Abstract
Several recent independent studies on macroscopic Ca currents have demonstrated the anomalous mole fraction effect in mixtures of Ca and Ba at concentrations of 10 mM or less. Recently, Hess and Tsien (1984; Nature 309) proposed a dual binding site model, based upon Eyring rate theory, to account for this effect in L-type cardiac Ca channels. This model predicts that the anomalous mole fraction effect can be accounted for solely in terms of open single channel permeation properties; it was able to adequately reproduce the effect for macroscopic Ca currents recorded in 10 mM solutions. However, the electrochemical gradients under which single Ca channel current recordings are routinely made with the patch clamp technique vary dramatically from those used for macroscopic Ca currents. To properly assess the general validity of the Hess and Tsien model at the single Ca channel level, the effects of both large electrical potentials and elevated divalent concentrations must be understood. Computer simulations were therefore carried out using the original parameters used by Hess and Tsien under conditions designed to mimic those used in patch clamp studies. The permeation behavior generated by this model is quite complex. In particular, hyperpolarization and increased divalent concentration combine to reduce and ultimately abolish the anomalous mole fraction effect. It may therefore be very difficult to observe the anomalous mole fraction effect at the single Ca channel level; the dual-site model displays a relationship between current and mole fraction generally associated with a single-site model under the conditions frequently employed to resolve single Ca channel activity. Nonetheless, analysis of such monotonic mole fraction behavior can still be used as a test for the general validity of the dual-site model. Apparent Kms for Ca and Ba can be extracted from such monotonic behavior, and may not only be functions of membrane potential but may also depend upon the total divalent cation concentration. This is a unique prediction which is incompatible with the simple single-site model. Our analysis provides (a) a possible resolution for the apparent discrepancies presently existing in the experimental literature regarding the existence of the anomalous mole fraction effect at the single Ca channel level, (b) a mechanistic description of previously unexplained observations on the voltage-dependence of the anomalous mole fraction effect, and (c) a useful theoretical framework for future experimentation designed to test the general validity of the dual binding site model of the Ca channel.
Full text
PDF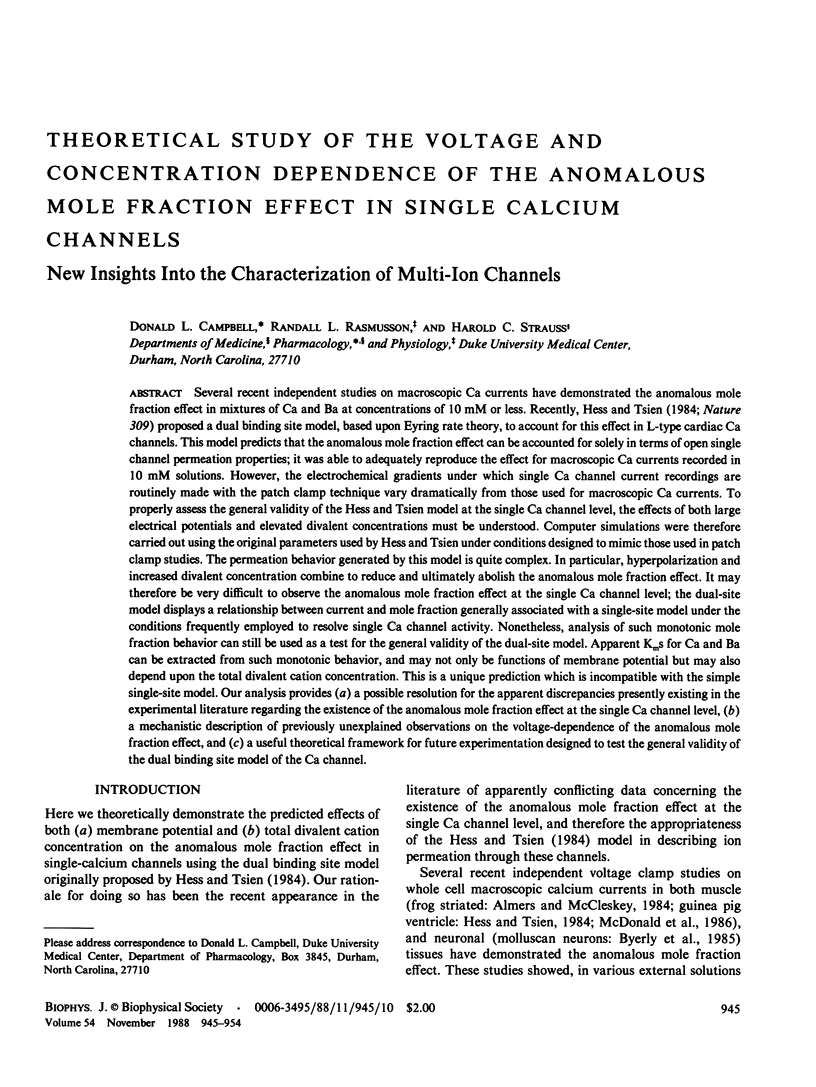
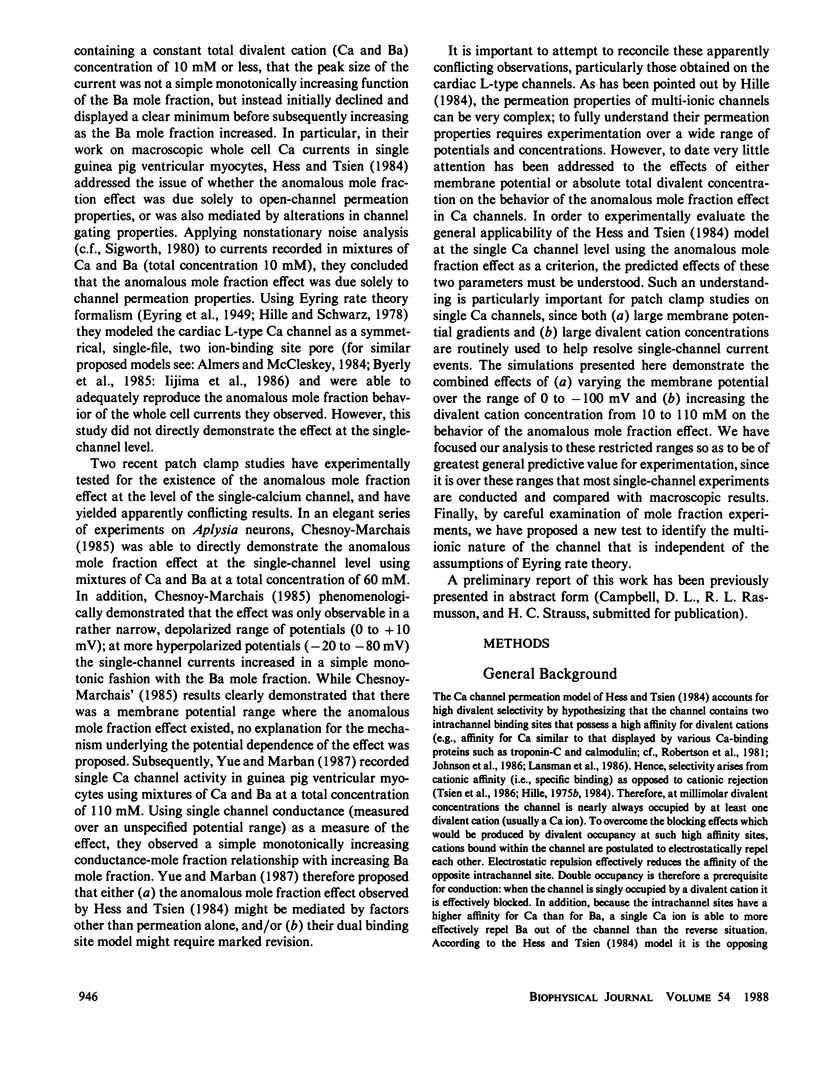
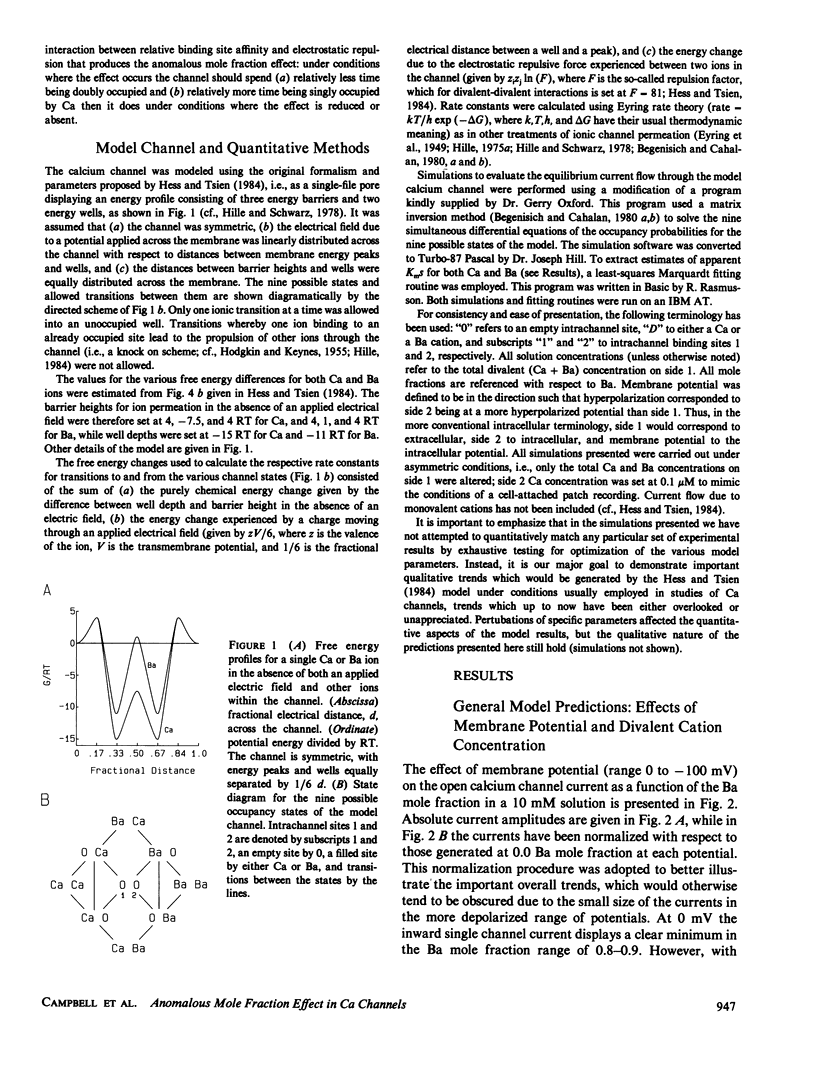
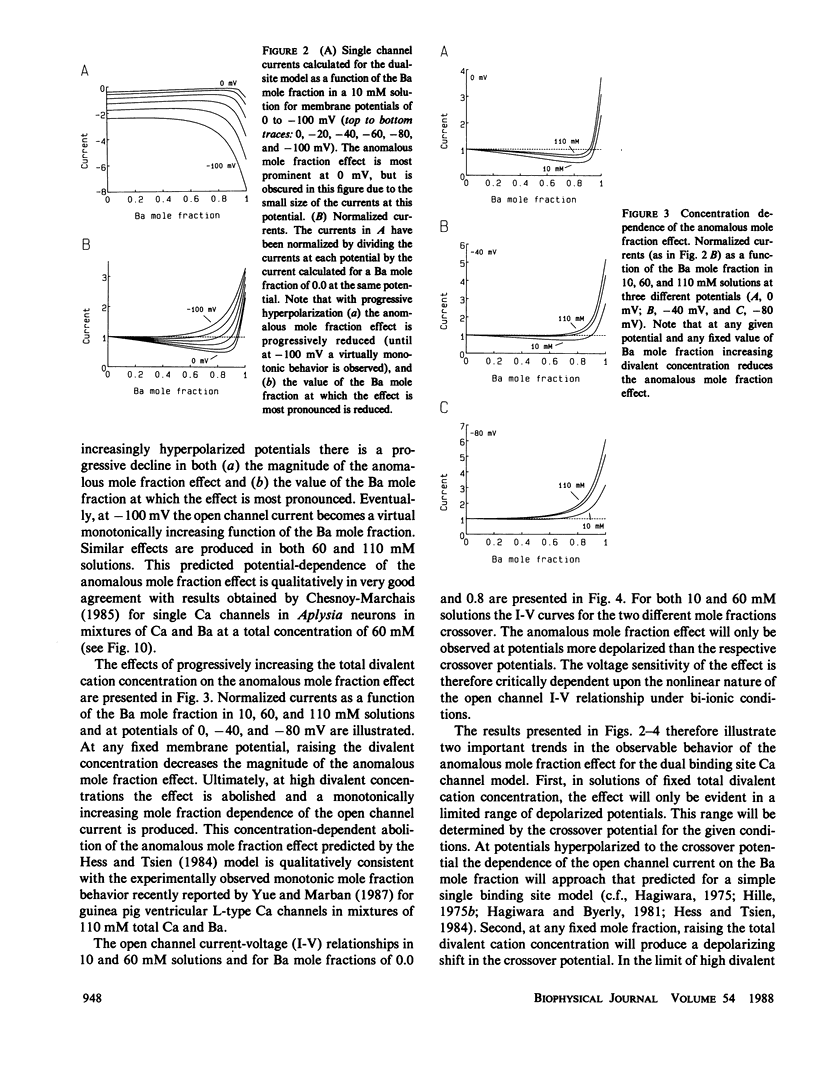
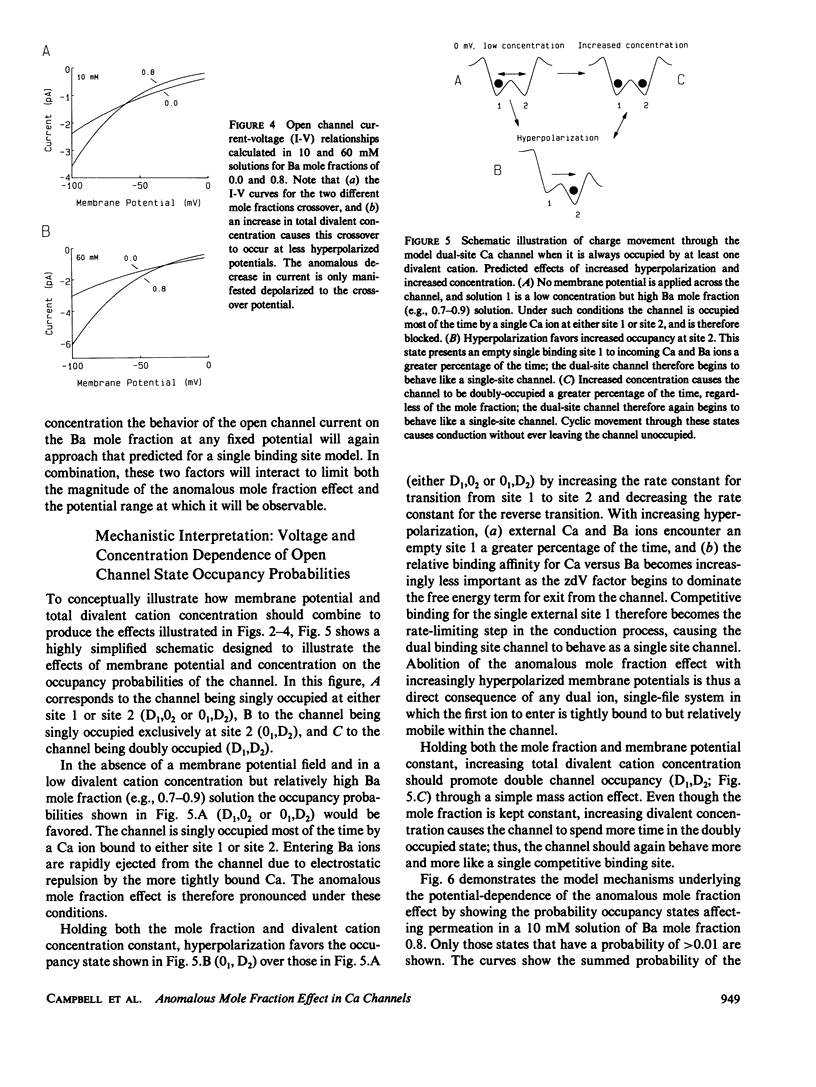
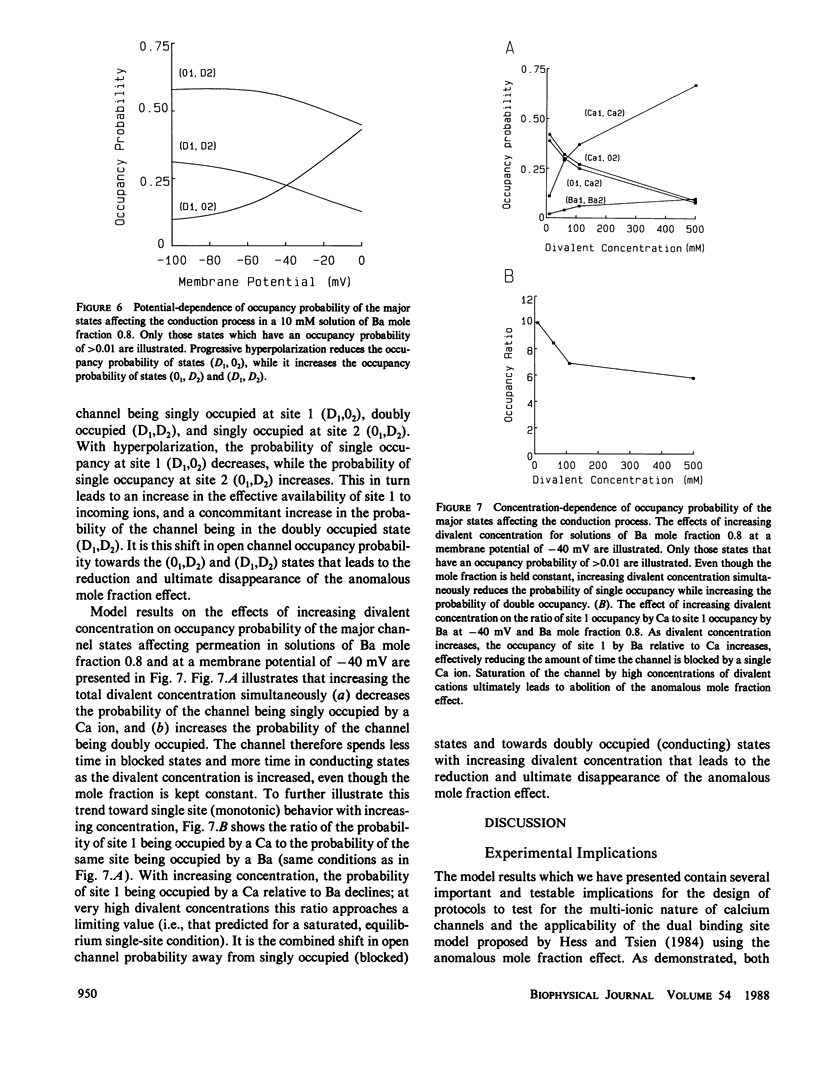
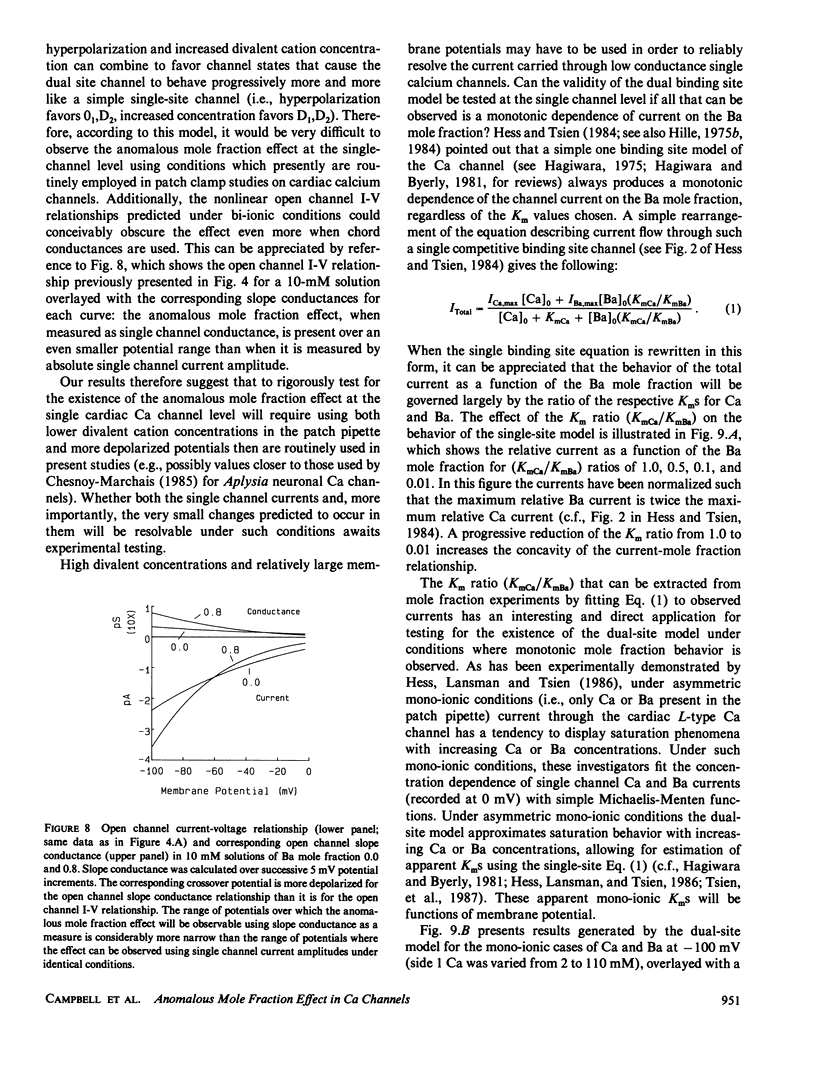
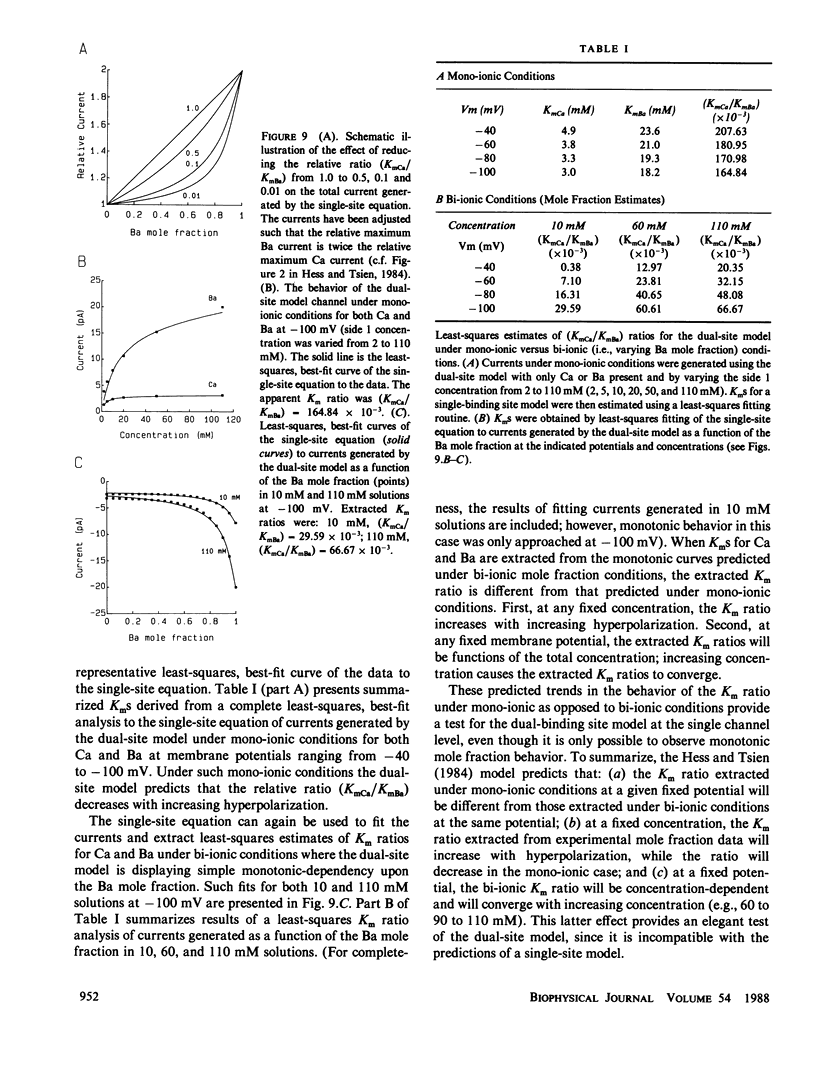
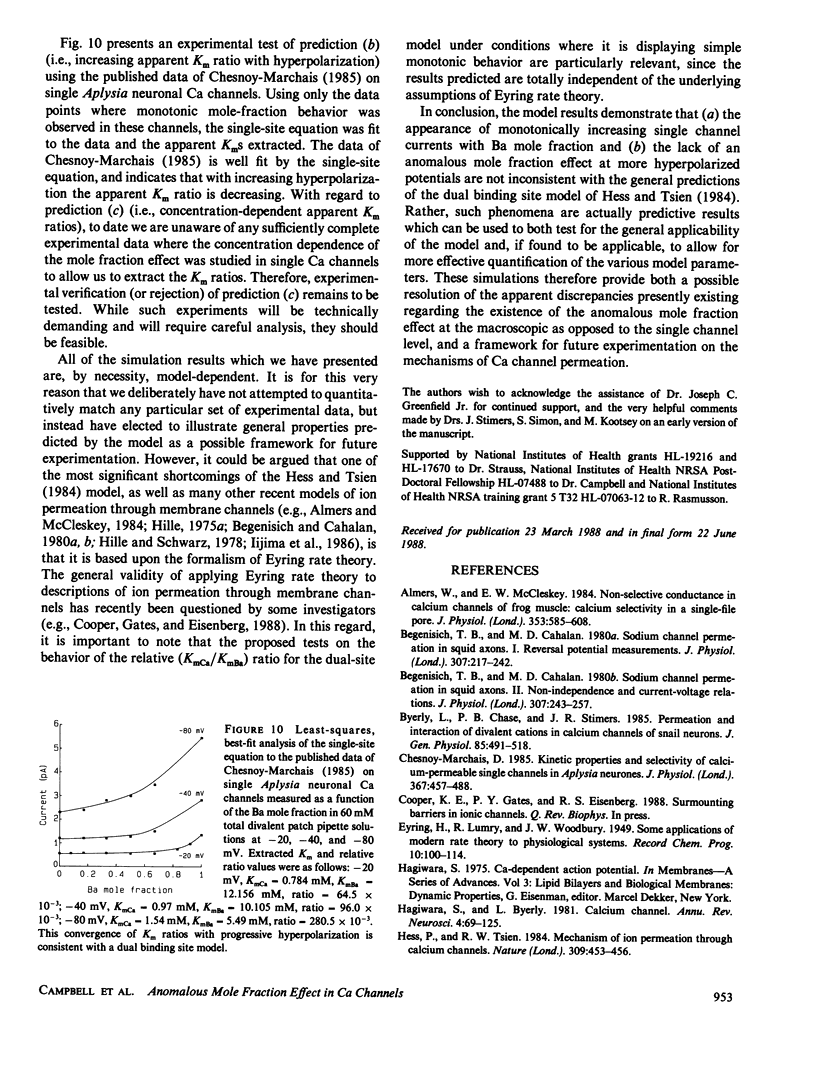
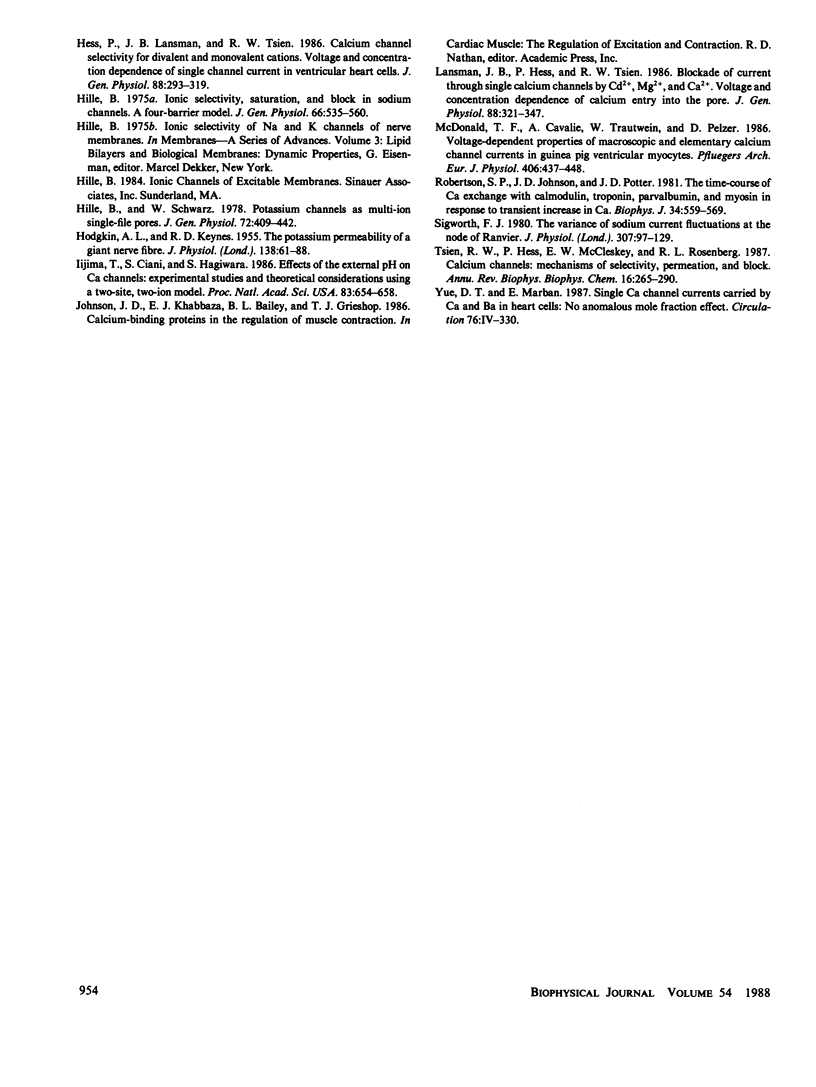
Selected References
These references are in PubMed. This may not be the complete list of references from this article.
- Almers W., McCleskey E. W. Non-selective conductance in calcium channels of frog muscle: calcium selectivity in a single-file pore. J Physiol. 1984 Aug;353:585–608. doi: 10.1113/jphysiol.1984.sp015352. [DOI] [PMC free article] [PubMed] [Google Scholar]
- Begenisich T. B., Cahalan M. D. Sodium channel permeation in squid axons. I: Reversal potential experiments. J Physiol. 1980 Oct;307:217–242. doi: 10.1113/jphysiol.1980.sp013432. [DOI] [PMC free article] [PubMed] [Google Scholar]
- Begenisich T. B., Cahalan M. D. Sodium channel permeation in squid axons. II: Non-independence and current-voltage relations. J Physiol. 1980 Oct;307:243–257. doi: 10.1113/jphysiol.1980.sp013433. [DOI] [PMC free article] [PubMed] [Google Scholar]
- Byerly L., Chase P. B., Stimers J. R. Permeation and interaction of divalent cations in calcium channels of snail neurons. J Gen Physiol. 1985 Apr;85(4):491–518. doi: 10.1085/jgp.85.4.491. [DOI] [PMC free article] [PubMed] [Google Scholar]
- Chesnoy-Marchais D. Kinetic properties and selectivity of calcium-permeable single channels in Aplysia neurones. J Physiol. 1985 Oct;367:457–488. doi: 10.1113/jphysiol.1985.sp015835. [DOI] [PMC free article] [PubMed] [Google Scholar]
- Grant J. D. Case of Former Chronic Suppuration with Epileptiform Attacks. Proc R Soc Med. 1912;5(OTOL):22–23. [PMC free article] [PubMed] [Google Scholar]
- HODGKIN A. L., KEYNES R. D. The potassium permeability of a giant nerve fibre. J Physiol. 1955 Apr 28;128(1):61–88. doi: 10.1113/jphysiol.1955.sp005291. [DOI] [PMC free article] [PubMed] [Google Scholar]
- Hagiwara S., Byerly L. Calcium channel. Annu Rev Neurosci. 1981;4:69–125. doi: 10.1146/annurev.ne.04.030181.000441. [DOI] [PubMed] [Google Scholar]
- Hess P., Lansman J. B., Tsien R. W. Calcium channel selectivity for divalent and monovalent cations. Voltage and concentration dependence of single channel current in ventricular heart cells. J Gen Physiol. 1986 Sep;88(3):293–319. doi: 10.1085/jgp.88.3.293. [DOI] [PMC free article] [PubMed] [Google Scholar]
- Hess P., Tsien R. W. Mechanism of ion permeation through calcium channels. 1984 May 31-Jun 6Nature. 309(5967):453–456. doi: 10.1038/309453a0. [DOI] [PubMed] [Google Scholar]
- Hille B. Ionic selectivity, saturation, and block in sodium channels. A four-barrier model. J Gen Physiol. 1975 Nov;66(5):535–560. doi: 10.1085/jgp.66.5.535. [DOI] [PMC free article] [PubMed] [Google Scholar]
- Hille B., Schwarz W. Potassium channels as multi-ion single-file pores. J Gen Physiol. 1978 Oct;72(4):409–442. doi: 10.1085/jgp.72.4.409. [DOI] [PMC free article] [PubMed] [Google Scholar]
- Iijima T., Ciani S., Hagiwara S. Effects of the external pH on Ca channels: experimental studies and theoretical considerations using a two-site, two-ion model. Proc Natl Acad Sci U S A. 1986 Feb;83(3):654–658. doi: 10.1073/pnas.83.3.654. [DOI] [PMC free article] [PubMed] [Google Scholar]
- Lansman J. B., Hess P., Tsien R. W. Blockade of current through single calcium channels by Cd2+, Mg2+, and Ca2+. Voltage and concentration dependence of calcium entry into the pore. J Gen Physiol. 1986 Sep;88(3):321–347. doi: 10.1085/jgp.88.3.321. [DOI] [PMC free article] [PubMed] [Google Scholar]
- McDonald T. F., Cavalié A., Trautwein W., Pelzer D. Voltage-dependent properties of macroscopic and elementary calcium channel currents in guinea pig ventricular myocytes. Pflugers Arch. 1986 May;406(5):437–448. doi: 10.1007/BF00583365. [DOI] [PubMed] [Google Scholar]
- Robertson S. P., Johnson J. D., Potter J. D. The time-course of Ca2+ exchange with calmodulin, troponin, parvalbumin, and myosin in response to transient increases in Ca2+. Biophys J. 1981 Jun;34(3):559–569. doi: 10.1016/S0006-3495(81)84868-0. [DOI] [PMC free article] [PubMed] [Google Scholar]
- Sigworth F. J. The variance of sodium current fluctuations at the node of Ranvier. J Physiol. 1980 Oct;307:97–129. doi: 10.1113/jphysiol.1980.sp013426. [DOI] [PMC free article] [PubMed] [Google Scholar]
- Tsien R. W., Hess P., McCleskey E. W., Rosenberg R. L. Calcium channels: mechanisms of selectivity, permeation, and block. Annu Rev Biophys Biophys Chem. 1987;16:265–290. doi: 10.1146/annurev.bb.16.060187.001405. [DOI] [PubMed] [Google Scholar]