Abstract
Enhanced resolution of rapid and complex anisotropy decays was obtained by measurement and analysis of data from progressively quenched samples. Collisional quenching by acrylamide was used to vary the mean decay time of indole or of the tryptophan fluorescence from melittin. Anisotropy decays were obtained from the frequency-response of the polarized emission at frequencies from 4 to 2,000 MHz. Quenching increases the fraction of the total emission, which occurs on the subnanosecond timescale, and thereby provides increased information on picosecond rotational motions or local motions in proteins. For monoexponential subnanosecond anisotropy decays, enhanced resolution is obtained by measurement of the most highly quenched samples. For complex anisotropy decays, such as those due to both local motions and overall protein rotational diffusion, superior resolution is obtained by simultaneous analysis of data from quenched and unquenched samples. We demonstrate that measurement of quenched samples greatly reduces the uncertainty of the 50-ps correlation time of indole in water at 20 degrees C, and allows resolution of the anisotropic rotation of indole with correlation times of 140 and 720 ps. The method was applied to melittin in the monomeric and tetrameric forms. With increased quenching, the anisotropy data showed decreasing contributions from overall protein rotation and increased contribution from picosecond tryptophan motions. The tryptophan residues in both the monomeric and the tetrameric forms of melittin displayed substantial local motions with correlation times near 0.16 and 0.06 ns, respectively. The amplitude of the local motion is twofold less in the tetramer. These highly resolved anisotropy decays should be valuable for comparison with molecular dynamics simulations of melittin.
Full text
PDF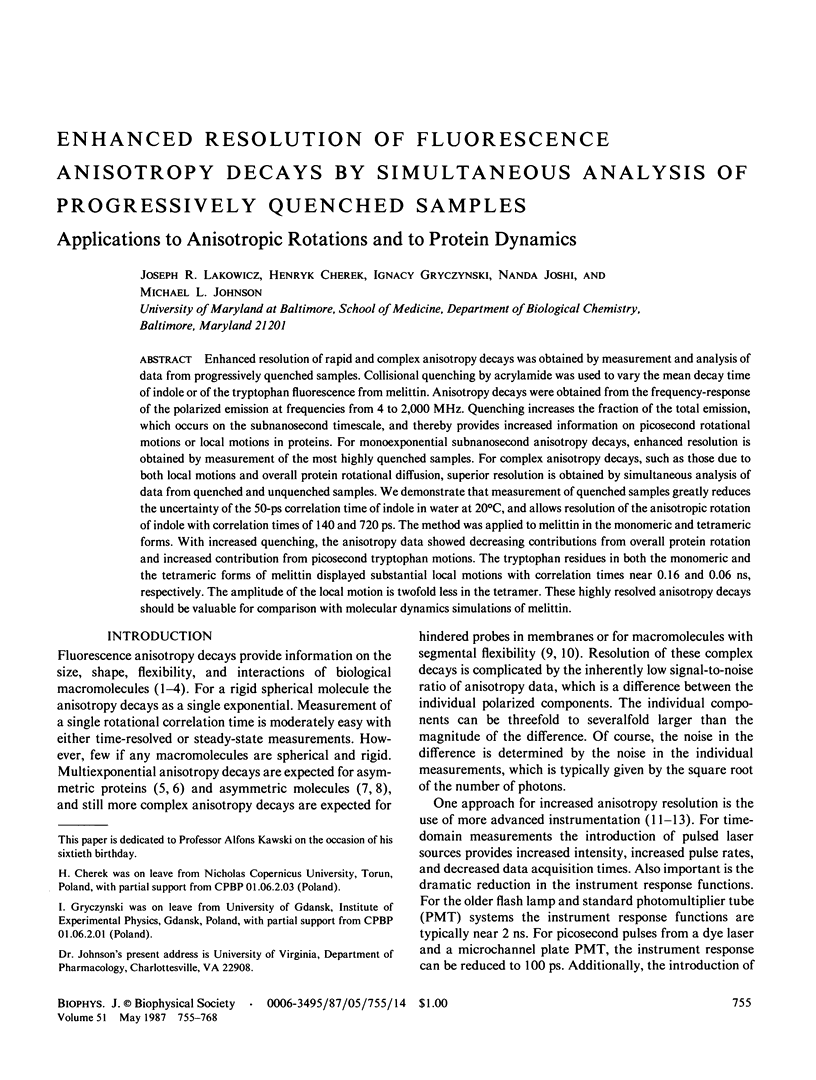
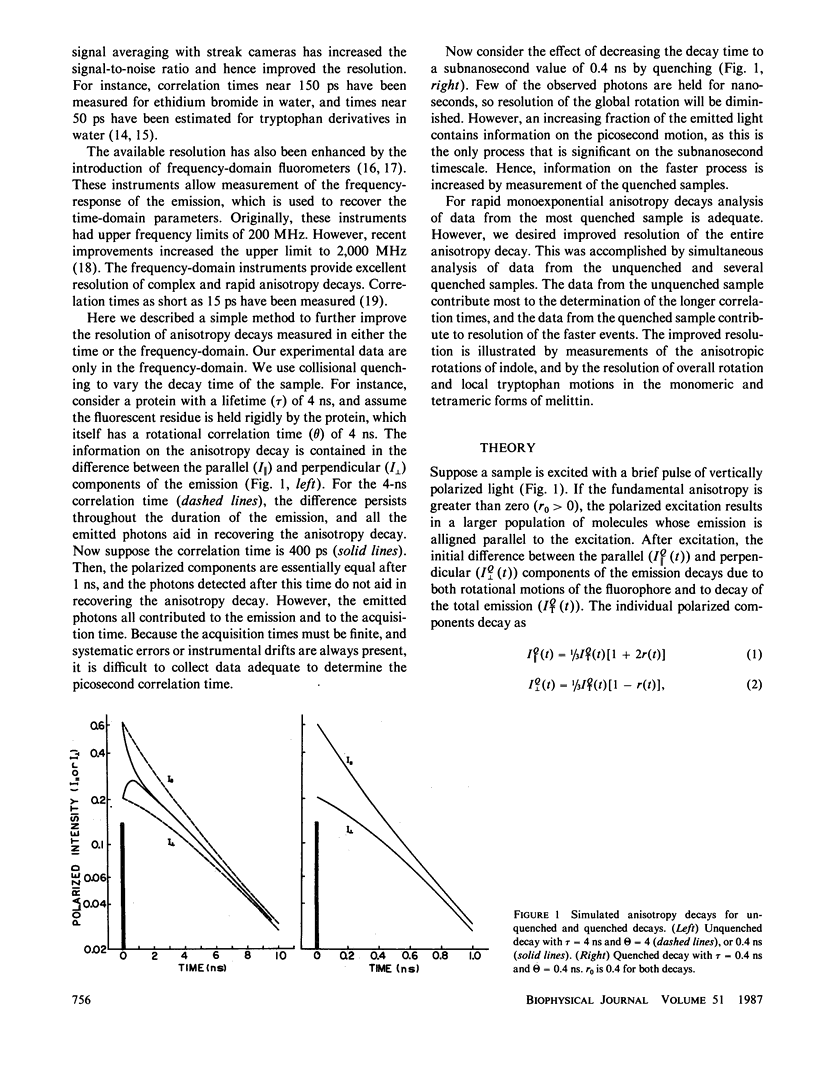
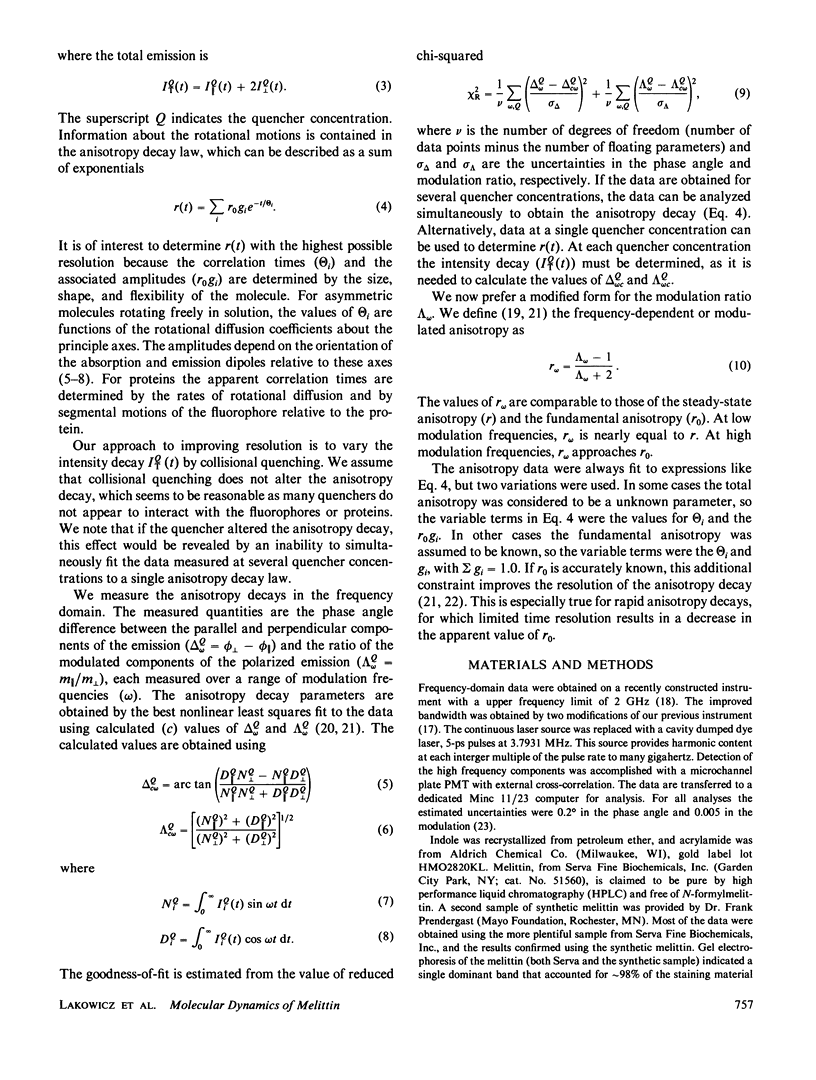
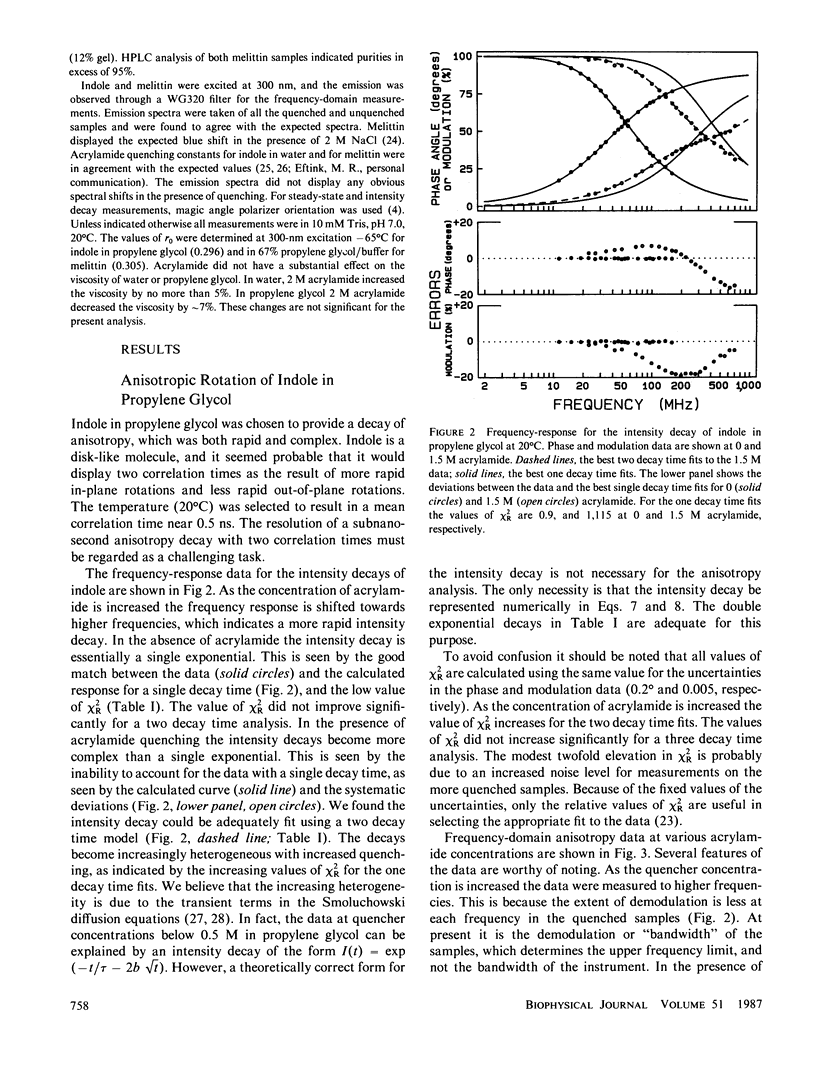
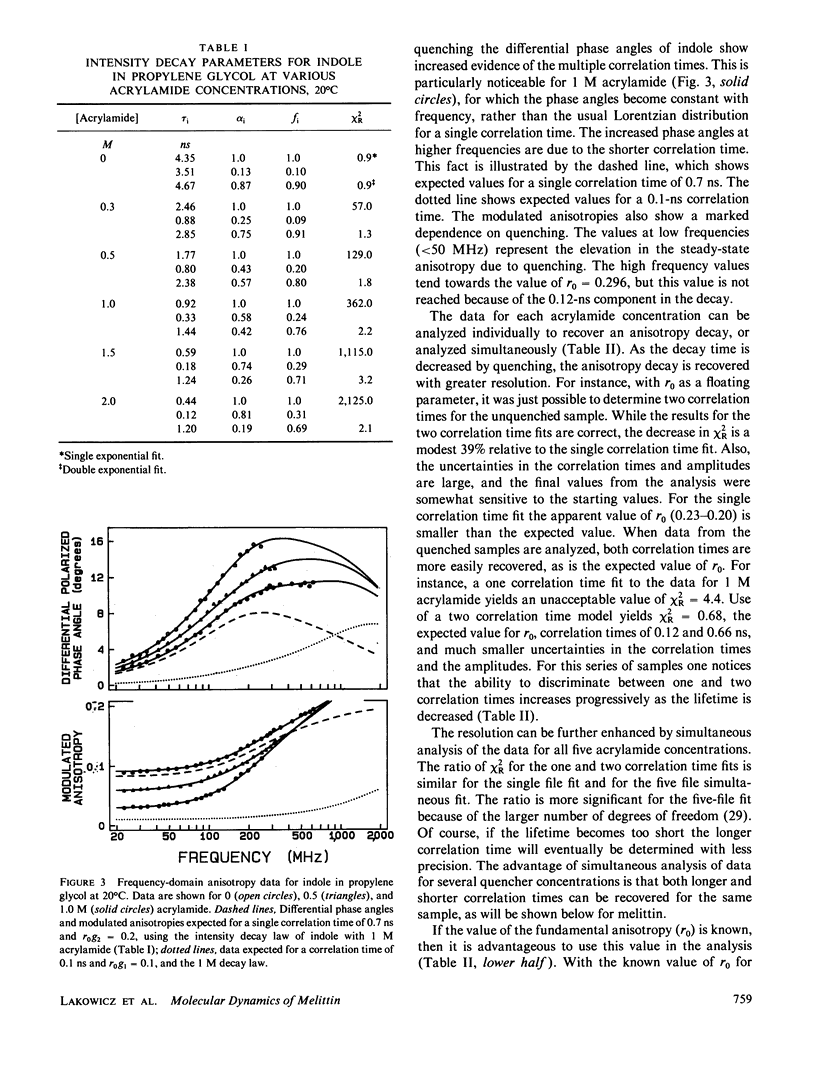
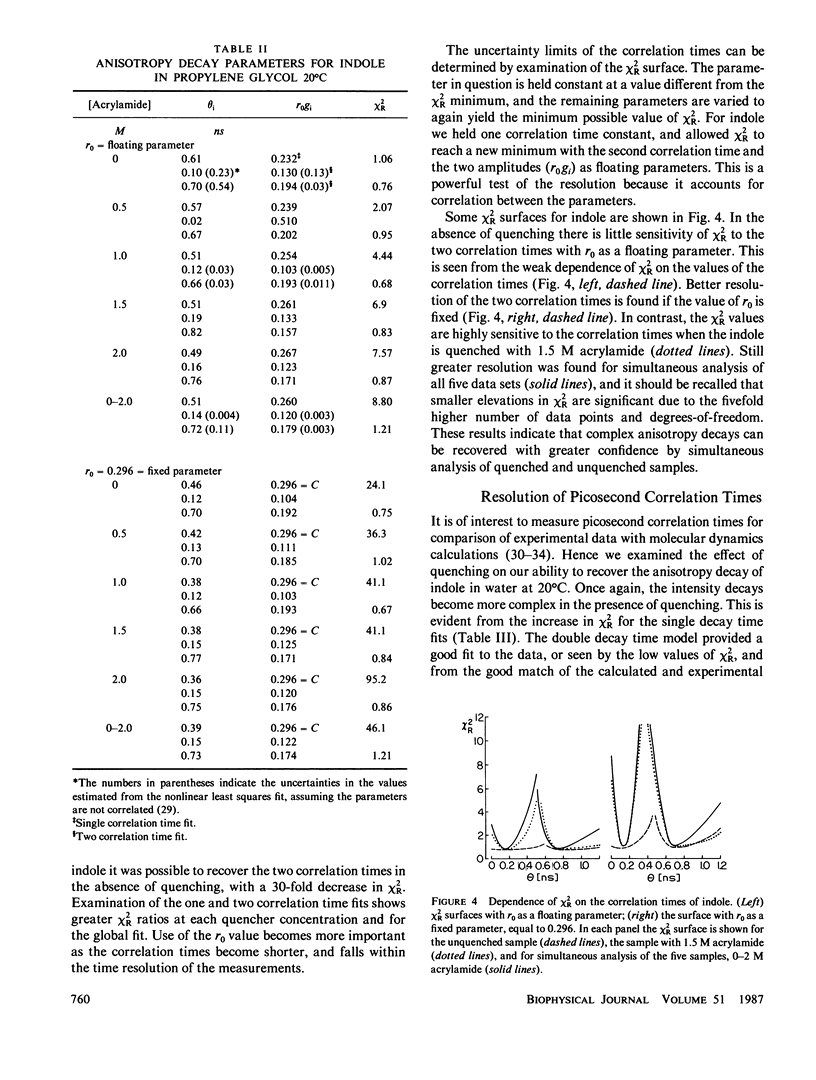
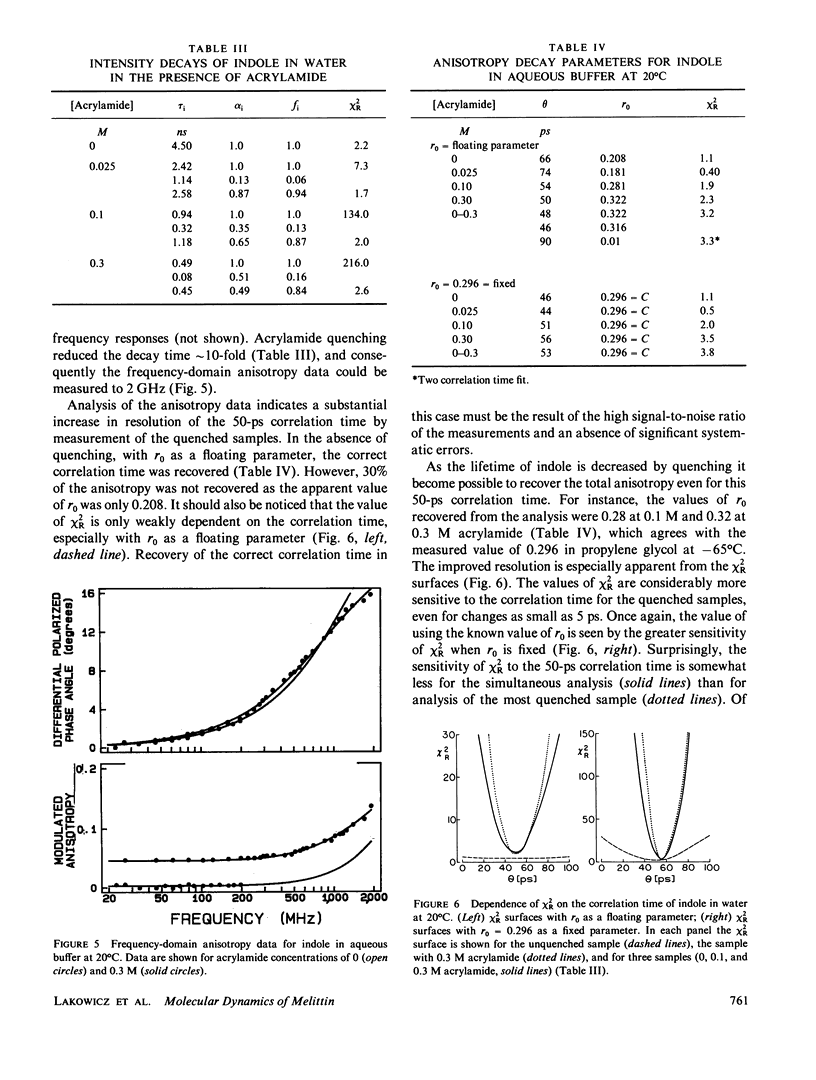
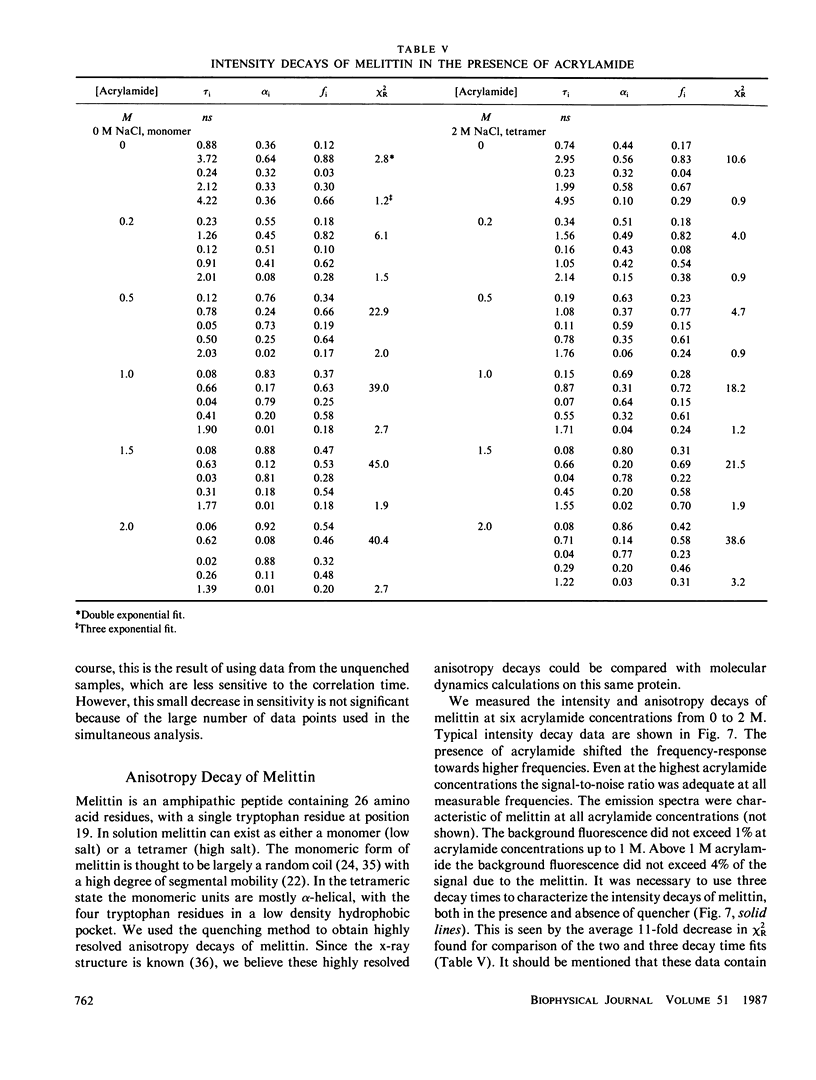
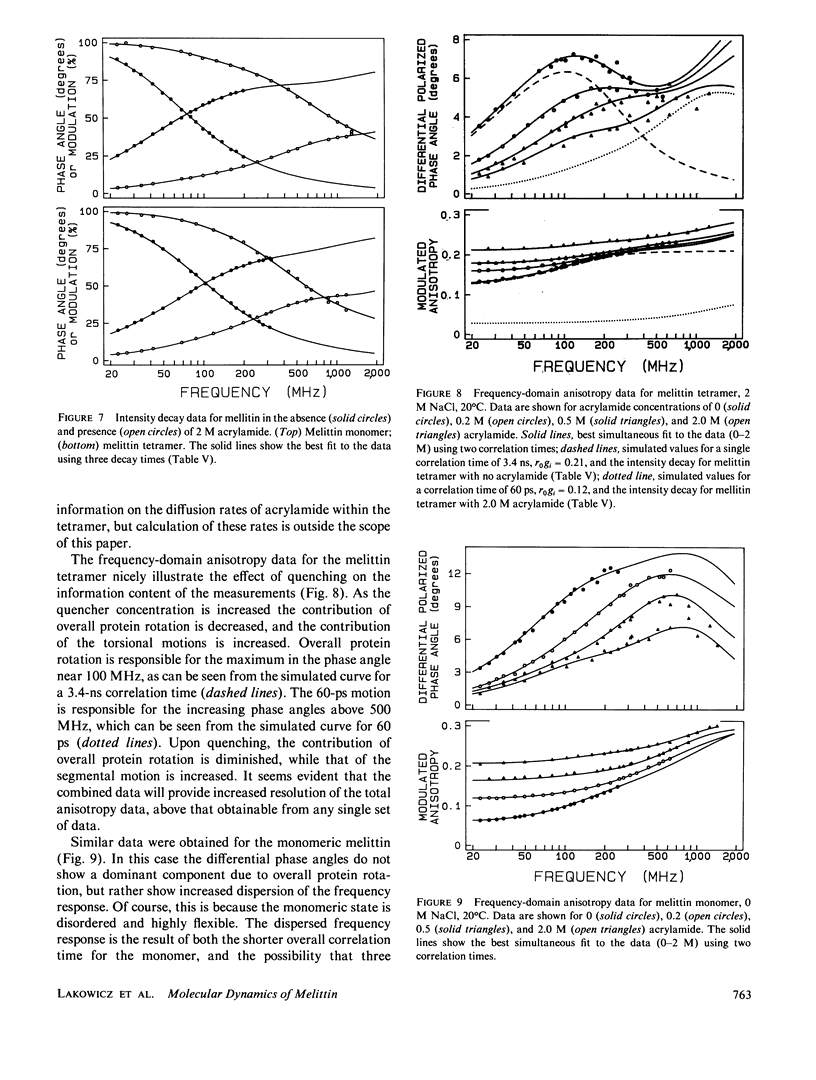
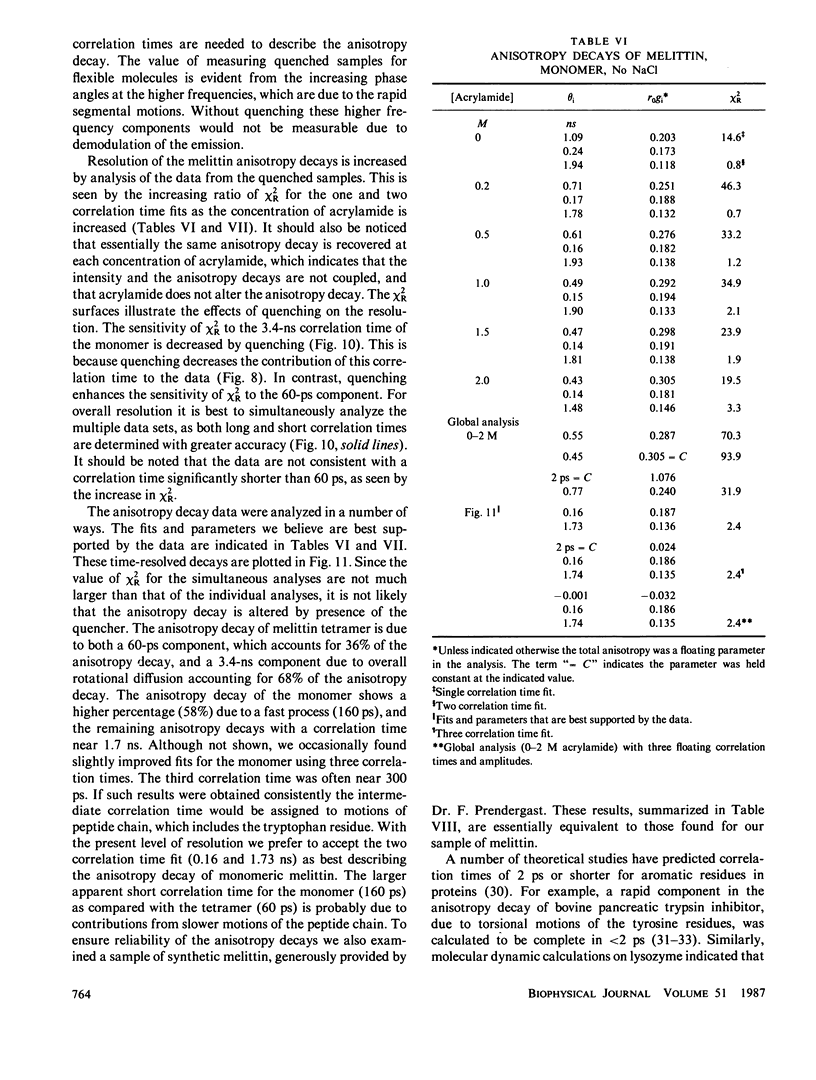
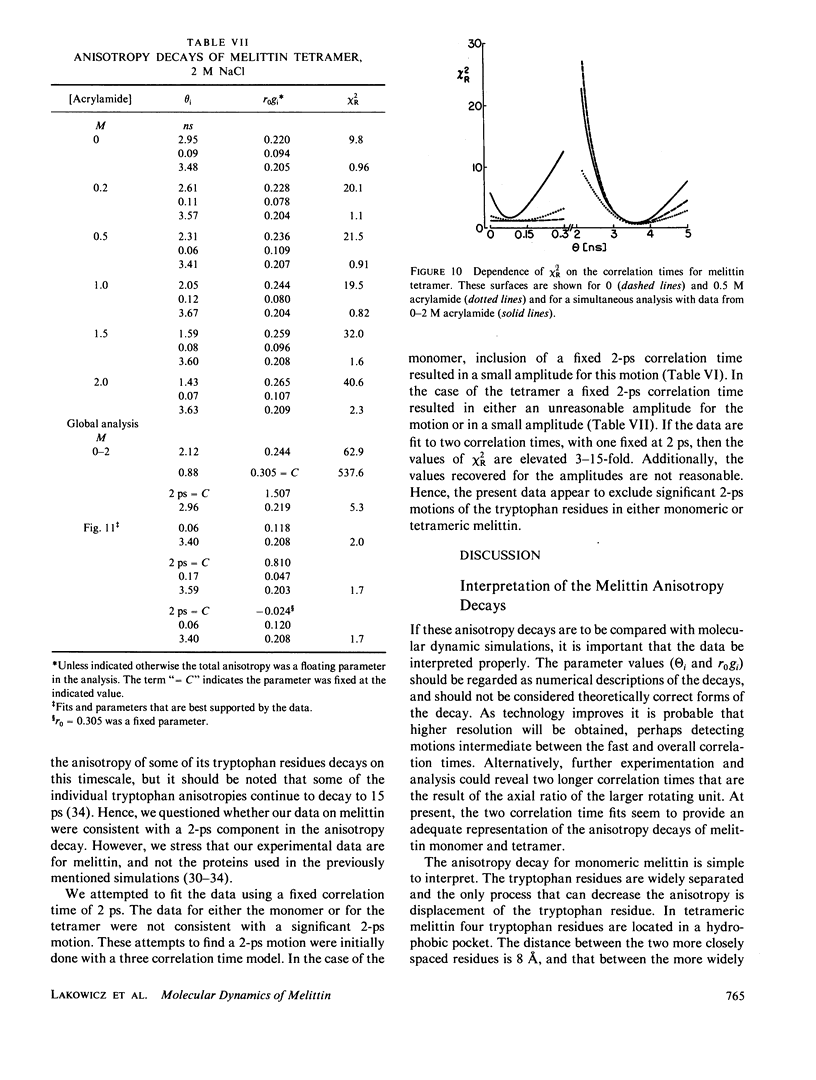
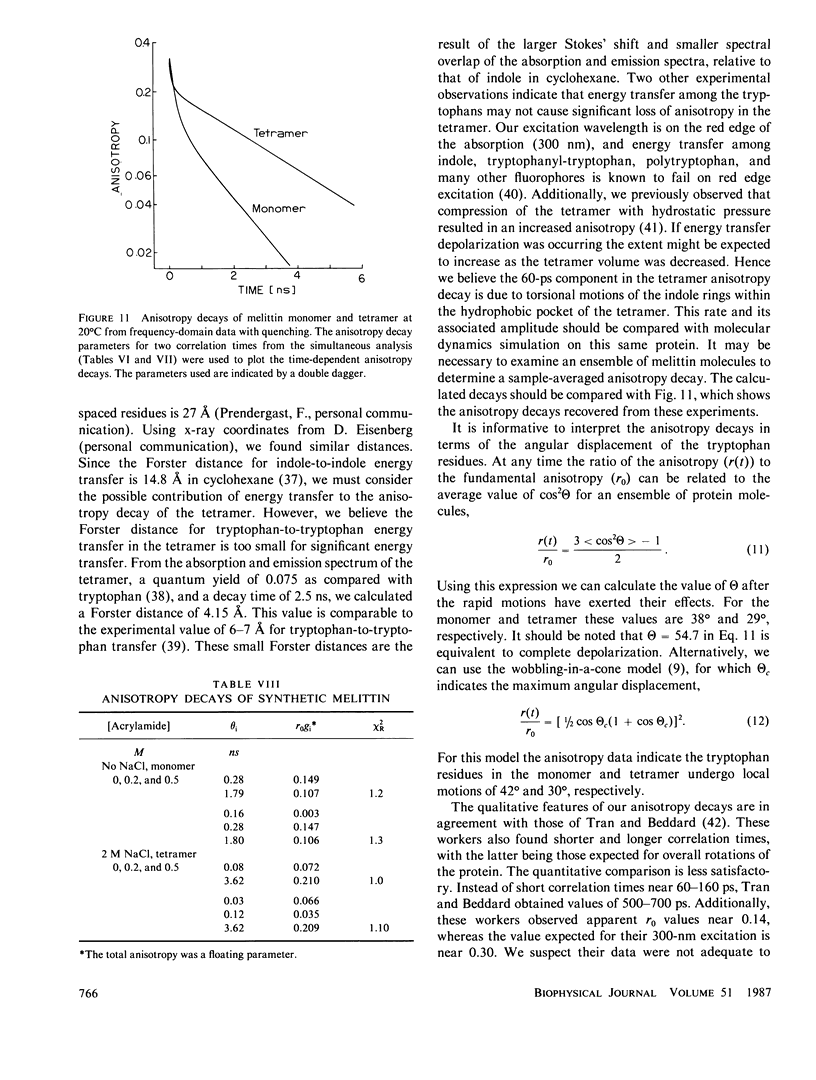
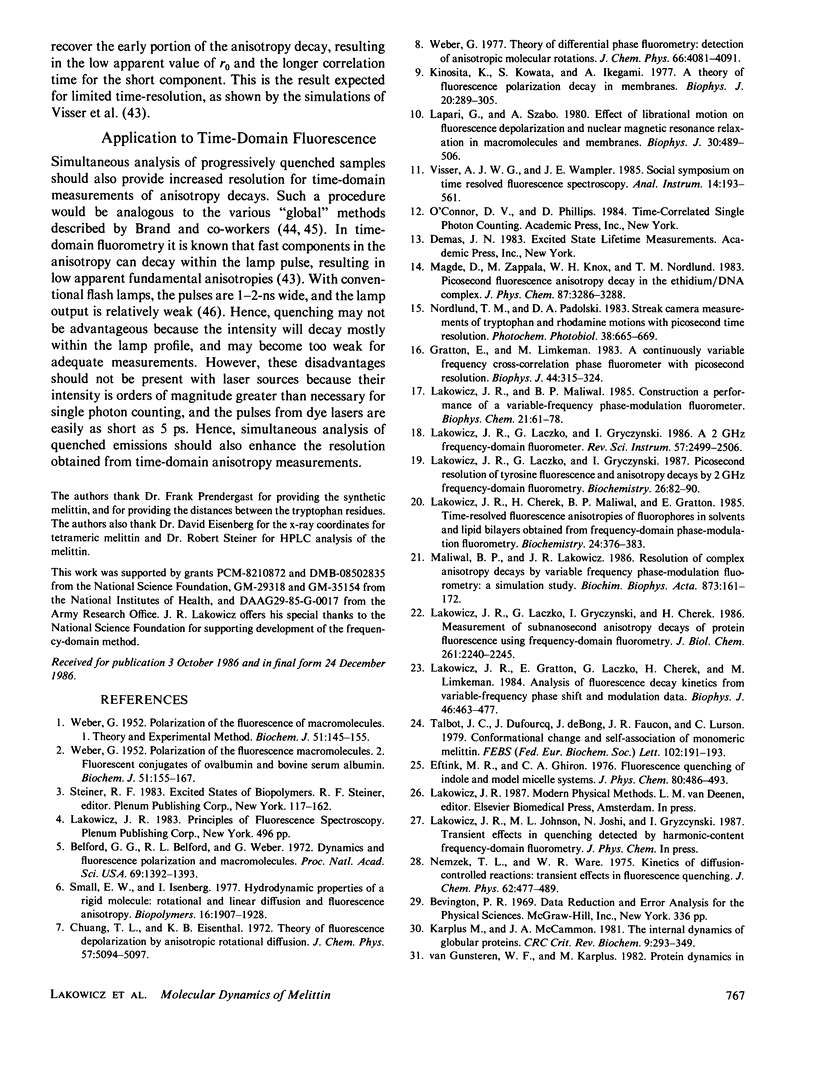
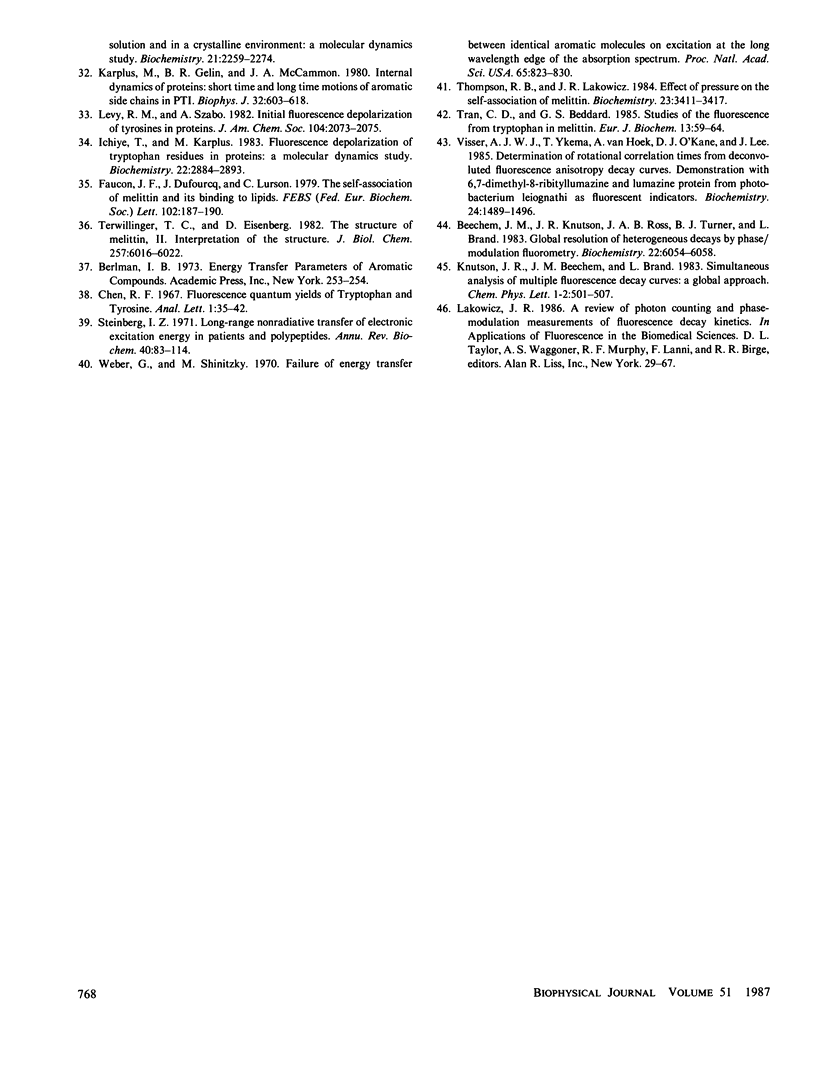
Selected References
These references are in PubMed. This may not be the complete list of references from this article.
- Belford G. G., Belford R. L., Weber G. Dynamics of fluorescence polarization in macromolecules. Proc Natl Acad Sci U S A. 1972 Jun;69(6):1392–1393. doi: 10.1073/pnas.69.6.1392. [DOI] [PMC free article] [PubMed] [Google Scholar]
- Faucon J. F., Dufourcq J., Lussan C. The self-association of melittin and its binding to lipids: an intrinsic fluorescence polarization study. FEBS Lett. 1979 Jun 1;102(1):187–190. doi: 10.1016/0014-5793(79)80956-4. [DOI] [PubMed] [Google Scholar]
- Gratton E., Limkeman M. A continuously variable frequency cross-correlation phase fluorometer with picosecond resolution. Biophys J. 1983 Dec;44(3):315–324. doi: 10.1016/S0006-3495(83)84305-7. [DOI] [PMC free article] [PubMed] [Google Scholar]
- Ichiye T., Karplus M. Fluorescence depolarization of tryptophan residues in proteins: a molecular dynamics study. Biochemistry. 1983 Jun 7;22(12):2884–2893. doi: 10.1021/bi00281a017. [DOI] [PubMed] [Google Scholar]
- Karplus M., Gelin B. R., McCammon J. A. Internal dynamics of proteins. Short time and long time motions of aromatic sidechains in PTI. Biophys J. 1980 Oct;32(1):603–618. doi: 10.1016/S0006-3495(80)84993-9. [DOI] [PMC free article] [PubMed] [Google Scholar]
- Karplus M., McCammon J. A. The internal dynamics of globular proteins. CRC Crit Rev Biochem. 1981;9(4):293–349. doi: 10.3109/10409238109105437. [DOI] [PubMed] [Google Scholar]
- Kinosita K., Jr, Kawato S., Ikegami A. A theory of fluorescence polarization decay in membranes. Biophys J. 1977 Dec;20(3):289–305. doi: 10.1016/S0006-3495(77)85550-1. [DOI] [PMC free article] [PubMed] [Google Scholar]
- Lakowicz J. R., Cherek H., Maliwal B. P. Time-resolved fluorescence anisotropies of diphenylhexatriene and perylene in solvents and lipid bilayers obtained from multifrequency phase-modulation fluorometry. Biochemistry. 1985 Jan 15;24(2):376–383. doi: 10.1021/bi00323a021. [DOI] [PMC free article] [PubMed] [Google Scholar]
- Lakowicz J. R., Laczko G., Cherek H., Gratton E., Limkeman M. Analysis of fluorescence decay kinetics from variable-frequency phase shift and modulation data. Biophys J. 1984 Oct;46(4):463–477. doi: 10.1016/S0006-3495(84)84043-6. [DOI] [PMC free article] [PubMed] [Google Scholar]
- Lakowicz J. R., Laczko G., Gryczynski I., Cherek H. Measurement of subnanosecond anisotropy decays of protein fluorescence using frequency-domain fluorometry. J Biol Chem. 1986 Feb 15;261(5):2240–2245. [PubMed] [Google Scholar]
- Lakowicz J. R., Laczko G., Gryczynski I. Picosecond resolution of tyrosine fluorescence and anisotropy decays by 2-GHz frequency-domain fluorometry. Biochemistry. 1987 Jan 13;26(1):82–90. doi: 10.1021/bi00375a012. [DOI] [PMC free article] [PubMed] [Google Scholar]
- Lakowicz J. R., Maliwal B. P. Construction and performance of a variable-frequency phase-modulation fluorometer. Biophys Chem. 1985 Jan;21(1):61–78. doi: 10.1016/0301-4622(85)85007-9. [DOI] [PubMed] [Google Scholar]
- Lipari G., Szabo A. Effect of librational motion on fluorescence depolarization and nuclear magnetic resonance relaxation in macromolecules and membranes. Biophys J. 1980 Jun;30(3):489–506. doi: 10.1016/S0006-3495(80)85109-5. [DOI] [PMC free article] [PubMed] [Google Scholar]
- Maliwal B. P., Lakowicz J. R. Resolution of complex anisotropy decays by variable frequency phase-modulation fluorometry: a stimulation study. Biochim Biophys Acta. 1986 Sep 26;873(2):161–172. doi: 10.1016/0167-4838(86)90042-7. [DOI] [PubMed] [Google Scholar]
- Small E. W., Isenberg I. Hydrodynamic properties of a rigid molecule: rotational and linear diffusion and fluorescence anisotropy. Biopolymers. 1977 Sep;16(9):1907–1928. doi: 10.1002/bip.1977.360160907. [DOI] [PubMed] [Google Scholar]
- Steinberg I. Z. Long-range nonradiative transfer of electronic excitation energy in proteins and polypeptides. Annu Rev Biochem. 1971;40:83–114. doi: 10.1146/annurev.bi.40.070171.000503. [DOI] [PubMed] [Google Scholar]
- Talbot J. C., Dufourcq J., de Bony J., Faucon J. F., Lussan C. Conformational change and self association of monomeric melittin. FEBS Lett. 1979 Jun 1;102(1):191–193. doi: 10.1016/0014-5793(79)80957-6. [DOI] [PubMed] [Google Scholar]
- Terwilliger T. C., Eisenberg D. The structure of melittin. II. Interpretation of the structure. J Biol Chem. 1982 Jun 10;257(11):6016–6022. [PubMed] [Google Scholar]
- Thompson R. B., Lakowicz J. R. Effect of pressure on the self-association of melittin. Biochemistry. 1984 Jul 17;23(15):3411–3417. doi: 10.1021/bi00310a005. [DOI] [PMC free article] [PubMed] [Google Scholar]
- Tran C. D., Beddard G. S. Studies of the fluorescence from tryptophan in melittin. Eur Biophys J. 1985;13(1):59–64. doi: 10.1007/BF00266310. [DOI] [PubMed] [Google Scholar]
- Visser A. J., Ykema T., van Hoek A., O'Kane D. J., Lee J. Determination of rotational correlation times from deconvoluted fluorescence anisotropy decay curves. Demonstration with 6,7-dimethyl-8-ribityllumazine and lumazine protein from Photobacterium leiognathi as fluorescent indicators. Biochemistry. 1985 Mar 12;24(6):1489–1496. doi: 10.1021/bi00327a030. [DOI] [PubMed] [Google Scholar]
- WEBER G. Polarization of the fluorescence of macromolecules. I. Theory and experimental method. Biochem J. 1952 May;51(2):145–155. doi: 10.1042/bj0510145. [DOI] [PMC free article] [PubMed] [Google Scholar]
- WEBER G. Polarization of the fluorescence of macromolecules. II. Fluorescent conjugates of ovalbumin and bovine serum albumin. Biochem J. 1952 May;51(2):155–167. doi: 10.1042/bj0510155. [DOI] [PMC free article] [PubMed] [Google Scholar]
- Weber G., Shinitzky M. Failure of Energy Transfer between Identical Aromatic Molecules on Excitation at the Long Wave Edge of the Absorption Spectrum. Proc Natl Acad Sci U S A. 1970 Apr;65(4):823–830. doi: 10.1073/pnas.65.4.823. [DOI] [PMC free article] [PubMed] [Google Scholar]
- van Gunsteren W. F., Karplus M. Protein dynamics in solution and in a crystalline environment: a molecular dynamics study. Biochemistry. 1982 May 11;21(10):2259–2274. doi: 10.1021/bi00539a001. [DOI] [PubMed] [Google Scholar]