Abstract
Several workers have identified molecular abnormalities associated with inherited blood disorders. The present work examines how these alterations in molecular structure affect the viscoelastic properties of the red blood cell membrane. Changes in the membrane shear modulus, the membrane viscosity, and the apparent membrane bending stiffness were observed in cells of eight patients having a variety of disorders: Two had reductions in the number of high-affinity ankyrin binding sites, two had abnormalities associated with the protein band 4.1, and six were known to be deficient in spectrin. The data suggest that the membrane shear modulus is proportional to the density of spectrin on the membrane and support the view that spectrin is primarily responsible for membrane shear elasticity. Although membranes having abnormalities associated with the function of ankyrin or band 4.1 exhibited reduced elasticity, the degree of mechanical dysfunction was quantitatively inconsistent with the extent of the molecular abnormality. This indicates that these skeletal components do not play a primary role in determining membrane shear elasticity. The membrane viscosity was reduced in seven of the eight patients studied. The reduction in viscosity was usually greater than the reduction in shear modulus, but the degree of reduction in viscosity was variable and did not correlate well with the degree of molecular abnormality.
Full text
PDF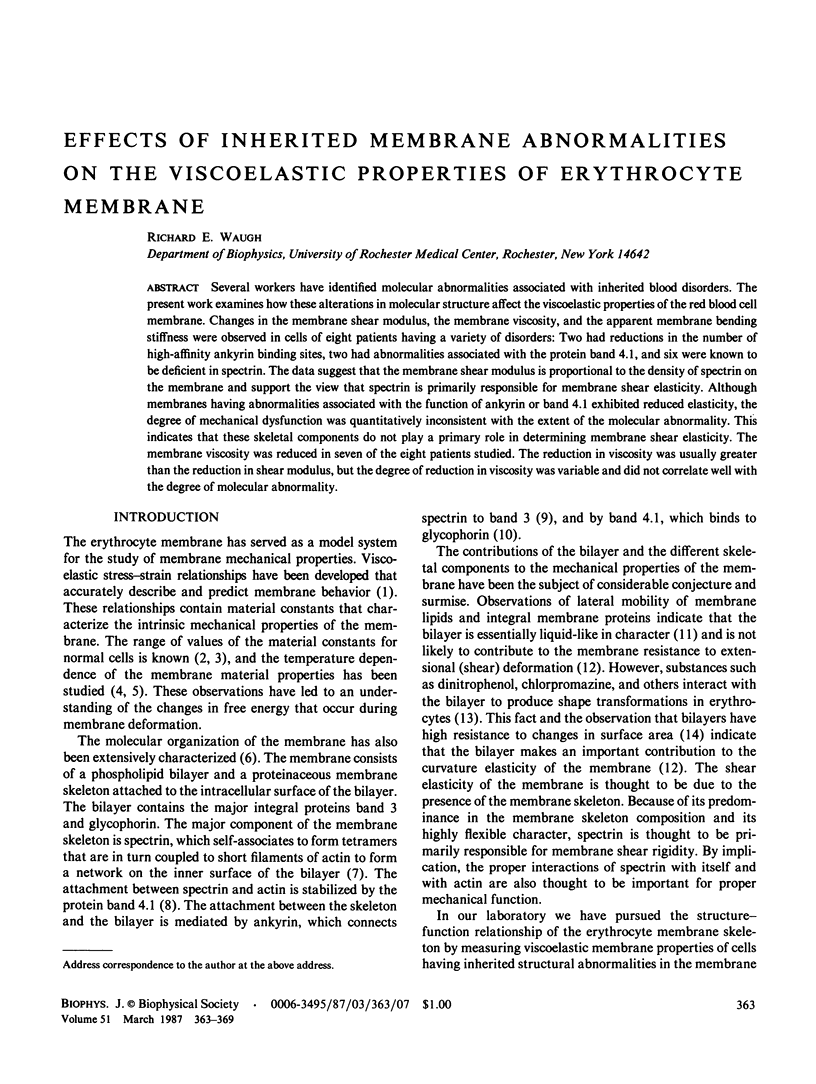
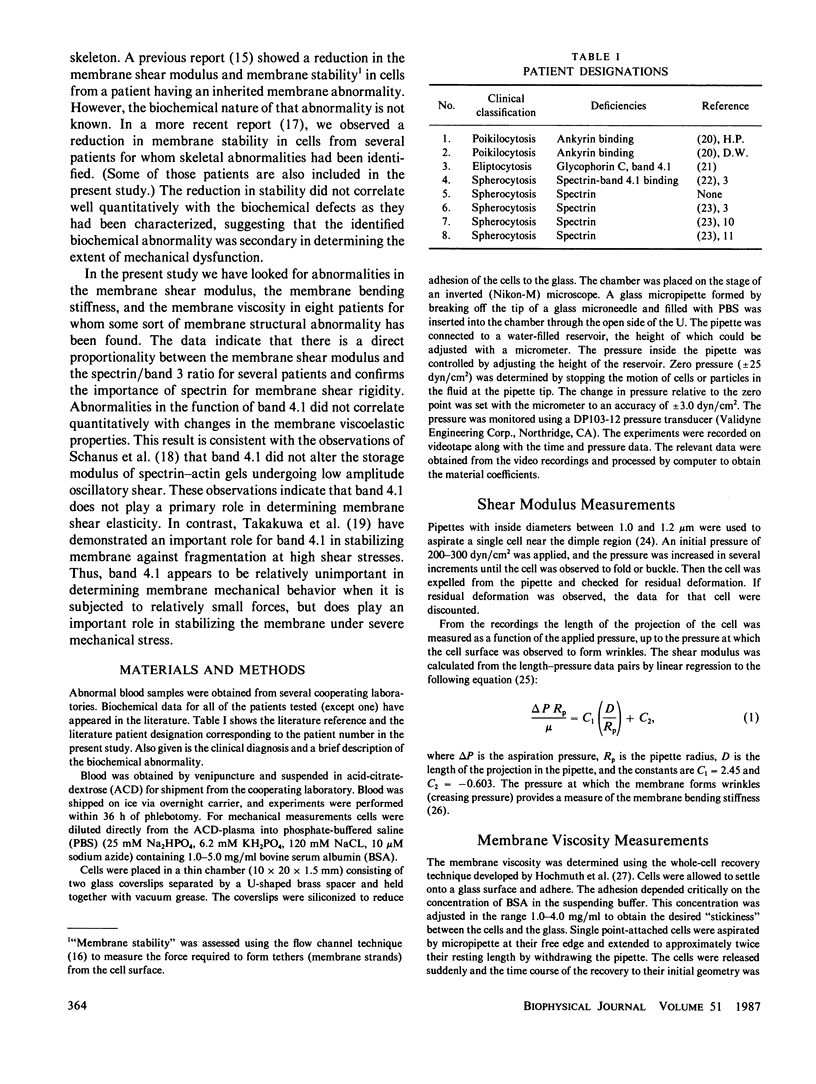
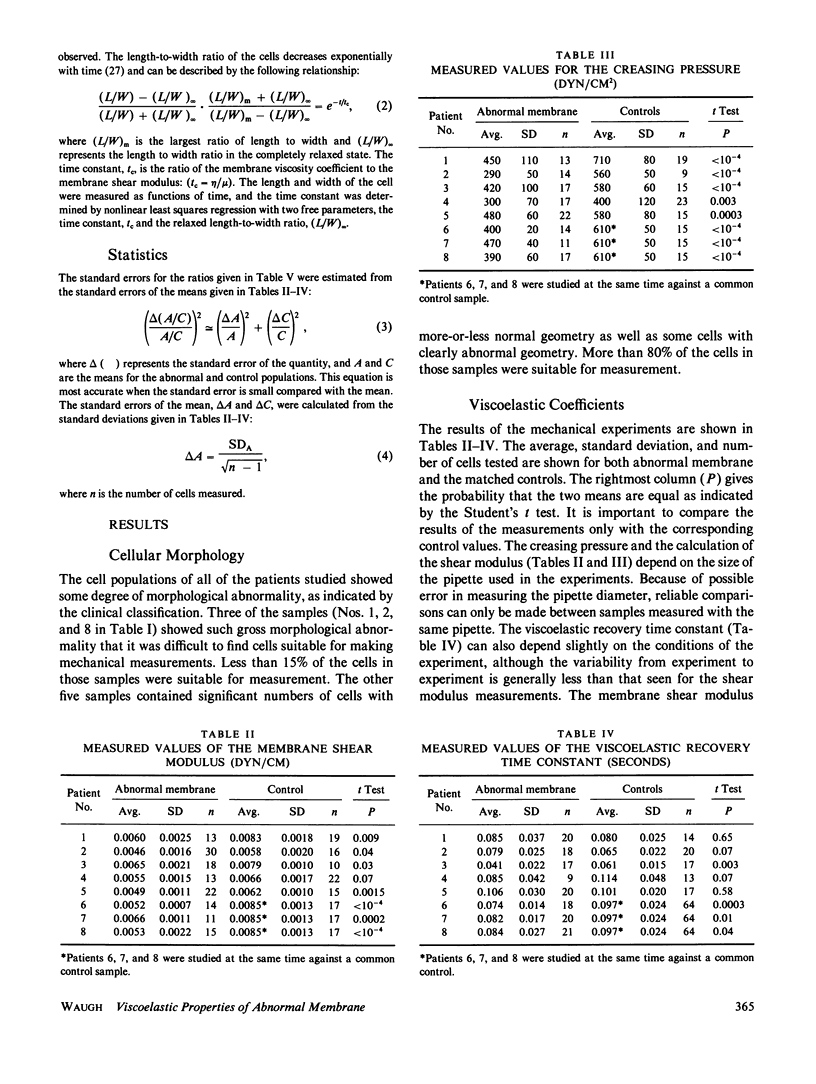
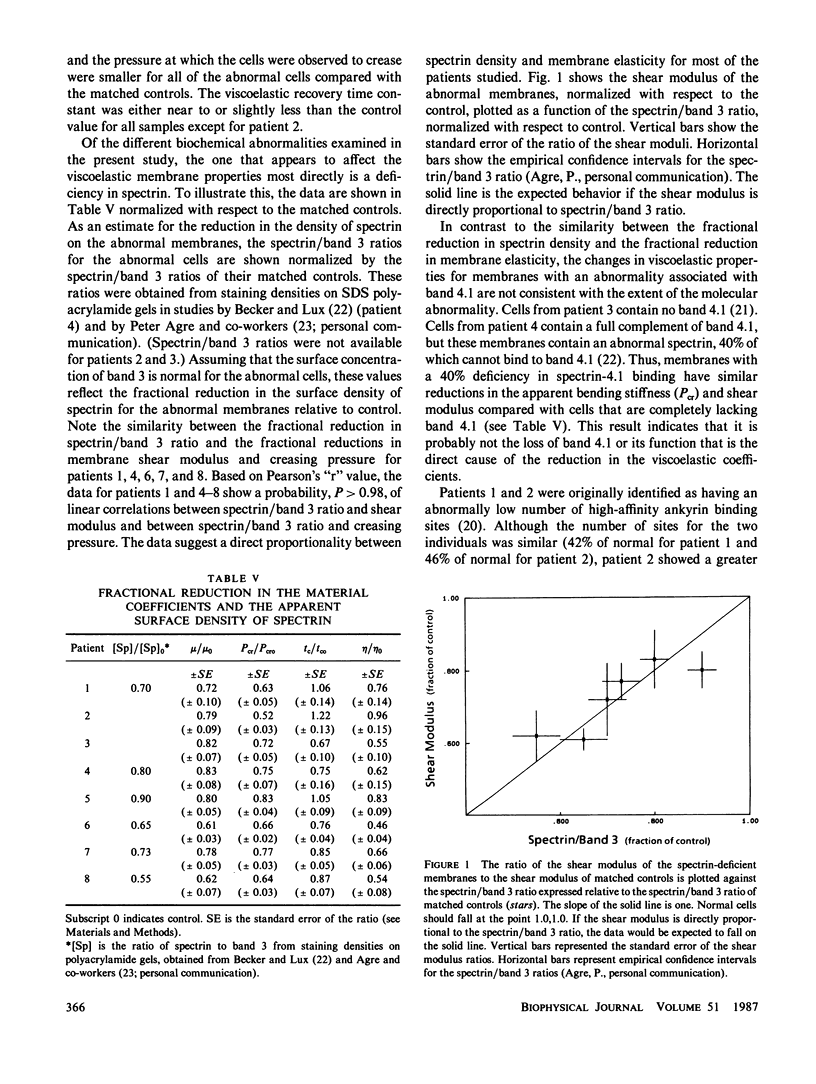
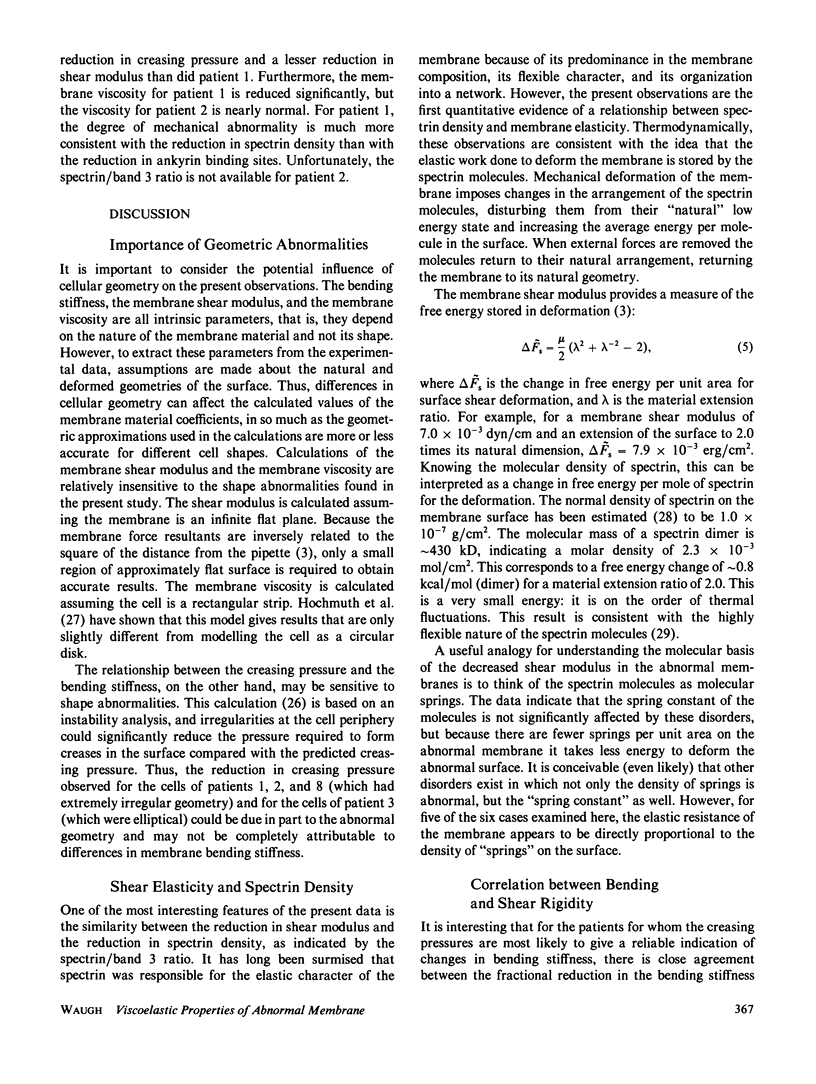
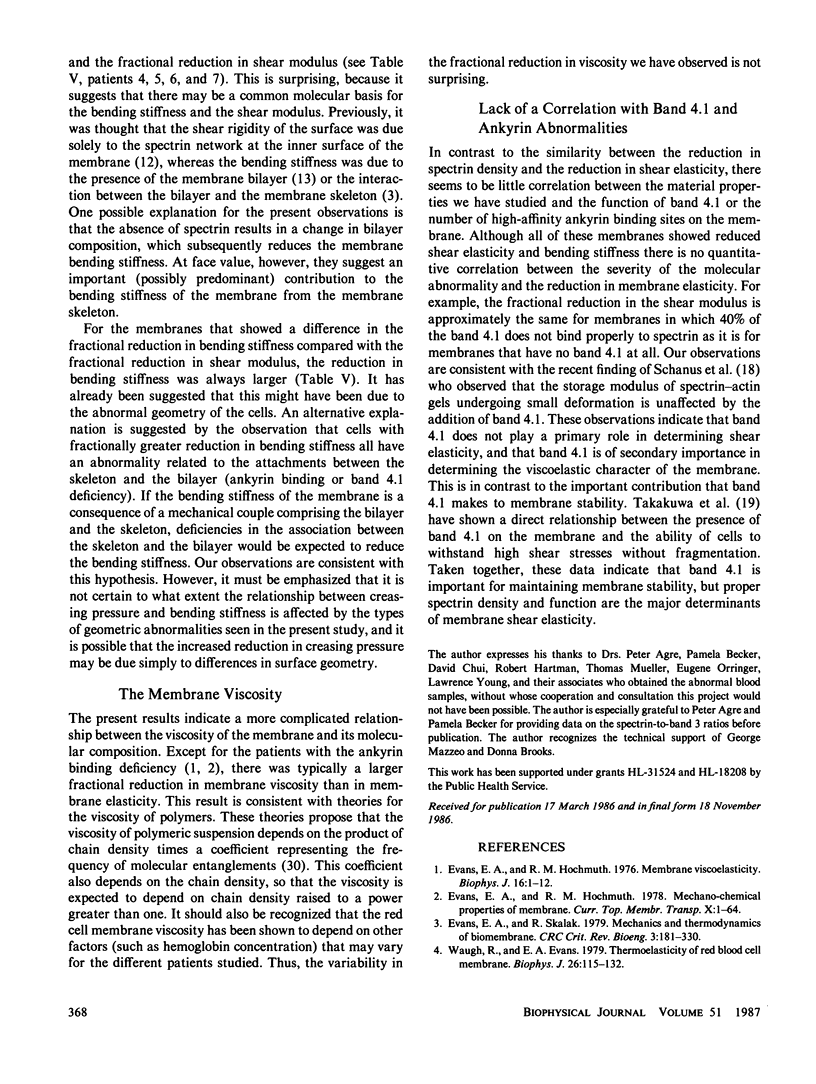
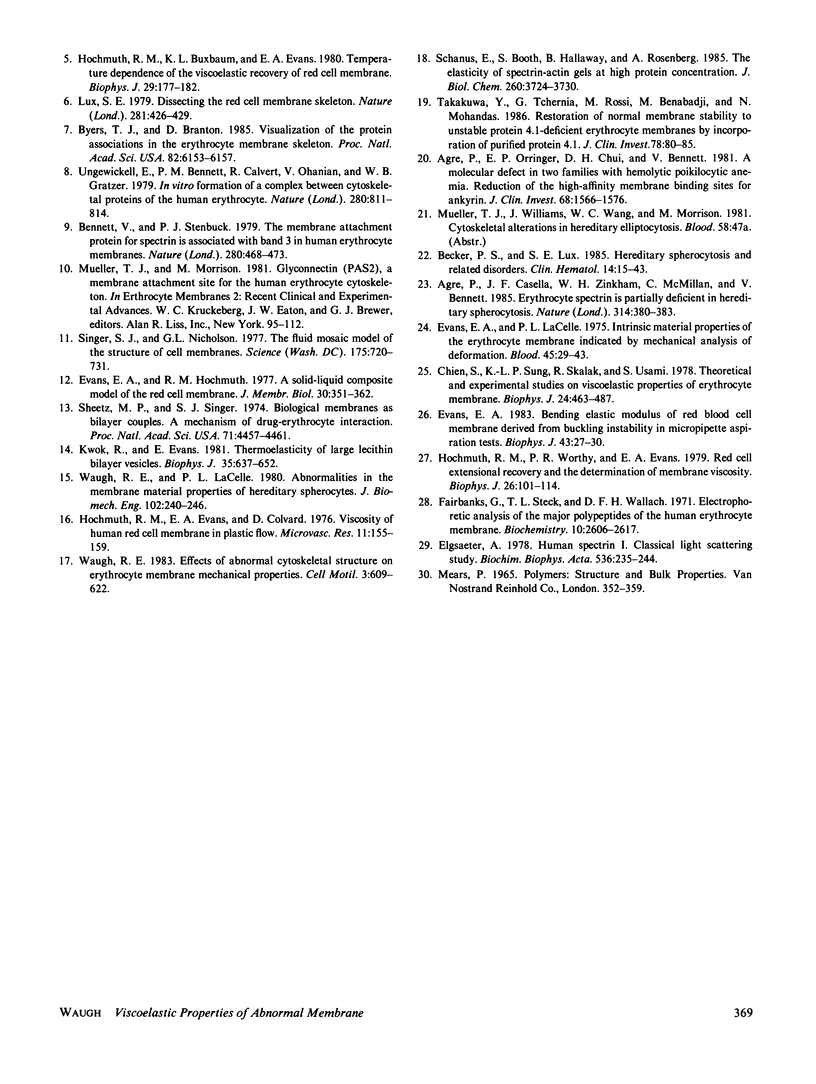
Selected References
These references are in PubMed. This may not be the complete list of references from this article.
- Agre P., Casella J. F., Zinkham W. H., McMillan C., Bennett V. Partial deficiency of erythrocyte spectrin in hereditary spherocytosis. 1985 Mar 28-Apr 3Nature. 314(6009):380–383. doi: 10.1038/314380a0. [DOI] [PubMed] [Google Scholar]
- Agre P., Orringer E. P., Chui D. H., Bennett V. A molecular defect in two families with hemolytic poikilocytic anemia: reduction of high affinity membrane binding sites for ankyrin. J Clin Invest. 1981 Dec;68(6):1566–1576. doi: 10.1172/JCI110411. [DOI] [PMC free article] [PubMed] [Google Scholar]
- Becker P. S., Lux S. E. Hereditary spherocytosis and related disorders. Clin Haematol. 1985 Feb;14(1):15–43. [PubMed] [Google Scholar]
- Bennett V., Stenbuck P. J. The membrane attachment protein for spectrin is associated with band 3 in human erythrocyte membranes. Nature. 1979 Aug 9;280(5722):468–473. doi: 10.1038/280468a0. [DOI] [PubMed] [Google Scholar]
- Byers T. J., Branton D. Visualization of the protein associations in the erythrocyte membrane skeleton. Proc Natl Acad Sci U S A. 1985 Sep;82(18):6153–6157. doi: 10.1073/pnas.82.18.6153. [DOI] [PMC free article] [PubMed] [Google Scholar]
- Chien S., Sung K. L., Skalak R., Usami S., Tözeren A. Theoretical and experimental studies on viscoelastic properties of erythrocyte membrane. Biophys J. 1978 Nov;24(2):463–487. doi: 10.1016/S0006-3495(78)85395-8. [DOI] [PMC free article] [PubMed] [Google Scholar]
- Elgsaeter A. Human spectrin. I. A classical light scattering study. Biochim Biophys Acta. 1978 Sep 26;536(1):235–244. doi: 10.1016/0005-2795(78)90069-7. [DOI] [PubMed] [Google Scholar]
- Evans E. A. Bending elastic modulus of red blood cell membrane derived from buckling instability in micropipet aspiration tests. Biophys J. 1983 Jul;43(1):27–30. doi: 10.1016/S0006-3495(83)84319-7. [DOI] [PMC free article] [PubMed] [Google Scholar]
- Evans E. A., Hochmuth R. M. A solid-liquid composite model of the red cell membrane. J Membr Biol. 1977 Jan 28;30(4):351–362. doi: 10.1007/BF01869676. [DOI] [PubMed] [Google Scholar]
- Evans E. A., Hochmuth R. M. Membrane viscoelasticity. Biophys J. 1976 Jan;16(1):1–11. doi: 10.1016/S0006-3495(76)85658-5. [DOI] [PMC free article] [PubMed] [Google Scholar]
- Evans E. A., La Celle P. L. Intrinsic material properties of the erythrocyte membrane indicated by mechanical analysis of deformation. Blood. 1975 Jan;45(1):29–43. [PubMed] [Google Scholar]
- Evans E. A., Skalak R. Mechanics and thermodynamics of biomembranes: part 1. CRC Crit Rev Bioeng. 1979 Oct;3(3):181–330. [PubMed] [Google Scholar]
- Fairbanks G., Steck T. L., Wallach D. F. Electrophoretic analysis of the major polypeptides of the human erythrocyte membrane. Biochemistry. 1971 Jun 22;10(13):2606–2617. doi: 10.1021/bi00789a030. [DOI] [PubMed] [Google Scholar]
- Hochmuth R. M., Buxbaum K. L., Evans E. A. Temperature dependence of the viscoelastic recovery of red cell membrane. Biophys J. 1980 Jan;29(1):177–182. doi: 10.1016/S0006-3495(80)85124-1. [DOI] [PMC free article] [PubMed] [Google Scholar]
- Hochmuth R. M., Evans E. A., Colvard D. F. Viscosity of human red cell membrane in plastic flow. Microvasc Res. 1976 Mar;11(2):155–159. doi: 10.1016/0026-2862(76)90047-9. [DOI] [PubMed] [Google Scholar]
- Hochmuth R. M., Worthy P. R., Evans E. A. Red cell extensional recovery and the determination of membrane viscosity. Biophys J. 1979 Apr;26(1):101–114. doi: 10.1016/S0006-3495(79)85238-8. [DOI] [PMC free article] [PubMed] [Google Scholar]
- Kwok R., Evans E. Thermoelasticity of large lecithin bilayer vesicles. Biophys J. 1981 Sep;35(3):637–652. doi: 10.1016/S0006-3495(81)84817-5. [DOI] [PMC free article] [PubMed] [Google Scholar]
- Lux S. E. Dissecting the red cell membrane skeleton. Nature. 1979 Oct 11;281(5731):426–429. doi: 10.1038/281426a0. [DOI] [PubMed] [Google Scholar]
- Schanus E., Booth S., Hallaway B., Rosenberg A. The elasticity of spectrin-actin gels at high protein concentration. J Biol Chem. 1985 Mar 25;260(6):3724–3730. [PubMed] [Google Scholar]
- Sheetz M. P., Singer S. J. Biological membranes as bilayer couples. A molecular mechanism of drug-erythrocyte interactions. Proc Natl Acad Sci U S A. 1974 Nov;71(11):4457–4461. doi: 10.1073/pnas.71.11.4457. [DOI] [PMC free article] [PubMed] [Google Scholar]
- Singer S. J., Nicolson G. L. The fluid mosaic model of the structure of cell membranes. Science. 1972 Feb 18;175(4023):720–731. doi: 10.1126/science.175.4023.720. [DOI] [PubMed] [Google Scholar]
- Takakuwa Y., Tchernia G., Rossi M., Benabadji M., Mohandas N. Restoration of normal membrane stability to unstable protein 4.1-deficient erythrocyte membranes by incorporation of purified protein 4.1. J Clin Invest. 1986 Jul;78(1):80–85. doi: 10.1172/JCI112577. [DOI] [PMC free article] [PubMed] [Google Scholar]
- Ungewickell E., Bennett P. M., Calvert R., Ohanian V., Gratzer W. B. In vitro formation of a complex between cytoskeletal proteins of the human erythrocyte. Nature. 1979 Aug 30;280(5725):811–814. doi: 10.1038/280811a0. [DOI] [PubMed] [Google Scholar]
- Waugh R. E. Effects of abnormal cytoskeletal structure on erythrocyte membrane mechanical properties. Cell Motil. 1983;3(5-6):609–622. doi: 10.1002/cm.970030526. [DOI] [PubMed] [Google Scholar]
- Waugh R., Evans E. A. Thermoelasticity of red blood cell membrane. Biophys J. 1979 Apr;26(1):115–131. doi: 10.1016/S0006-3495(79)85239-X. [DOI] [PMC free article] [PubMed] [Google Scholar]