Abstract
The restricted rotational diffusion of an axially symmetric particle is simulated by the Brownian dynamics technique. In addition to the wobbling-in-a-cone model, several continuous potentials are considered. The particle studied is particularly simple: a sphere anchored to a point fixed in space. However, presenting the results in a convenient, reduced form, they are valid for any axially symmetric particle. From simulated rotational trajectories, we calculate (P2(cos alpha] as a function of t, where alpha is the angle between two orientations separated by time t and P2 is the second Legendre polynomial. This correlation function is closely related to time-resolved electro-optic and spectroscopic properties. Simulated results for the cone model are in excellent agreement with the quasiexact results of Lipari and Szabo (1981, J. Chem. Phys., 75:2971-2976). Thus we confirm the good performance of the simulation technique and the validity of our working conditions. Novel results are presented for continuous restricting potentials, V(theta). The (P2) results for V = 1/2K theta 2 and V = Q(1 - cos theta) are practically the same if K and Q are chosen so tht the long-time (P2) values coincide. Thus, the quadratic potential seems to be a good representation of any monotonically increasing potential. However, for an uniaxial potential such as V = Csin2 theta, the decay is appreciably faster. The (P2) decays simulated for the continuous potentials are analyzed by the monoexponential version of the cone model. We found that such an analysis produces an overestimation of the true rotational diffusion coefficient of approximately 15% only, although for uniaxial potentials the error may be larger.
Full text
PDF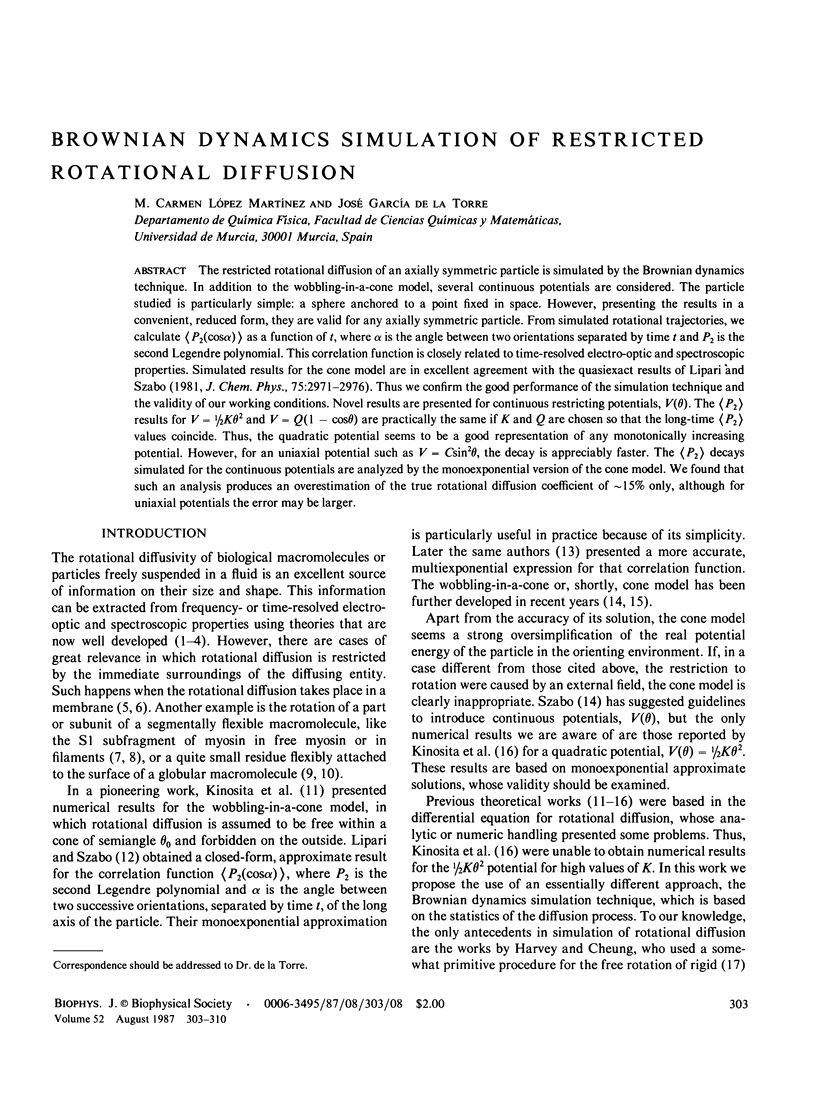
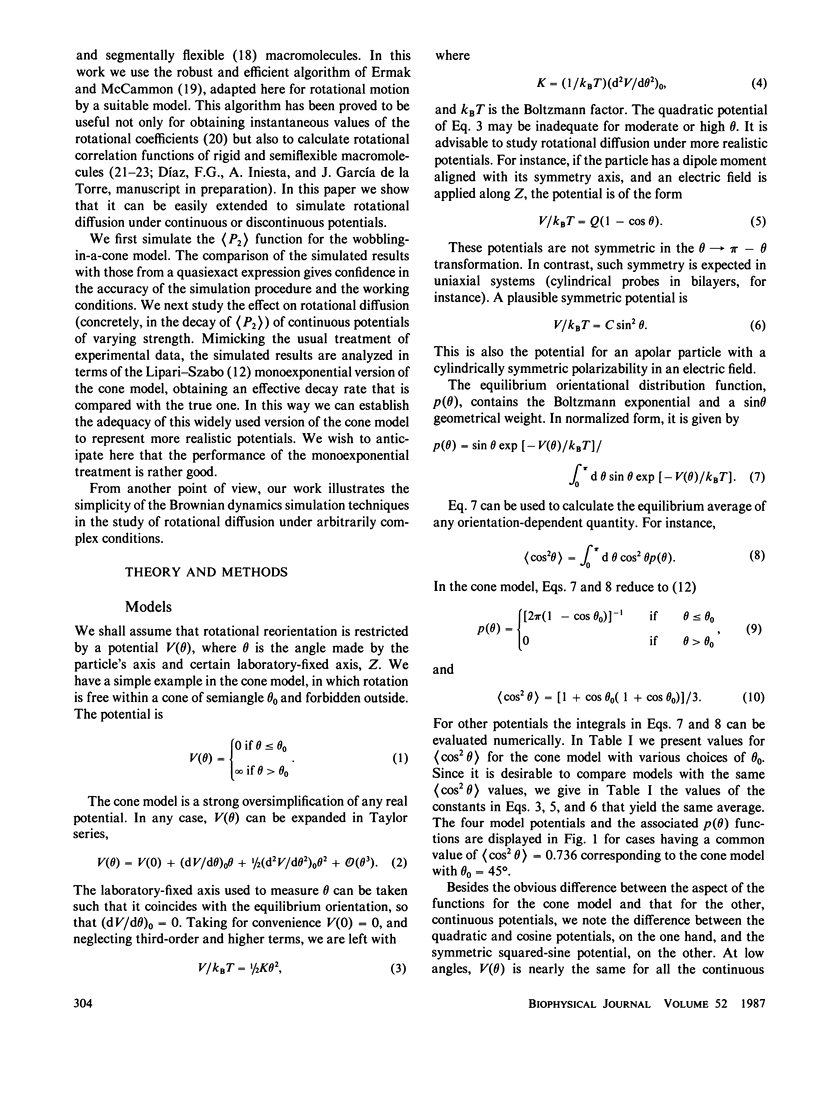
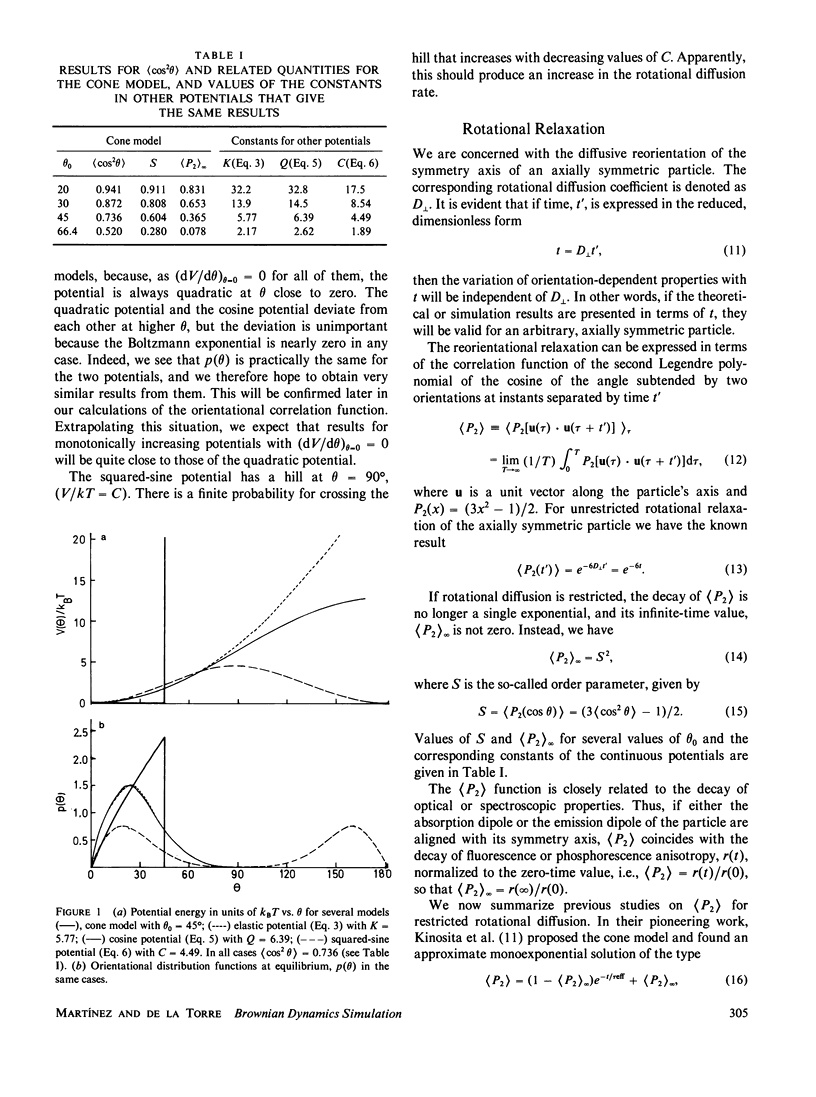
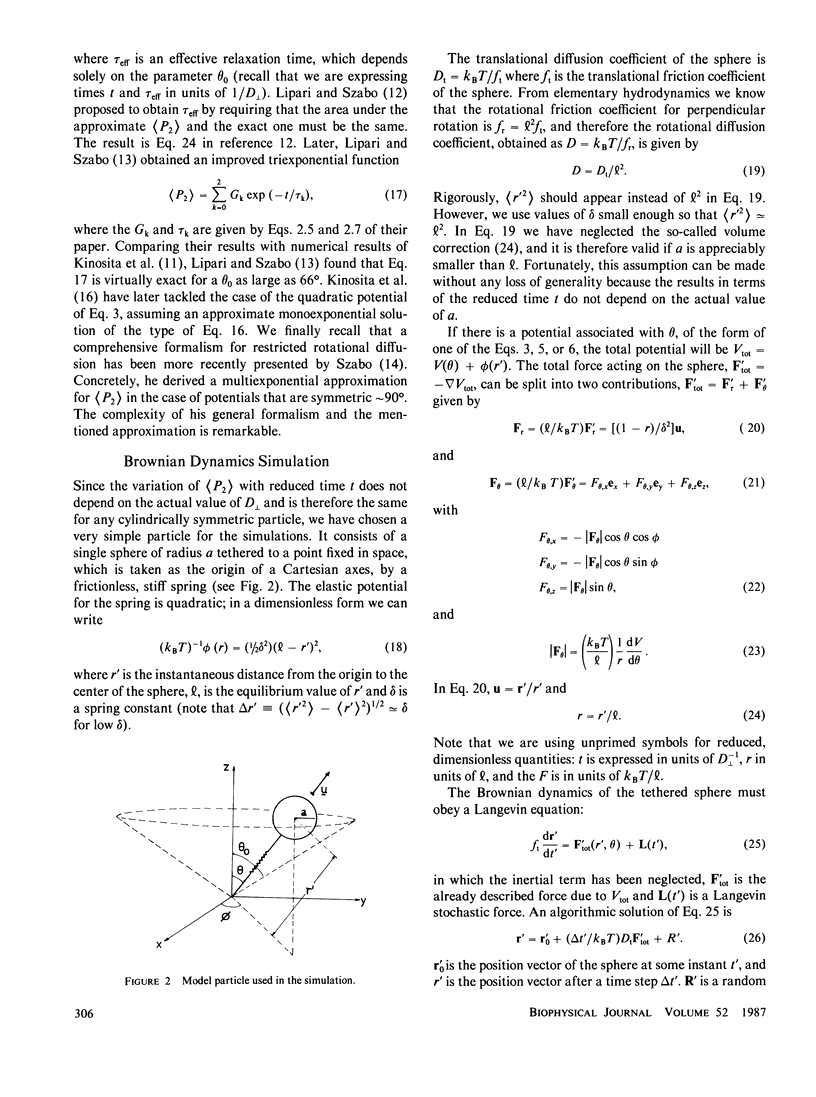
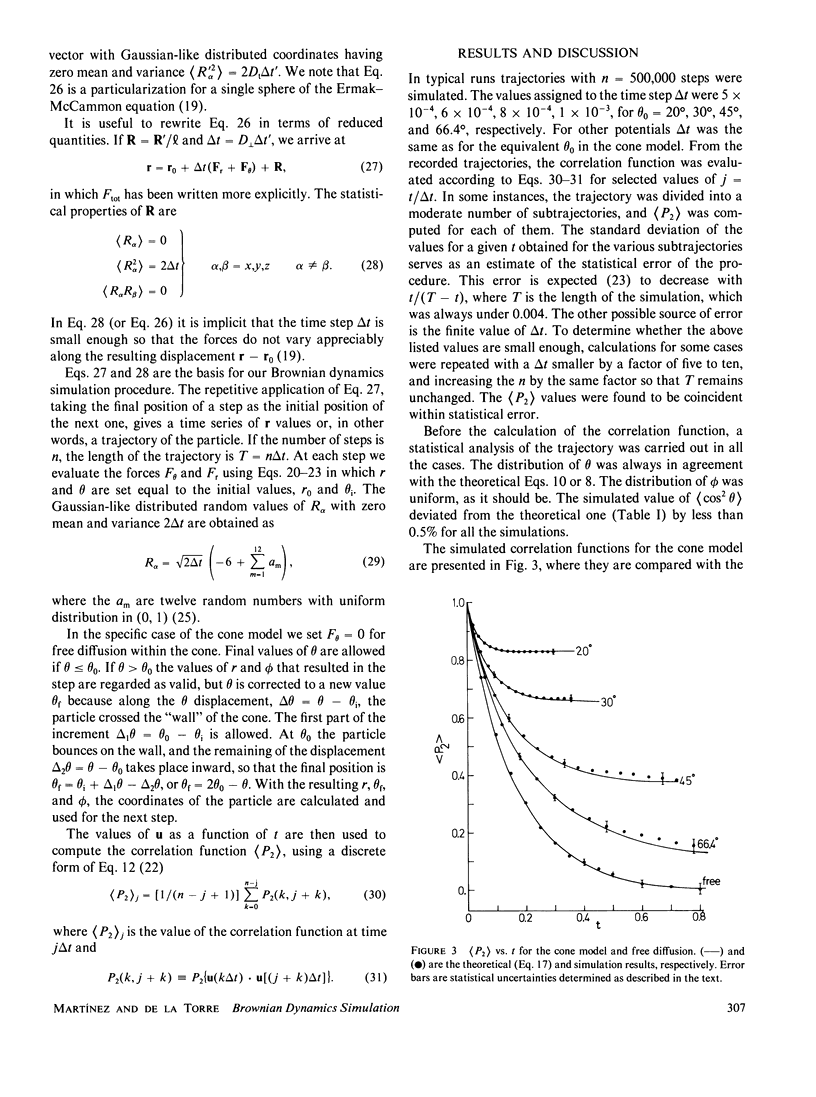
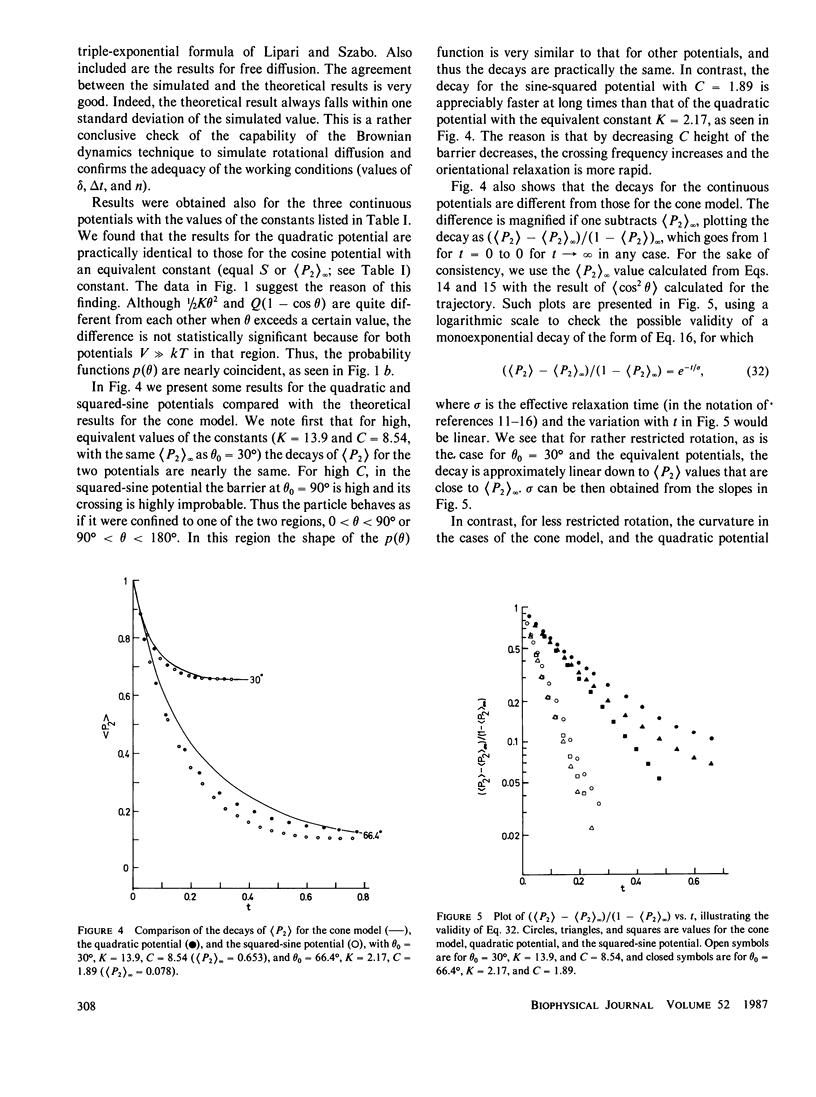
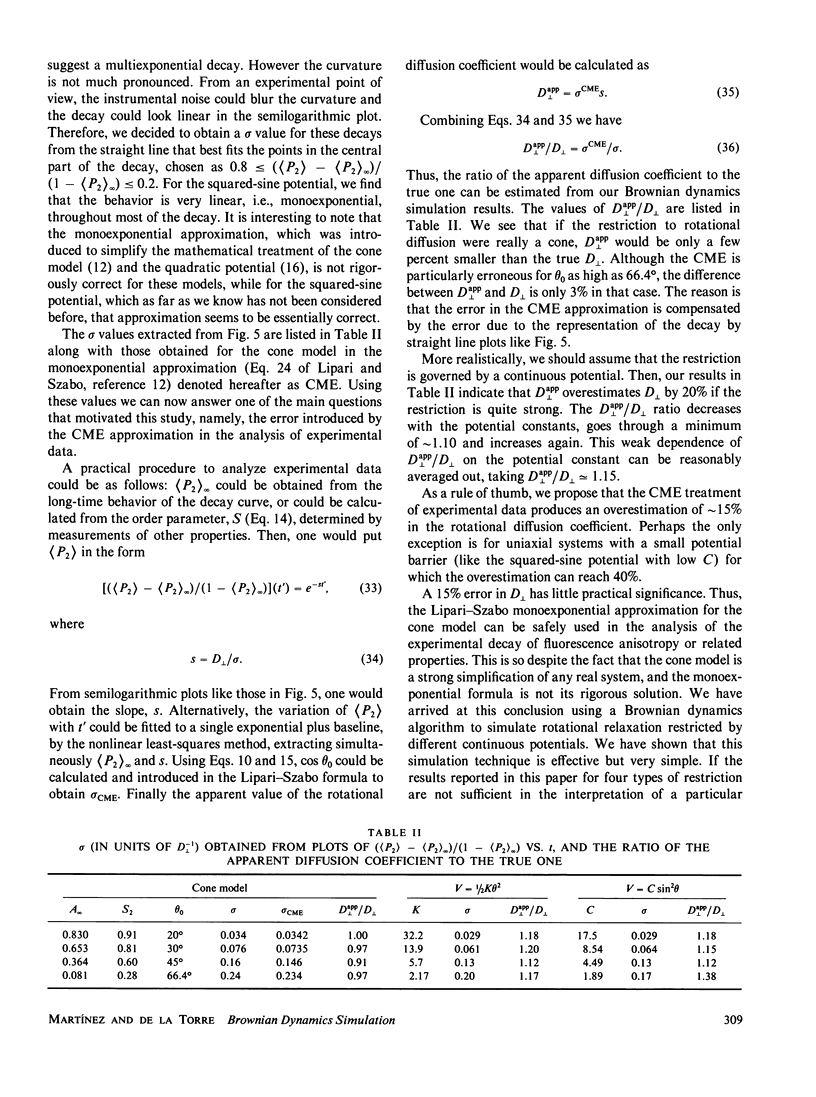
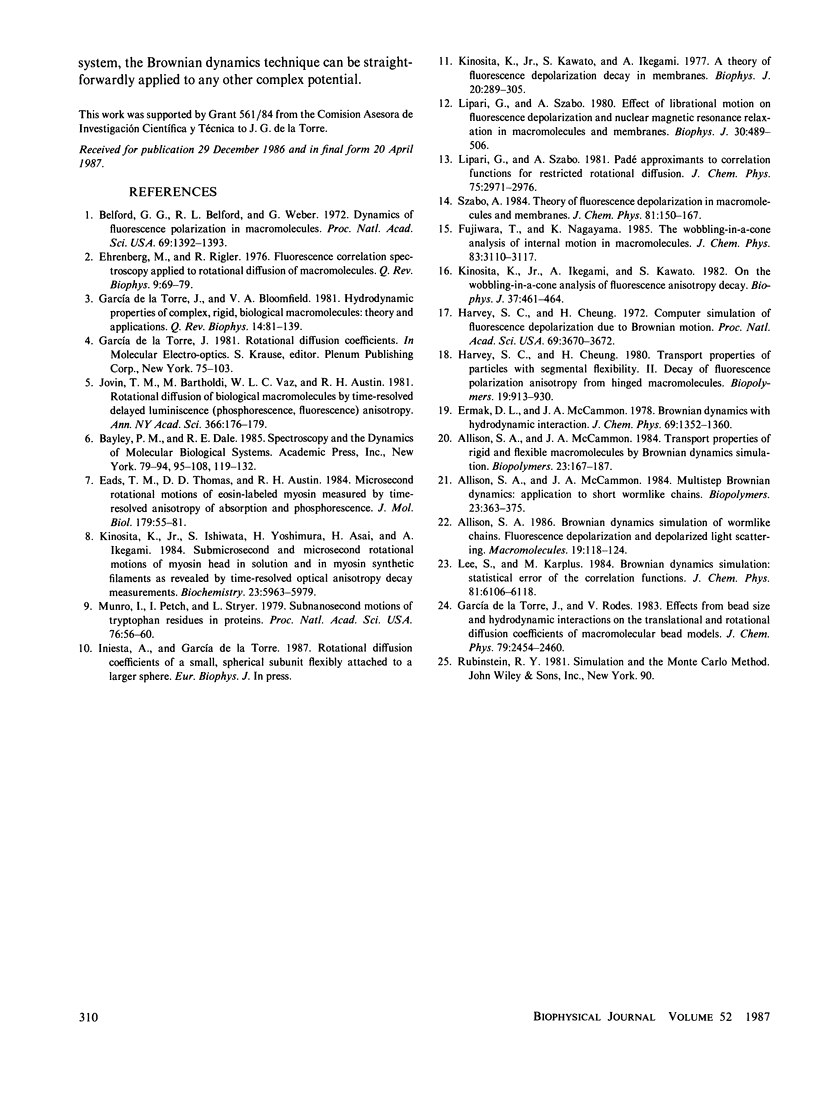
Selected References
These references are in PubMed. This may not be the complete list of references from this article.
- Allison S. A., McCammon J. A. Multistep Brownian dynamics: application to short wormlike chains. Biopolymers. 1984 Feb;23(2):363–375. doi: 10.1002/bip.360230214. [DOI] [PubMed] [Google Scholar]
- Belford G. G., Belford R. L., Weber G. Dynamics of fluorescence polarization in macromolecules. Proc Natl Acad Sci U S A. 1972 Jun;69(6):1392–1393. doi: 10.1073/pnas.69.6.1392. [DOI] [PMC free article] [PubMed] [Google Scholar]
- Eads T. M., Thomas D. D., Austin R. H. Microsecond rotational motions of eosin-labeled myosin measured by time-resolved anisotropy of absorption and phosphorescence. J Mol Biol. 1984 Oct 15;179(1):55–81. doi: 10.1016/0022-2836(84)90306-1. [DOI] [PubMed] [Google Scholar]
- Ehrenberg M., Rigler R. Fluorescence correlation spectroscopy applied to rotational diffusion of macromolecules. Q Rev Biophys. 1976 Feb;9(1):69–81. doi: 10.1017/s003358350000216x. [DOI] [PubMed] [Google Scholar]
- Garcia de la Torre J. G., Bloomfield V. A. Hydrodynamic properties of complex, rigid, biological macromolecules: theory and applications. Q Rev Biophys. 1981 Feb;14(1):81–139. doi: 10.1017/s0033583500002080. [DOI] [PubMed] [Google Scholar]
- Harvey S. C., Cheung H. C. Computer simulation of fluorescence depolarization due to brownian motion. Proc Natl Acad Sci U S A. 1972 Dec;69(12):3670–3672. doi: 10.1073/pnas.69.12.3670. [DOI] [PMC free article] [PubMed] [Google Scholar]
- Jovin T. M., Bartholdi M., Vaz W. L., Austin R. H. Rotational diffusion of biological macromolecules by time-resolved delayed luminescence (phosphorescence, fluorescence) anisotropy. Ann N Y Acad Sci. 1981;366:176–196. doi: 10.1111/j.1749-6632.1981.tb20753.x. [DOI] [PubMed] [Google Scholar]
- Kinosita K., Jr, Ikegami A., Kawato S. On the wobbling-in-cone analysis of fluorescence anisotropy decay. Biophys J. 1982 Feb;37(2):461–464. doi: 10.1016/S0006-3495(82)84692-4. [DOI] [PMC free article] [PubMed] [Google Scholar]
- Kinosita K., Jr, Kawato S., Ikegami A. A theory of fluorescence polarization decay in membranes. Biophys J. 1977 Dec;20(3):289–305. doi: 10.1016/S0006-3495(77)85550-1. [DOI] [PMC free article] [PubMed] [Google Scholar]
- Lipari G., Szabo A. Effect of librational motion on fluorescence depolarization and nuclear magnetic resonance relaxation in macromolecules and membranes. Biophys J. 1980 Jun;30(3):489–506. doi: 10.1016/S0006-3495(80)85109-5. [DOI] [PMC free article] [PubMed] [Google Scholar]
- Munro I., Pecht I., Stryer L. Subnanosecond motions of tryptophan residues in proteins. Proc Natl Acad Sci U S A. 1979 Jan;76(1):56–60. doi: 10.1073/pnas.76.1.56. [DOI] [PMC free article] [PubMed] [Google Scholar]