Abstract
Electron paramagnetic resonance (EPR) spectroscopy of paramagnetic derivatives of ATP has been used to probe the angular distribution of myosin in glycerinated muscle fibers. Three nucleotide spin labels have been prepared with the nitroxide free radical moiety attached, via an ester linkage to either: the 2' or 3' positions of the ribose unit of ATP (SL-ATP), the 2' position of 3' deoxy ATP (2'SL-dATP), or the 3' position of 2' deoxy ATP (3'SL-dATP). In muscle fibers, these nucleotides are quickly hydrolyzed to their diphosphate forms. All three diphosphate analogues bind to the nucleotide site of myosin with similar affinities: rabbit psoas fibers, 7 X 10(3)/M; insect flight muscle, 5 X 10(3)/M; and rabbit soleus muscle, 2 X 10(4)/M. Analysis of the spectra showed that the principal z-axis of the nitroxide attached to bound nucleotides was oriented with respect to the filament axis. The principal axes of 3'SL-dADP and 2'SL-dADP appeared to be preferentially aligned at mean angles of 67 degrees +/- 4 degrees and 55 degrees +/- 5 degrees, respectively. The distribution of probes about these angles can be described by Gaussians with widths of 16 degrees +/- 4 degrees and 13 degrees +/- 5 degrees, respectively. The spectrum of bound SL-ADP was a linear combination of the spectra of the two deoxy analogues. These orientations were the same in the three muscle types examined, indicating a high degree of homology in the nucleotide binding site. Applying static strains as high as 0.2 N/mm2 to muscle fibers caused no change in the orientation of myosin-bound, spin-labeled nucleotides. When muscle fibers were stretched to decrease actin and myosin filament overlap, bound SL-ADP produced EPR spectra indicative of probes with a highly disordered angular distribution. Sodium vanadate and SL-ATP caused fiber stiffness to decrease, and the EPR spectrum of the bound analogue indicated an increase in the fraction of disoriented probes with a concomitant decrease in the fraction of oriented probes. These findings indicate that when myosin is bound to actin its nucleotide site is highly oriented relative to the fiber axis, and when this interaction is removed the orientation of the nucleotide site becomes highly disordered.
Full text
PDF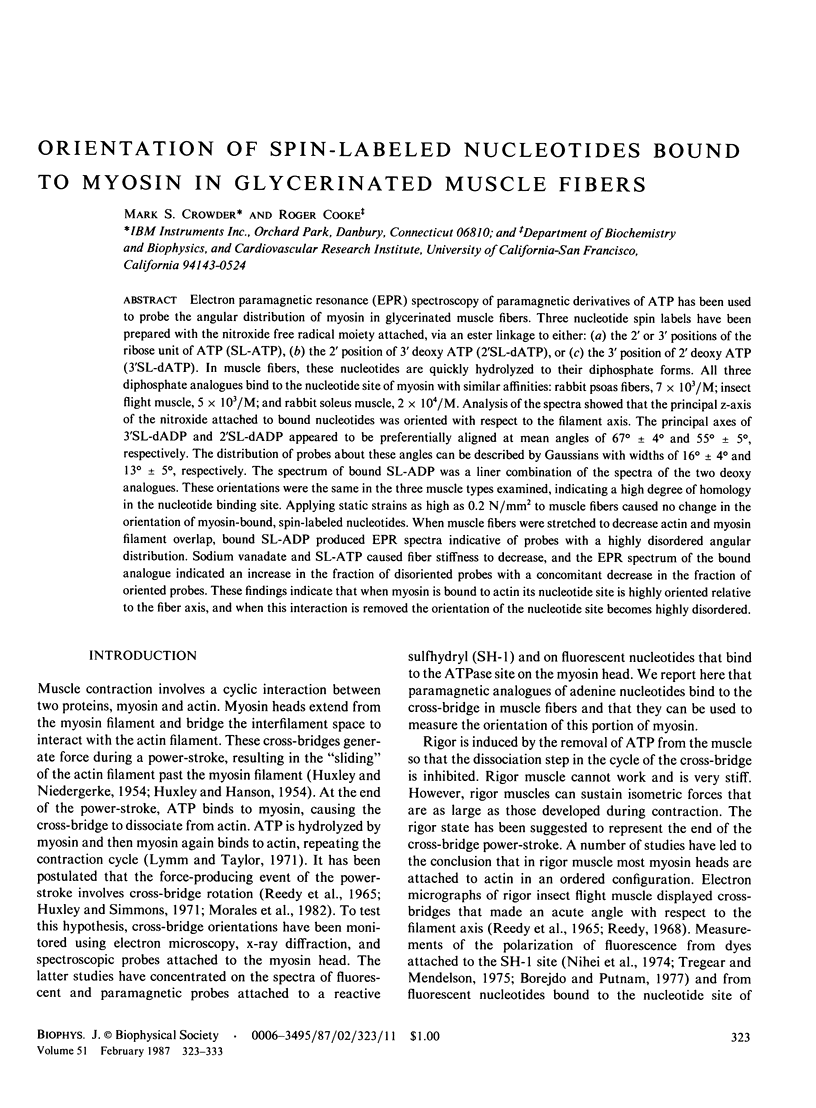
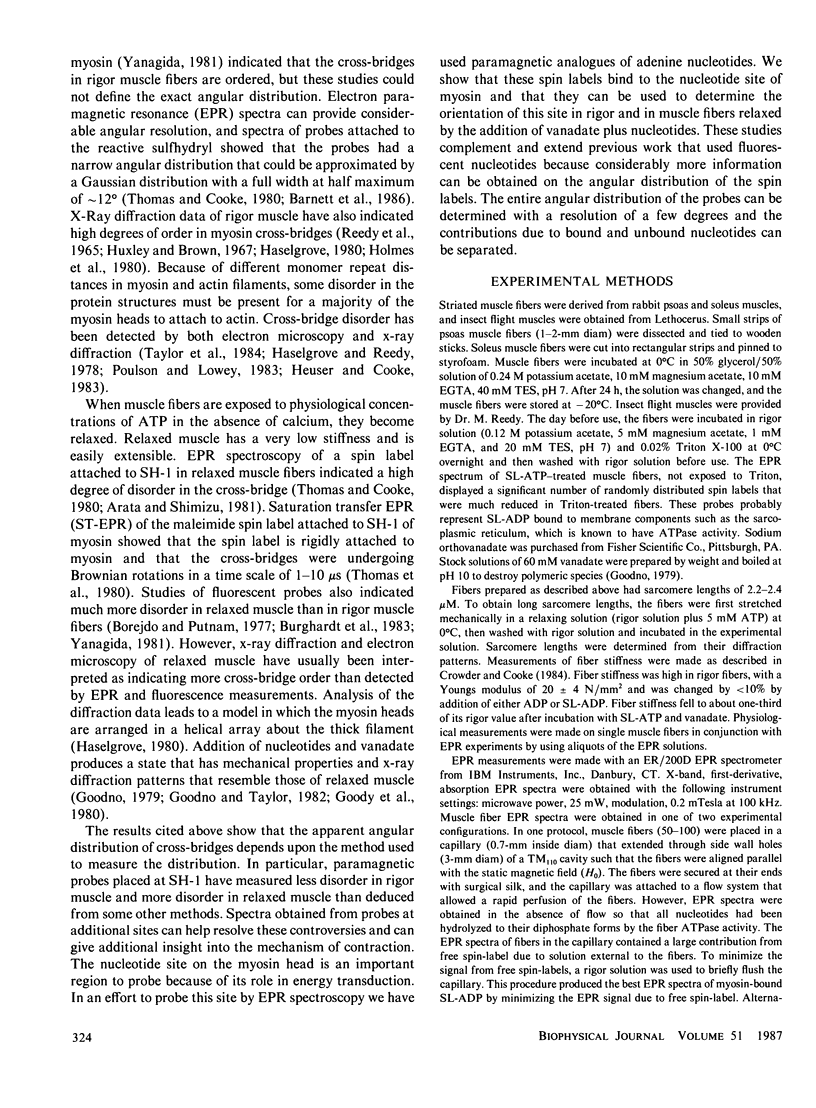
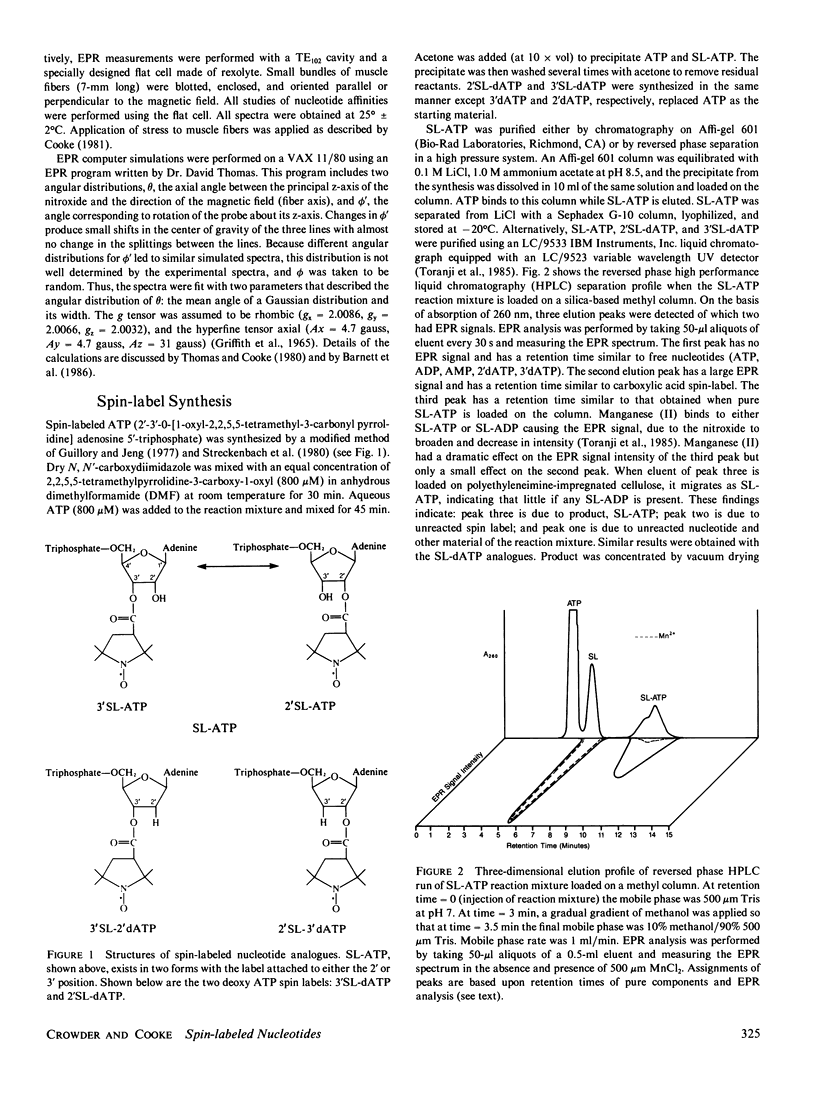
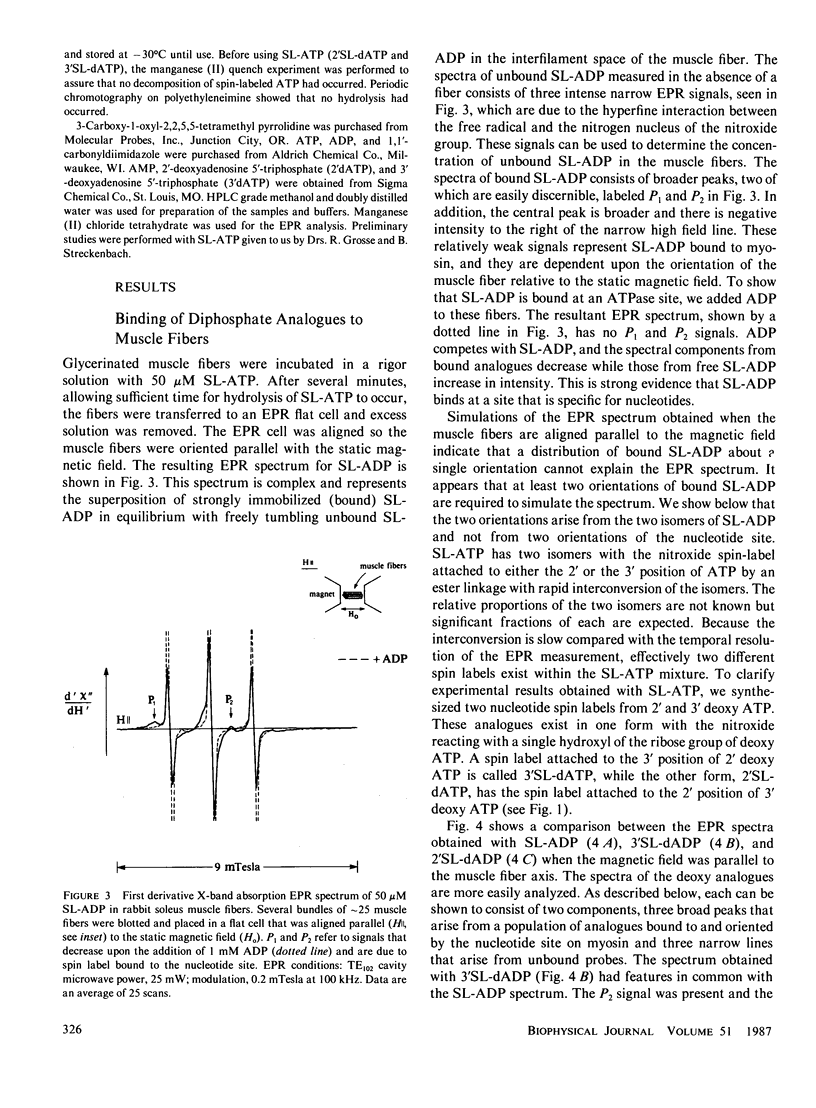
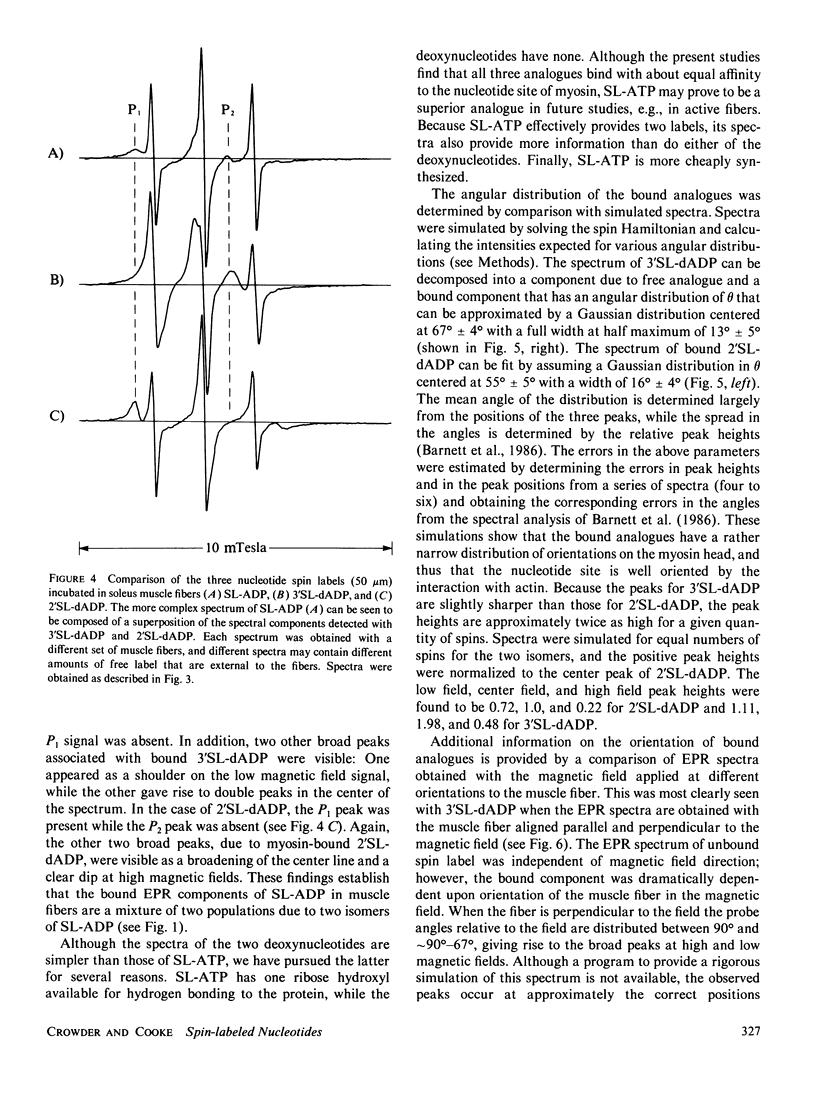
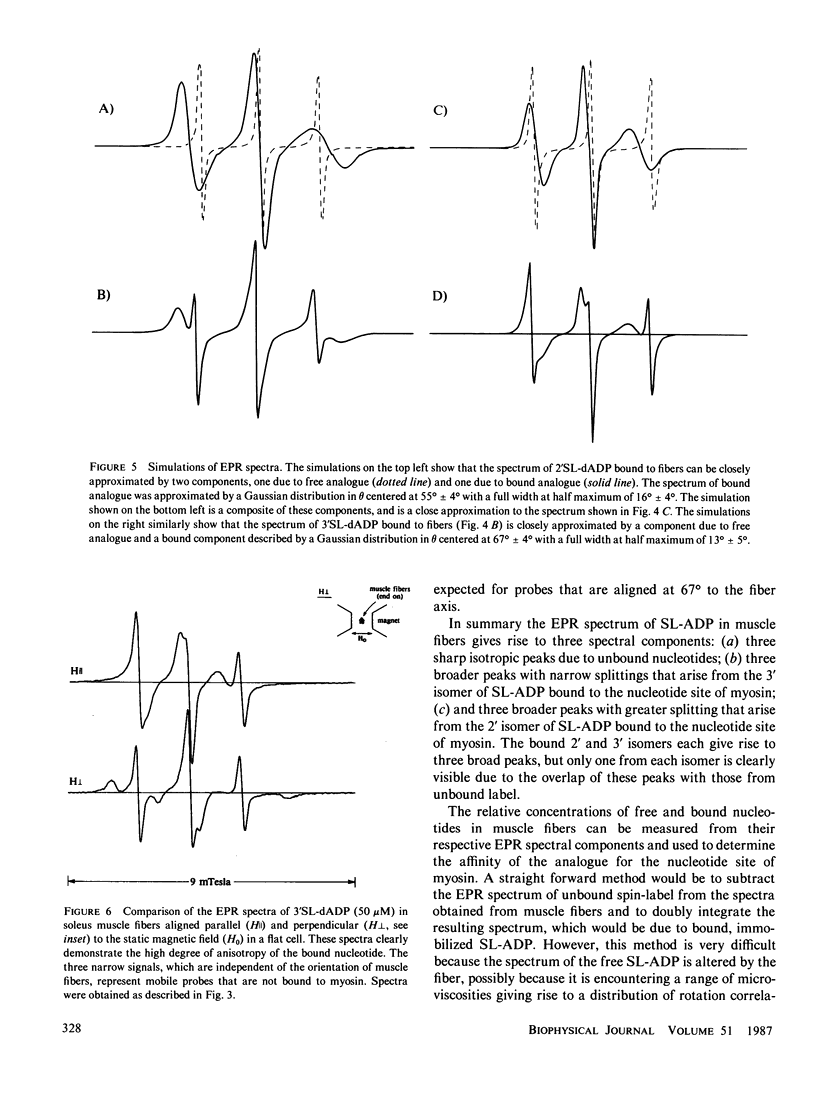
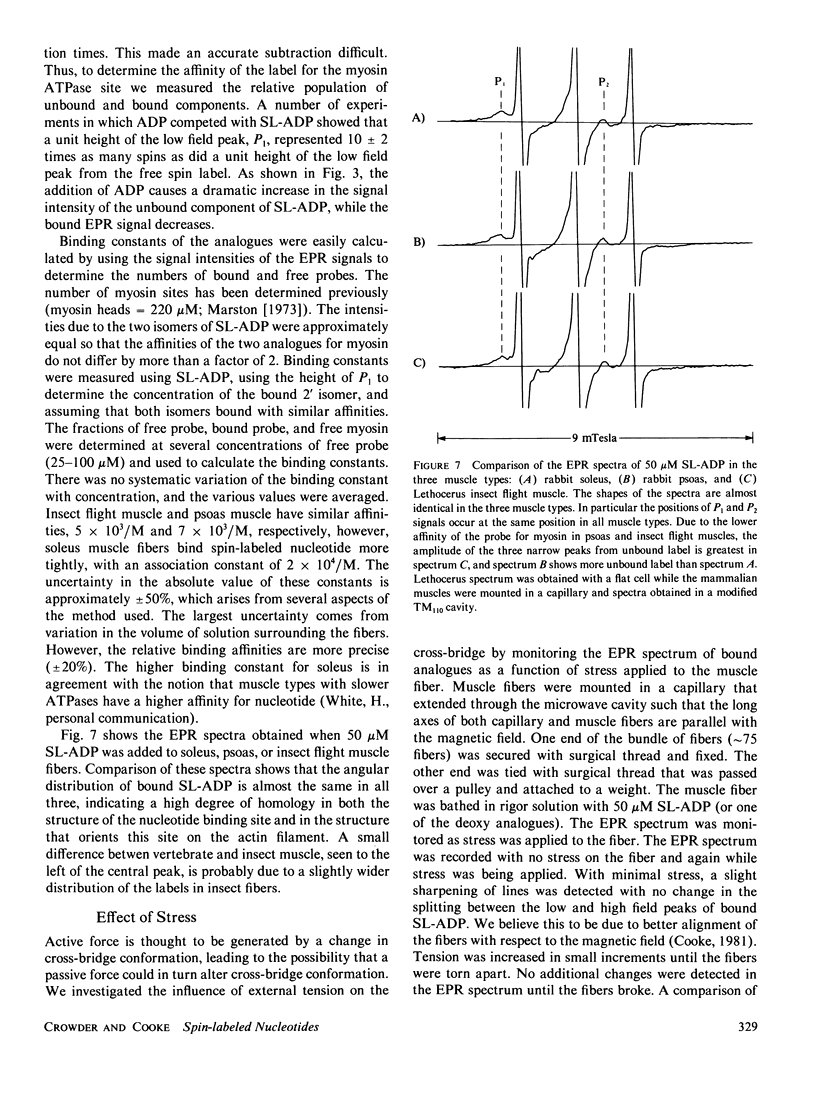
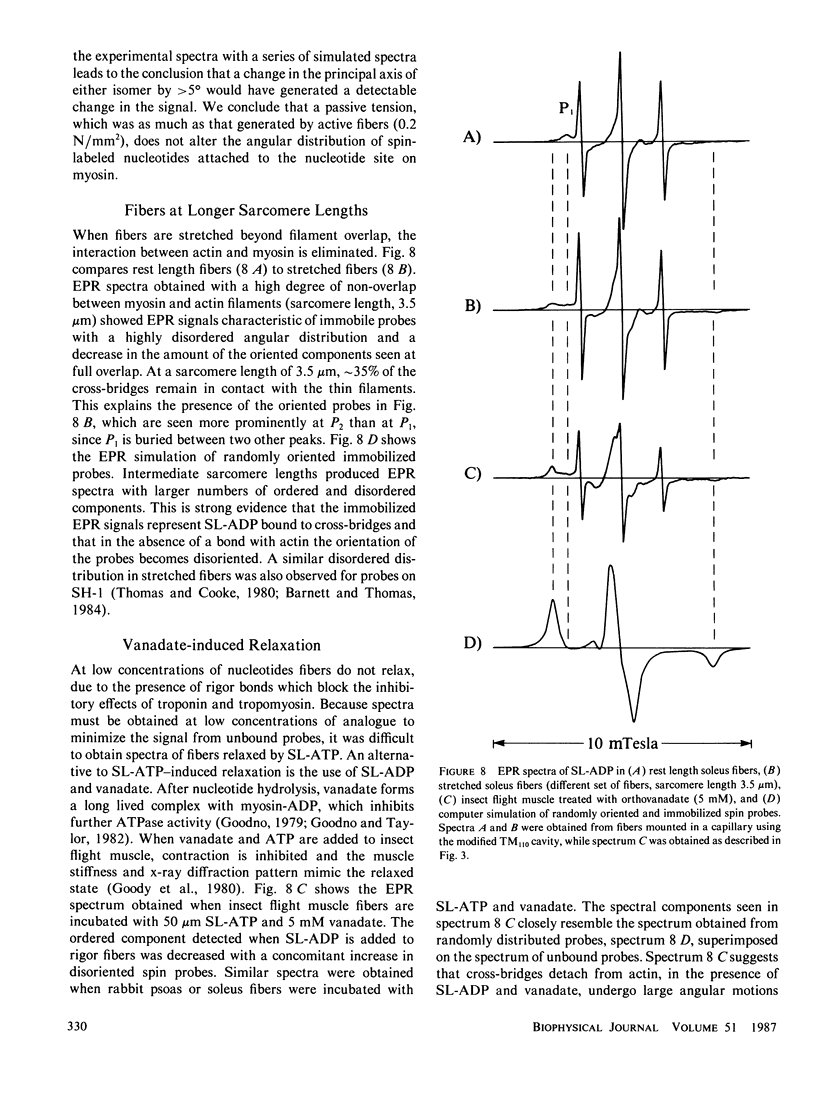
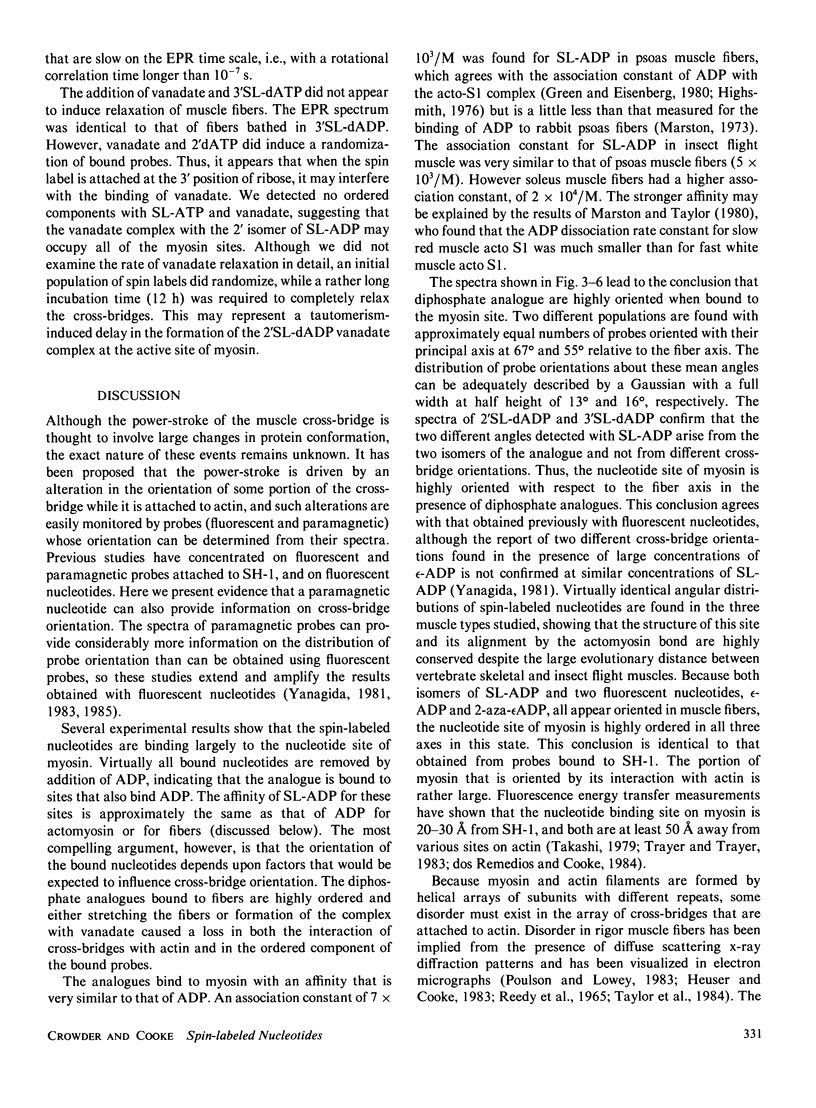
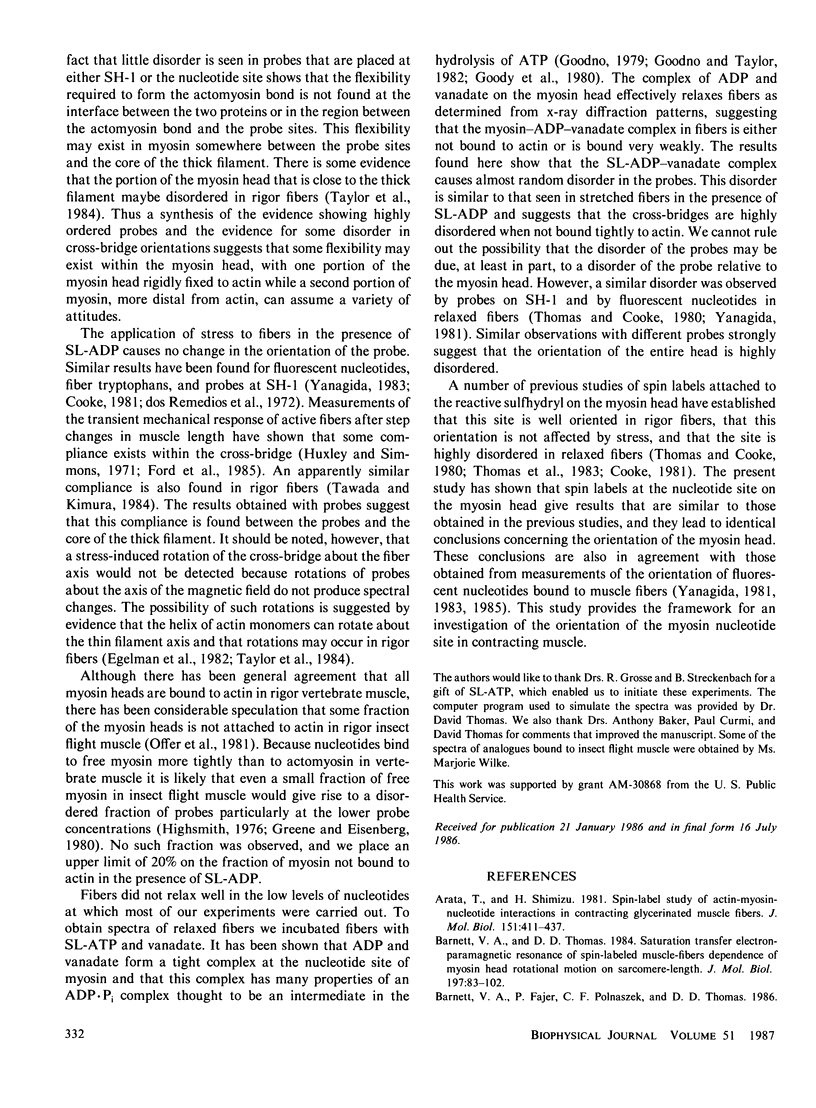
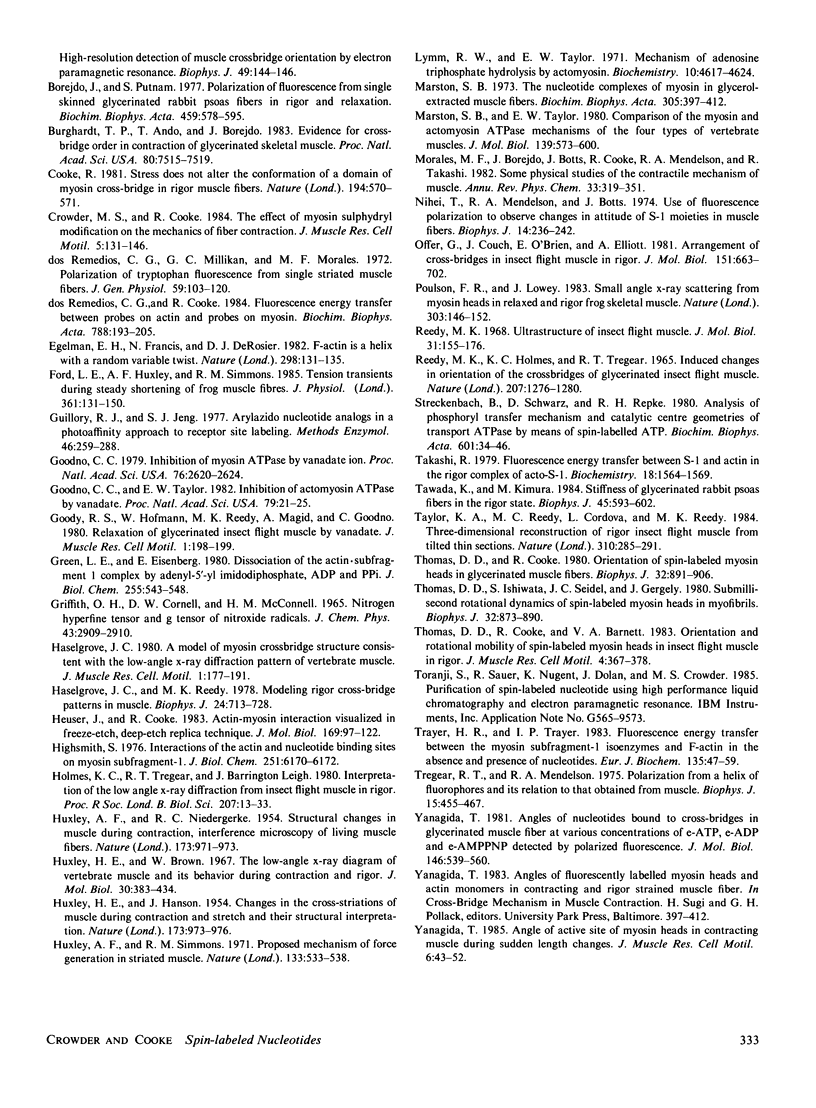
Selected References
These references are in PubMed. This may not be the complete list of references from this article.
- Arata T., Shimizu H. Spin-label study of actin-myosin-nucleotide interactions in contracting glycerinated muscle fibers. J Mol Biol. 1981 Sep 25;151(3):411–437. doi: 10.1016/0022-2836(81)90004-8. [DOI] [PubMed] [Google Scholar]
- Barnett V. A., Fajer P., Polnaszek C. F., Thomas D. D. High-Resolution Detection of muscle Crossbridge Orientation by Electron Paramagnetic Resonance. Biophys J. 1986 Jan;49(1):144–147. doi: 10.1016/S0006-3495(86)83628-1. [DOI] [PMC free article] [PubMed] [Google Scholar]
- Barnett V. A., Thomas D. D. Saturation transfer electron paramagnetic resonance of spin-labeled muscle fibers. Dependence of myosin head rotational motion on sarcomere length. J Mol Biol. 1984 Oct 15;179(1):83–102. doi: 10.1016/0022-2836(84)90307-3. [DOI] [PubMed] [Google Scholar]
- Borejdo J., Putnam S. Polarization of fluorescence from single skinned glycerinated rabbit psoas fibers in rigor and relaxation. Biochim Biophys Acta. 1977 Mar 11;459(3):578–595. doi: 10.1016/0005-2728(77)90056-1. [DOI] [PubMed] [Google Scholar]
- Burghardt T. P., Ando T., Borejdo J. Evidence for cross-bridge order in contraction of glycerinated skeletal muscle. Proc Natl Acad Sci U S A. 1983 Dec;80(24):7515–7519. doi: 10.1073/pnas.80.24.7515. [DOI] [PMC free article] [PubMed] [Google Scholar]
- Cooke R. Stress does not alter the conformation of a domain of the myosin cross-bridge in rigor muscle fibres. Nature. 1981 Dec 10;294(5841):570–571. doi: 10.1038/294570a0. [DOI] [PubMed] [Google Scholar]
- Crowder M. S., Cooke R. The effect of myosin sulphydryl modification on the mechanics of fibre contraction. J Muscle Res Cell Motil. 1984 Apr;5(2):131–146. doi: 10.1007/BF00712152. [DOI] [PubMed] [Google Scholar]
- Dos Remedios C. G., Cooke R. Fluorescence energy transfer between probes on actin and probes on myosin. Biochim Biophys Acta. 1984 Jul 31;788(2):193–205. doi: 10.1016/0167-4838(84)90262-0. [DOI] [PubMed] [Google Scholar]
- Dos Remedios C. G., Millikan R. G., Morales M. F. Polarization of tryptophan fluorescence from single striated muscle fibers. A molecular probe of contractile state. J Gen Physiol. 1972 Jan;59(1):103–120. doi: 10.1085/jgp.59.1.103. [DOI] [PMC free article] [PubMed] [Google Scholar]
- Egelman E. H., Francis N., DeRosier D. J. F-actin is a helix with a random variable twist. Nature. 1982 Jul 8;298(5870):131–135. doi: 10.1038/298131a0. [DOI] [PubMed] [Google Scholar]
- Ford L. E., Huxley A. F., Simmons R. M. Tension transients during steady shortening of frog muscle fibres. J Physiol. 1985 Apr;361:131–150. doi: 10.1113/jphysiol.1985.sp015637. [DOI] [PMC free article] [PubMed] [Google Scholar]
- Goodno C. C. Inhibition of myosin ATPase by vanadate ion. Proc Natl Acad Sci U S A. 1979 Jun;76(6):2620–2624. doi: 10.1073/pnas.76.6.2620. [DOI] [PMC free article] [PubMed] [Google Scholar]
- Goodno C. C., Taylor E. W. Inhibition of actomyosin ATPase by vanadate. Proc Natl Acad Sci U S A. 1982 Jan;79(1):21–25. doi: 10.1073/pnas.79.1.21. [DOI] [PMC free article] [PubMed] [Google Scholar]
- Greene L. E., Eisenberg E. Dissociation of the actin.subfragment 1 complex by adenyl-5'-yl imidodiphosphate, ADP, and PPi. J Biol Chem. 1980 Jan 25;255(2):543–548. [PubMed] [Google Scholar]
- Guillory R. J., Jeng S. J. Arylazido nucleotide analogs in a photoaffinity approach to receptor site labeling. Methods Enzymol. 1977;46:259–288. doi: 10.1016/s0076-6879(77)46029-4. [DOI] [PubMed] [Google Scholar]
- HUXLEY A. F., NIEDERGERKE R. Structural changes in muscle during contraction; interference microscopy of living muscle fibres. Nature. 1954 May 22;173(4412):971–973. doi: 10.1038/173971a0. [DOI] [PubMed] [Google Scholar]
- HUXLEY H., HANSON J. Changes in the cross-striations of muscle during contraction and stretch and their structural interpretation. Nature. 1954 May 22;173(4412):973–976. doi: 10.1038/173973a0. [DOI] [PubMed] [Google Scholar]
- Haselgrove J. C. A model of myosin crossbridge structure consistent with the low-angle x-ray diffraction pattern of vertebrate muscle. J Muscle Res Cell Motil. 1980 Jun;1(2):177–191. doi: 10.1007/BF00711798. [DOI] [PubMed] [Google Scholar]
- Haselgrove J. C., Reedy M. K. Modeling rigor cross-bridge patterns in muscle I. Initial studies of the rigor lattice of insect flight muscle. Biophys J. 1978 Dec;24(3):713–728. doi: 10.1016/S0006-3495(78)85415-0. [DOI] [PMC free article] [PubMed] [Google Scholar]
- Heuser J. E., Cooke R. Actin-myosin interactions visualized by the quick-freeze, deep-etch replica technique. J Mol Biol. 1983 Sep 5;169(1):97–122. doi: 10.1016/s0022-2836(83)80177-6. [DOI] [PubMed] [Google Scholar]
- Highsmith S. Interactions of the actin and nucleotide binding sites on myosin subfragment 1. J Biol Chem. 1976 Oct 25;251(20):6170–6172. [PubMed] [Google Scholar]
- Huxley A. F., Simmons R. M. Proposed mechanism of force generation in striated muscle. Nature. 1971 Oct 22;233(5321):533–538. doi: 10.1038/233533a0. [DOI] [PubMed] [Google Scholar]
- Huxley H. E., Brown W. The low-angle x-ray diagram of vertebrate striated muscle and its behaviour during contraction and rigor. J Mol Biol. 1967 Dec 14;30(2):383–434. doi: 10.1016/s0022-2836(67)80046-9. [DOI] [PubMed] [Google Scholar]
- Lymn R. W., Taylor E. W. Mechanism of adenosine triphosphate hydrolysis by actomyosin. Biochemistry. 1971 Dec 7;10(25):4617–4624. doi: 10.1021/bi00801a004. [DOI] [PubMed] [Google Scholar]
- Marston S. B., Taylor E. W. Comparison of the myosin and actomyosin ATPase mechanisms of the four types of vertebrate muscles. J Mol Biol. 1980 Jun 5;139(4):573–600. doi: 10.1016/0022-2836(80)90050-9. [DOI] [PubMed] [Google Scholar]
- Marston S. The nucleotide complexes of myosin in glycerol-extracted muscle fibres. Biochim Biophys Acta. 1973 May 30;305(2):397–412. doi: 10.1016/0005-2728(73)90186-2. [DOI] [PubMed] [Google Scholar]
- Nihei T., Mendelson R. A., Botts J. Use of fluorescence polarization to observe changes in attitude of S-1 moieties in muscle fibers. Biophys J. 1974 Mar;14(3):236–242. doi: 10.1016/S0006-3495(74)85911-4. [DOI] [PMC free article] [PubMed] [Google Scholar]
- Offer G., Couch J., O'Brien E., Elliott A. Arrangement of cross-bridges in insect flight muscle in rigor. J Mol Biol. 1981 Oct 5;151(4):663–702. doi: 10.1016/0022-2836(81)90429-0. [DOI] [PubMed] [Google Scholar]
- Reedy M. K., Holmes K. C., Tregear R. T. Induced changes in orientation of the cross-bridges of glycerinated insect flight muscle. Nature. 1965 Sep 18;207(5003):1276–1280. doi: 10.1038/2071276a0. [DOI] [PubMed] [Google Scholar]
- Reedy M. K. Ultrastructure of insect flight muscle. I. Screw sense and structural grouping in the rigor cross-bridge lattice. J Mol Biol. 1968 Jan 28;31(2):155–176. doi: 10.1016/0022-2836(68)90437-3. [DOI] [PubMed] [Google Scholar]
- Streckenbach B., Schwarz D., Repke K. R. Analysis of phosphoryl transfer mechanism and catalytic centre geometries of transport ATPase by means of spin-labelled ATP. Biochim Biophys Acta. 1980 Sep 2;601(1):34–46. doi: 10.1016/0005-2736(80)90511-8. [DOI] [PubMed] [Google Scholar]
- Tawada K., Kimura M. Stiffness of glycerinated rabbit psoas fibers in the rigor state. Filament-overlap relation. Biophys J. 1984 Mar;45(3):593–602. doi: 10.1016/S0006-3495(84)84197-1. [DOI] [PMC free article] [PubMed] [Google Scholar]
- Taylor K. A., Reedy M. C., Córdova L., Reedy M. K. Three-dimensional reconstruction of rigor insect flight muscle from tilted thin sections. 1984 Jul 26-Aug 1Nature. 310(5975):285–291. doi: 10.1038/310285a0. [DOI] [PubMed] [Google Scholar]
- Thomas D. D., Cooke R., Barnett V. A. Orientation and rotational mobility of spin-labelled myosin heads in insect flight muscle in rigor. J Muscle Res Cell Motil. 1983 Jun;4(3):367–378. doi: 10.1007/BF00712002. [DOI] [PubMed] [Google Scholar]
- Thomas D. D., Cooke R. Orientation of spin-labeled myosin heads in glycerinated muscle fibers. Biophys J. 1980 Dec;32(3):891–906. doi: 10.1016/S0006-3495(80)85024-7. [DOI] [PMC free article] [PubMed] [Google Scholar]
- Thomas D. D., Ishiwata S., Seidel J. C., Gergely J. Submillisecond rotational dynamics of spin-labeled myosin heads in myofibrils. Biophys J. 1980 Dec;32(3):873–889. doi: 10.1016/S0006-3495(80)85023-5. [DOI] [PMC free article] [PubMed] [Google Scholar]
- Trayer H. R., Trayer I. P. Fluorescence energy transfer between the myosin subfragment-1 isoenzymes and F-actin in the absence and presence of nucleotides. Eur J Biochem. 1983 Sep 1;135(1):47–59. doi: 10.1111/j.1432-1033.1983.tb07616.x. [DOI] [PubMed] [Google Scholar]
- Tregear R. T., Mendelson R. A. Polarization from a helix of fluorophores and its relation to that obtained from muscle. Biophys J. 2009 Jan 1;15(5):455–467. doi: 10.1016/S0006-3495(75)85830-9. [DOI] [PMC free article] [PubMed] [Google Scholar]
- Yanagida T. Angle of active site of myosin heads in contracting muscle during sudden length changes. J Muscle Res Cell Motil. 1985 Feb;6(1):43–52. doi: 10.1007/BF00712310. [DOI] [PubMed] [Google Scholar]
- Yanagida T. Angles of nucleotides bound to cross-bridges in glycerinated muscle fiber at various concentrations of epsilon-ATP, epsilon-ADP and epsilon-AMPPNP detected by polarized fluorescence. J Mol Biol. 1981 Mar 15;146(4):539–560. doi: 10.1016/0022-2836(81)90046-2. [DOI] [PubMed] [Google Scholar]