Abstract
The Ca2+-induced loss of deformability in human erythrocytes and the recovery of the lost deformability by stomatocytogenic reagents were investigated by means of a new flow electron paramagnetic resonance (EPR) spin label method, which provides information on deformation and orientation characteristics of spin labeled erythrocytes in shear flow. The Ca2+-induced loss of deformability is attributed mainly to the increase in intracellular viscosity resulting from efflux of intracellular potassium ions and water (Gardos effect). Partial recovery of the lost deformability is demonstrated in the presence of stomatocytogenic reagents, such as chlorpromazine, trifluoperazine, W-7, and calmidazolium (R24571). The recovery can not be explained solely by suppression of the Gardos effect due to the reagents. Incorporation of an optimal amount of the reagents into the membrane appears to compensate for the membrane modification due to Ca2+ ions to restore a part of the lost deformability.
Full text
PDF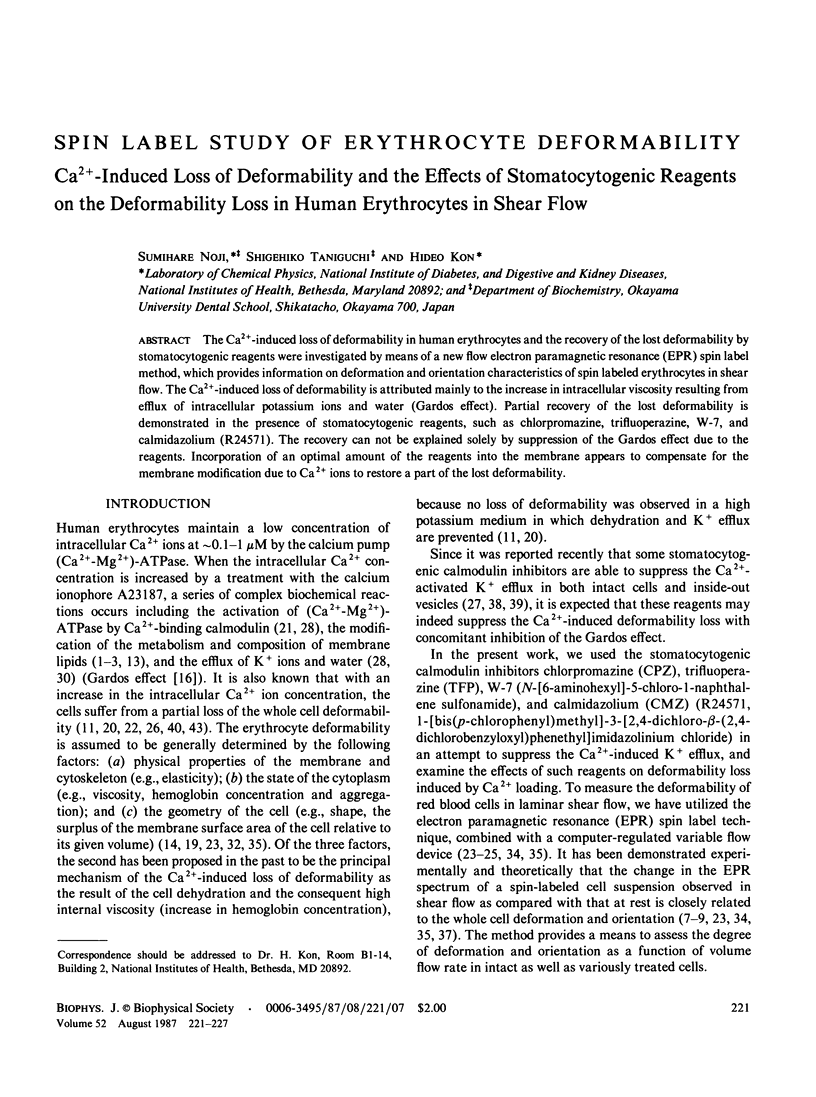
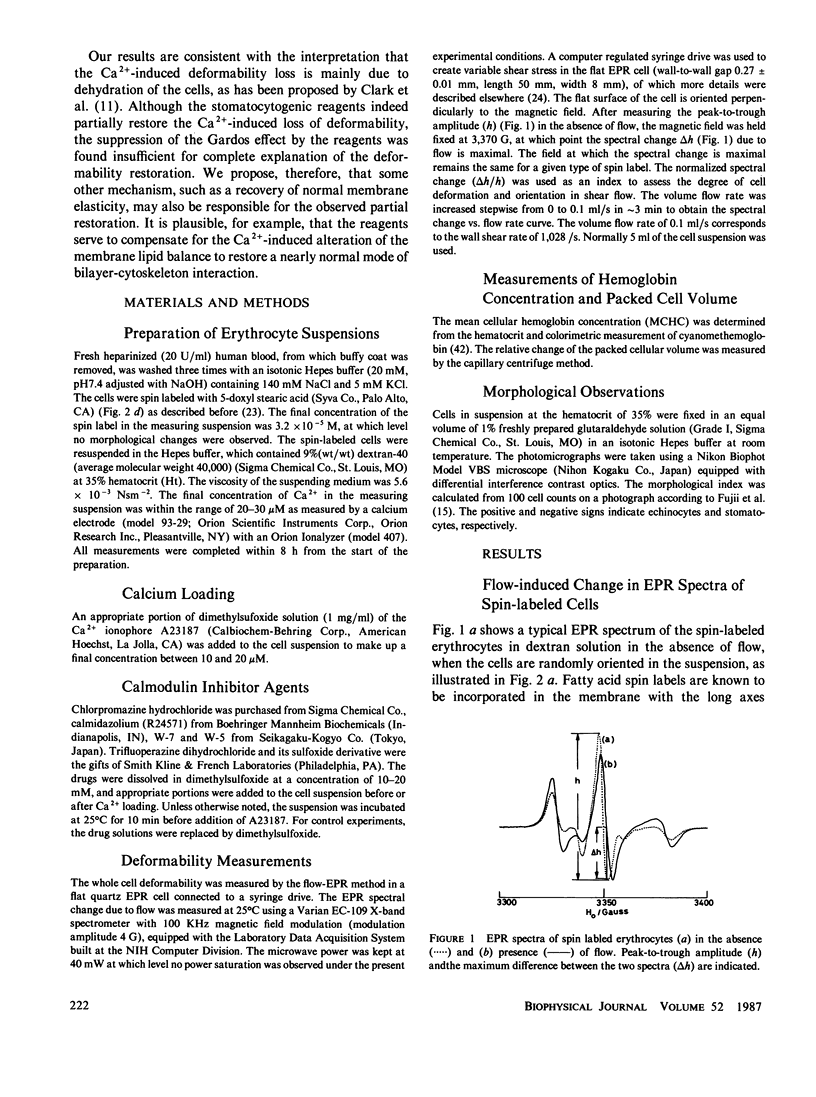
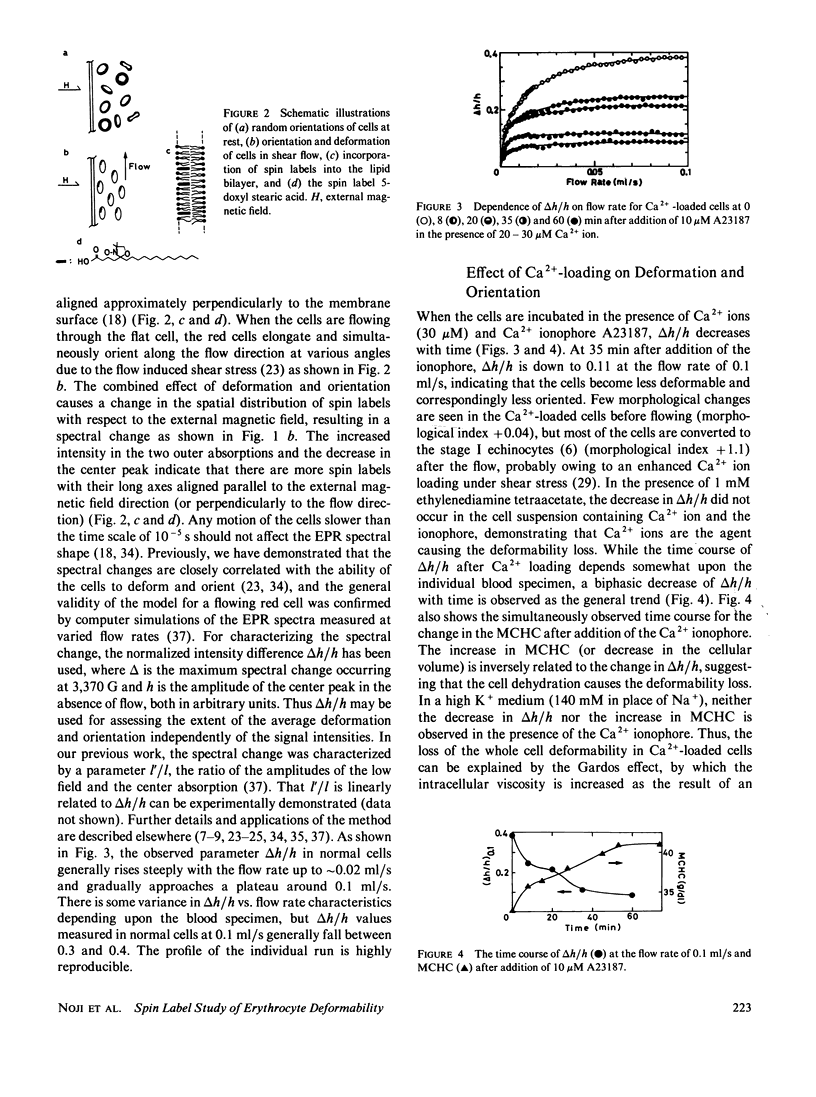
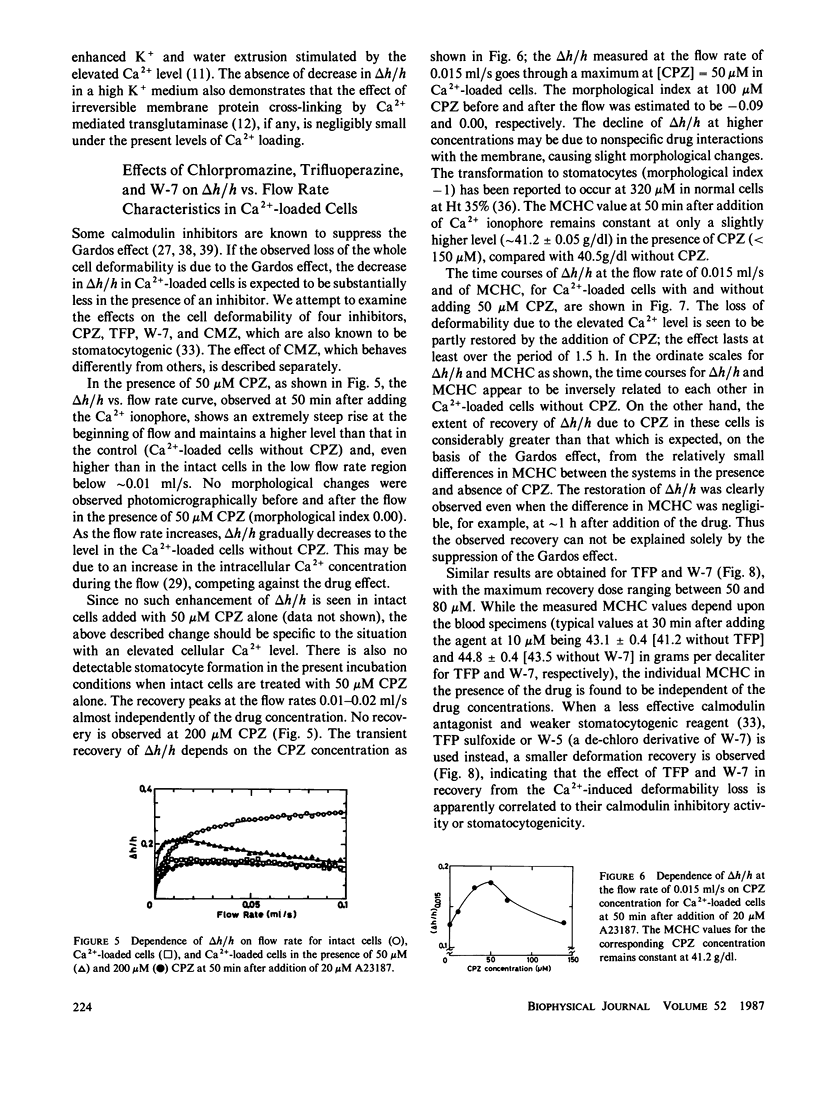
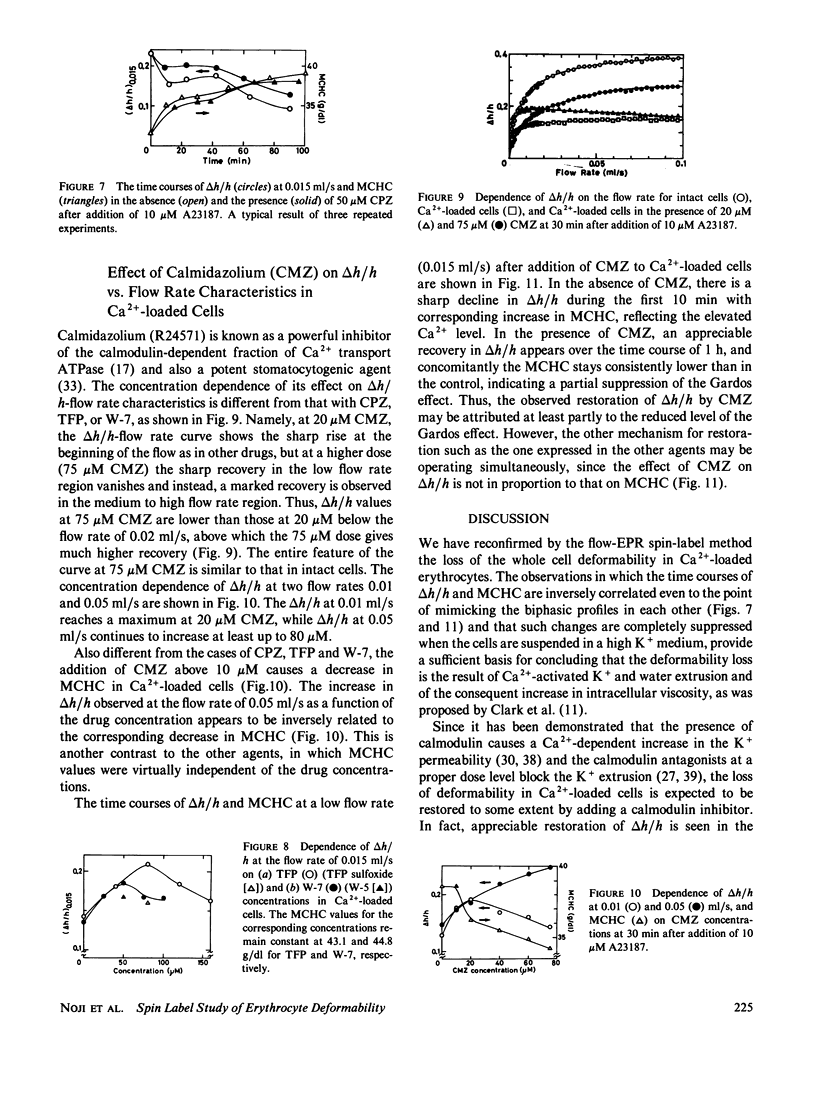
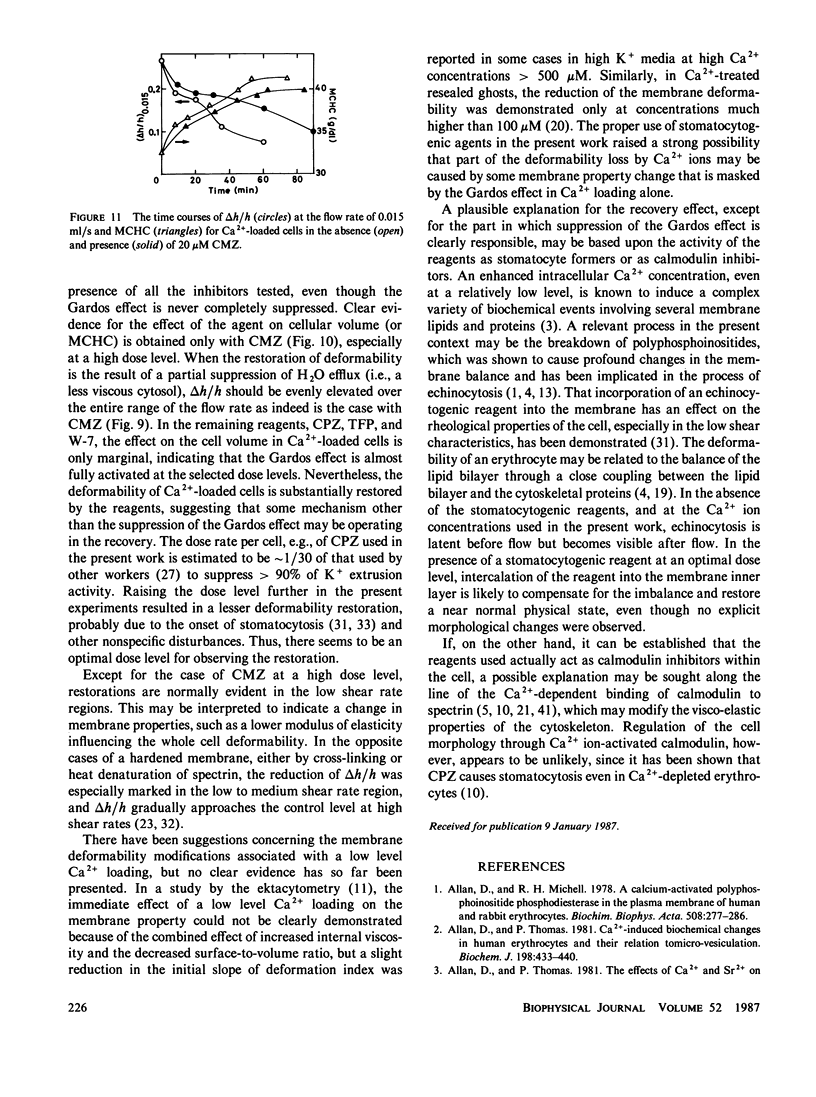
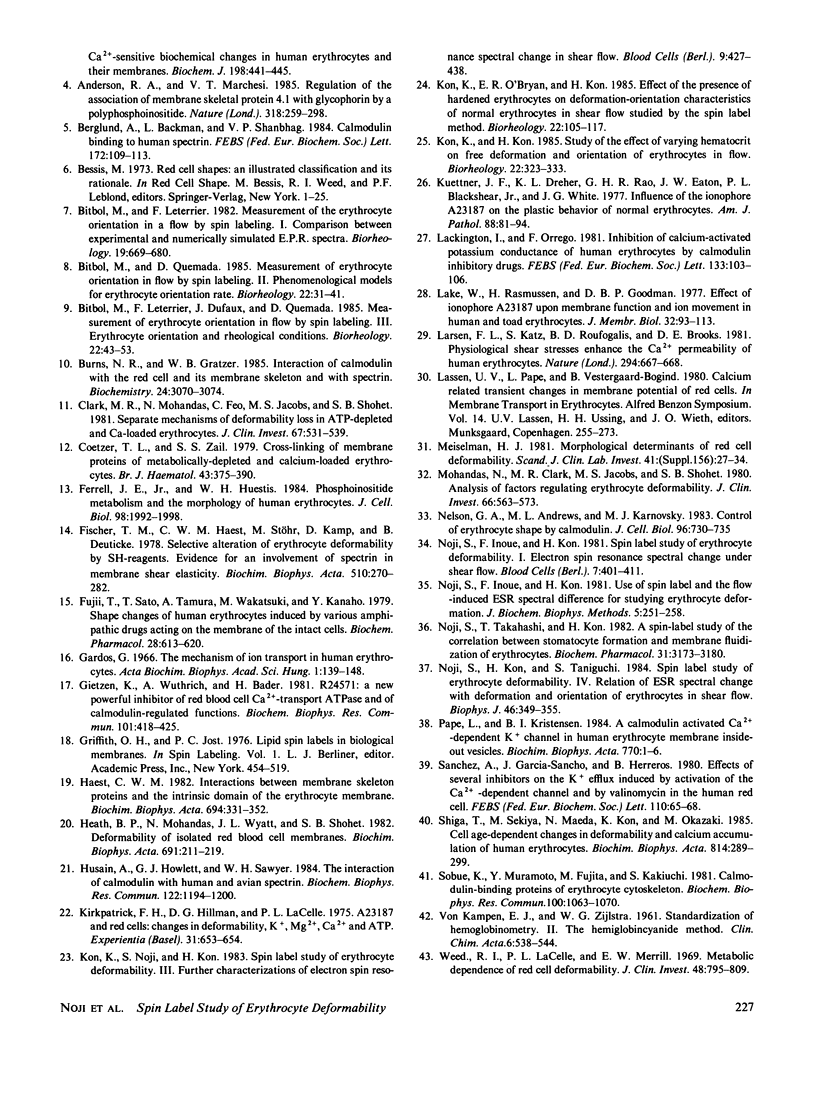
Selected References
These references are in PubMed. This may not be the complete list of references from this article.
- Allan D., Michell R. H. A calcium-activated polyphosphoinositide phosphodiesterase in the plasma membrane of human and rabbit erythrocytes. Biochim Biophys Acta. 1978 Apr 4;508(2):277–286. doi: 10.1016/0005-2736(78)90330-9. [DOI] [PubMed] [Google Scholar]
- Allan D., Thomas P. Ca2+-induced biochemical changes in human erythrocytes and their relation to microvesiculation. Biochem J. 1981 Sep 15;198(3):433–440. doi: 10.1042/bj1980433. [DOI] [PMC free article] [PubMed] [Google Scholar]
- Allan D., Thomas P. The effects of Ca2+ and Sr2+ on Ca2+-sensitive biochemical changes in human erythrocytes and their membranes. Biochem J. 1981 Sep 15;198(3):441–445. doi: 10.1042/bj1980441. [DOI] [PMC free article] [PubMed] [Google Scholar]
- Anderson R. A., Marchesi V. T. Regulation of the association of membrane skeletal protein 4.1 with glycophorin by a polyphosphoinositide. Nature. 1985 Nov 21;318(6043):295–298. doi: 10.1038/318295a0. [DOI] [PubMed] [Google Scholar]
- Berglund A., Backman L., Shanbhag V. P. Calmodulin binding to human spectrin. FEBS Lett. 1984 Jun 25;172(1):109–113. doi: 10.1016/0014-5793(84)80884-4. [DOI] [PubMed] [Google Scholar]
- Bitbol M., Leterrier F., Dufaux J., Quemada D. Measurement of erythrocyte orientation in flow by spin labeling III--erythrocyte orientation and rheological conditions. Biorheology. 1985;22(1):43–53. doi: 10.3233/bir-1985-22104. [DOI] [PubMed] [Google Scholar]
- Bitbol M., Leterrier F. Measurement of the erythrocyte orientation in a flow by spin labeling. Biorheology. 1982;19(6):669–680. doi: 10.3233/bir-1982-19601. [DOI] [PubMed] [Google Scholar]
- Bitbol M., Quemada D. Measurement of erythrocyte orientation in flow by spin labeling II--phenomenological models for erythrocyte orientation rate. Biorheology. 1985;22(1):31–42. doi: 10.3233/bir-1985-22103. [DOI] [PubMed] [Google Scholar]
- Burns N. R., Gratzer W. B. Interaction of calmodulin with the red cell and its membrane skeleton and with spectrin. Biochemistry. 1985 Jun 4;24(12):3070–3074. doi: 10.1021/bi00333a040. [DOI] [PubMed] [Google Scholar]
- Clark M. R., Mohandas N., Feo C., Jacobs M. S., Shohet S. B. Separate mechanisms of deformability loss in ATP-depleted and Ca-loaded erythrocytes. J Clin Invest. 1981 Feb;67(2):531–539. doi: 10.1172/JCI110063. [DOI] [PMC free article] [PubMed] [Google Scholar]
- Coetzer T. L., Zail S. S. Cross-linking of membrane proteins of metabolically-depleted and calcium-loaded erythrocytes. Br J Haematol. 1979 Nov;43(3):375–390. doi: 10.1111/j.1365-2141.1979.tb03765.x. [DOI] [PubMed] [Google Scholar]
- Ferrell J. E., Jr, Huestis W. H. Phosphoinositide metabolism and the morphology of human erythrocytes. J Cell Biol. 1984 Jun;98(6):1992–1998. doi: 10.1083/jcb.98.6.1992. [DOI] [PMC free article] [PubMed] [Google Scholar]
- Fischer T. M., Haest C. W., Stöhr M., Kamp D., Deuticke B. Selective alteration of erythrocyte deformabiliby by SH-reagents: evidence for an involvement of spectrin in membrane shear elasticity. Biochim Biophys Acta. 1978 Jul 4;510(2):270–282. doi: 10.1016/0005-2736(78)90027-5. [DOI] [PubMed] [Google Scholar]
- Fujii T., Sato T., Tamura A., Wakatsuki M., Kanaho Y. Shape changes of human erythrocytes induced by various amphipathic drugs acting on the membrane of the intact cells. Biochem Pharmacol. 1979 Mar 1;28(5):613–620. doi: 10.1016/0006-2952(79)90144-8. [DOI] [PubMed] [Google Scholar]
- Gietzen K., Wüthrich A., Bader H. R 24571: a new powerful inhibitor of red blood cell Ca++-transport ATPase and of calmodulin-regulated functions. Biochem Biophys Res Commun. 1981 Jul 30;101(2):418–425. doi: 10.1016/0006-291x(81)91276-6. [DOI] [PubMed] [Google Scholar]
- Haest C. W. Interactions between membrane skeleton proteins and the intrinsic domain of the erythrocyte membrane. Biochim Biophys Acta. 1982 Dec;694(4):331–352. doi: 10.1016/0304-4157(82)90001-6. [DOI] [PubMed] [Google Scholar]
- Heath B. P., Mohandas N., Wyatt J. L., Shohet S. B. Deformability of isolated red blood cell membranes. Biochim Biophys Acta. 1982 Oct 7;691(2):211–219. doi: 10.1016/0005-2736(82)90409-6. [DOI] [PubMed] [Google Scholar]
- Husain A., Howlett G. J., Sawyer W. H. The interaction of calmodulin with human and avian spectrin. Biochem Biophys Res Commun. 1984 Aug 16;122(3):1194–1200. doi: 10.1016/0006-291x(84)91218-x. [DOI] [PubMed] [Google Scholar]
- Kirkpatrick F. H., Hillman D. G., La Celle P. L. A23187 and red cells: changes in deformability, K+, Mg-2+, Ca-2+ and ATP. Experientia. 1975 Jun 15;31(6):653–654. doi: 10.1007/BF01944610. [DOI] [PubMed] [Google Scholar]
- Kon K., Kon H. Study of the effect of varying hematocrit on free deformation and orientation of erythrocytes in flow. Biorheology. 1985;22(4):323–333. doi: 10.3233/bir-1985-22405. [DOI] [PubMed] [Google Scholar]
- Kon K., Noji S., Kon H. Spin label study of erythrocyte deformability. III. Further characterizations of electron spin resonance spectral change in shear flow. Blood Cells. 1983;9(3):427–441. [PubMed] [Google Scholar]
- Kon K., O'Bryan E. R., Kon H. Effect of the presence of hardened erythrocytes on deformation-orientation characteristics of normal erythrocytes in shear flow studied by the spin label method. Biorheology. 1985;22(2):105–117. doi: 10.3233/bir-1985-22202. [DOI] [PubMed] [Google Scholar]
- Kuettner J. F., Dreher K. L., Rao G. H., Eaton J. W., Blackshear P. L., Jr, White J. G. Influence of the ionophore A23187 on the plastic behavior of normal erythrocytes. Am J Pathol. 1977 Jul;88(1):81–94. [PMC free article] [PubMed] [Google Scholar]
- Lackington I., Orrego F. Inhibition of calcium-activated potassium conductance of human erythrocytes by calmodulin inhibitory drugs. FEBS Lett. 1981 Oct 12;133(1):103–106. doi: 10.1016/0014-5793(81)80481-4. [DOI] [PubMed] [Google Scholar]
- Lake W., Rasmussen H., Goodman D. B. Effect of ionophore A23187 upon membrane function and ion movement in human and toad erythrocytes. J Membr Biol. 1977 Apr 7;32(1-2):93–113. doi: 10.1007/BF01905211. [DOI] [PubMed] [Google Scholar]
- Larsen F. L., Katz S., Roufogalis B. D., Brooks D. E. Physiological shear stresses enhance the Ca2+ permeability of human erythrocytes. Nature. 1981 Dec 17;294(5842):667–668. doi: 10.1038/294667a0. [DOI] [PubMed] [Google Scholar]
- Meiselman H. J. Morphological determinants of red cell deformability. Scand J Clin Lab Invest Suppl. 1981;156:27–34. doi: 10.3109/00365518109097426. [DOI] [PubMed] [Google Scholar]
- Mohandas N., Clark M. R., Jacobs M. S., Shohet S. B. Analysis of factors regulating erythrocyte deformability. J Clin Invest. 1980 Sep;66(3):563–573. doi: 10.1172/JCI109888. [DOI] [PMC free article] [PubMed] [Google Scholar]
- Nelson G. A., Andrews M. L., Karnovsky M. J. Control of erythrocyte shape by calmodulin. J Cell Biol. 1983 Mar;96(3):730–735. doi: 10.1083/jcb.96.3.730. [DOI] [PMC free article] [PubMed] [Google Scholar]
- Noji S., Inoue F., Kon H. Spin label study of erythrocyte deformability I. Electron spin resonance spectral change under shear flow. Blood Cells. 1981;7(2):401–415. [PubMed] [Google Scholar]
- Noji S., Inoue F., Kon H. Use of spin label and the flow-induced ESR spectral difference for studying erythrocyte deformation. J Biochem Biophys Methods. 1981 Dec;5(5):251–258. doi: 10.1016/0165-022x(81)90035-x. [DOI] [PubMed] [Google Scholar]
- Noji S., Kon H., Taniguchi S. Spin-label studies of erythrocyte deformability. IV. Relation of electron spin resonance spectral change with deformation and orientation of erythrocytes in shear flow. Biophys J. 1984 Sep;46(3):349–355. doi: 10.1016/S0006-3495(84)84031-X. [DOI] [PMC free article] [PubMed] [Google Scholar]
- Noji S., Takahashi T., Kon H. A spin-label study of the correlation between stomatocyte formation and membrane fluidization of erythrocytes. Biochem Pharmacol. 1982 Oct 15;31(20):3173–3180. doi: 10.1016/0006-2952(82)90546-9. [DOI] [PubMed] [Google Scholar]
- Pape L., Kristensen B. I. A calmodulin activated Ca2+-dependent K+ channel in human erythrocyte membrane inside-out vesicles. Biochim Biophys Acta. 1984 Feb 29;770(1):1–6. doi: 10.1016/0005-2736(84)90065-8. [DOI] [PubMed] [Google Scholar]
- Sanchez A., García-Sancho J., Herreros B. Effects of several inhibitors on the K+ efflux induced by activation of the Ca2+-dependent channel and by valinomycin in the human red cell. FEBS Lett. 1980 Jan 28;110(1):65–68. doi: 10.1016/0014-5793(80)80024-x. [DOI] [PubMed] [Google Scholar]
- Shiga T., Sekiya M., Maeda N., Kon K., Okazaki M. Cell age-dependent changes in deformability and calcium accumulation of human erythrocytes. Biochim Biophys Acta. 1985 Apr 11;814(2):289–299. doi: 10.1016/0005-2736(85)90447-x. [DOI] [PubMed] [Google Scholar]
- Sobue K., Muramoto Y., Fujita M., Kakiuchi S. Calmodulin-binding protein of erythrocyte cytoskeleton. Biochem Biophys Res Commun. 1981 Jun 16;100(3):1063–1070. doi: 10.1016/0006-291x(81)91931-8. [DOI] [PubMed] [Google Scholar]
- Weed R. I., LaCelle P. L., Merrill E. W. Metabolic dependence of red cell deformability. J Clin Invest. 1969 May;48(5):795–809. doi: 10.1172/JCI106038. [DOI] [PMC free article] [PubMed] [Google Scholar]
- van KAMPEN E., ZIJLSTRA W. G. Standardization of hemoglobinometry. II. The hemiglobincyanide method. Clin Chim Acta. 1961 Jul;6:538–544. doi: 10.1016/0009-8981(61)90145-0. [DOI] [PubMed] [Google Scholar]