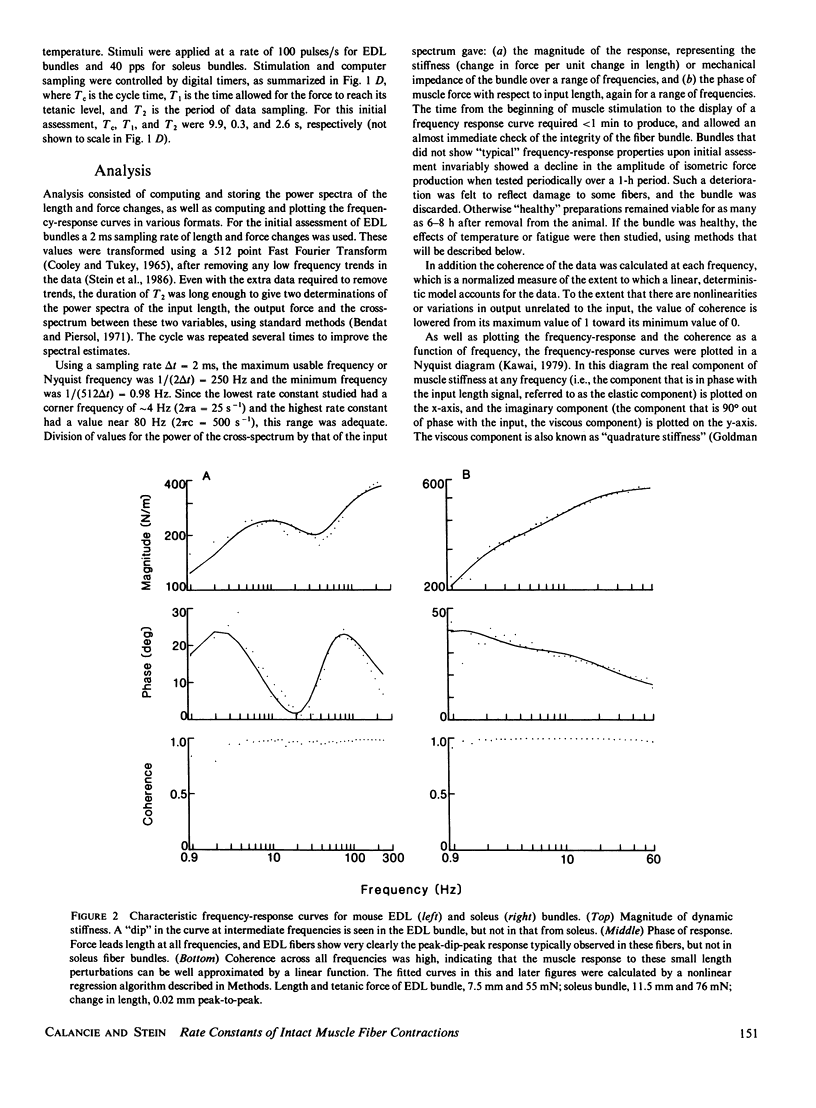
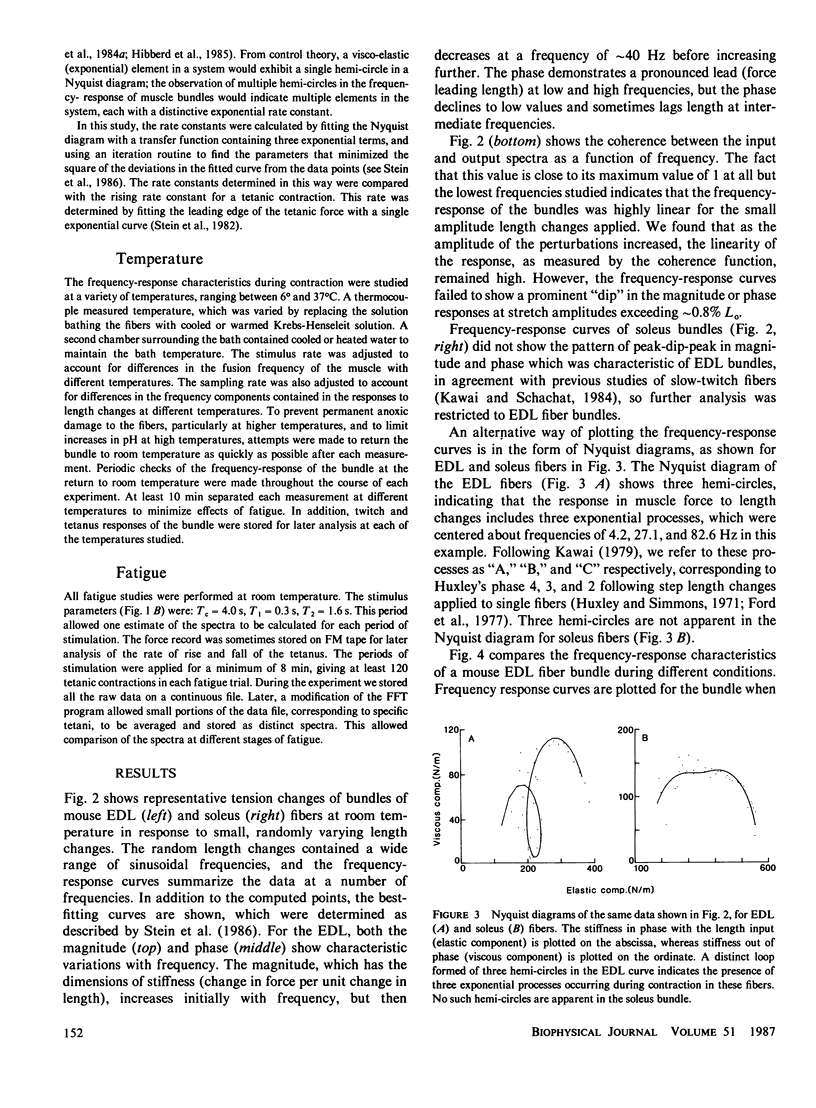
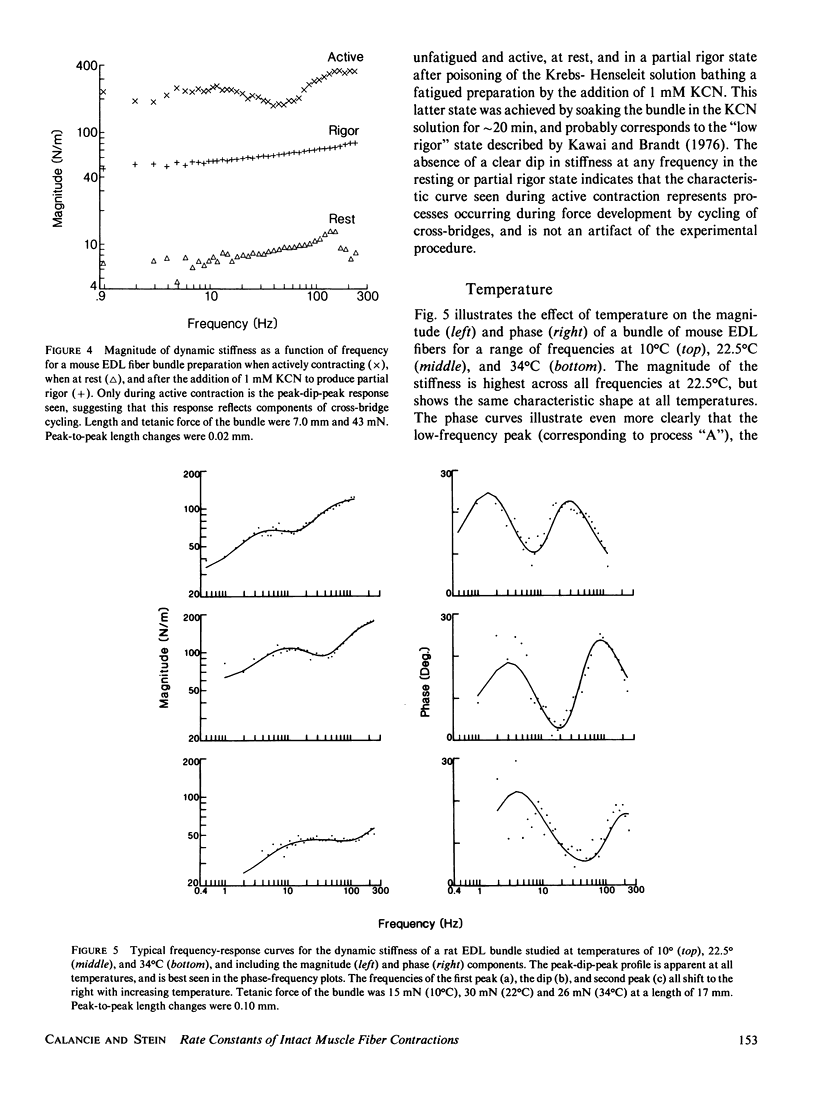
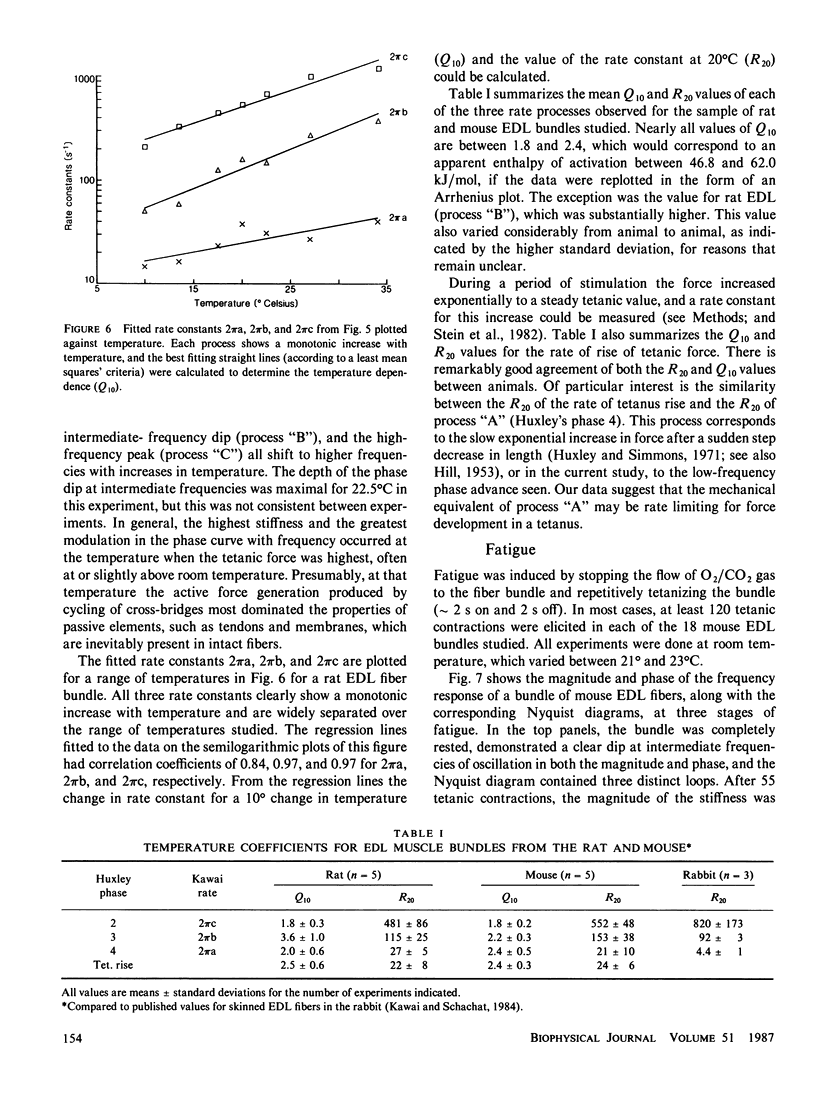
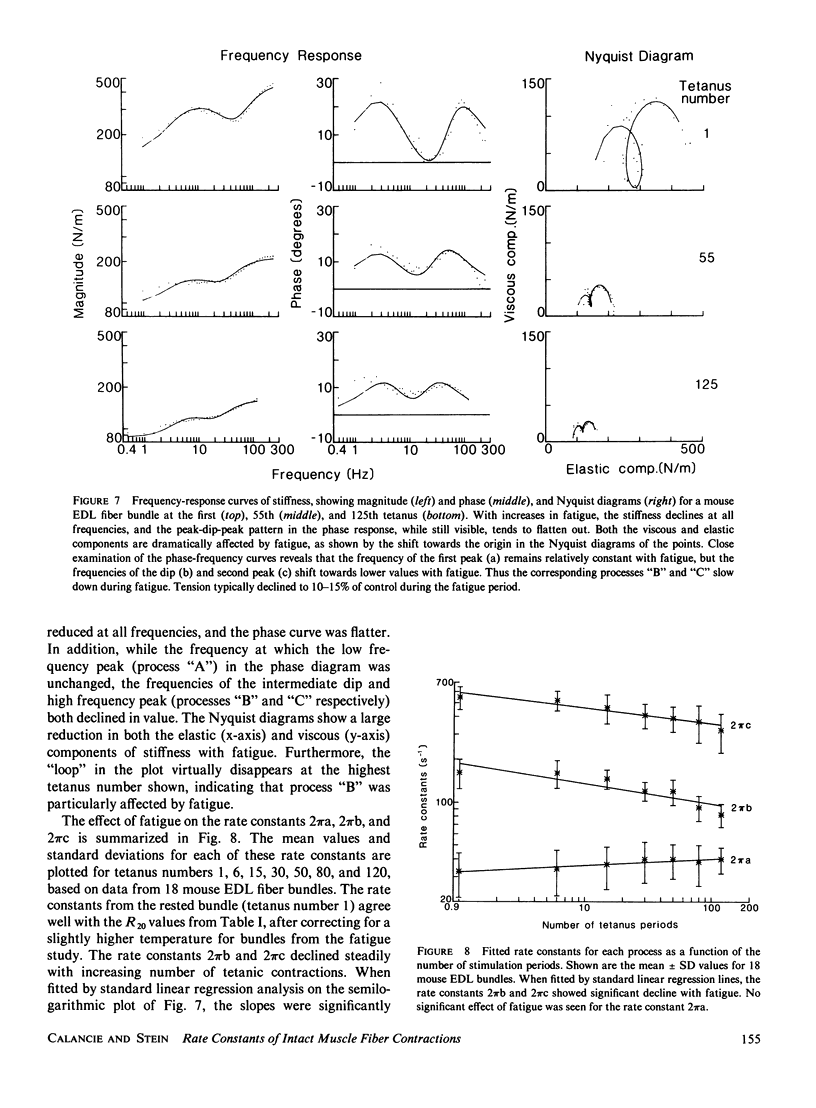
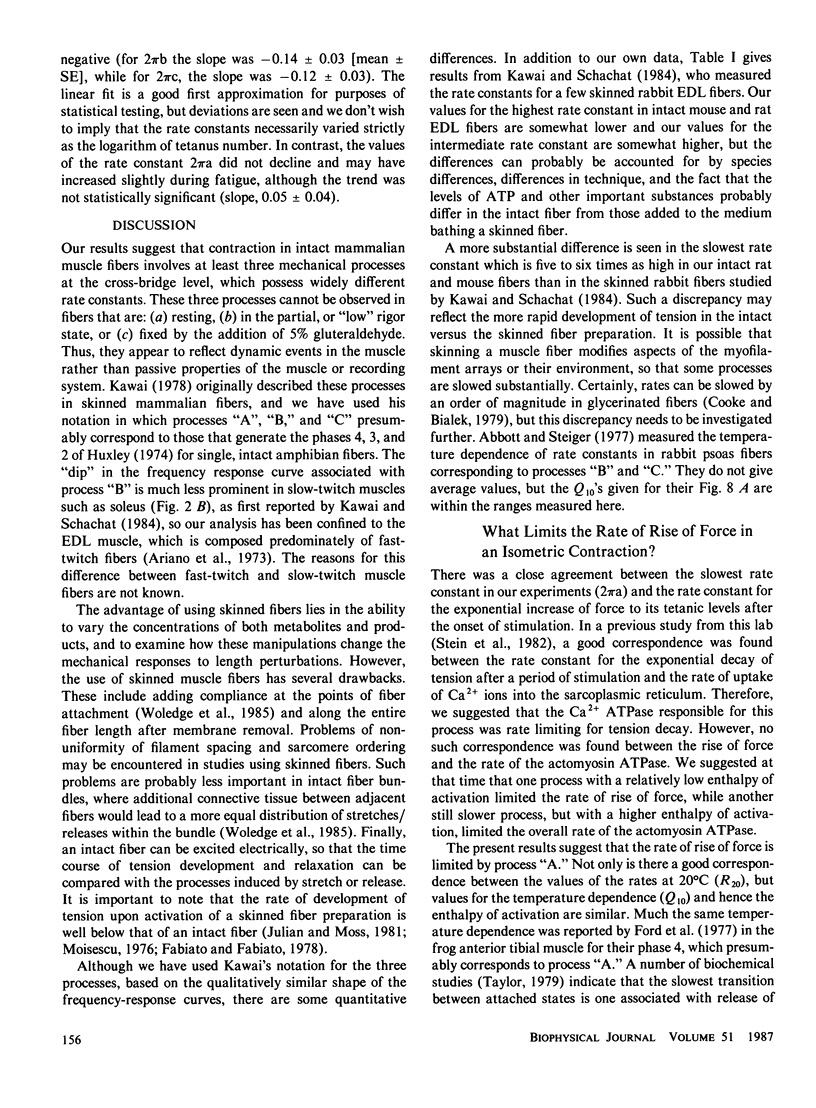
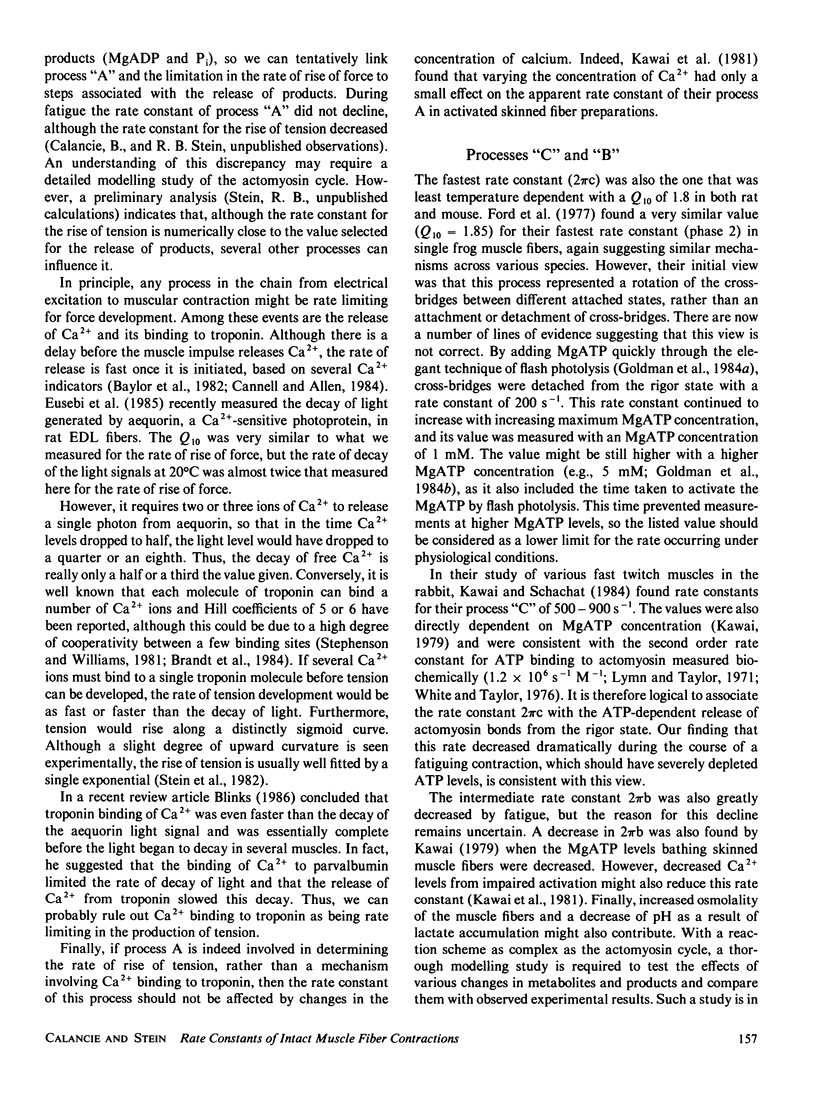
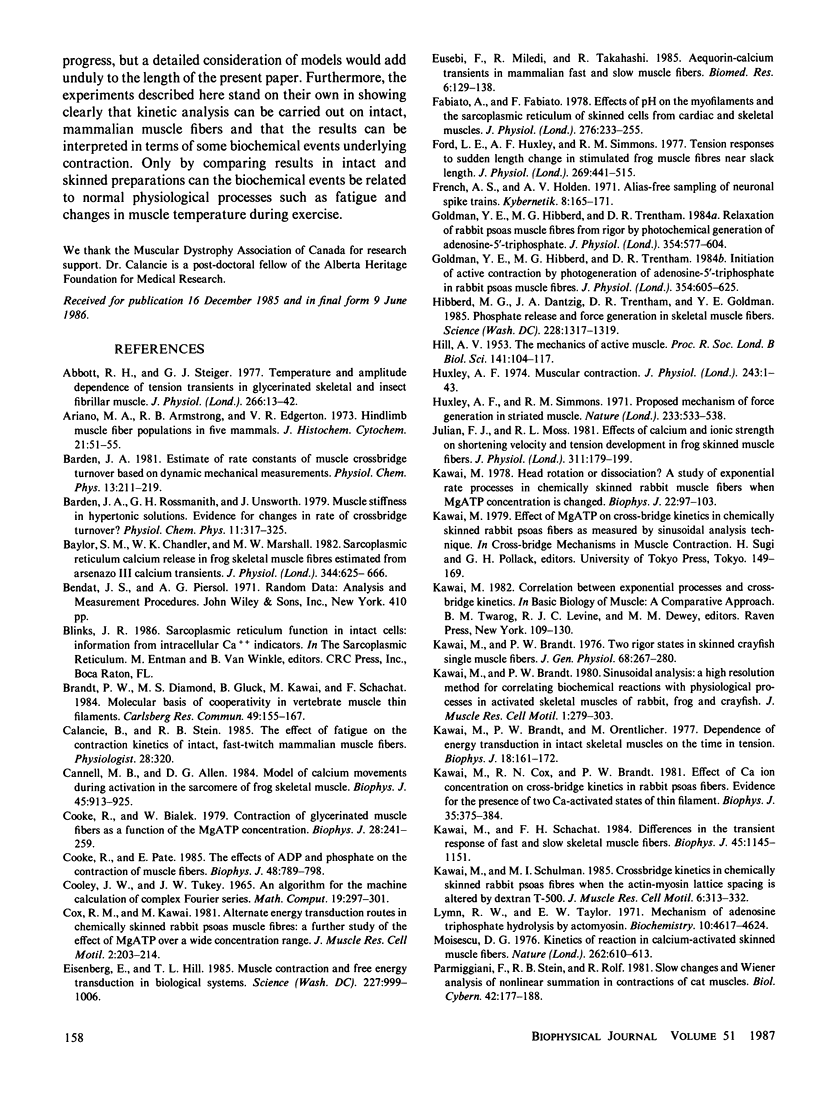
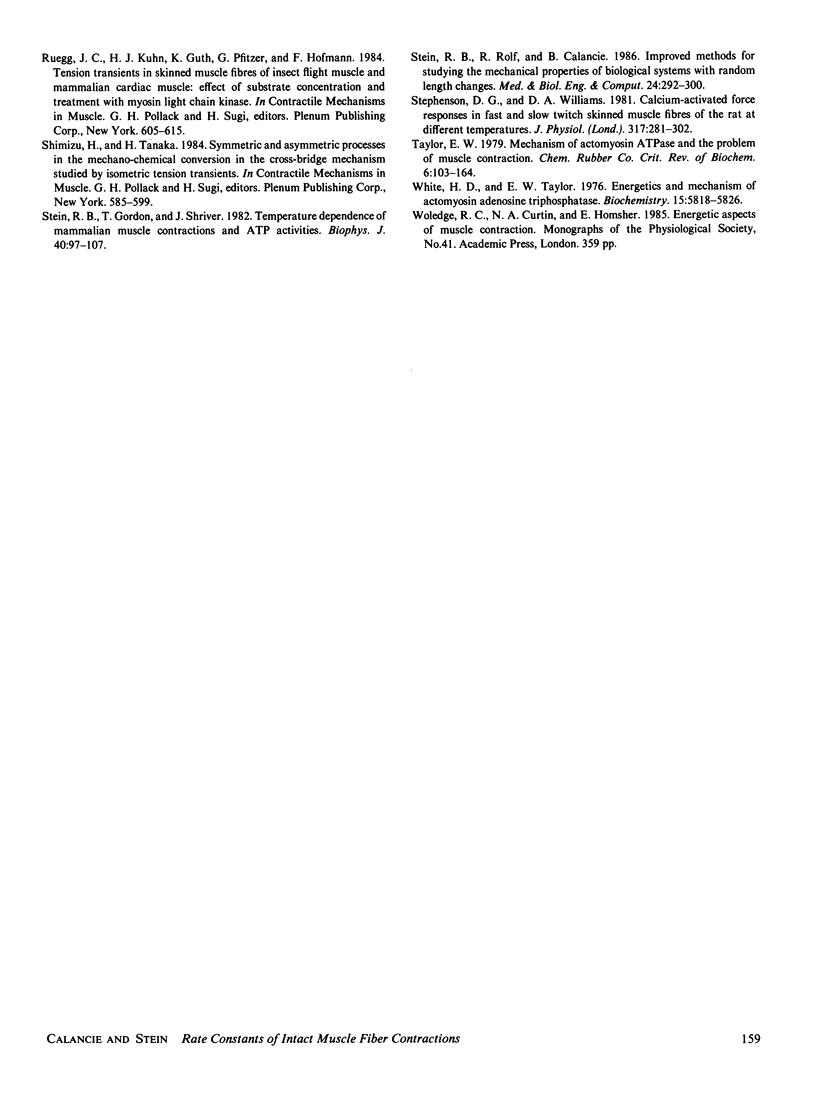
Selected References
These references are in PubMed. This may not be the complete list of references from this article.
- Abbott R. H., Steiger G. J. Temperature and amplitude dependence of tension transients in glycerinated skeletal and insect fibrillar muscle. J Physiol. 1977 Mar;266(1):13–42. doi: 10.1113/jphysiol.1977.sp011754. [DOI] [PMC free article] [PubMed] [Google Scholar]
- Ariano M. A., Armstrong R. B., Edgerton V. R. Hindlimb muscle fiber populations of five mammals. J Histochem Cytochem. 1973 Jan;21(1):51–55. doi: 10.1177/21.1.51. [DOI] [PubMed] [Google Scholar]
- Barden J. A. Estimate of rate constants of muscle crossbridge turnover based on dynamic mechanical measurements. Physiol Chem Phys. 1981;13(3):211–219. [PubMed] [Google Scholar]
- Baylor S. M., Chandler W. K., Marshall M. W. Sarcoplasmic reticulum calcium release in frog skeletal muscle fibres estimated from Arsenazo III calcium transients. J Physiol. 1983 Nov;344:625–666. doi: 10.1113/jphysiol.1983.sp014959. [DOI] [PMC free article] [PubMed] [Google Scholar]
- Cannell M. B., Allen D. G. Model of calcium movements during activation in the sarcomere of frog skeletal muscle. Biophys J. 1984 May;45(5):913–925. doi: 10.1016/S0006-3495(84)84238-1. [DOI] [PMC free article] [PubMed] [Google Scholar]
- Cooke R., Bialek W. Contraction of glycerinated muscle fibers as a function of the ATP concentration. Biophys J. 1979 Nov;28(2):241–258. doi: 10.1016/S0006-3495(79)85174-7. [DOI] [PMC free article] [PubMed] [Google Scholar]
- Cooke R., Pate E. The effects of ADP and phosphate on the contraction of muscle fibers. Biophys J. 1985 Nov;48(5):789–798. doi: 10.1016/S0006-3495(85)83837-6. [DOI] [PMC free article] [PubMed] [Google Scholar]
- Cox R. N., Kawai M. Alternate energy transduction routes in chemically skinned rabbit psoas muscle fibres: a further study of the effect of MgATP over a wide concentration range. J Muscle Res Cell Motil. 1981 Jun;2(2):203–214. doi: 10.1007/BF00711870. [DOI] [PubMed] [Google Scholar]
- Eisenberg E., Hill T. L. Muscle contraction and free energy transduction in biological systems. Science. 1985 Mar 1;227(4690):999–1006. doi: 10.1126/science.3156404. [DOI] [PubMed] [Google Scholar]
- Fabiato A., Fabiato F. Effects of pH on the myofilaments and the sarcoplasmic reticulum of skinned cells from cardiace and skeletal muscles. J Physiol. 1978 Mar;276:233–255. doi: 10.1113/jphysiol.1978.sp012231. [DOI] [PMC free article] [PubMed] [Google Scholar]
- Ford L. E., Huxley A. F., Simmons R. M. Tension responses to sudden length change in stimulated frog muscle fibres near slack length. J Physiol. 1977 Jul;269(2):441–515. doi: 10.1113/jphysiol.1977.sp011911. [DOI] [PMC free article] [PubMed] [Google Scholar]
- French A. S., Holden A. V. Alias-free sampling of neuronal spike trains. Kybernetik. 1971 May;8(5):165–171. doi: 10.1007/BF00291117. [DOI] [PubMed] [Google Scholar]
- Goldman Y. E., Hibberd M. G., Trentham D. R. Initiation of active contraction by photogeneration of adenosine-5'-triphosphate in rabbit psoas muscle fibres. J Physiol. 1984 Sep;354:605–624. doi: 10.1113/jphysiol.1984.sp015395. [DOI] [PMC free article] [PubMed] [Google Scholar]
- Goldman Y. E., Hibberd M. G., Trentham D. R. Relaxation of rabbit psoas muscle fibres from rigor by photochemical generation of adenosine-5'-triphosphate. J Physiol. 1984 Sep;354:577–604. doi: 10.1113/jphysiol.1984.sp015394. [DOI] [PMC free article] [PubMed] [Google Scholar]
- HILL A. V. The mechanics of active muscle. Proc R Soc Lond B Biol Sci. 1953 Mar 11;141(902):104–117. doi: 10.1098/rspb.1953.0027. [DOI] [PubMed] [Google Scholar]
- Hibberd M. G., Dantzig J. A., Trentham D. R., Goldman Y. E. Phosphate release and force generation in skeletal muscle fibers. Science. 1985 Jun 14;228(4705):1317–1319. doi: 10.1126/science.3159090. [DOI] [PubMed] [Google Scholar]
- Huxley A. F., Simmons R. M. Proposed mechanism of force generation in striated muscle. Nature. 1971 Oct 22;233(5321):533–538. doi: 10.1038/233533a0. [DOI] [PubMed] [Google Scholar]
- Julian F. J., Moss R. L. Effects of calcium and ionic strength on shortening velocity and tension development in frog skinned muscle fibres. J Physiol. 1981 Feb;311:179–199. doi: 10.1113/jphysiol.1981.sp013580. [DOI] [PMC free article] [PubMed] [Google Scholar]
- Kawai M., Brandt P. W. Sinusoidal analysis: a high resolution method for correlating biochemical reactions with physiological processes in activated skeletal muscles of rabbit, frog and crayfish. J Muscle Res Cell Motil. 1980 Sep;1(3):279–303. doi: 10.1007/BF00711932. [DOI] [PubMed] [Google Scholar]
- Kawai M., Brandt P. W. Two rigor states in skinned crayfish single muscle fibers. J Gen Physiol. 1976 Sep;68(3):267–280. doi: 10.1085/jgp.68.3.267. [DOI] [PMC free article] [PubMed] [Google Scholar]
- Kawai M., Brandt P., Orentlicher M. Dependence of energy transduction in intact skeletal muscles on the time in tension. Biophys J. 1977 May;18(2):161–172. doi: 10.1016/S0006-3495(77)85605-1. [DOI] [PMC free article] [PubMed] [Google Scholar]
- Kawai M., Cox R. N., Brandt P. W. Effect of Ca ion concentration on cross-bridge kinetics in rabbit psoas fibers. Evidence for the presence of two Ca-activated states of thin filament. Biophys J. 1981 Aug;35(2):375–384. doi: 10.1016/S0006-3495(81)84796-0. [DOI] [PMC free article] [PubMed] [Google Scholar]
- Kawai M. Head rotation or dissociation? A study of exponential rate processes in chemically skinned rabbit muscle fibers when MgATP concentration is changed. Biophys J. 1978 Apr;22(1):97–103. doi: 10.1016/S0006-3495(78)85473-3. [DOI] [PMC free article] [PubMed] [Google Scholar]
- Kawai M., Schachat F. H. Differences in the transient response of fast and slow skeletal muscle fibers. Correlations between complex modulus and myosin light chains. Biophys J. 1984 Jun;45(6):1145–1151. doi: 10.1016/S0006-3495(84)84262-9. [DOI] [PMC free article] [PubMed] [Google Scholar]
- Kawai M., Schulman M. I. Crossbridge kinetics in chemically skinned rabbit psoas fibres when the actin-myosin lattice spacing is altered by dextran T-500. J Muscle Res Cell Motil. 1985 Jun;6(3):313–332. doi: 10.1007/BF00713172. [DOI] [PubMed] [Google Scholar]
- Lymn R. W., Taylor E. W. Mechanism of adenosine triphosphate hydrolysis by actomyosin. Biochemistry. 1971 Dec 7;10(25):4617–4624. doi: 10.1021/bi00801a004. [DOI] [PubMed] [Google Scholar]
- Moisescu D. G. Kinetics of reaction in calcium-activated skinned muscle fibres. Nature. 1976 Aug 12;262(5569):610–613. doi: 10.1038/262610a0. [DOI] [PubMed] [Google Scholar]
- Parmiggiani F., Stein R. B., Rolf R. Slow changes and Wiener analysis of nonlinear summation in contractions in cat muscles. Biol Cybern. 1982;42(3):177–188. doi: 10.1007/BF00340074. [DOI] [PubMed] [Google Scholar]
- Rüegg J. C., Kuhn H. J., Güth K., Pfitzer G., Hofmann F. Tension transients in skinned muscle fibres of insect flight muscle and mammalian cardiac muscle: effect of substrate concentration and treatment with myosin light chain kinase. Adv Exp Med Biol. 1984;170:605–615. doi: 10.1007/978-1-4684-4703-3_56. [DOI] [PubMed] [Google Scholar]
- Shimizu H., Tanaka H. Symmetric and asymmetric processes in the mechano-chemical conversion in the cross-bridge mechanism studied by isometric tension transients. Adv Exp Med Biol. 1984;170:585–599. doi: 10.1007/978-1-4684-4703-3_54. [DOI] [PubMed] [Google Scholar]
- Stein R. B., Gordon T., Shriver J. Temperature dependence of mammalian muscle contractions and ATPase activities. Biophys J. 1982 Nov;40(2):97–107. doi: 10.1016/S0006-3495(82)84464-0. [DOI] [PMC free article] [PubMed] [Google Scholar]
- Stein R. B., Rolf R., Calancie B. Improved methods for studying the mechanical properties of biological systems with random length changes. Med Biol Eng Comput. 1986 May;24(3):292–300. doi: 10.1007/BF02441626. [DOI] [PubMed] [Google Scholar]
- Stephenson D. G., Williams D. A. Calcium-activated force responses in fast- and slow-twitch skinned muscle fibres of the rat at different temperatures. J Physiol. 1981 Aug;317:281–302. doi: 10.1113/jphysiol.1981.sp013825. [DOI] [PMC free article] [PubMed] [Google Scholar]
- Taylor E. W. Mechanism of actomyosin ATPase and the problem of muscle contraction. CRC Crit Rev Biochem. 1979;6(2):103–164. doi: 10.3109/10409237909102562. [DOI] [PubMed] [Google Scholar]
- White H. D., Taylor E. W. Energetics and mechanism of actomyosin adenosine triphosphatase. Biochemistry. 1976 Dec 28;15(26):5818–5826. doi: 10.1021/bi00671a020. [DOI] [PubMed] [Google Scholar]