Abstract
To test the possible role of lysine residues in Na channel function the effects of several imidoesters on Na and gating currents were studied in voltage-clamped single frog nerve fibers. Mono- and bisimidoesters were used. These reagents modify amino groups exclusively and do not change the net charge. The three bisimidoesters used easily introduce cross-links between neighboring amino groups. Their structure is almost identical; only the length of the spacers between the two amino-reactive groups is different. An irreversible reduction of Na currents and gating currents was observed with the longest (dimethyl suberimidate [DMS]) and the shortest (dimethyl adipimidate [DMA]) of the cross-linkers used. Of the three cross-linking reagents only the shortest made Na current inactivation slow and incomplete. The steady-state inactivation curve, h infinity (E), was shifted by greater than 25 mV in the hyperpolarizing direction by each of the reagents. The voltage dependence of activation, however, remained unchanged. Furthermore, the effects of two different monoimidoesters (ethyl acetimidate [EAI] and isethionyl acetimidate [IAI]) on gating currents were tested. EAI can penetrate a membrane, whereas IAI is membrane impermeant. IAI was almost without effect, whereas EAI caused a considerable reduction of the gating currents. EAI and DMS reduced the Qoff/Qon ratio without affecting the decay of the Na currents. The results show that lysine residues are critically involved in Na channel gating.
Full text
PDF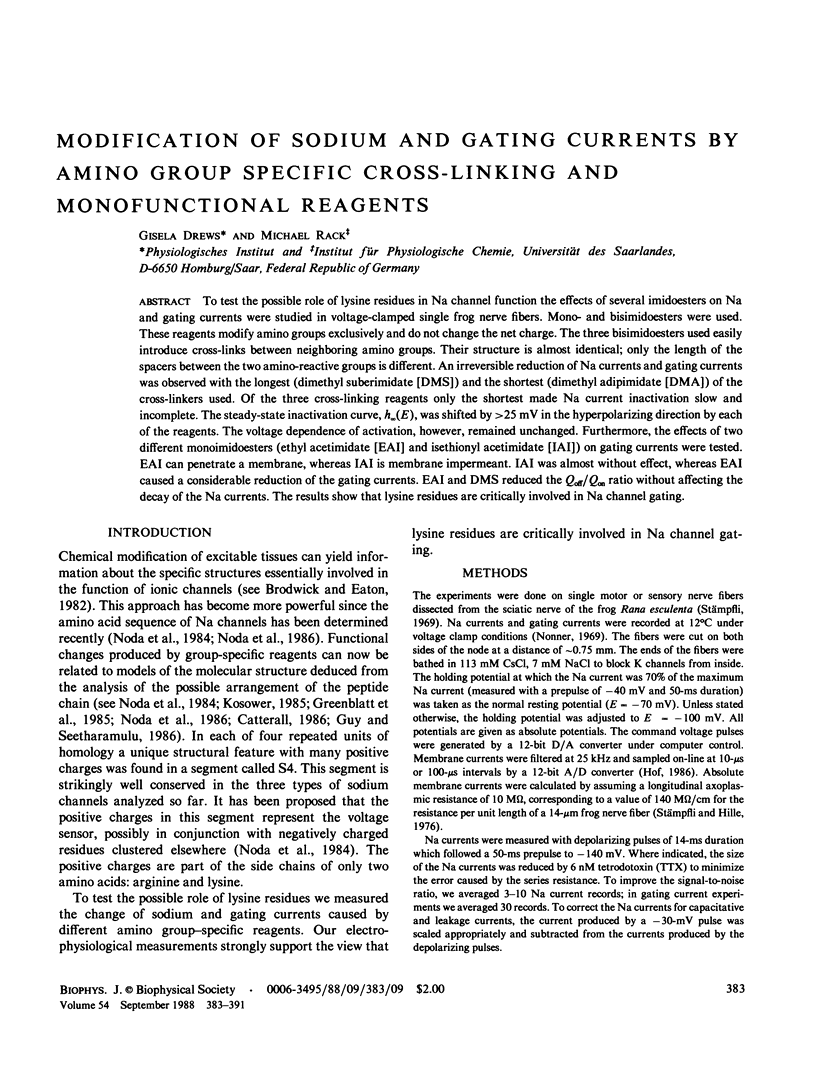
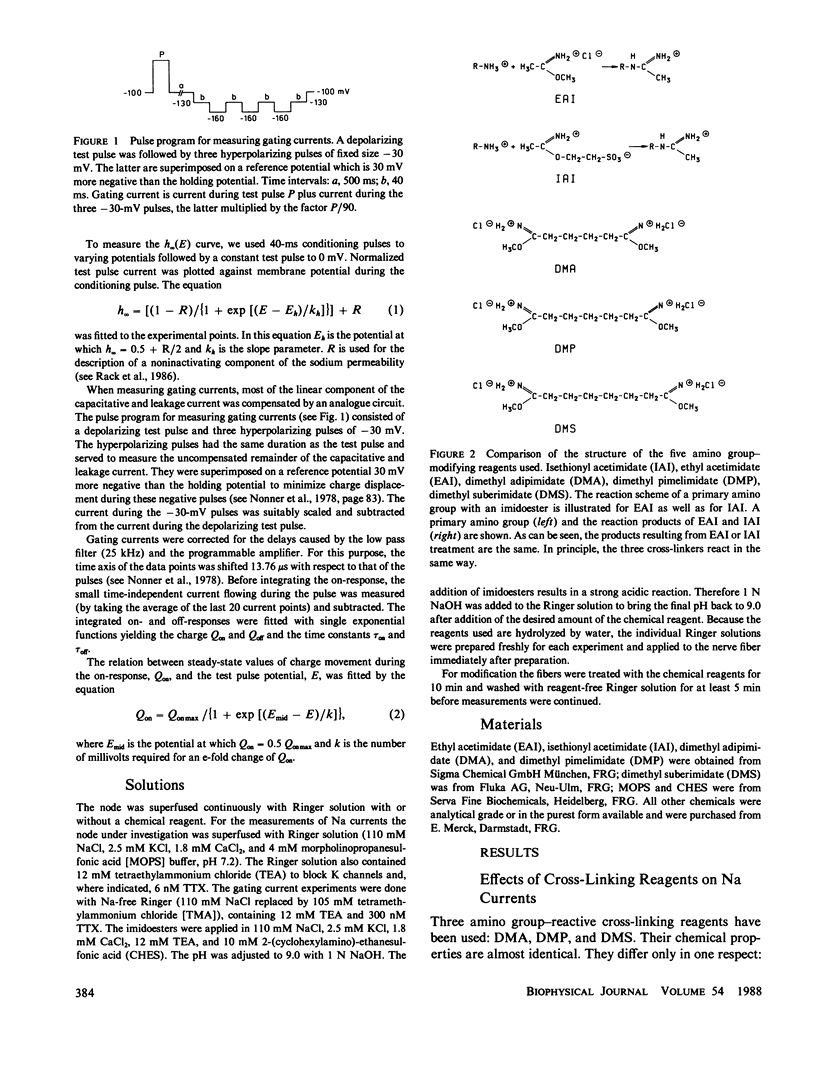
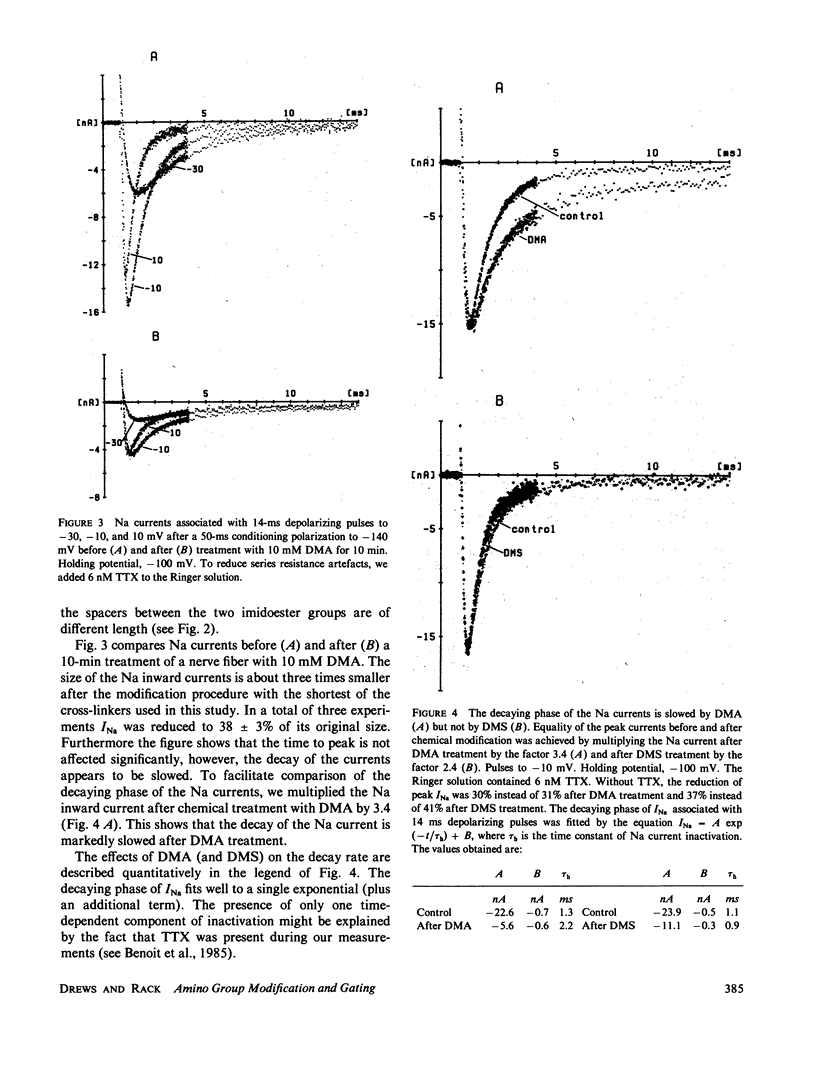
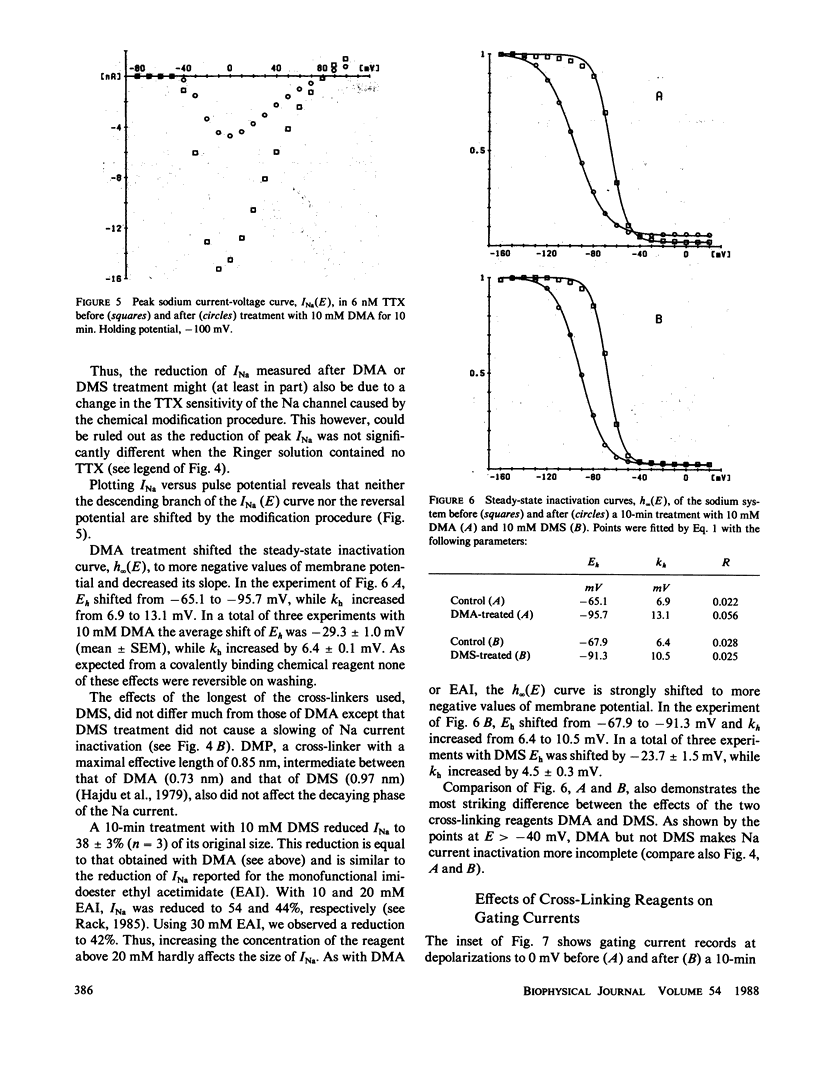
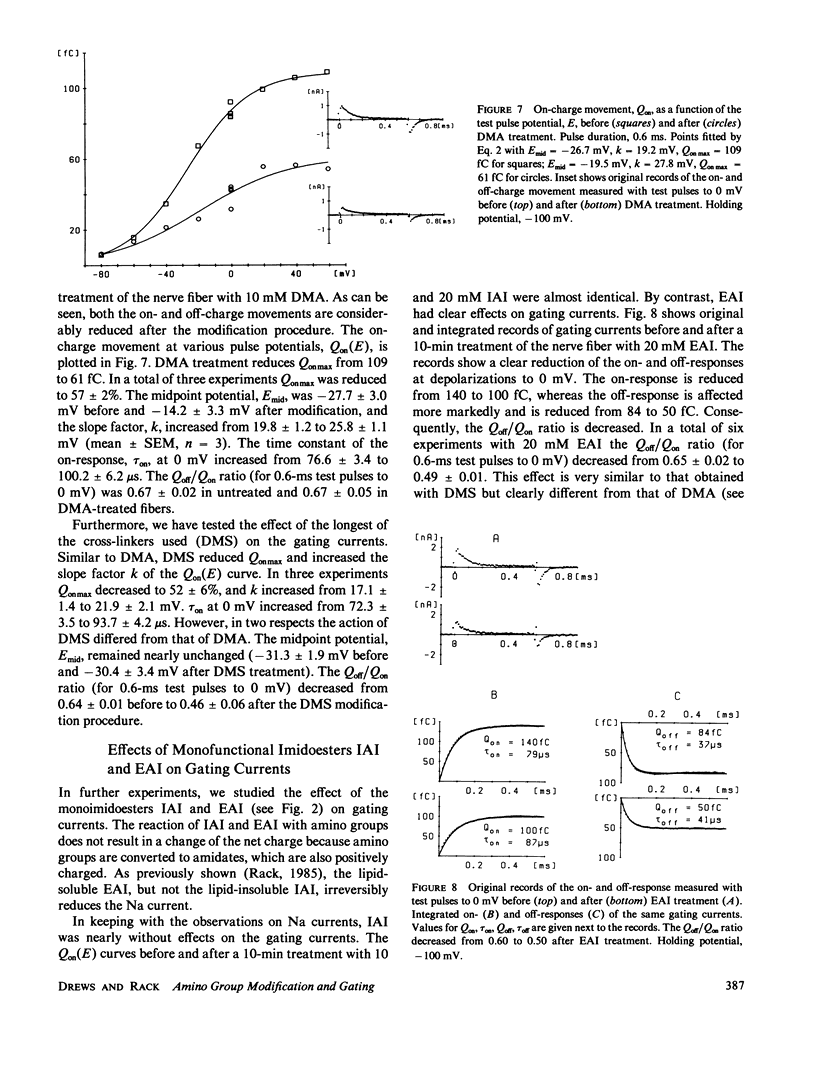
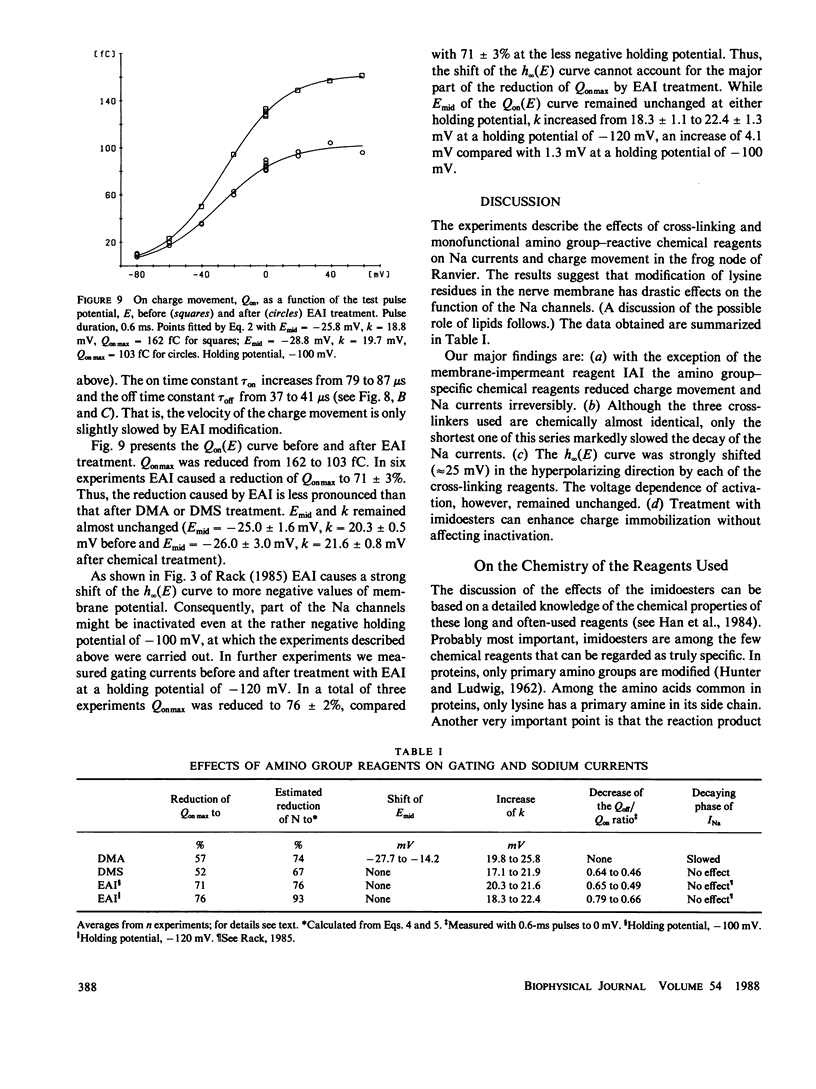
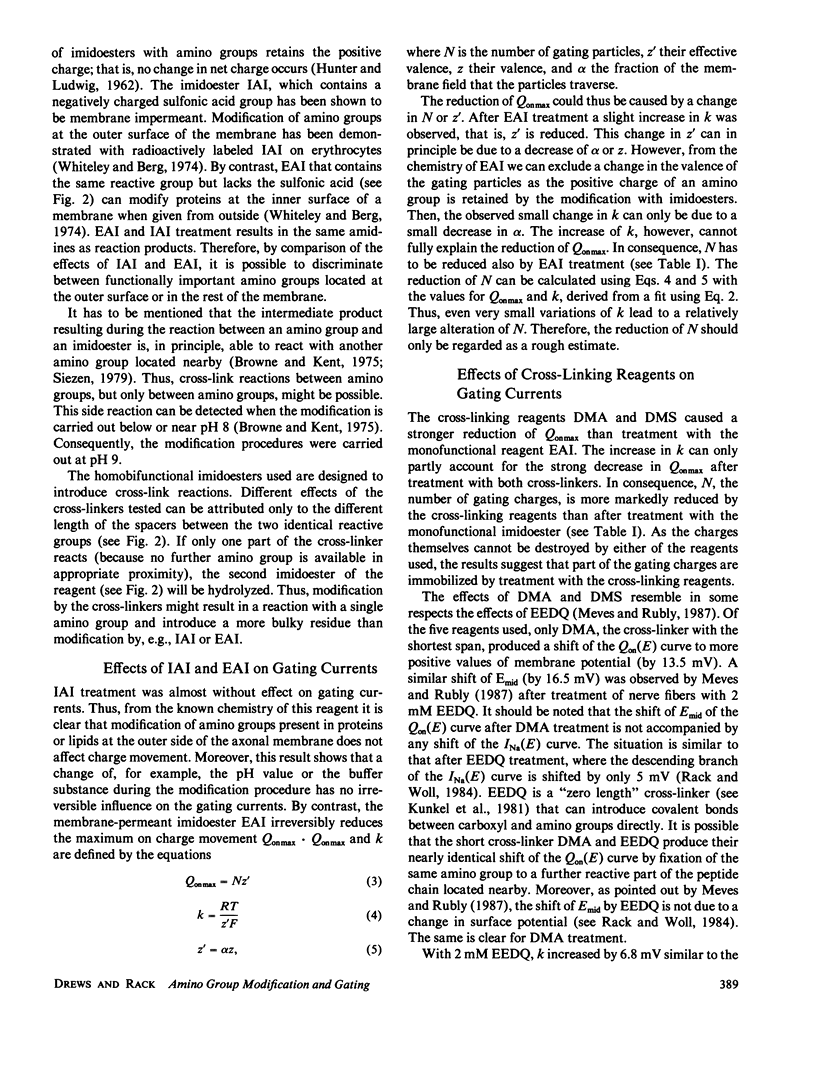
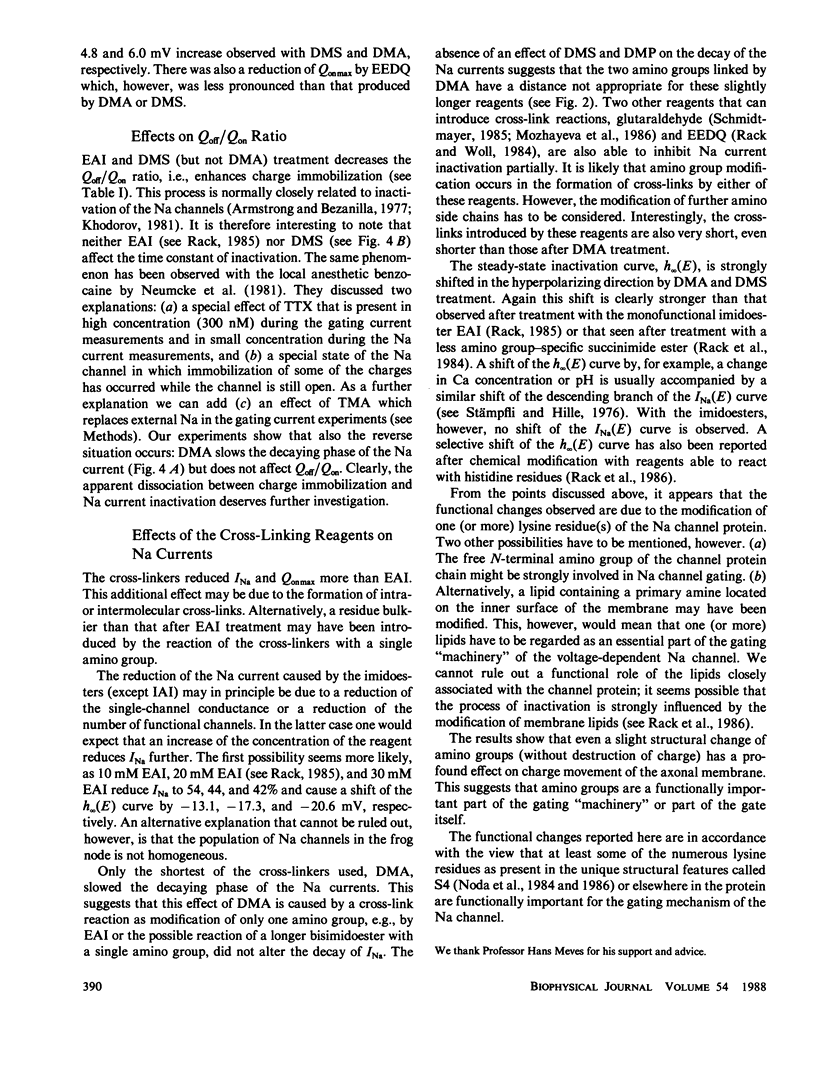
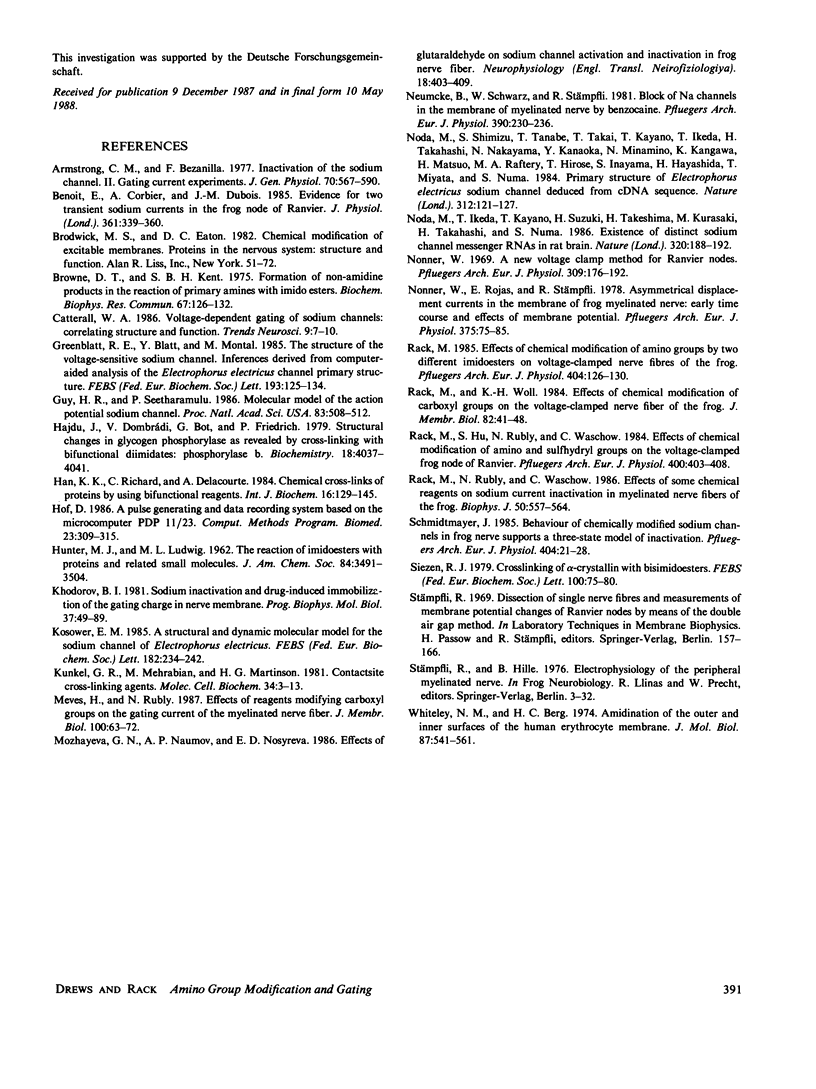
Selected References
These references are in PubMed. This may not be the complete list of references from this article.
- Armstrong C. M., Bezanilla F. Inactivation of the sodium channel. II. Gating current experiments. J Gen Physiol. 1977 Nov;70(5):567–590. doi: 10.1085/jgp.70.5.567. [DOI] [PMC free article] [PubMed] [Google Scholar]
- Benoit E., Corbier A., Dubois J. M. Evidence for two transient sodium currents in the frog node of Ranvier. J Physiol. 1985 Apr;361:339–360. doi: 10.1113/jphysiol.1985.sp015649. [DOI] [PMC free article] [PubMed] [Google Scholar]
- Brodwick M. S., Eaton D. C. Chemical modification of excitable membranes. Prog Clin Biol Res. 1982;79:51–72. [PubMed] [Google Scholar]
- Browne D. T., Kent S. B. Formation of non-amidine products in the reaction of primary amines with imido esters. Biochem Biophys Res Commun. 1975 Nov 3;67(1):126–132. doi: 10.1016/0006-291x(75)90292-2. [DOI] [PubMed] [Google Scholar]
- Greenblatt R. E., Blatt Y., Montal M. The structure of the voltage-sensitive sodium channel. Inferences derived from computer-aided analysis of the Electrophorus electricus channel primary structure. FEBS Lett. 1985 Dec 2;193(2):125–134. doi: 10.1016/0014-5793(85)80136-8. [DOI] [PubMed] [Google Scholar]
- Guy H. R., Seetharamulu P. Molecular model of the action potential sodium channel. Proc Natl Acad Sci U S A. 1986 Jan;83(2):508–512. doi: 10.1073/pnas.83.2.508. [DOI] [PMC free article] [PubMed] [Google Scholar]
- Hajdu J., Dombrádi V., Bot G., Friedrich P. Structural changes in glycogen phosphorlase as revealed by cross-linking with bifunctional diimidates: phosphorylase b. Biochemistry. 1979 Sep 4;18(18):4037–4041. doi: 10.1021/bi00585a030. [DOI] [PubMed] [Google Scholar]
- Hof D. A pulse generating and data recording system based on the microcomputer PDP 11/23. Comput Methods Programs Biomed. 1986 Dec;23(3):309–315. doi: 10.1016/0169-2607(86)90065-9. [DOI] [PubMed] [Google Scholar]
- Khodorov B. I. Sodium inactivation and drug-induced immobilization of the gating charge in nerve membrane. Prog Biophys Mol Biol. 1981;37(2):49–89. doi: 10.1016/0079-6107(82)90020-7. [DOI] [PubMed] [Google Scholar]
- Kosower E. M. A structural and dynamic molecular model for the sodium channel of Electrophorus electricus. FEBS Lett. 1985 Mar 25;182(2):234–242. doi: 10.1016/0014-5793(85)80306-9. [DOI] [PubMed] [Google Scholar]
- Kunkel G. R., Mehrabian M., Martinson H. G. Contact-site cross-linking agents. Mol Cell Biochem. 1981 Jan 20;34(1):3–13. doi: 10.1007/BF02354846. [DOI] [PubMed] [Google Scholar]
- Meves H., Rubly N. Effects of reagents modifying carboxyl groups on the gating current of the myelinated nerve fiber. J Membr Biol. 1987;100(1):63–72. doi: 10.1007/BF02209141. [DOI] [PubMed] [Google Scholar]
- Neumcke B., Schwarz W., Stämpfli R. Block of Na channels in the membrane of myelinated nerve by benzocaine. Pflugers Arch. 1981 Jun;390(3):230–236. doi: 10.1007/BF00658267. [DOI] [PubMed] [Google Scholar]
- Noda M., Ikeda T., Kayano T., Suzuki H., Takeshima H., Kurasaki M., Takahashi H., Numa S. Existence of distinct sodium channel messenger RNAs in rat brain. Nature. 1986 Mar 13;320(6058):188–192. doi: 10.1038/320188a0. [DOI] [PubMed] [Google Scholar]
- Noda M., Shimizu S., Tanabe T., Takai T., Kayano T., Ikeda T., Takahashi H., Nakayama H., Kanaoka Y., Minamino N. Primary structure of Electrophorus electricus sodium channel deduced from cDNA sequence. Nature. 1984 Nov 8;312(5990):121–127. doi: 10.1038/312121a0. [DOI] [PubMed] [Google Scholar]
- Nonner W. A new voltage clamp method for Ranvier nodes. Pflugers Arch. 1969;309(2):176–192. doi: 10.1007/BF00586967. [DOI] [PubMed] [Google Scholar]
- Nonner W., Rojas E., Stämpfli R. Asymmetrical displacement currents in the membrane of frog myelinated nerve: early time course and effects of membrane potential. Pflugers Arch. 1978 Jun 21;375(1):75–85. doi: 10.1007/BF00584151. [DOI] [PubMed] [Google Scholar]
- Rack M. Effects of chemical modification of amino groups by two different imidoesters on voltage-clamped nerve fibres of the frog. Pflugers Arch. 1985 May;404(2):126–130. doi: 10.1007/BF00585407. [DOI] [PubMed] [Google Scholar]
- Rack M., Hu S. L., Rubly N., Waschow C. Effects of chemical modification of amino and sulfhydryl groups on the voltage-clamped frog node of Ranvier. Pflugers Arch. 1984 Apr;400(4):403–408. doi: 10.1007/BF00587540. [DOI] [PubMed] [Google Scholar]
- Rack M., Rubly N., Waschow C. Effects of some chemical reagents on sodium current inactivation in myelinated nerve fibers of the frog. Biophys J. 1986 Oct;50(4):557–564. doi: 10.1016/S0006-3495(86)83495-6. [DOI] [PMC free article] [PubMed] [Google Scholar]
- Rack M., Woll K. H. Effects of chemical modification of carboxyl groups on the voltage-clamped nerve fiber of the frog. J Membr Biol. 1984;82(1):41–48. doi: 10.1007/BF01870730. [DOI] [PubMed] [Google Scholar]
- Schmidtmayer J. Behaviour of chemically modified sodium channels in frog nerve supports a three-state model of inactivation. Pflugers Arch. 1985 May;404(1):21–28. doi: 10.1007/BF00581486. [DOI] [PubMed] [Google Scholar]
- Siezen R. J. Crosslinking of alpha-crystallin with bisimidoesters. Evidence for polyamidine formation at pH8 from an increase in positive charges on the polypeptide chains. FEBS Lett. 1979 Apr 1;100(1):75–80. doi: 10.1016/0014-5793(79)81134-5. [DOI] [PubMed] [Google Scholar]
- Whiteley N. M., Berg H. C. Amidination of the outer and inner surfaces of the human erythrocyte membrane. J Mol Biol. 1974 Aug 15;87(3):541–561. doi: 10.1016/0022-2836(74)90103-x. [DOI] [PubMed] [Google Scholar]