Abstract
Kimmich and co-workers (cf., Winter, F., and R. Kimmich. 1982. Biochim. Biophys. Acta. 719:292-298) discovered peaks in the magnetic field-dependent longitudinal relaxation rate (1/T1) of water protons of muscle tissue, cells, and dehydrated protein in the field range 0.5-5 MHz (proton Larmor frequency), and argued that the peaks resulted from cross relaxation associated with quadrupolar splittings of the 14N nuclei of protein NH groups. More recently, analogous peaks were found in homogenates of calf eye lens (Beaulieu, C.F., J.I. Clark, R.D. Brown III, M. Spiller, and S. H. Koenig, 1987. Abstr. Soc. Magn. Res. Med., 6th, New York. 598-599), which are essentially concentrated protein solutions, and were measured with sufficient precision to allow resolution of the relaxation spectra into several peaks and the intrinsic linewidths to be determined. Here, we analyze these relaxation spectra, as well as earlier data on rat heart (Koenig, S. H., R. D. Brown III, D. Adams, D. Emerson, and C. G. Harrison. 1984. Invest. Radiol. 19:76-81) in some detail, and suggest a specific pathway for the cross relaxation to which we apply the theory of relaxation quantitatively. The view that emerges is that, at fields such that the proton Zeeman energy of the NH protons matches an 14N quadrupolar splitting, relaxation of these protons is by cross relaxation to the 14N nuclei which in turn transfer excess energy to the protein. The correlation time for the NH proton interaction is the T2 of the 14N nuclei, approximately 10(-6) s, whereas T1 of the NH protons is approximately 1.25 ms. At these energy level crossings, the NH protons become relaxation sinks for protons of rapidly exchanging (-3 x 109 s-1) water molecules hydrogen bonded to the same backbone carbonyl oxygens as the NH protons. The lifetime of this hydrogen bond (-3 x 10-10 s) then becomes the correlation time for the water proton-NH proton interaction which, though short, is much longer than the analogous correlation time (-5 x 10-12 s) in pure water; the enhanced interaction results in peaks in the field-dependent 1/ T, of the solvent protons. There are few data on the lifetime of such bonds, but the results here conform with the recent considerations of Bennett, H. F., R. D. Brown III, S. H. Koenig, and H. M. Swartz. 1987. Magn. Reson. Med. 4:93-111, regarding hydrogen bond lifetimes for water molecules bound to macromolecules. The recent precise field-dependent relaxation data, here combined with both a quantitative theory and the fact that the magnitude of the 14N peaks is very concentration sensitive, allow, at least for lens proteins, a study of protein-protein interactions difficult to investigate by other methods.
Full text
PDF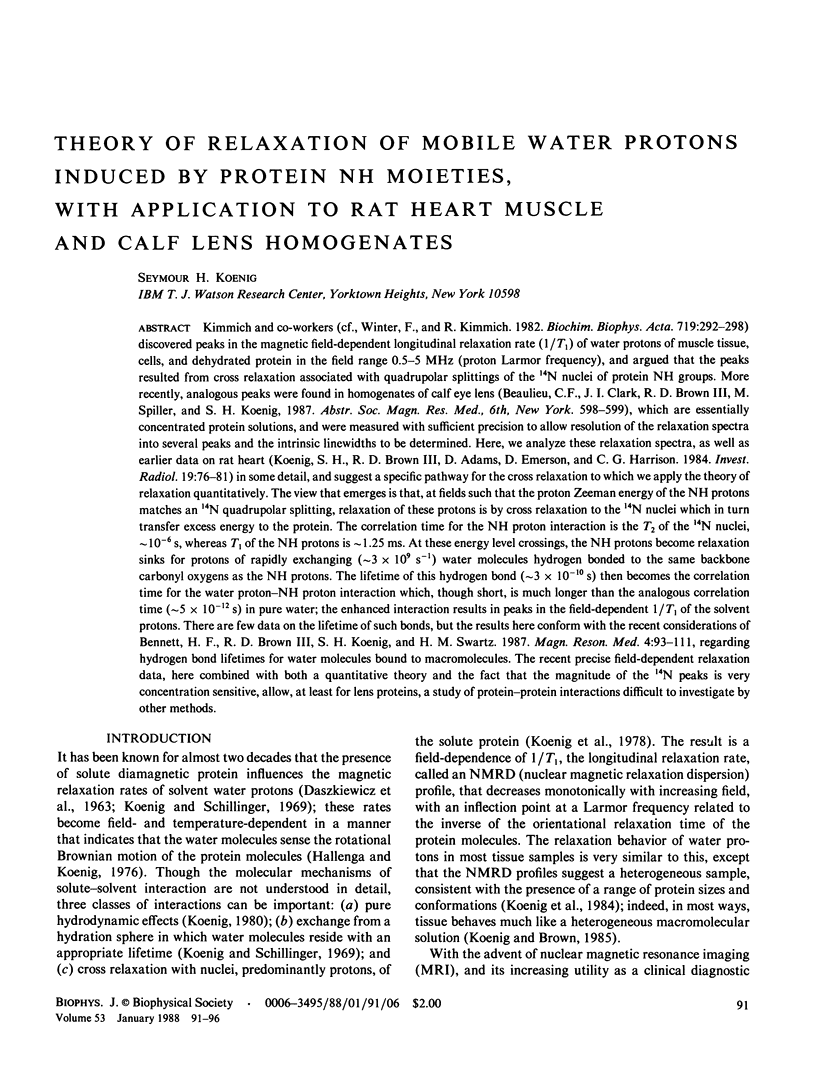
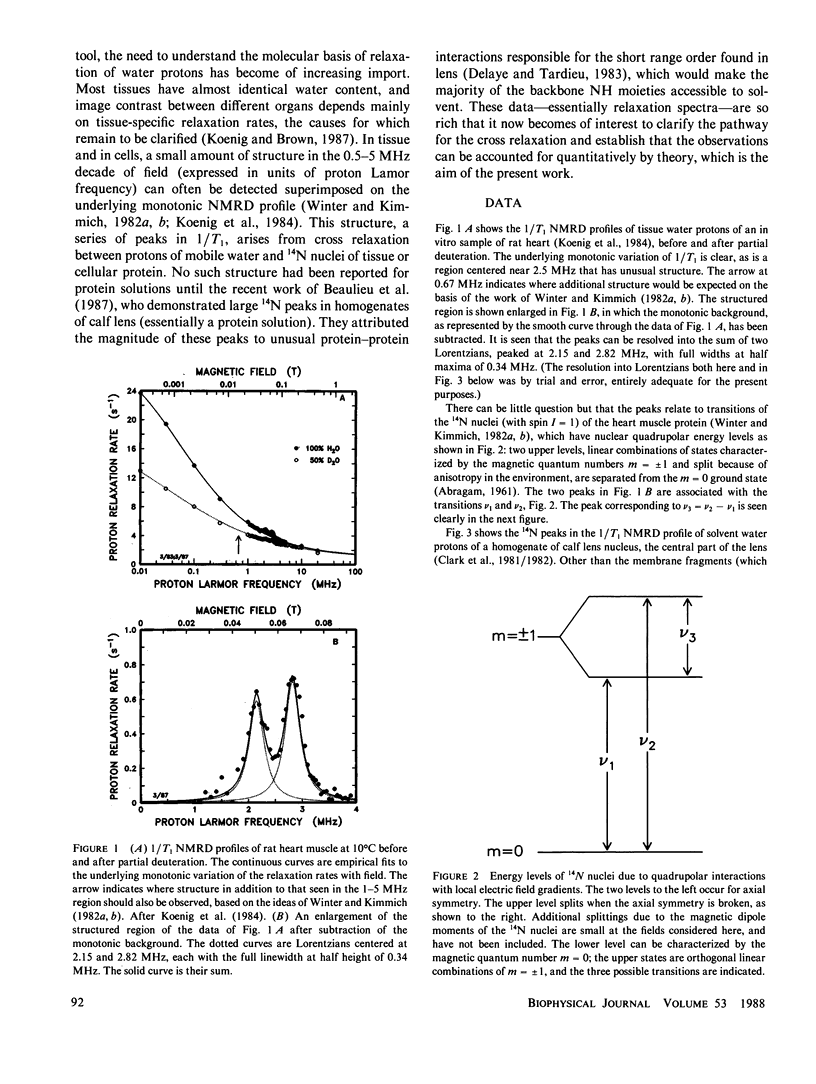
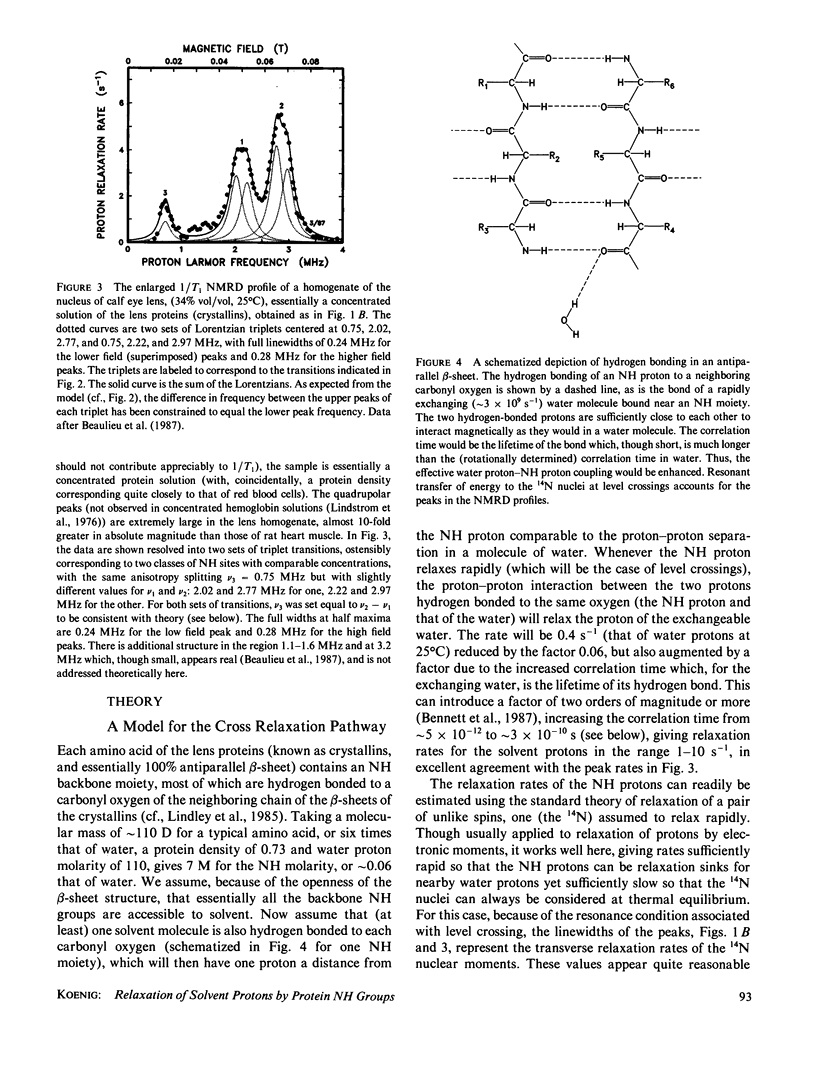
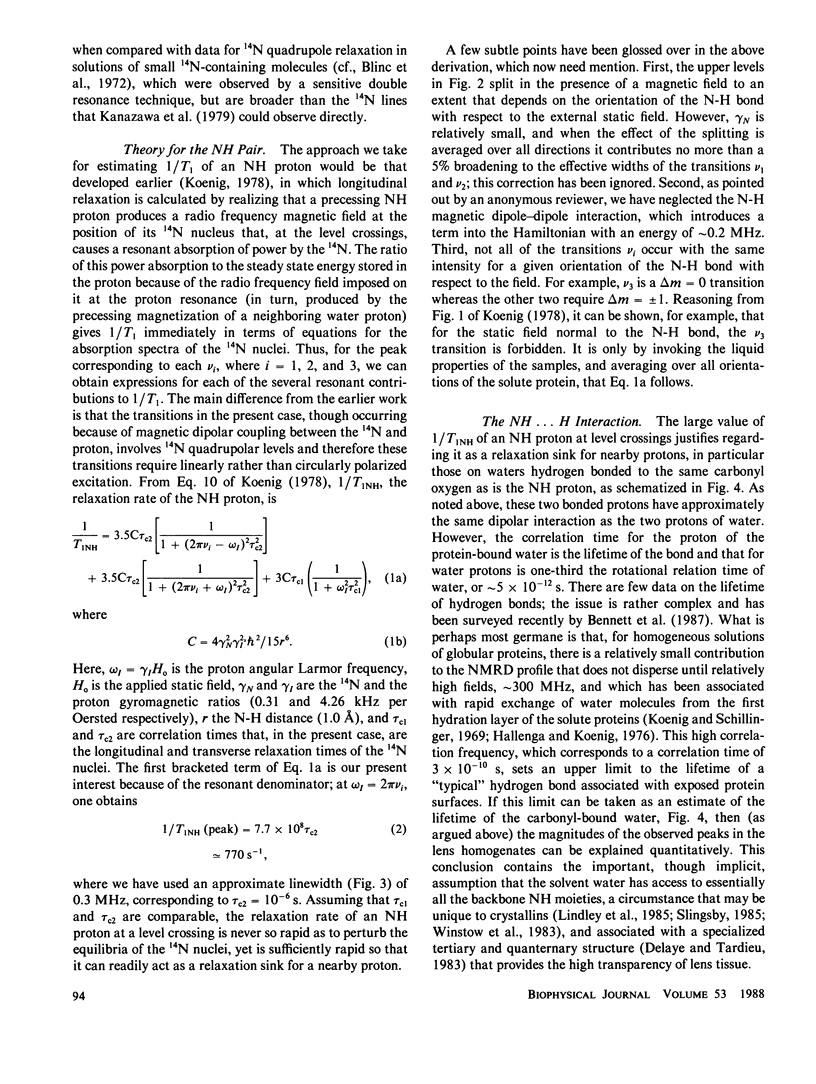
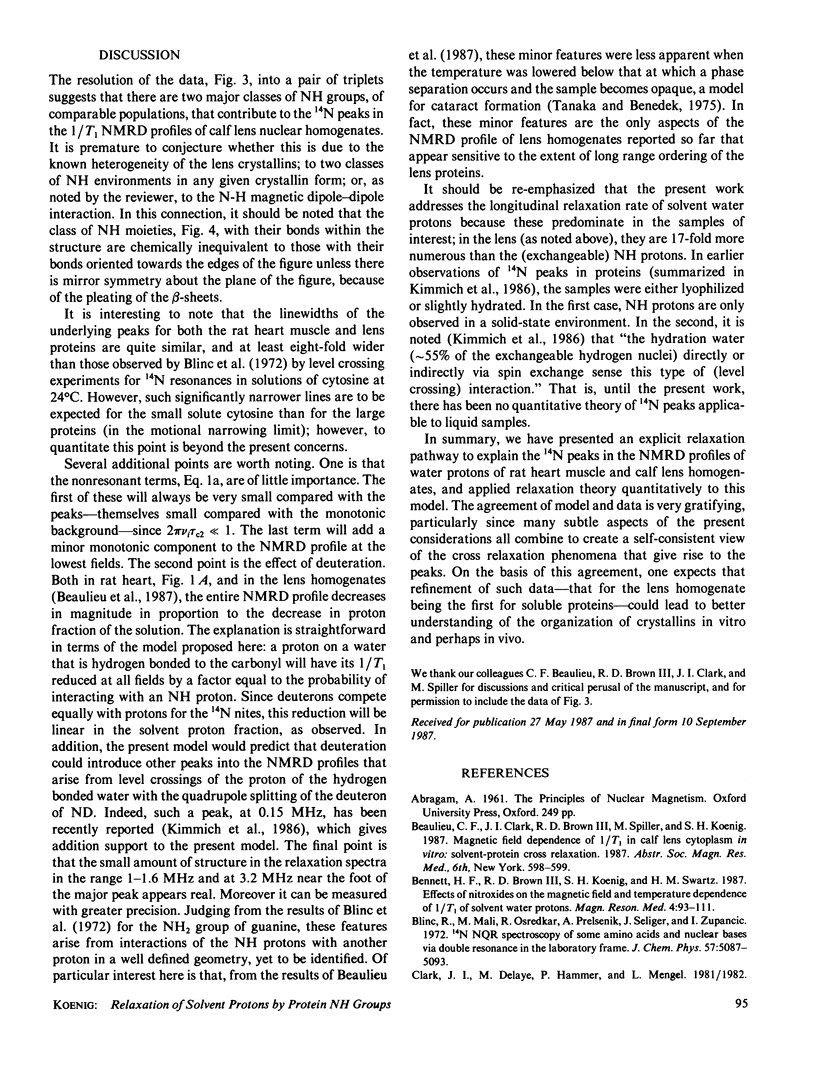
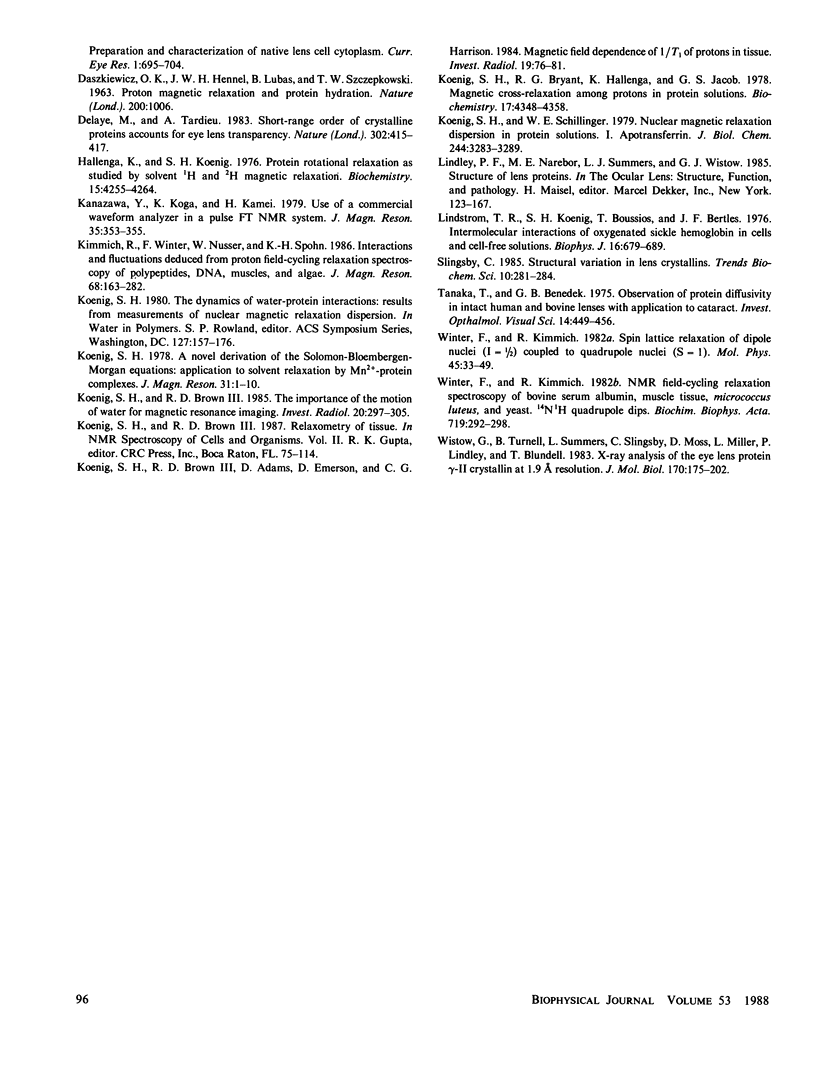
Selected References
These references are in PubMed. This may not be the complete list of references from this article.
- Bennett H. F., Brown R. D., 3rd, Koenig S. H., Swartz H. M. Effects of nitroxides on the magnetic field and temperature dependence of 1/T1 of solvent water protons. Magn Reson Med. 1987 Feb;4(2):93–111. doi: 10.1002/mrm.1910040202. [DOI] [PubMed] [Google Scholar]
- Delaye M., Tardieu A. Short-range order of crystallin proteins accounts for eye lens transparency. 1983 Mar 31-Apr 6Nature. 302(5907):415–417. doi: 10.1038/302415a0. [DOI] [PubMed] [Google Scholar]
- Hallenga K., Koenig S. H. Protein rotational relaxation as studied by solvent 1H and 2H magnetic relaxation. Biochemistry. 1976 Sep 21;15(19):4255–4264. doi: 10.1021/bi00664a019. [DOI] [PubMed] [Google Scholar]
- Koenig S. H., Brown R. D., 3rd, Adams D., Emerson D., Harrison C. G. Magnetic field dependence of 1/T1 of protons in tissue. Invest Radiol. 1984 Mar-Apr;19(2):76–81. doi: 10.1097/00004424-198403000-00002. [DOI] [PubMed] [Google Scholar]
- Koenig S. H., Brown R. D., 3rd The importance of the motion of water for magnetic resonance imaging. Invest Radiol. 1985 May-Jun;20(3):297–305. doi: 10.1097/00004424-198505000-00013. [DOI] [PubMed] [Google Scholar]
- Koenig S. H., Bryant R. G., Hallenga K., Jacob G. S. Magnetic cross-relaxation among protons in protein solutions. Biochemistry. 1978 Oct 3;17(20):4348–4358. doi: 10.1021/bi00613a037. [DOI] [PubMed] [Google Scholar]
- Koenig S. H., Schillinger W. E. Nuclear magnetic relaxation dispersion in protein solutions. I. Apotransferrin. J Biol Chem. 1969 Jun 25;244(12):3283–3289. [PubMed] [Google Scholar]
- Lindstrom T. R., Koenig S. H., Boussios T., Bertles J. F. Intermolecular interactions of oxygenated sickle hemoglobin molecules in cells and cell-free solutions. Biophys J. 1976 Jun;16(6):679–689. doi: 10.1016/S0006-3495(76)85721-9. [DOI] [PMC free article] [PubMed] [Google Scholar]
- Tanaka T., Benedek G. B. Observation of protein diffusivity in intact human and bovine lenses with application to cataract. Invest Ophthalmol. 1975 Jun;14(6):449–456. [PubMed] [Google Scholar]
- Winter F., Kimmich R. NMR field-cycling relaxation spectroscopy of bovine serum albumin, muscle tissue, Micrococcus luteus and yeast. 14N1H-quadrupole dips. Biochim Biophys Acta. 1982 Nov 24;719(2):292–298. doi: 10.1016/0304-4165(82)90101-5. [DOI] [PubMed] [Google Scholar]
- Wistow G., Turnell B., Summers L., Slingsby C., Moss D., Miller L., Lindley P., Blundell T. X-ray analysis of the eye lens protein gamma-II crystallin at 1.9 A resolution. J Mol Biol. 1983 Oct 15;170(1):175–202. doi: 10.1016/s0022-2836(83)80232-0. [DOI] [PubMed] [Google Scholar]