Abstract
An open circuit kinetic model was developed to calculate the time course of proximal tubule cell pH, solute concentrations, and volume in response to induced perturbations in luminal or peritubular fluid composition. Solute fluxes were calculated from electrokinetic equations containing terms for known carrier saturabilities, allosteric dependences, and ion coupling ratios. Apical and basolateral membrane potentials were determined iteratively from the requirements of cell electroneutrality and equal opposing transcellular and paracellular currents. The model converged to membrane potentials accurate to 0.05% in one to four iterations. Model variables included cell concentrations of Na, K, HCO3, glucose, pH (uniform CO2), volume, and apical and basolateral membrane potentials. The basic model contained passive apical membrane transport of Na/H, Na/glucose, H and K, basolateral transport of Na/3HCO3, K, H, and glucose, and paracellular transport of Na, K, Cl, and HCO3; apical H and basolateral 3Na/2K-ATPases were present. Apical Na/H and basolateral K transport were regulated allosterically by pH. Apical Na/H transport, basolateral Na/3HCO3 transport, and the 3Na/2K-ATPase were saturable. Model parameters were chosen from data in the rat proximal tubule. Model predictions for the magnitude and time course of cell pH, Na, and membrane potential in response to rapid changes in apical and peritubular Na and HCO3 were in excellent agreement with experiment. In addition, the model requires that there exist an apical H-ATPase, basolateral Na/3HCO3 transport saturable with HCO3, and electroneutral basolateral K transport.
Full text
PDF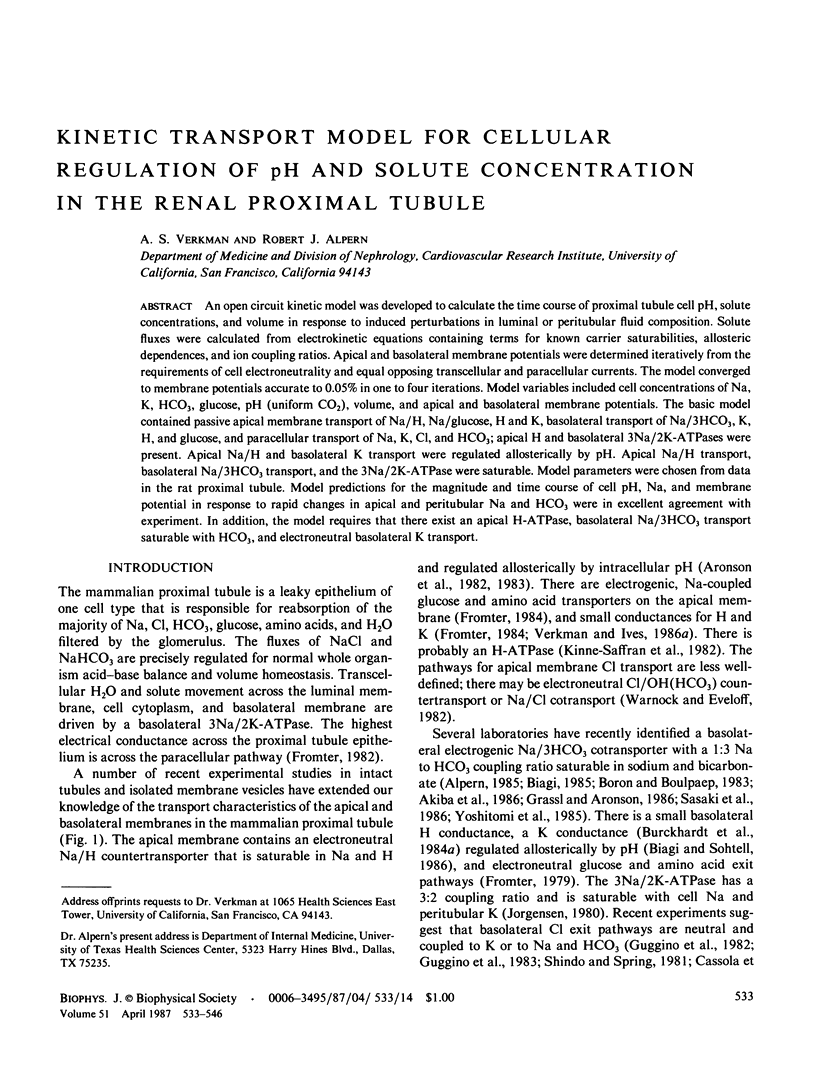
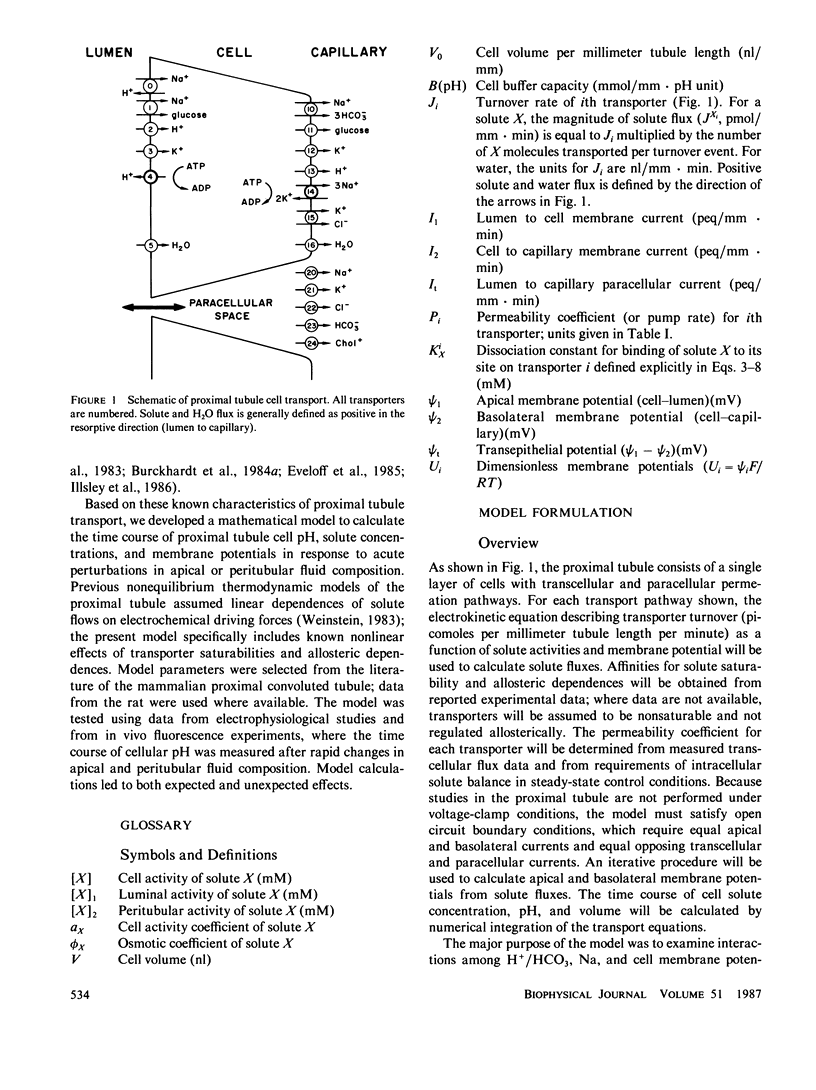
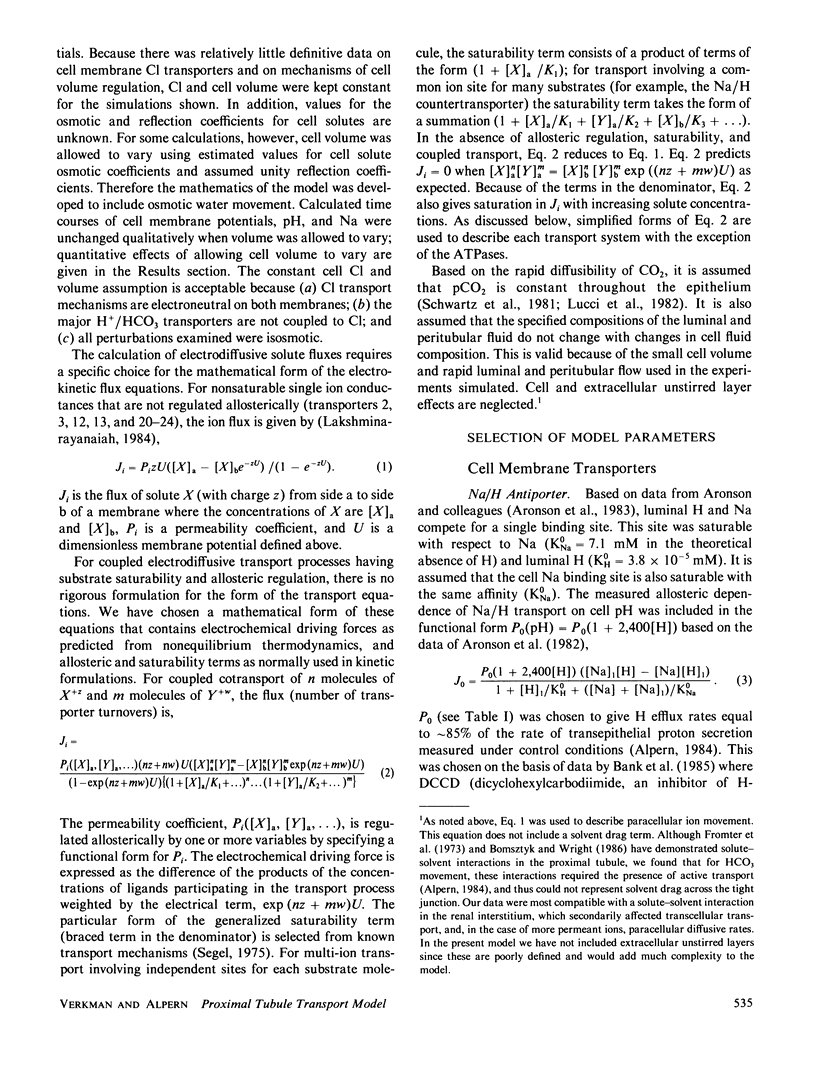
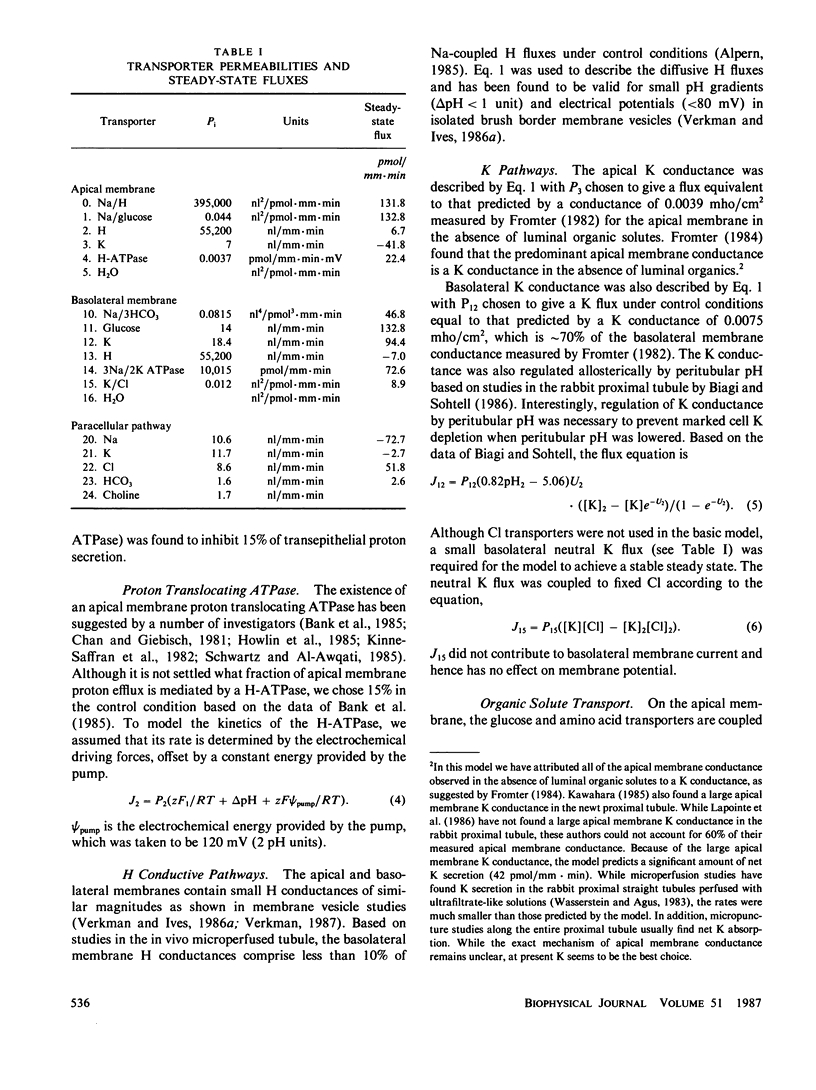
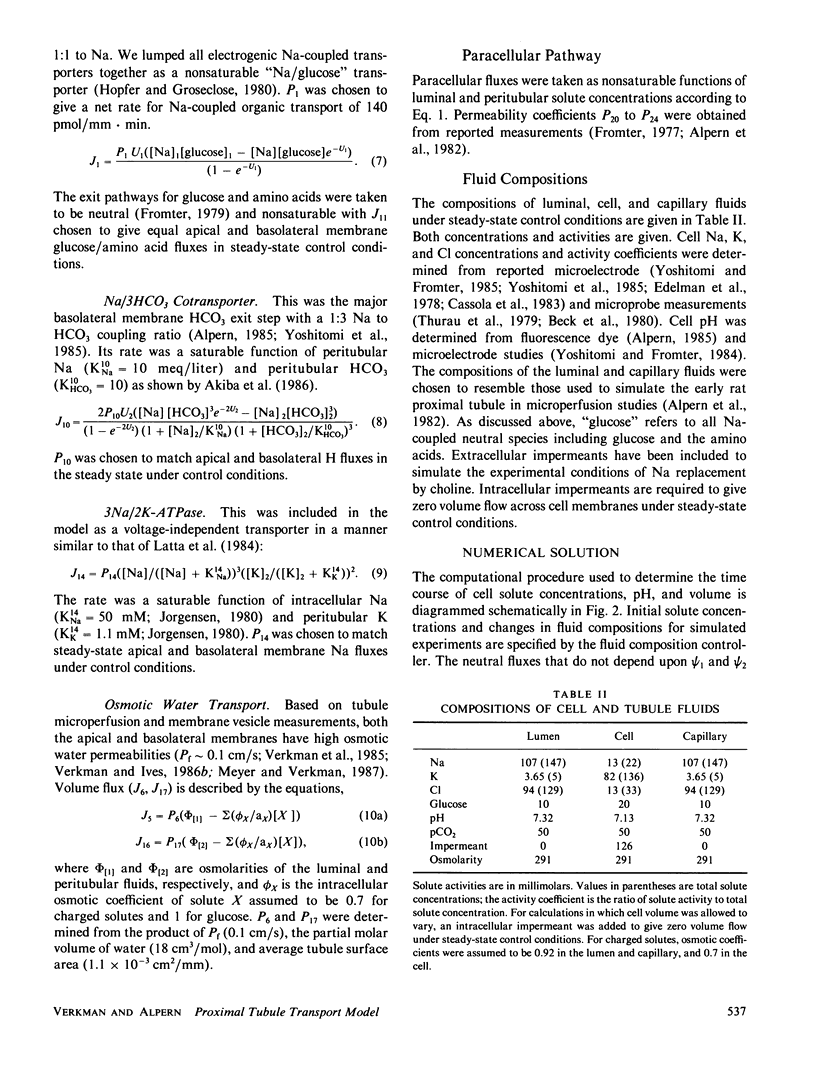
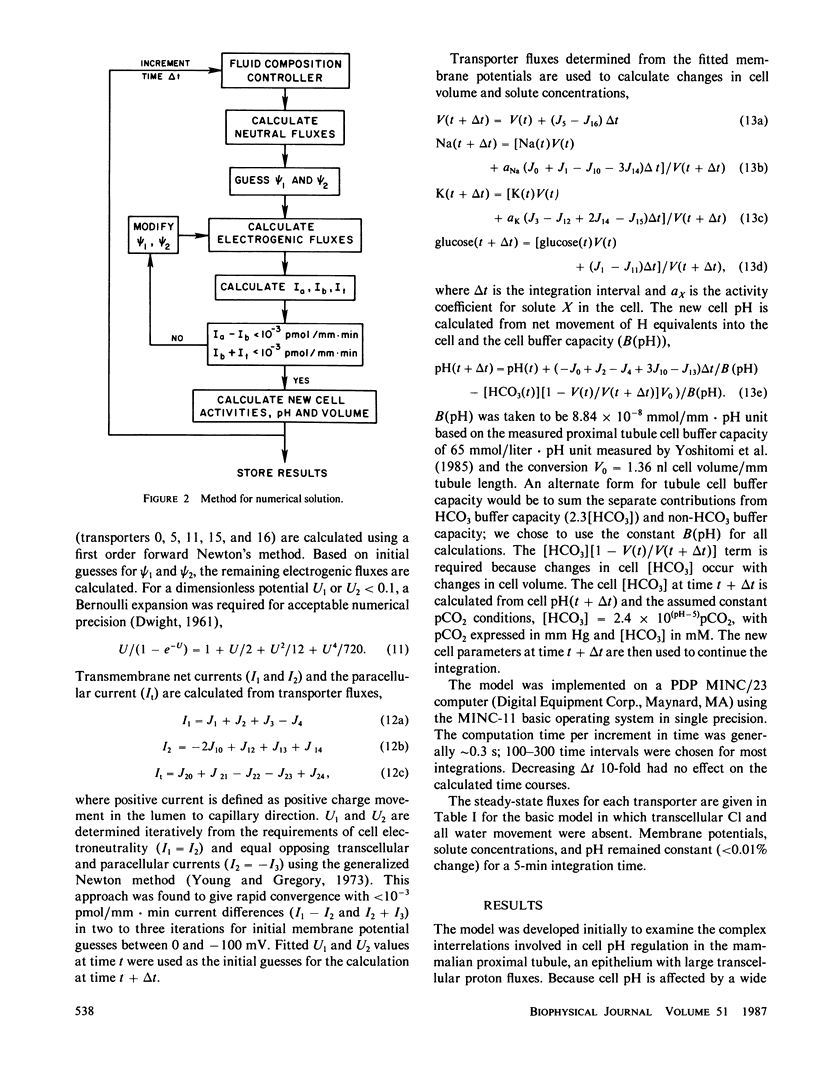
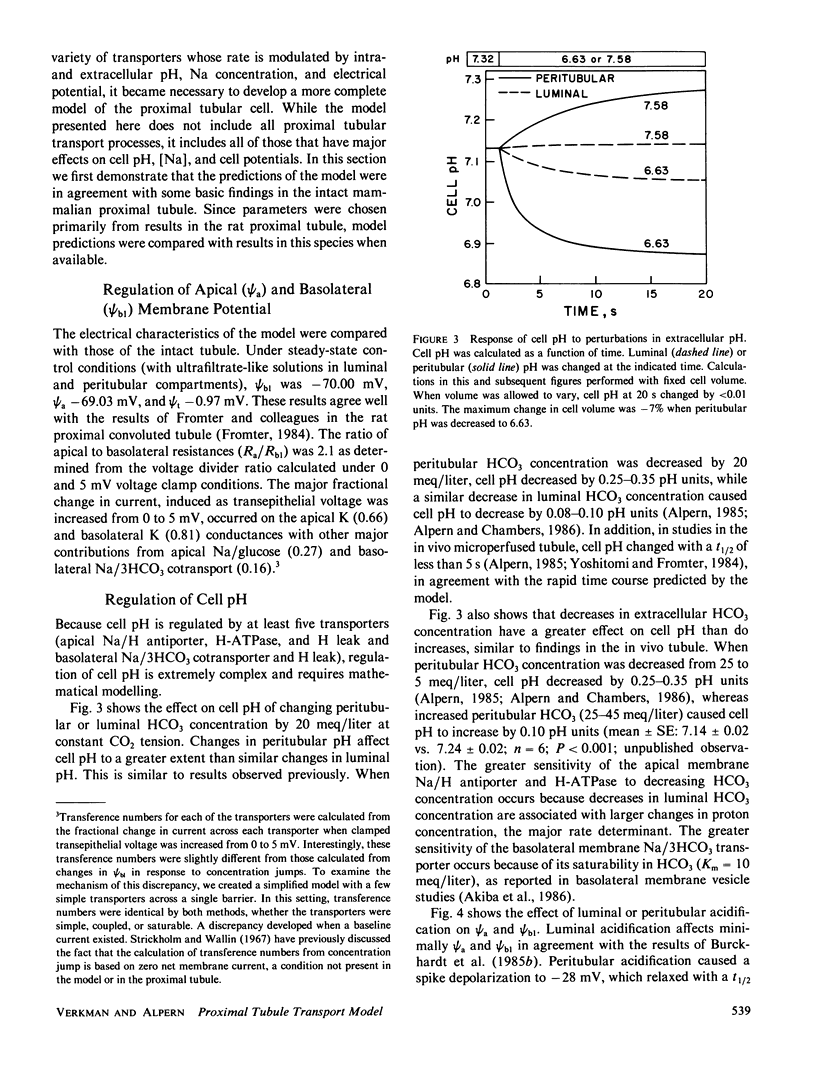
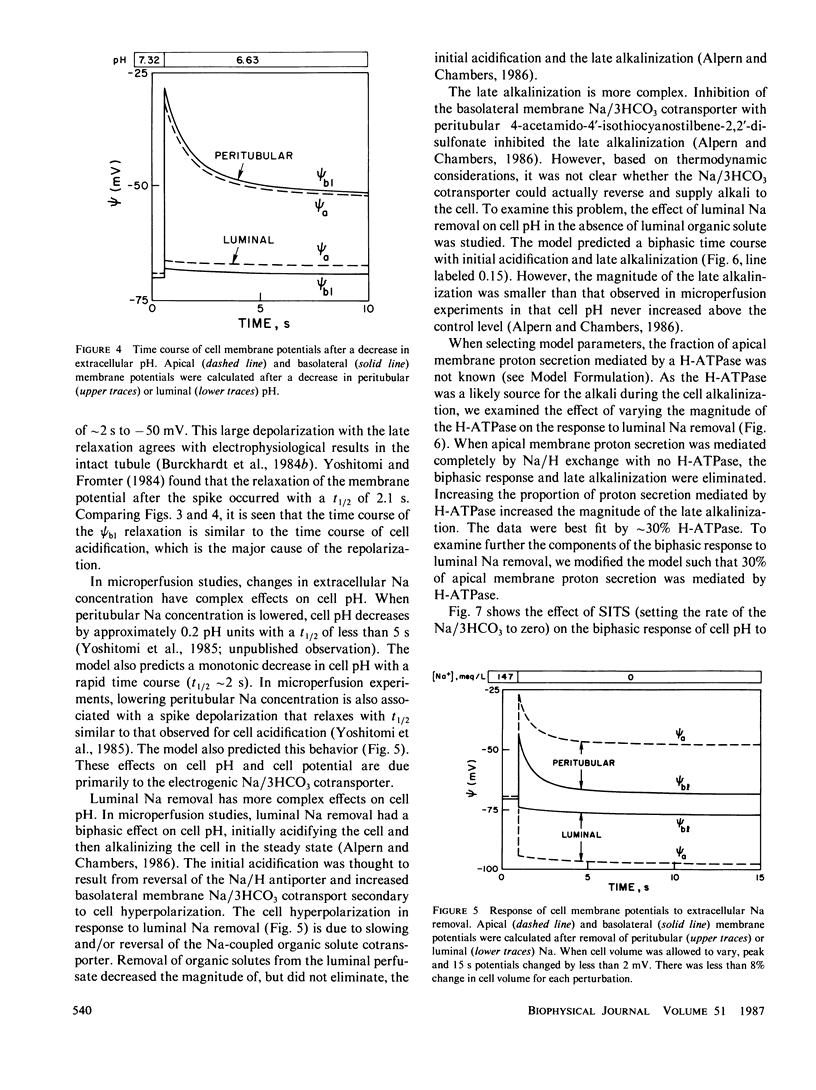
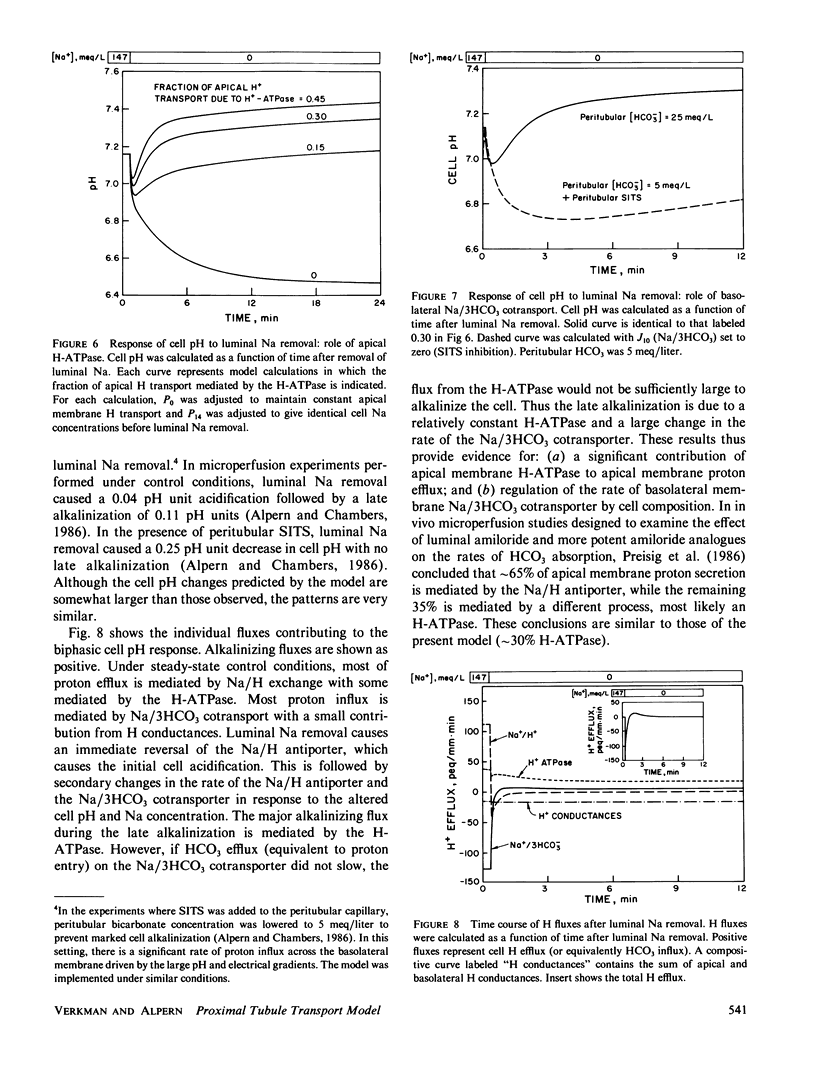
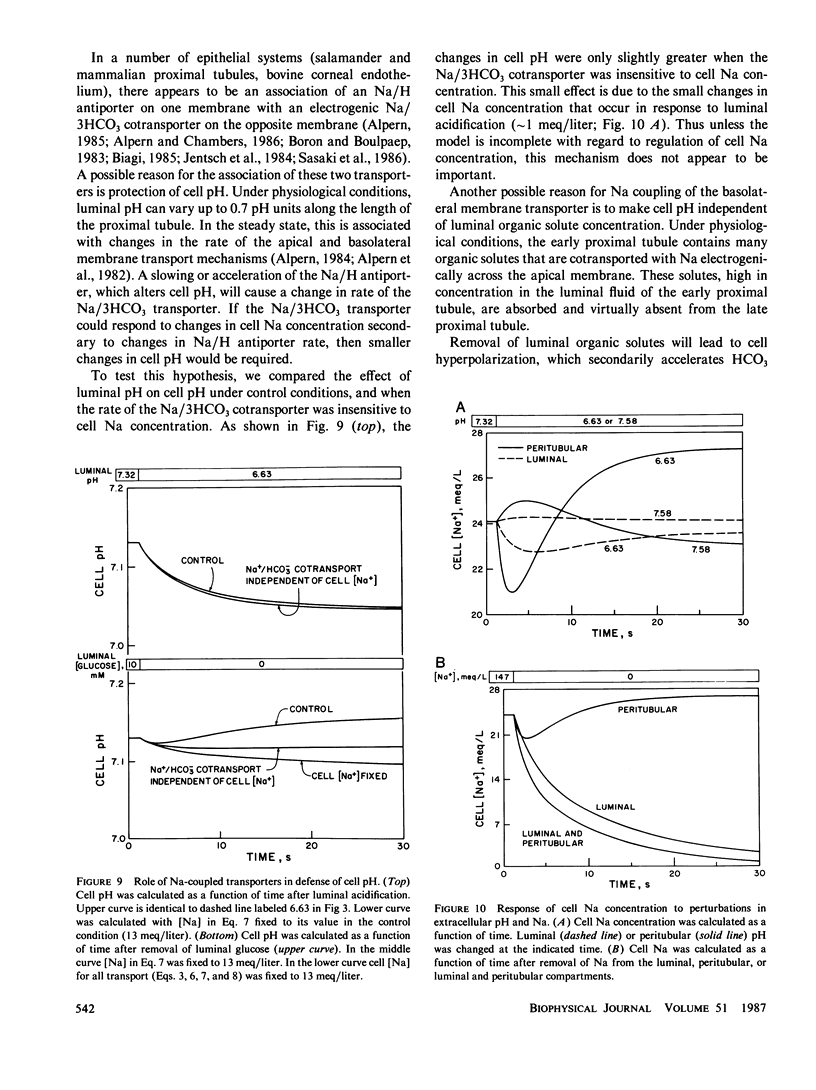
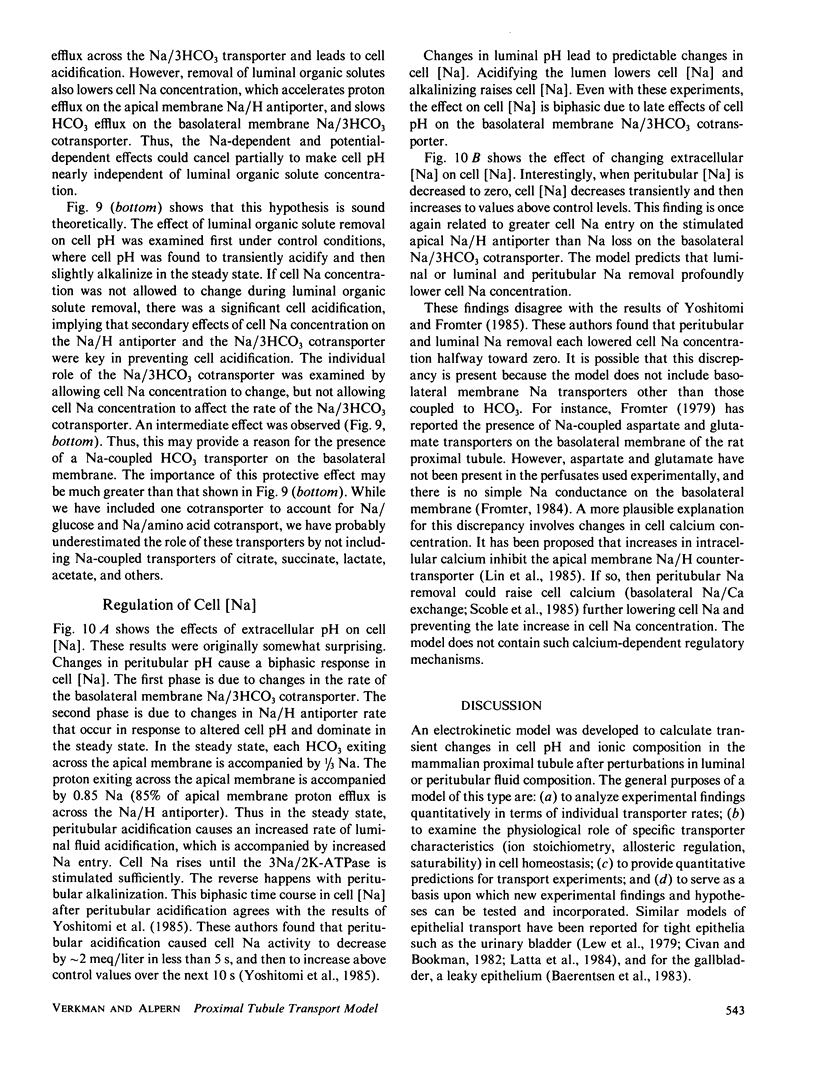
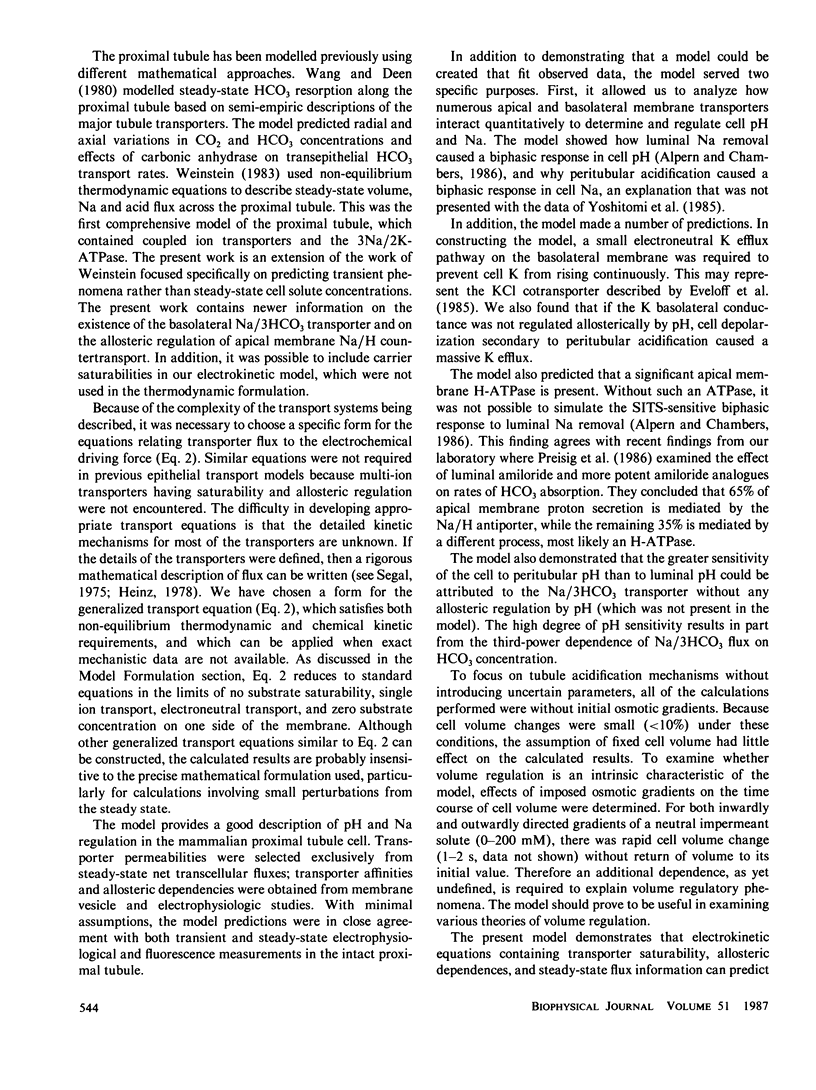
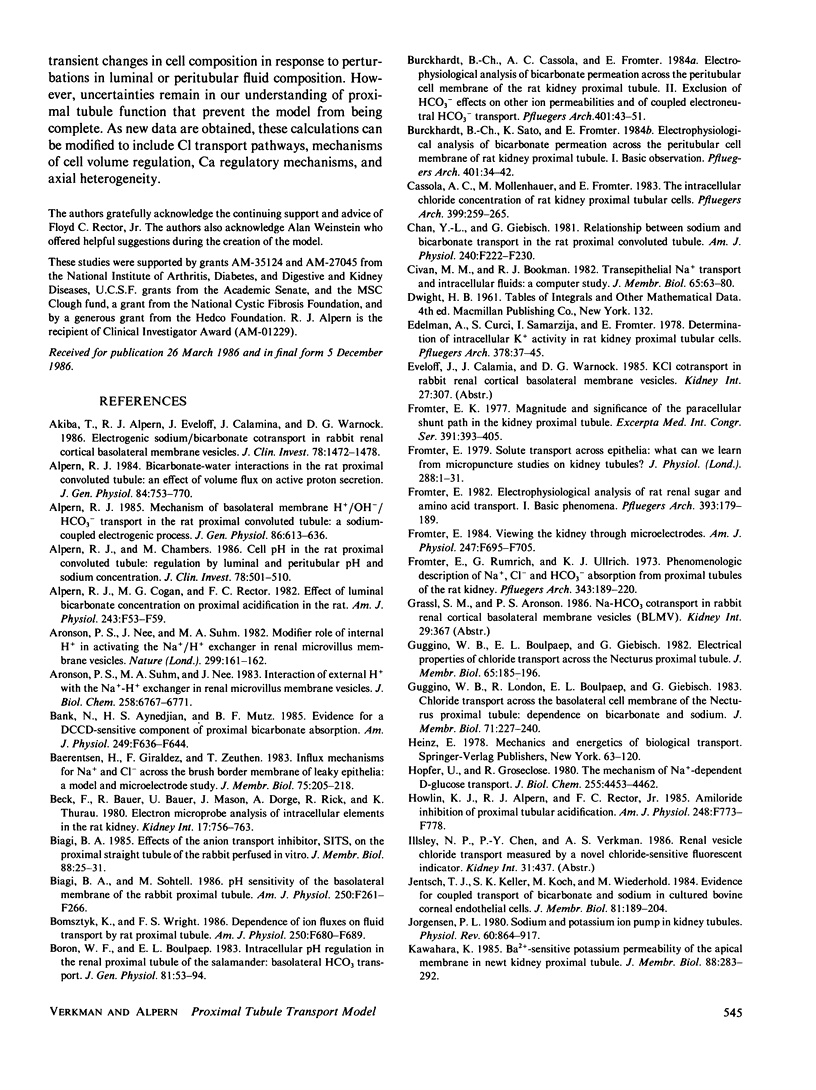
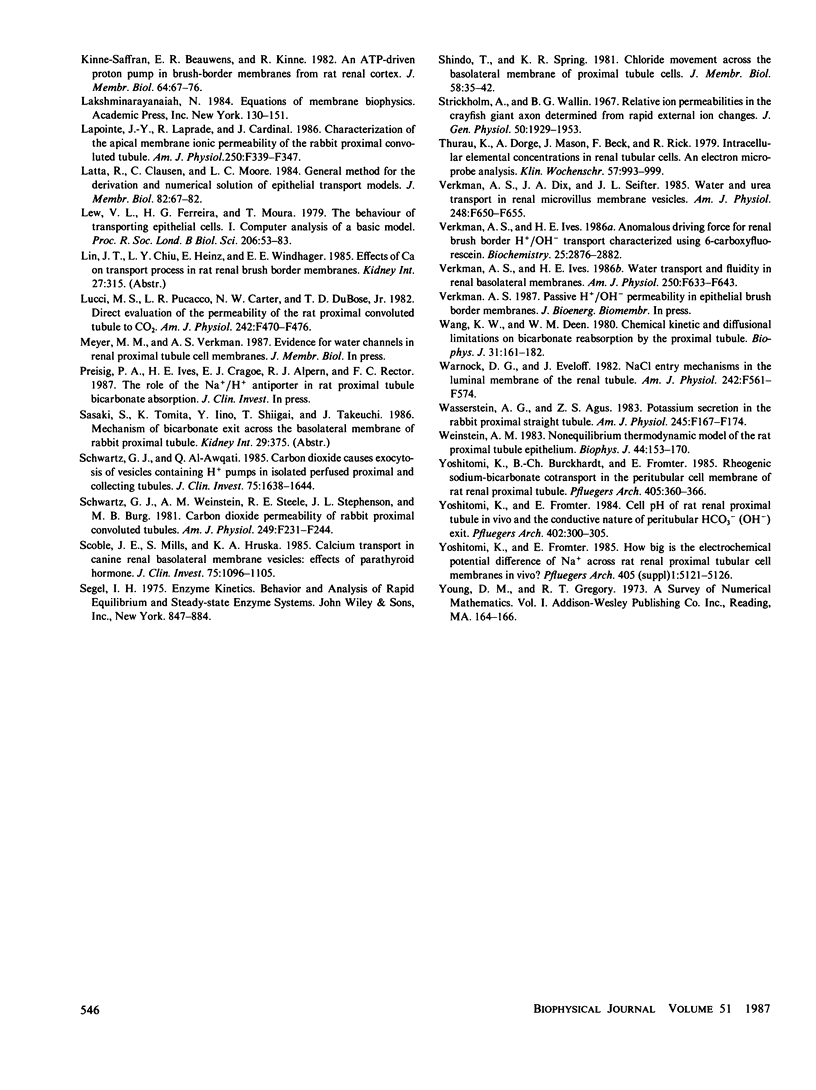
Selected References
These references are in PubMed. This may not be the complete list of references from this article.
- Akiba T., Alpern R. J., Eveloff J., Calamina J., Warnock D. G. Electrogenic sodium/bicarbonate cotransport in rabbit renal cortical basolateral membrane vesicles. J Clin Invest. 1986 Dec;78(6):1472–1478. doi: 10.1172/JCI112738. [DOI] [PMC free article] [PubMed] [Google Scholar]
- Alpern R. J. Bicarbonate-water interactions in the rat proximal convoluted tubule. An effect of volume flux on active proton secretion. J Gen Physiol. 1984 Nov;84(5):753–770. doi: 10.1085/jgp.84.5.753. [DOI] [PMC free article] [PubMed] [Google Scholar]
- Alpern R. J., Cogan M. G., Rector F. C., Jr Effect of luminal bicarbonate concentration on proximal acidification in the rat. Am J Physiol. 1982 Jul;243(1):F53–F59. doi: 10.1152/ajprenal.1982.243.1.F53. [DOI] [PubMed] [Google Scholar]
- Alpern R. J. Mechanism of basolateral membrane H+/OH-/HCO-3 transport in the rat proximal convoluted tubule. A sodium-coupled electrogenic process. J Gen Physiol. 1985 Nov;86(5):613–636. doi: 10.1085/jgp.86.5.613. [DOI] [PMC free article] [PubMed] [Google Scholar]
- Aronson P. S., Nee J., Suhm M. A. Modifier role of internal H+ in activating the Na+-H+ exchanger in renal microvillus membrane vesicles. Nature. 1982 Sep 9;299(5879):161–163. doi: 10.1038/299161a0. [DOI] [PubMed] [Google Scholar]
- Aronson P. S., Suhm M. A., Nee J. Interaction of external H+ with the Na+-H+ exchanger in renal microvillus membrane vesicles. J Biol Chem. 1983 Jun 10;258(11):6767–6771. [PubMed] [Google Scholar]
- Baerentsen H., Giraldez F., Zeuthen T. Influx mechanisms for Na+ and Cl- across the brush border membrane of leaky epithelia: a model and microelectrode study. J Membr Biol. 1983;75(3):205–218. doi: 10.1007/BF01871951. [DOI] [PubMed] [Google Scholar]
- Bank N., Aynedjian H. S., Mutz B. F. Evidence for a DCCD-sensitive component of proximal bicarbonate reabsorption. Am J Physiol. 1985 Nov;249(5 Pt 2):F636–F644. doi: 10.1152/ajprenal.1985.249.5.F636. [DOI] [PubMed] [Google Scholar]
- Beck F., Bauer R., Bauer U., Mason J., Dörge A., Rick R., Thurau K. Electron microprobe analysis of intracellular elements in the rat kidney. Kidney Int. 1980 Jun;17(6):756–763. doi: 10.1038/ki.1980.88. [DOI] [PubMed] [Google Scholar]
- Biagi B. A. Effects of the anion transport inhibitor, SITS, on the proximal straight tubule of the rabbit perfused in vitro. J Membr Biol. 1985;88(1):25–31. doi: 10.1007/BF01871210. [DOI] [PubMed] [Google Scholar]
- Biagi B. A., Sohtell M. pH sensitivity of the basolateral membrane of the rabbit proximal tubule. Am J Physiol. 1986 Feb;250(2 Pt 2):F261–F266. doi: 10.1152/ajprenal.1986.250.2.F261. [DOI] [PubMed] [Google Scholar]
- Bomsztyk K., Wright F. S. Dependence of ion fluxes on fluid transport by rat proximal tubule. Am J Physiol. 1986 Apr;250(4 Pt 2):F680–F689. doi: 10.1152/ajprenal.1986.250.4.F680. [DOI] [PubMed] [Google Scholar]
- Boron W. F., Boulpaep E. L. Intracellular pH regulation in the renal proximal tubule of the salamander. Basolateral HCO3- transport. J Gen Physiol. 1983 Jan;81(1):53–94. doi: 10.1085/jgp.81.1.53. [DOI] [PMC free article] [PubMed] [Google Scholar]
- Burckhardt B. C., Cassola A. C., Frömter E. Electrophysiological analysis of bicarbonate permeation across the peritubular cell membrane of rat kidney proximal tubule. II. Exclusion of HCO3(-)-effects on other ion permeabilities and of coupled electroneutral HCO3(-)-transport. Pflugers Arch. 1984 May;401(1):43–51. doi: 10.1007/BF00581531. [DOI] [PubMed] [Google Scholar]
- Burckhardt B. C., Sato K., Frömter E. Electrophysiological analysis of bicarbonate permeation across the peritubular cell membrane of rat kidney proximal tubule. I. Basic observations. Pflugers Arch. 1984 May;401(1):34–42. doi: 10.1007/BF00581530. [DOI] [PubMed] [Google Scholar]
- Cassola A. C., Mollenhauer M., Frömter E. The intracellular chloride activity of rat kidney proximal tubular cells. Pflugers Arch. 1983 Dec;399(4):259–265. doi: 10.1007/BF00652749. [DOI] [PubMed] [Google Scholar]
- Chan Y. L., Giebisch G. Relationship between sodium and bicarbonate transport in the rat proximal convoluted tubule. Am J Physiol. 1981 Mar;240(3):F222–F230. doi: 10.1152/ajprenal.1981.240.3.F222. [DOI] [PubMed] [Google Scholar]
- Civan M. M., Bookman R. J. Transepithelial Na+ transport and the intracellular fluids: a computer study. J Membr Biol. 1982;65(1-2):63–80. doi: 10.1007/BF01870470. [DOI] [PubMed] [Google Scholar]
- Edelman A., Curci S., Samarzija I., Frömter E. Determination of intracellular K+ activity in rat kidney proximal tubular cells. Pflugers Arch. 1978 Dec 15;378(1):37–45. doi: 10.1007/BF00581956. [DOI] [PubMed] [Google Scholar]
- Frömter E. Electrophysiological analysis of rat renal sugar and amino acid transport. I. Basic phenomena. Pflugers Arch. 1982 Apr;393(2):179–189. doi: 10.1007/BF00582942. [DOI] [PubMed] [Google Scholar]
- Frömter E., Rumrich G., Ullrich K. J. Phenomenologic description of Na+, Cl- and HCO-3 absorption from proximal tubules of rat kidney. Pflugers Arch. 1973 Oct 22;343(3):189–220. doi: 10.1007/BF00586045. [DOI] [PubMed] [Google Scholar]
- Frömter E. The Feldberg Lecture 1976. Solute transport across epithelia: what can we learn from micropuncture studies in kidney tubules? J Physiol. 1979 Mar;288:1–31. [PMC free article] [PubMed] [Google Scholar]
- Frömter E. Viewing the kidney through microelectrodes. Am J Physiol. 1984 Nov;247(5 Pt 2):F695–F705. doi: 10.1152/ajprenal.1984.247.5.F695. [DOI] [PubMed] [Google Scholar]
- Guggino W. B., Boulpaep E. L., Giebisch G. Electrical properties of chloride transport across the necturus proximal tubule. J Membr Biol. 1982;65(3):185–196. doi: 10.1007/BF01869962. [DOI] [PubMed] [Google Scholar]
- Guggino W. B., London R., Boulpaep E. L., Giebisch G. Chloride transport across the basolateral cell membrane of the Necturus proximal tubule: dependence on bicarbonate and sodium. J Membr Biol. 1983;71(3):227–240. doi: 10.1007/BF01875464. [DOI] [PubMed] [Google Scholar]
- Hopfer U., Groseclose R. The mechanism of Na+-dependent D-glucose transport. J Biol Chem. 1980 May 25;255(10):4453–4462. [PubMed] [Google Scholar]
- Howlin K. J., Alpern R. J., Rector F. C., Jr Amiloride inhibition of proximal tubular acidification. Am J Physiol. 1985 Jun;248(6 Pt 2):F773–F778. doi: 10.1152/ajprenal.1985.248.6.F773. [DOI] [PubMed] [Google Scholar]
- Jentsch T. J., Keller S. K., Koch M., Wiederholt M. Evidence for coupled transport of bicarbonate and sodium in cultured bovine corneal endothelial cells. J Membr Biol. 1984;81(3):189–204. doi: 10.1007/BF01868713. [DOI] [PubMed] [Google Scholar]
- Jørgensen P. L. Sodium and potassium ion pump in kidney tubules. Physiol Rev. 1980 Jul;60(3):864–917. doi: 10.1152/physrev.1980.60.3.864. [DOI] [PubMed] [Google Scholar]
- Kawahara K. Ba2+-sensitive potassium permeability of the apical membrane in newt kidney proximal tubule. J Membr Biol. 1985;88(3):283–292. doi: 10.1007/BF01871092. [DOI] [PubMed] [Google Scholar]
- Kinne-Saffran E., Beauwens R., Kinne R. An ATP-driven proton pump in brush-border membranes from rat renal cortex. J Membr Biol. 1982;64(1-2):67–76. doi: 10.1007/BF01870769. [DOI] [PubMed] [Google Scholar]
- Lapointe J. Y., Laprade R., Cardinal J. Characterization of the apical membrane ionic permeability of the rabbit proximal convoluted tubule. Am J Physiol. 1986 Feb;250(2 Pt 2):F339–F347. doi: 10.1152/ajprenal.1986.250.2.F339. [DOI] [PubMed] [Google Scholar]
- Latta R., Clausen C., Moore L. C. General method for the derivation and numerical solution of epithelial transport models. J Membr Biol. 1984;82(1):67–82. doi: 10.1007/BF01870733. [DOI] [PubMed] [Google Scholar]
- Lew V. L., Ferreira H. G., Moura T. The behaviour of transporting epithelial cells. I. Computer analysis of a basic model. Proc R Soc Lond B Biol Sci. 1979 Nov 30;206(1162):53–83. doi: 10.1098/rspb.1979.0091. [DOI] [PubMed] [Google Scholar]
- Lucci M. S., Pucacco L. R., Carter N. W., DuBose T. D., Jr Direct evaluation of the permeability of the rat proximal convoluted tubule to CO2. Am J Physiol. 1982 May;242(5):F470–F476. doi: 10.1152/ajprenal.1982.242.5.F470. [DOI] [PubMed] [Google Scholar]
- Schwartz G. J., Al-Awqati Q. Carbon dioxide causes exocytosis of vesicles containing H+ pumps in isolated perfused proximal and collecting tubules. J Clin Invest. 1985 May;75(5):1638–1644. doi: 10.1172/JCI111871. [DOI] [PMC free article] [PubMed] [Google Scholar]
- Schwartz G. J., Weinstein A. M., Steele R. E., Stephenson J. L., Burg M. B. Carbon dioxide permeability of rabbit proximal convoluted tubules. Am J Physiol. 1981 Mar;240(3):F231–F244. doi: 10.1152/ajprenal.1981.240.3.F231. [DOI] [PubMed] [Google Scholar]
- Scoble J. E., Mills S., Hruska K. A. Calcium transport in canine renal basolateral membrane vesicles. Effects of parathyroid hormone. J Clin Invest. 1985 Apr;75(4):1096–1105. doi: 10.1172/JCI111803. [DOI] [PMC free article] [PubMed] [Google Scholar]
- Shindo T., Spring K. R. Chloride movement across the basolateral membrane of proximal tubule cells. J Membr Biol. 1981 Jan 30;58(1):35–42. doi: 10.1007/BF01871032. [DOI] [PubMed] [Google Scholar]
- Strickholm A., Wallin B. G. Relative ion permeabilities in the crayfish giant axon determined from rapid external ion changes. J Gen Physiol. 1967 Aug;50(7):1929–1953. doi: 10.1085/jgp.50.7.1929. [DOI] [PMC free article] [PubMed] [Google Scholar]
- Thurau K., Dörge A., Mason J., Beck F., Rick R. Intracellular elemental concentrations in renal tubular cells. An electron microprobe analysis. Klin Wochenschr. 1979 Oct 1;57(19):993–999. doi: 10.1007/BF01479984. [DOI] [PubMed] [Google Scholar]
- Verkman A. S., Dix J. A., Seifter J. L. Water and urea transport in renal microvillus membrane vesicles. Am J Physiol. 1985 May;248(5 Pt 2):F650–F655. doi: 10.1152/ajprenal.1985.248.5.F650. [DOI] [PubMed] [Google Scholar]
- Verkman A. S., Ives H. E. Anomalous driving force for renal brush border H+/OH-transport characterized by using 6-carboxyfluorescein. Biochemistry. 1986 May 20;25(10):2876–2882. doi: 10.1021/bi00358a021. [DOI] [PubMed] [Google Scholar]
- Verkman A. S., Ives H. E. Water permeability and fluidity of renal basolateral membranes. Am J Physiol. 1986 Apr;250(4 Pt 2):F633–F643. doi: 10.1152/ajprenal.1986.250.4.F633. [DOI] [PubMed] [Google Scholar]
- Wang K. W., Deen W. M. Chemical kinetic and diffusional limitations on bicarbonate reabsorption by the proximal tubule. Biophys J. 1980 Aug;31(2):161–182. doi: 10.1016/S0006-3495(80)85048-X. [DOI] [PMC free article] [PubMed] [Google Scholar]
- Warnock D. G., Eveloff J. NaCl entry mechanisms in the luminal membrane of the renal tubule. Am J Physiol. 1982 Jun;242(6):F561–F574. doi: 10.1152/ajprenal.1982.242.6.F561. [DOI] [PubMed] [Google Scholar]
- Wasserstein A. G., Agus Z. S. Potassium secretion in the rabbit proximal straight tubule. Am J Physiol. 1983 Aug;245(2):F167–F174. doi: 10.1152/ajprenal.1983.245.2.F167. [DOI] [PubMed] [Google Scholar]
- Weinstein A. M. Nonequilibrium thermodynamic model of the rat proximal tubule epithelium. Biophys J. 1983 Nov;44(2):153–170. doi: 10.1016/S0006-3495(83)84287-8. [DOI] [PMC free article] [PubMed] [Google Scholar]
- Yoshitomi K., Burckhardt B. C., Frömter E. Rheogenic sodium-bicarbonate cotransport in the peritubular cell membrane of rat renal proximal tubule. Pflugers Arch. 1985 Dec;405(4):360–366. doi: 10.1007/BF00595689. [DOI] [PubMed] [Google Scholar]
- Yoshitomi K., Frömter E. Cell pH of rat renal proximal tubule in vivo and the conductive nature of peritubular HCO3- (OH-) exit. Pflugers Arch. 1984 Nov;402(3):300–305. doi: 10.1007/BF00585513. [DOI] [PubMed] [Google Scholar]