Abstract
An effect of internal citrate ions on excitation-contraction coupling in skeletal muscle is described. The threshold for contraction was measured in rat extensor digitorum longus, (EDL), and soleus muscle fibers using a two microelectrode voltage clamp technique with either KCl-filled or K3 citrate-filled current electrodes. Contraction thresholds were stable for many minutes with KCl current electrodes. In contrast, thresholds fell progressively towards the resting membrane potential, by as much as -15 mV over a period of 10 to 20 min of voltage-clamp with citrate current electrodes. In addition, prepulse inhibition was suppressed, subthreshold activation enhanced and steady-state inactivation shifted to more negative potentials. Fibers recovered slowly from these effects when the citrate electrode was withdrawn and replaced with a KCl electrode. The changes in contraction threshold suggest that citrate ions act on the muscle activation system at an intracellular site, since the citrate permeability of the surface membrane is probably very low. An internal citrate concentration of 5 mM was calculated to result from citrate diffusion out of the microelectrode into the recording area for 20 min. 5 mM citrate added to an artificial cell lowered the free calcium concentration from 240 to 31 microM. It is suggested that citrate modifies excitation-contraction coupling either by acting upon an anion-dependent step in activation or by reducing the free calcium and/or free magnesium concentration in the myoplasm.
Full text
PDF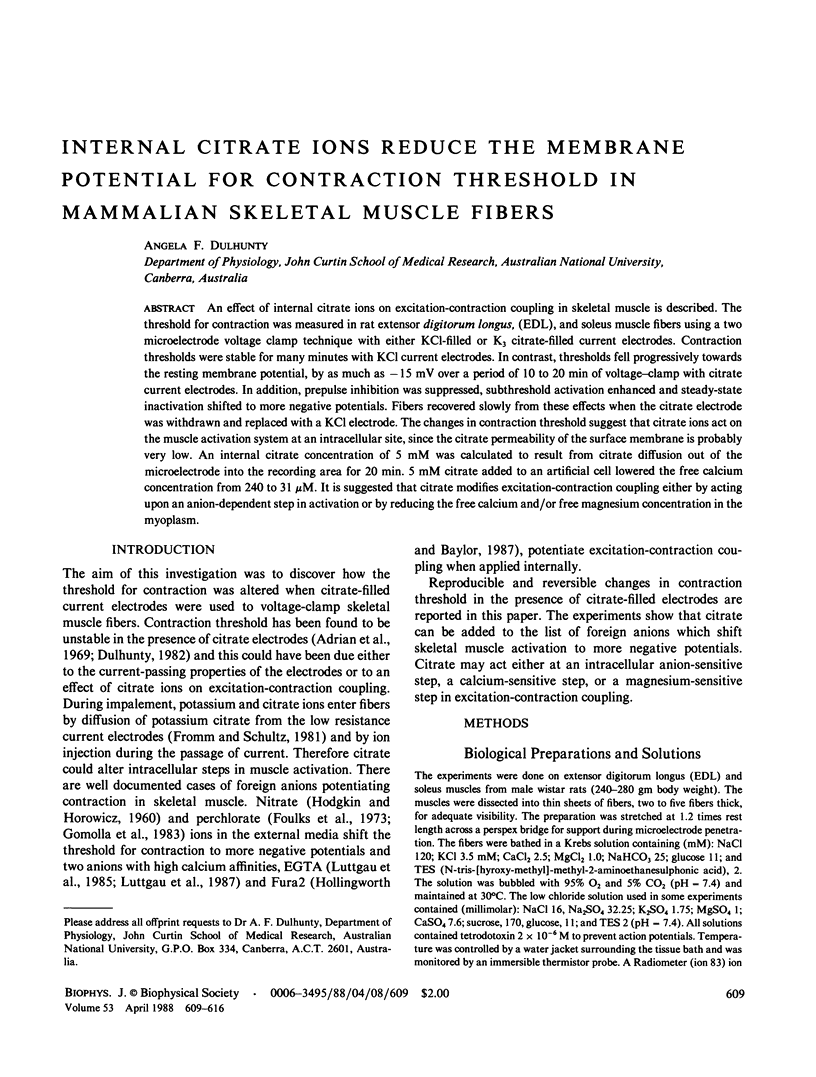
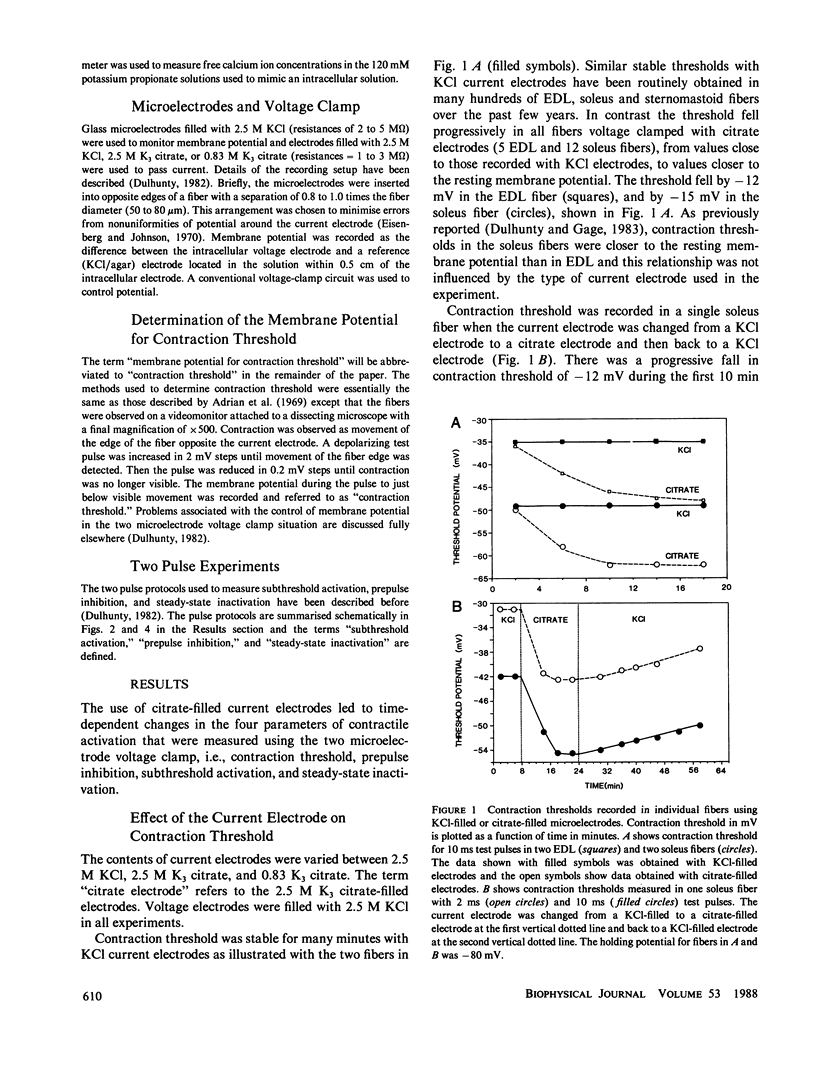
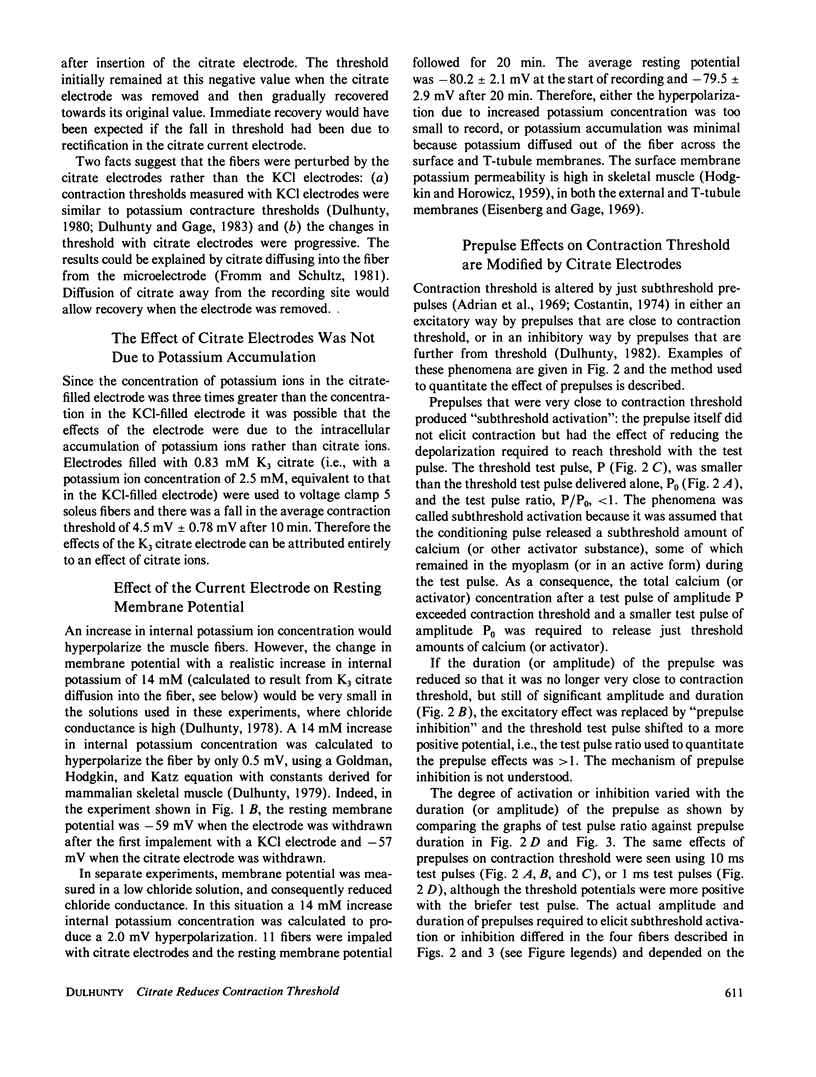
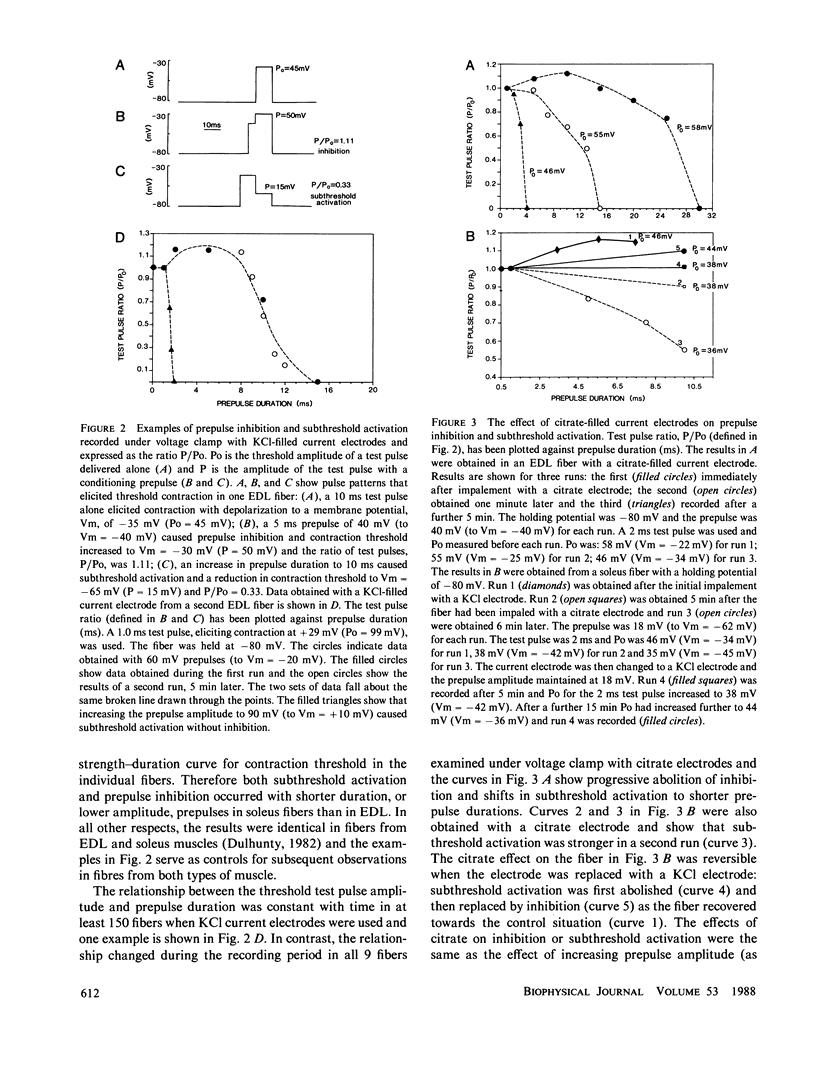
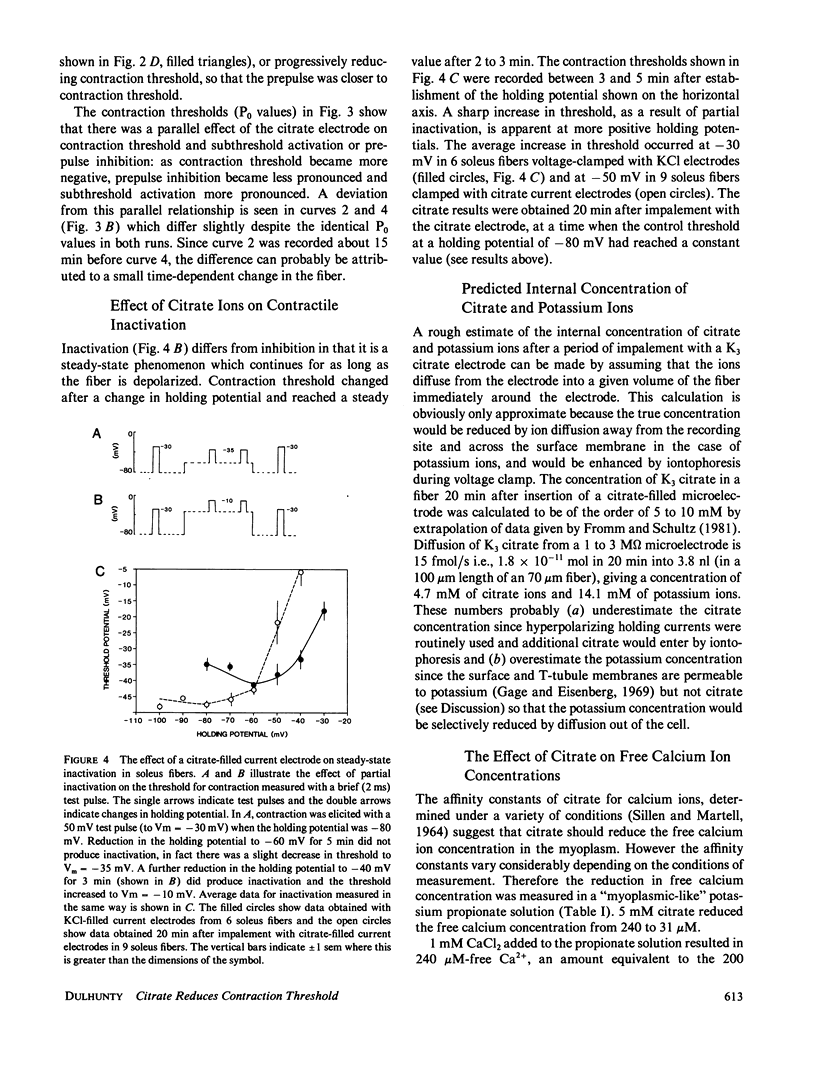
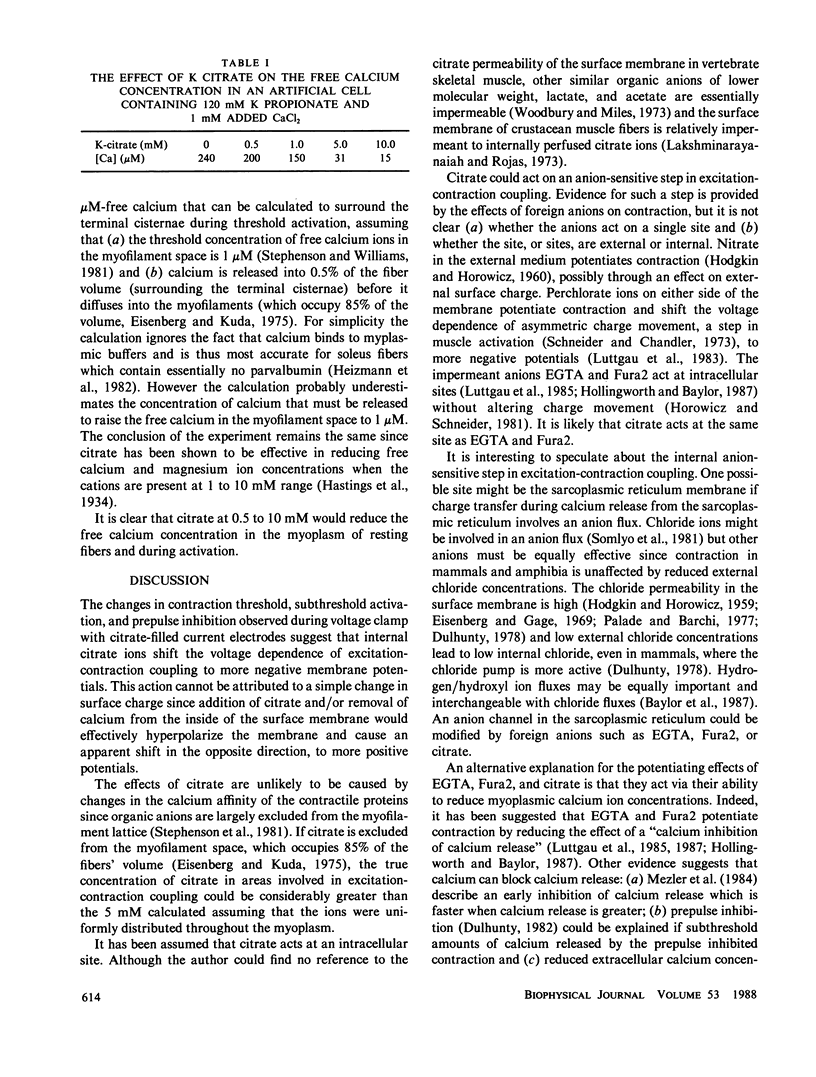
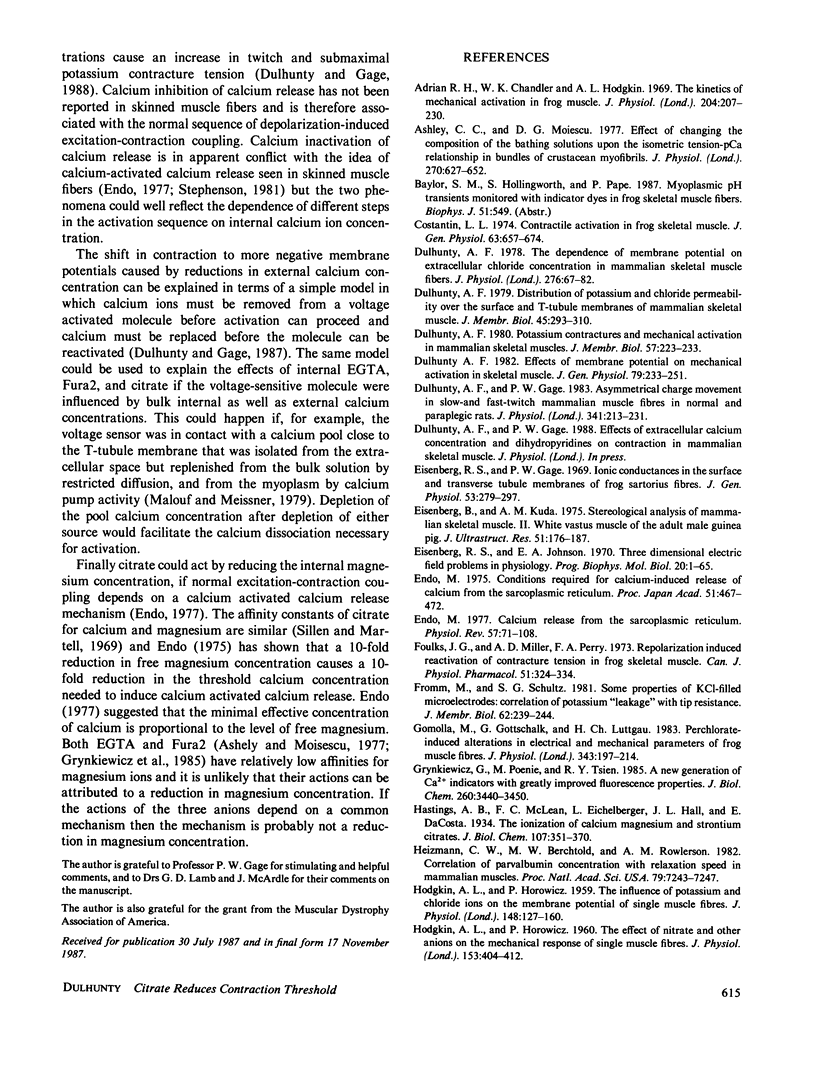
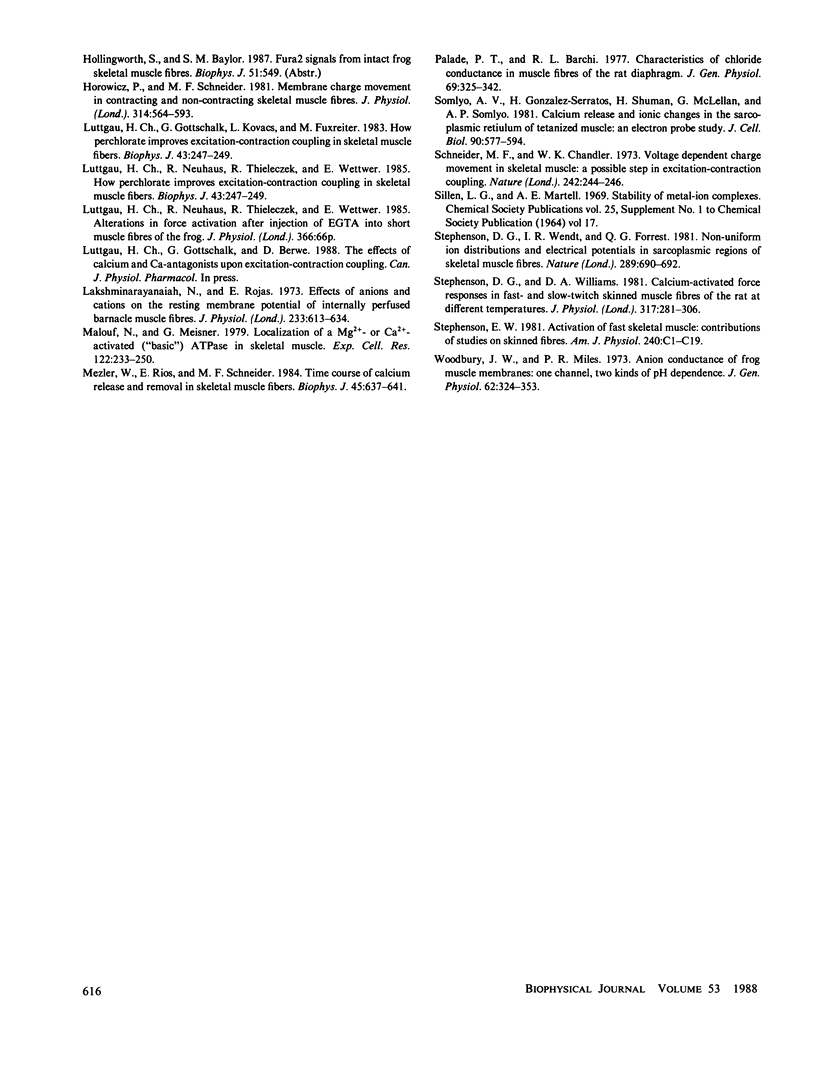
Selected References
These references are in PubMed. This may not be the complete list of references from this article.
- Adrian R. H., Chandler W. K., Hodgkin A. L. The kinetics of mechanical activation in frog muscle. J Physiol. 1969 Sep;204(1):207–230. doi: 10.1113/jphysiol.1969.sp008909. [DOI] [PMC free article] [PubMed] [Google Scholar]
- Ashley C. C., Moisescu D. G. Effect of changing the composition of the bathing solutions upon the isometric tension-pCa relationship in bundles of crustacean myofibrils. J Physiol. 1977 Sep;270(3):627–652. doi: 10.1113/jphysiol.1977.sp011972. [DOI] [PMC free article] [PubMed] [Google Scholar]
- Costantin L. L. Contractile activation in frog skeletal muscle. J Gen Physiol. 1974 Jun;63(6):657–674. doi: 10.1085/jgp.63.6.657. [DOI] [PMC free article] [PubMed] [Google Scholar]
- Dulhunty A. F. Distribution of potassium and chloride permeability over the surface and T-tubule membranes of mammalian skeletal muscle. J Membr Biol. 1979 Apr 9;45(3-4):293–310. doi: 10.1007/BF01869290. [DOI] [PubMed] [Google Scholar]
- Dulhunty A. F. Effects of membrane potential on mechanical activation in skeletal muscle. J Gen Physiol. 1982 Feb;79(2):233–251. doi: 10.1085/jgp.79.2.233. [DOI] [PMC free article] [PubMed] [Google Scholar]
- Dulhunty A. F., Gage P. W. Asymmetrical charge movement in slow- and fast-twitch mammalian muscle fibres in normal and paraplegic rats. J Physiol. 1983 Aug;341:213–231. doi: 10.1113/jphysiol.1983.sp014802. [DOI] [PMC free article] [PubMed] [Google Scholar]
- Dulhunty A. F. Potassium contractures and mechanical activation in mammalian skeletal muscles. J Membr Biol. 1980 Dec 30;57(3):223–233. doi: 10.1007/BF01869590. [DOI] [PubMed] [Google Scholar]
- Dulhunty A. F. The dependence of membrane potential on extracellular chloride concentration in mammalian skeletal muscle fibres. J Physiol. 1978 Mar;276:67–82. doi: 10.1113/jphysiol.1978.sp012220. [DOI] [PMC free article] [PubMed] [Google Scholar]
- Eisenberg B. R., Kuda A. M. Stereological analysis of mammalian skeletal muscle. II. White vastus muscle of the adult guinea pig. J Ultrastruct Res. 1975 May;51(2):176–187. doi: 10.1016/s0022-5320(75)80146-8. [DOI] [PubMed] [Google Scholar]
- Eisenberg R. S., Gage P. W. Ionic conductances of the surface and transverse tubular membranes of frog sartorius fibers. J Gen Physiol. 1969 Mar;53(3):279–297. doi: 10.1085/jgp.53.3.279. [DOI] [PMC free article] [PubMed] [Google Scholar]
- Endo M. Calcium release from the sarcoplasmic reticulum. Physiol Rev. 1977 Jan;57(1):71–108. doi: 10.1152/physrev.1977.57.1.71. [DOI] [PubMed] [Google Scholar]
- Foulks J. G., Miller J. A., Perry F. A. Repolarization-induced reactivation of contracture tension in frog skeletal muscle. Can J Physiol Pharmacol. 1973 May;51(5):324–334. doi: 10.1139/y73-049. [DOI] [PubMed] [Google Scholar]
- Fromm M., Schultz S. G. Some properties of KCl-filled microelectrodes: correlation of potassium "leakage" with tip resistance. J Membr Biol. 1981;62(3):239–244. doi: 10.1007/BF01998169. [DOI] [PubMed] [Google Scholar]
- Gomolla M., Gottschalk G., Lüttgau H. C. Perchlorate-induced alterations in electrical and mechanical parameters of frog skeletal muscle fibres. J Physiol. 1983 Oct;343:197–214. doi: 10.1113/jphysiol.1983.sp014888. [DOI] [PMC free article] [PubMed] [Google Scholar]
- Grynkiewicz G., Poenie M., Tsien R. Y. A new generation of Ca2+ indicators with greatly improved fluorescence properties. J Biol Chem. 1985 Mar 25;260(6):3440–3450. [PubMed] [Google Scholar]
- HODGKIN A. L., HOROWICZ P. The effect of nitrate and other anions on the mechanical response of single muscle fibres. J Physiol. 1960 Sep;153:404–412. doi: 10.1113/jphysiol.1960.sp006542. [DOI] [PMC free article] [PubMed] [Google Scholar]
- HODGKIN A. L., HOROWICZ P. The influence of potassium and chloride ions on the membrane potential of single muscle fibres. J Physiol. 1959 Oct;148:127–160. doi: 10.1113/jphysiol.1959.sp006278. [DOI] [PMC free article] [PubMed] [Google Scholar]
- Heizmann C. W., Berchtold M. W., Rowlerson A. M. Correlation of parvalbumin concentration with relaxation speed in mammalian muscles. Proc Natl Acad Sci U S A. 1982 Dec;79(23):7243–7247. doi: 10.1073/pnas.79.23.7243. [DOI] [PMC free article] [PubMed] [Google Scholar]
- Horowicz P., Schneider M. F. Membrane charge movement in contracting and non-contracting skeletal muscle fibres. J Physiol. 1981 May;314:565–593. doi: 10.1113/jphysiol.1981.sp013725. [DOI] [PMC free article] [PubMed] [Google Scholar]
- Lakshminarayanaiah N., Rojas E. Effects of anions and cations on the resting membrane potential of internally perfused barnacle muscle fibres. J Physiol. 1973 Sep;233(3):613–634. doi: 10.1113/jphysiol.1973.sp010326. [DOI] [PMC free article] [PubMed] [Google Scholar]
- Lüttgau H. C., Gottschalk G., Kovács L., Fuxreiter M. How perchlorate improves excitation-contraction coupling in skeletal muscle fibers. Biophys J. 1983 Aug;43(2):247–249. doi: 10.1016/S0006-3495(83)84346-X. [DOI] [PMC free article] [PubMed] [Google Scholar]
- Malouf N. N., Meissner G. Localization of a Mg2+- or Ca2+-activated ("basic") ATPase in skeletal muscle. Exp Cell Res. 1979 Sep;122(2):233–250. doi: 10.1016/0014-4827(79)90301-x. [DOI] [PubMed] [Google Scholar]
- Melzer W., Rios E., Schneider M. F. Time course of calcium release and removal in skeletal muscle fibers. Biophys J. 1984 Mar;45(3):637–641. doi: 10.1016/S0006-3495(84)84203-4. [DOI] [PMC free article] [PubMed] [Google Scholar]
- Palade P. T., Barchi R. L. Characteristics of the chloride conductance in muscle fibers of the rat diaphragm. J Gen Physiol. 1977 Mar;69(3):325–342. doi: 10.1085/jgp.69.3.325. [DOI] [PMC free article] [PubMed] [Google Scholar]
- Schneider M. F., Chandler W. K. Voltage dependent charge movement of skeletal muscle: a possible step in excitation-contraction coupling. Nature. 1973 Mar 23;242(5395):244–246. doi: 10.1038/242244a0. [DOI] [PubMed] [Google Scholar]
- Somlyo A. V., Gonzalez-Serratos H. G., Shuman H., McClellan G., Somlyo A. P. Calcium release and ionic changes in the sarcoplasmic reticulum of tetanized muscle: an electron-probe study. J Cell Biol. 1981 Sep;90(3):577–594. doi: 10.1083/jcb.90.3.577. [DOI] [PMC free article] [PubMed] [Google Scholar]
- Stephenson D. G., Wendt I. R., Forrest Q. G. Non-uniform ion distributions and electrical potentials in sarcoplasmic regions of skeletal muscle fibres. Nature. 1981 Feb 19;289(5799):690–692. doi: 10.1038/289690a0. [DOI] [PubMed] [Google Scholar]
- Stephenson D. G., Williams D. A. Calcium-activated force responses in fast- and slow-twitch skinned muscle fibres of the rat at different temperatures. J Physiol. 1981 Aug;317:281–302. doi: 10.1113/jphysiol.1981.sp013825. [DOI] [PMC free article] [PubMed] [Google Scholar]
- Woodbury J. W., Miles P. R. Anion conductance of frog muscle membranes: one channel, two kinds of pH dependence. J Gen Physiol. 1973 Sep;62(3):324–353. doi: 10.1085/jgp.62.3.324. [DOI] [PMC free article] [PubMed] [Google Scholar]