Abstract
The 77-K resonance Raman vibrational spectrum of intact goldfish rod photoreceptors containing 3,4-dehydro (A2) retinal is dominated by scattering from the 9-cis component of the steady state at all excitation wavelengths. Intact goldfish photoreceptors were regenerated with an A1-retinal chromophore to determine whether this behavior is caused by the protein or the chromophore. The resulting Raman spectrum was typical of an A1-pigment exhibiting significant scattering from all three components of the steady state: rhodopsin, bathorhodopsin, and isorhodopsin. Furthermore, regeneration of bovine opsin with A2-retinal produces a characteristic "A2-Raman spectrum" that is dominated by scattering from the 9-cis pigment. We conclude that the differences between the Raman spectra of the A1-and A2-pigments are caused by some intrinsic difference in the photochemical properties of the retinal chromophores. To quantitate these observations, the 77-K adsorption spectra and the photochemical quantum yields (phi) of the native A2-goldfish and the regenerated A2-bovine pigments were measured. In the goldfish A2-pigment, the value of phi 4 (9-cis----trans) is 0.05; phi 3 (trans----9-cis) is 0.10; and phi 2 (trans----11-cis) is 0.35. By contrast, in the bovine A1-pigment, these quantum yields are 0.10, 0.053, and 0.50, respectively. The reduced value of phi 4 and the increased value of phi 3 in the goldfish pigment confirms that the 9-cis isomer is photochemically more stable in A2-pigments.
Full text
PDF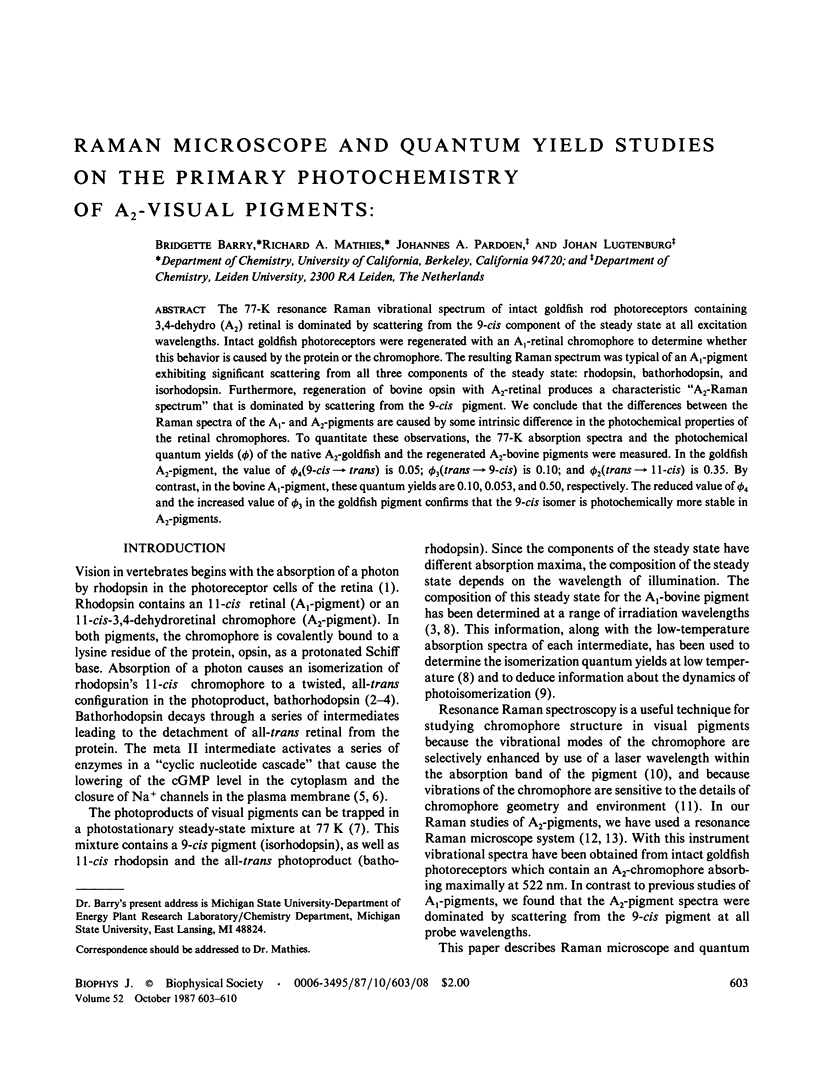
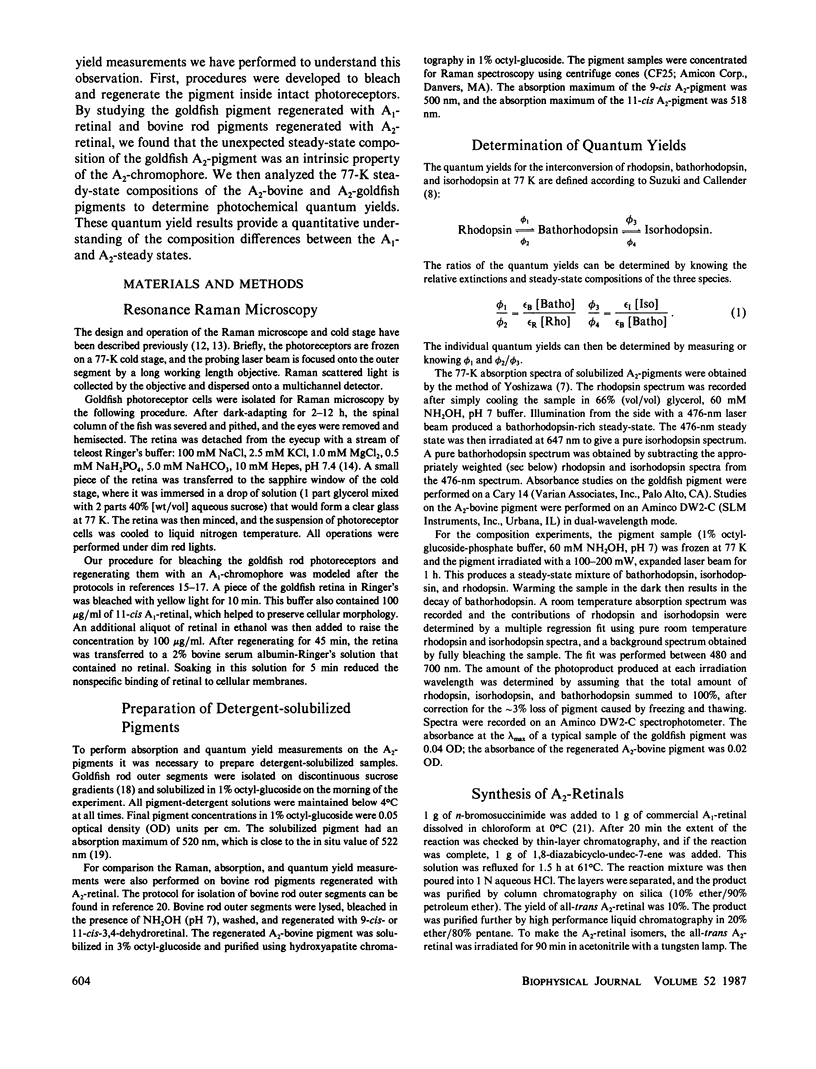
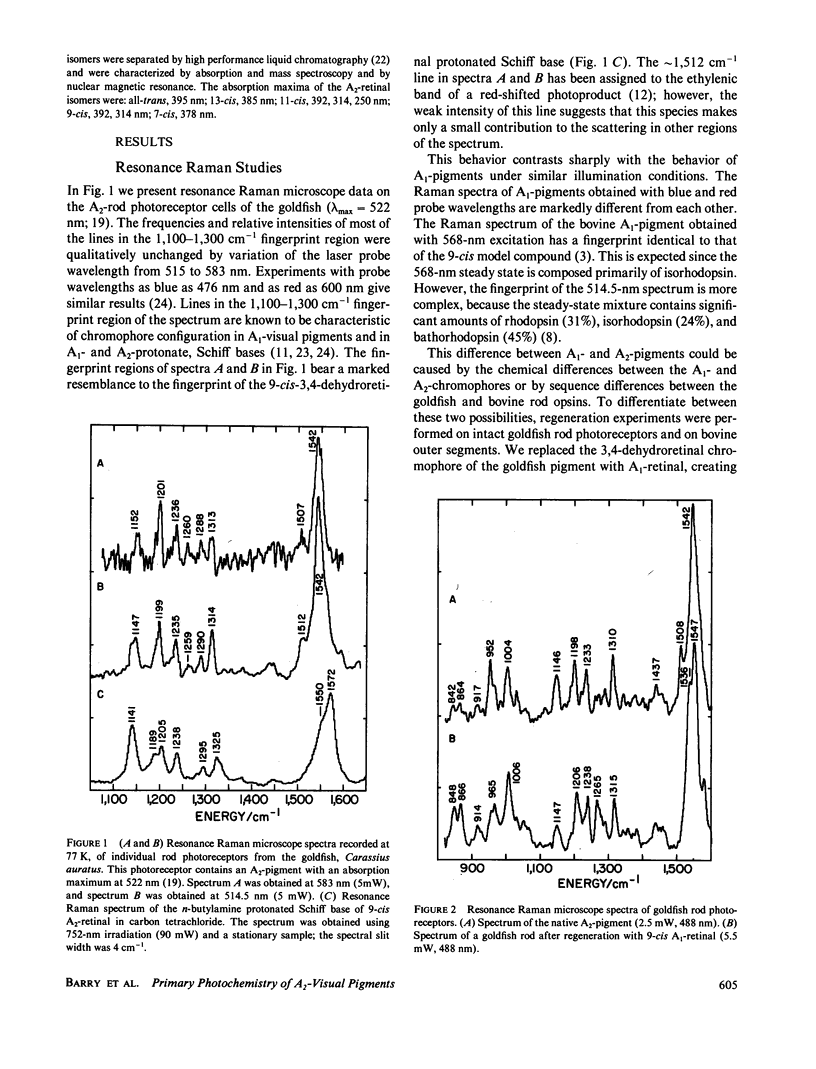
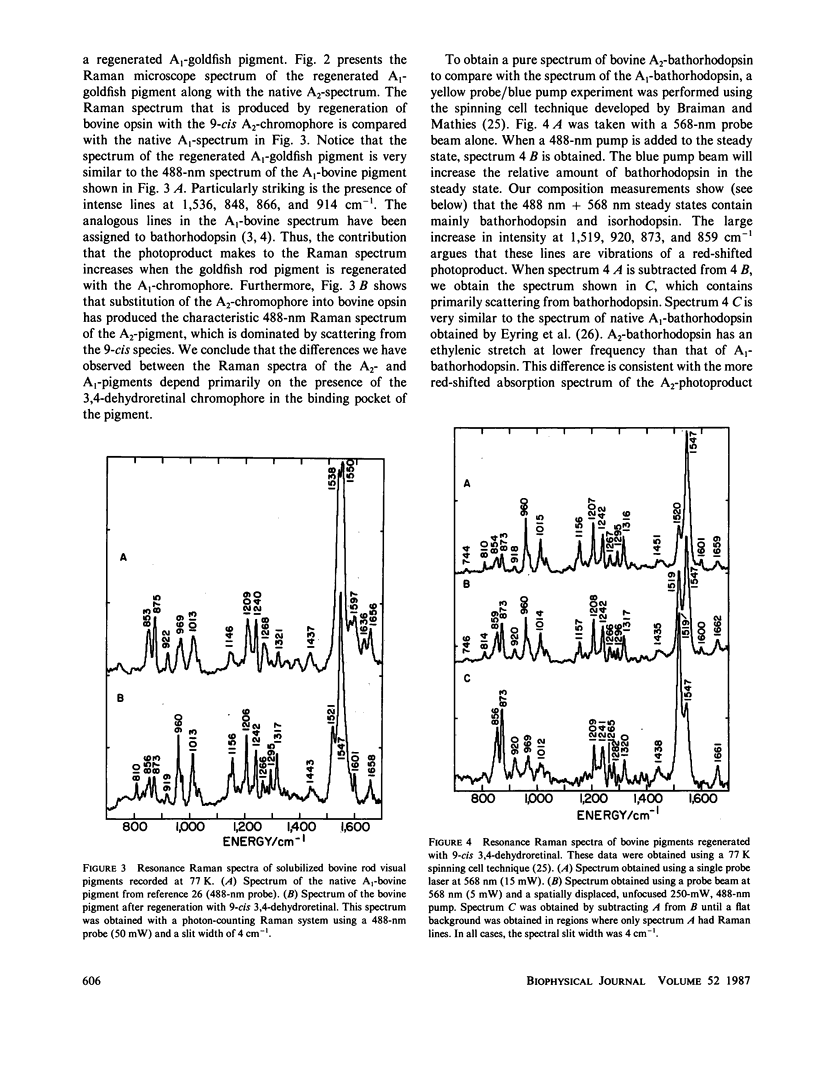
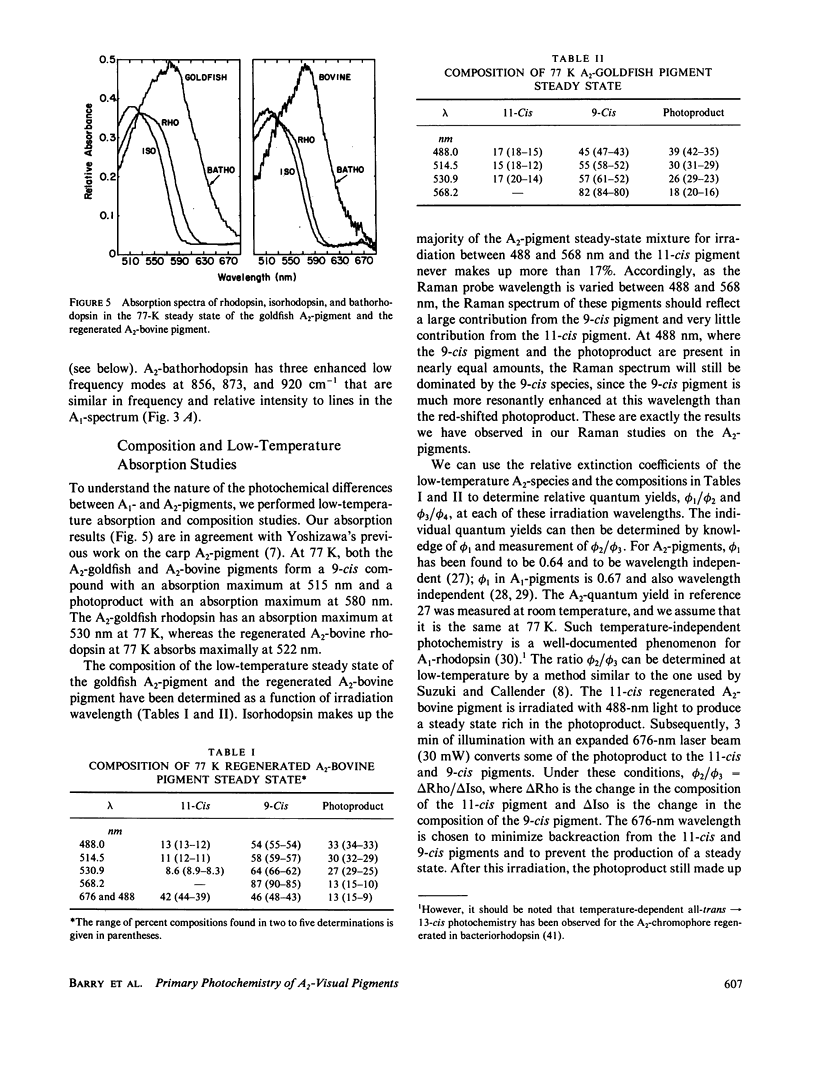
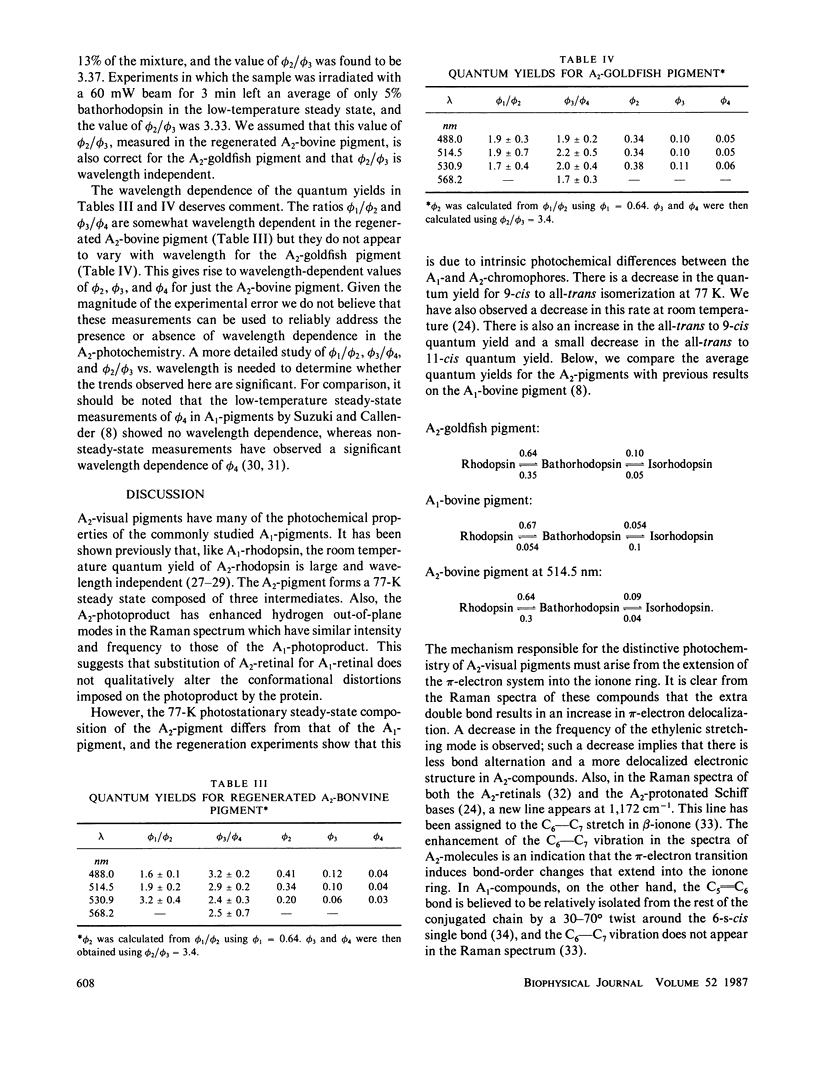
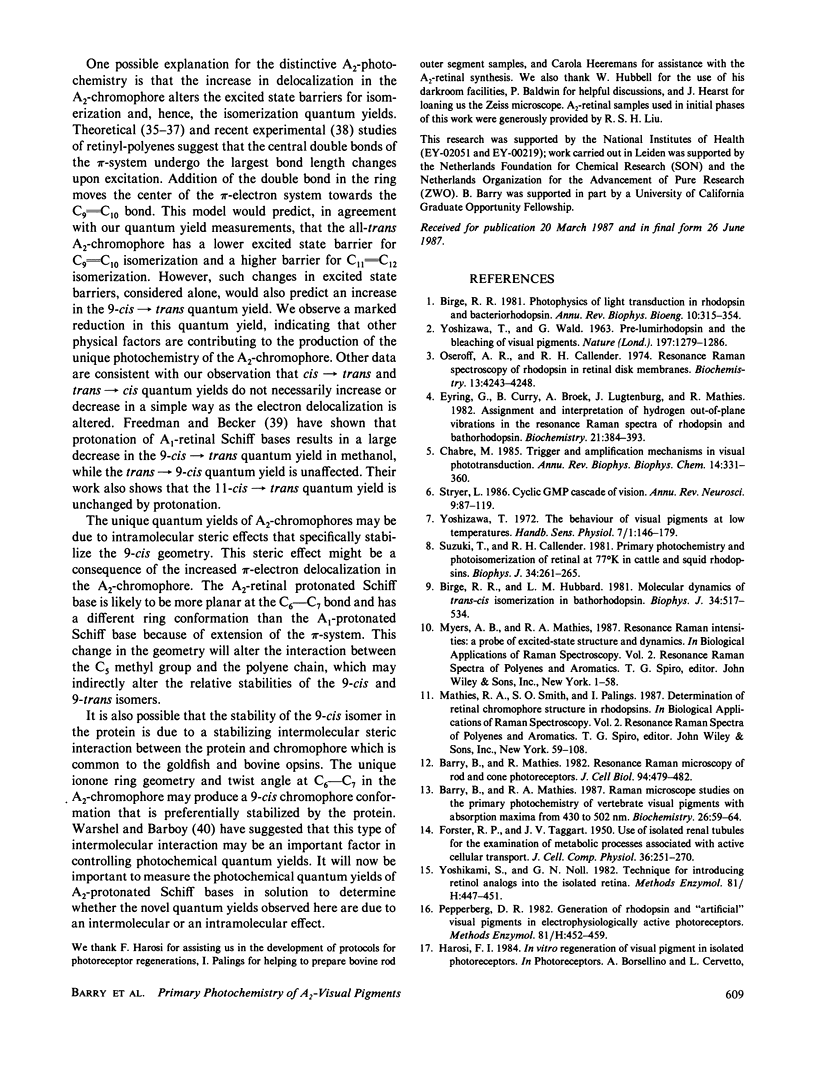
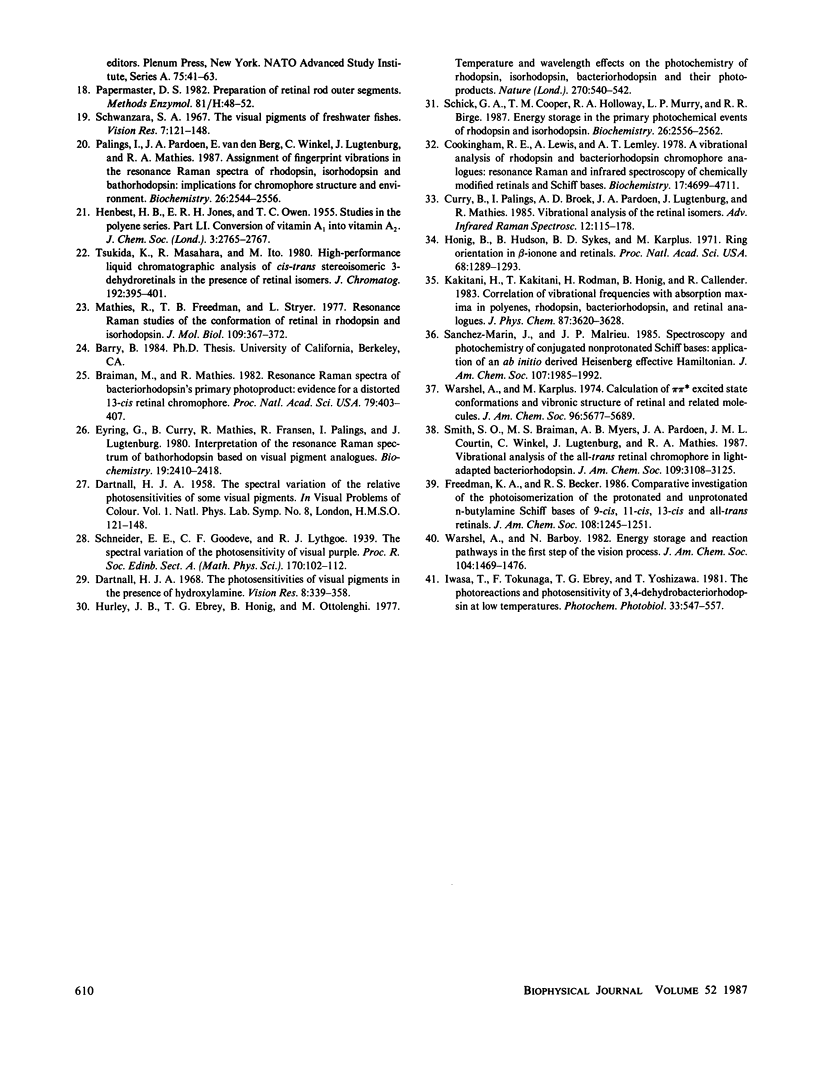
Selected References
These references are in PubMed. This may not be the complete list of references from this article.
- Barry B., Mathies R. A. Raman microscope studies on the primary photochemistry of vertebrate visual pigments with absorption maxima from 430 to 502 nm. Biochemistry. 1987 Jan 13;26(1):59–64. doi: 10.1021/bi00375a009. [DOI] [PubMed] [Google Scholar]
- Barry B., Mathies R. Resonance Raman microscopy of rod and cone photoreceptors. J Cell Biol. 1982 Aug;94(2):479–482. doi: 10.1083/jcb.94.2.479. [DOI] [PMC free article] [PubMed] [Google Scholar]
- Birge R. R., Hubbard L. M. Molecular dynamics of trans-cis isomerization in bathorhodopsin. Biophys J. 1981 Jun;34(3):517–534. doi: 10.1016/S0006-3495(81)84865-5. [DOI] [PMC free article] [PubMed] [Google Scholar]
- Birge R. R. Photophysics of light transduction in rhodopsin and bacteriorhodopsin. Annu Rev Biophys Bioeng. 1981;10:315–354. doi: 10.1146/annurev.bb.10.060181.001531. [DOI] [PubMed] [Google Scholar]
- Braiman M., Mathies R. Resonance Raman spectra of bacteriorhodopsin's primary photoproduct: evidence for a distorted 13-cis retinal chromophore. Proc Natl Acad Sci U S A. 1982 Jan;79(2):403–407. doi: 10.1073/pnas.79.2.403. [DOI] [PMC free article] [PubMed] [Google Scholar]
- Chabre M. Trigger and amplification mechanisms in visual phototransduction. Annu Rev Biophys Biophys Chem. 1985;14:331–360. doi: 10.1146/annurev.bb.14.060185.001555. [DOI] [PubMed] [Google Scholar]
- Cookingham R. E., Lewis A., Lemley A. T. A vibrational analysis of rhodopsin and bacteriorhodopsin chromophore analogues: resonance Raman and infrared spectroscopy of chemically modified retinals and Schiff bases. Biochemistry. 1978 Oct 31;17(22):4699–4711. doi: 10.1021/bi00615a017. [DOI] [PubMed] [Google Scholar]
- Dartnall H. J. The photosensitivities of visual pigments in the presence of hydroxylamine. Vision Res. 1968 Apr;8(4):339–358. doi: 10.1016/0042-6989(68)90104-1. [DOI] [PubMed] [Google Scholar]
- Eyring G., Curry B., Broek A., Lugtenburg J., Mathies R. Assignment and interpretation of hydrogen out-of-plane vibrations in the resonance Raman spectra of rhodopsin and bathorhodopsin. Biochemistry. 1982 Jan 19;21(2):384–393. doi: 10.1021/bi00531a028. [DOI] [PubMed] [Google Scholar]
- Eyring G., Curry B., Mathies R., Fransen R., Palings I., Lugtenburg J. Interpretation of the resonance Raman spectrum of bathorhodopsin based on visual pigment analogues. Biochemistry. 1980 May 27;19(11):2410–2418. doi: 10.1021/bi00552a020. [DOI] [PubMed] [Google Scholar]
- FORSTER R. P., TAGGART J. V. Use of isolated renal tubules for the examination of metabolic processes associated with active cellular transport. J Cell Physiol. 1950 Oct;36(2):251–270. doi: 10.1002/jcp.1030360210. [DOI] [PubMed] [Google Scholar]
- Honig B., Hudson B., Sykes B. D., Karplus M. Ring orientation in -ionone and retinals. Proc Natl Acad Sci U S A. 1971 Jun;68(6):1289–1293. doi: 10.1073/pnas.68.6.1289. [DOI] [PMC free article] [PubMed] [Google Scholar]
- Hurley J. B., Ebrey T. G., Honig B., Ottolenghi M. Temperature and wavelength effects on the photochemistry of rhodopsin, isorhodopsin, bacteriorhodopsin and their photoproducts. Nature. 1977 Dec 8;270(5637):540–542. doi: 10.1038/270540a0. [DOI] [PubMed] [Google Scholar]
- Mathies R., Freedman T. B., Stryer L. Resonance Raman studies of the conformation of retinal in rhodopsin and isorhodopsin. J Mol Biol. 1977 Jan 15;109(2):367–372. doi: 10.1016/s0022-2836(77)80040-5. [DOI] [PubMed] [Google Scholar]
- Oseroff A. R., Callender R. H. Resonance Raman spectroscopy of rhodopsin in retinal disk membranes. Biochemistry. 1974 Sep 24;13(20):4243–4248. doi: 10.1021/bi00717a027. [DOI] [PubMed] [Google Scholar]
- Palings I., Pardoen J. A., van den Berg E., Winkel C., Lugtenburg J., Mathies R. A. Assignment of fingerprint vibrations in the resonance Raman spectra of rhodopsin, isorhodopsin, and bathorhodopsin: implications for chromophore structure and environment. Biochemistry. 1987 May 5;26(9):2544–2556. doi: 10.1021/bi00383a021. [DOI] [PubMed] [Google Scholar]
- Papermaster D. S. Preparation of retinal rod outer segments. Methods Enzymol. 1982;81:48–52. doi: 10.1016/s0076-6879(82)81010-0. [DOI] [PubMed] [Google Scholar]
- Pepperberg D. R. Generation of rhodopsin and "artificial" visual pigments in electrophysiologically active photoreceptors. Methods Enzymol. 1982;81:452–459. doi: 10.1016/s0076-6879(82)81063-x. [DOI] [PubMed] [Google Scholar]
- Schick G. A., Cooper T. M., Holloway R. A., Murray L. P., Birge R. R. Energy storage in the primary photochemical events of rhodopsin and isorhodopsin. Biochemistry. 1987 May 5;26(9):2556–2562. doi: 10.1021/bi00383a022. [DOI] [PubMed] [Google Scholar]
- Schwanzara S. A. The visual pigments of freshwater fishes. Vision Res. 1967 Mar;7(3):121–148. doi: 10.1016/0042-6989(67)90079-x. [DOI] [PubMed] [Google Scholar]
- Stryer L. Cyclic GMP cascade of vision. Annu Rev Neurosci. 1986;9:87–119. doi: 10.1146/annurev.ne.09.030186.000511. [DOI] [PubMed] [Google Scholar]
- Suzuki T., Callender R. H. Primary photochemistry and photoisomerization of retinal at 77 degrees K in cattle and squid rhodopsins. Biophys J. 1981 May;34(2):261–270. doi: 10.1016/S0006-3495(81)84848-5. [DOI] [PMC free article] [PubMed] [Google Scholar]
- Warshel A., Karplus M. Calculation of pi-pi excited state conformations and vibronic structure of retinal and related molecules. J Am Chem Soc. 1974 Sep 4;96(18):5677–5689. doi: 10.1021/ja00825a001. [DOI] [PubMed] [Google Scholar]
- YOSHIZAWA T., WALD G. Pre-lumirhodopsin and the bleaching of visual pigments. Nature. 1963 Mar 30;197:1279–1286. doi: 10.1038/1971279a0. [DOI] [PubMed] [Google Scholar]
- Yoshikami S., Nöll G. N. Technique for introducing retinol analogs into the isolated retina. Methods Enzymol. 1982;81:447–451. doi: 10.1016/s0076-6879(82)81062-8. [DOI] [PubMed] [Google Scholar]