Abstract
The molecular basis for the elasticity of the human erythrocyte membrane was explored. Skeletons were released from ghosts in Triton X-100 and their dimensions followed by dark-field microscopy and packed volume. The rest size of skeletons was assumed to reflect the balance point between expansion (deformation) driven by electrostatic repulsions among the excess of fixed negative charges on the proteins and contraction (recovery) driven by their elasticity. The size of skeletons decreased with increasing temperature. This finding suggests that entropy drives elasticity. The requisite entropy change could be associated with either the configurational freedom of flexible protein chains or with the solvation of side chains exposed during protein dissociation (hydrophobic effects). To distinguish between these two alternatives, we tested the impact of two weak denaturants, 10% ethanol and 20 nM lithium 3,5-diiodosalicylate. Both agents reversibly promoted the expansion of skeletons, presumably by reducing their elasticity. Since the conformation of random coils and globular proteins should not be significantly altered by these mild treatments, this finding strongly suggests a role for weak interdomain and/or interprotein associations. We conclude that the elasticity of the red cell membrane skeleton may not derive from the configurational entropy of flexible coils. Rather, the elastic energy may arise from reversible dissociations of weak but specific intramolecular and/or intermolecular contacts, presumably within deformed spectrin filaments.
Full text
PDF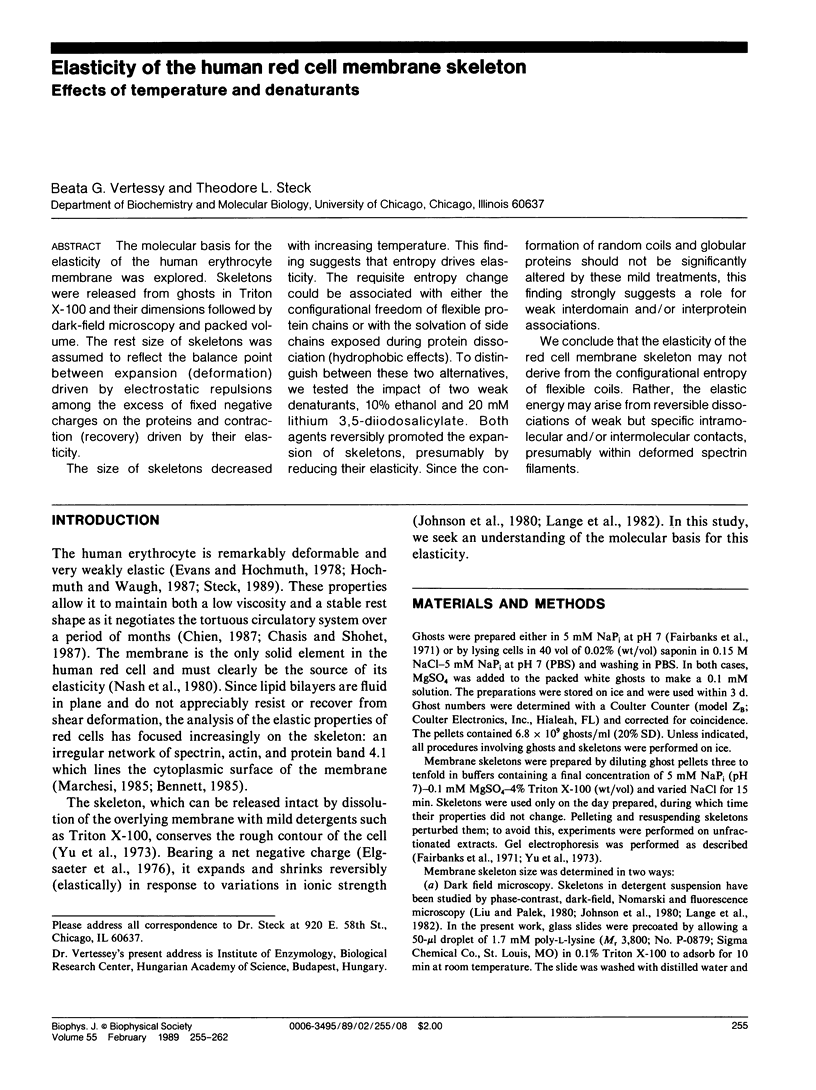
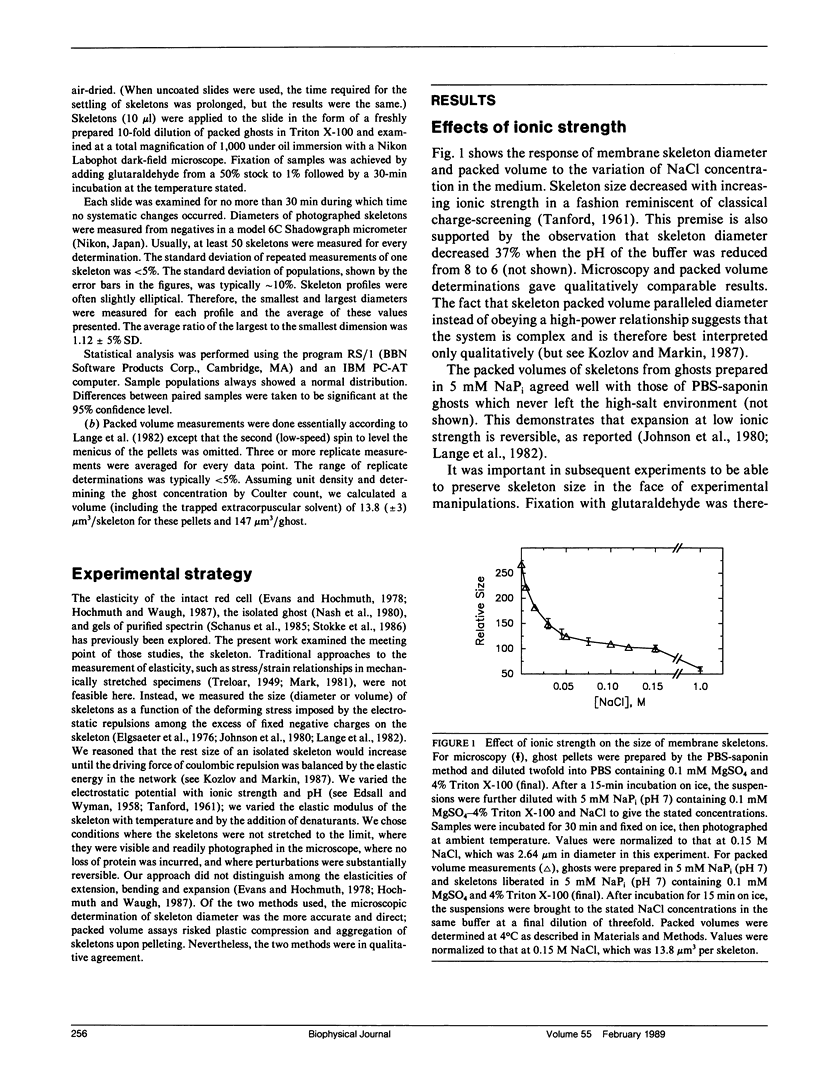
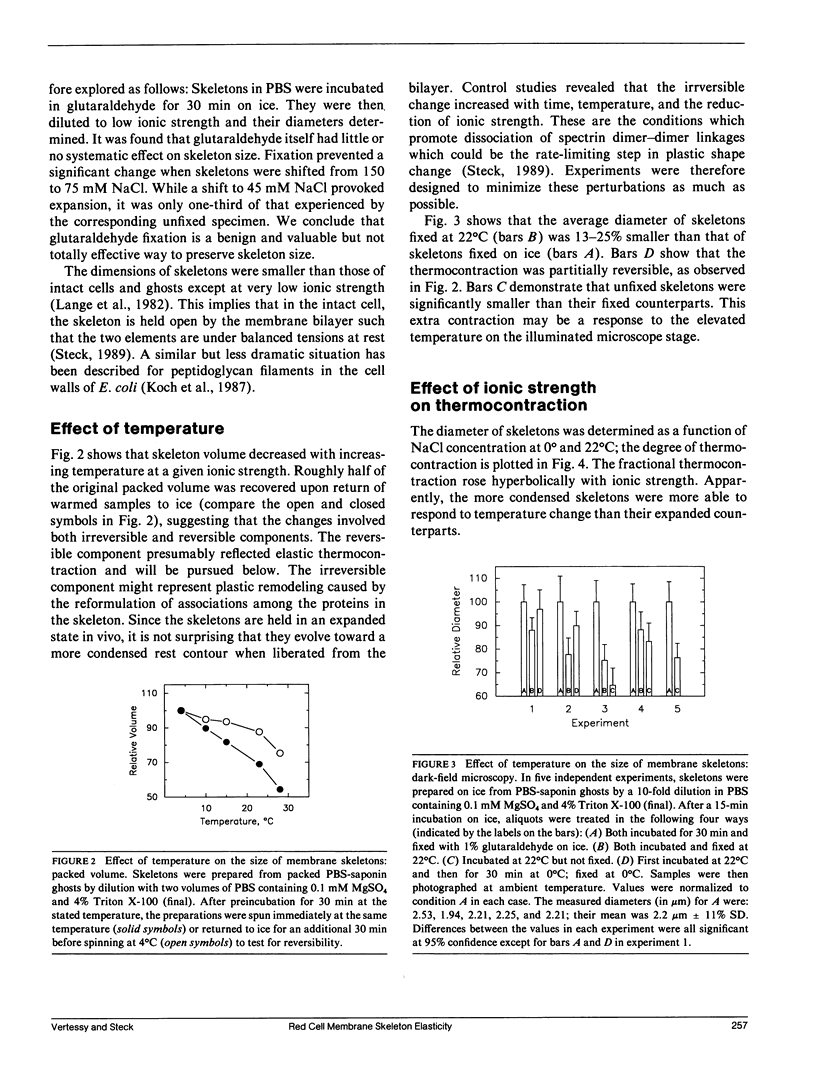
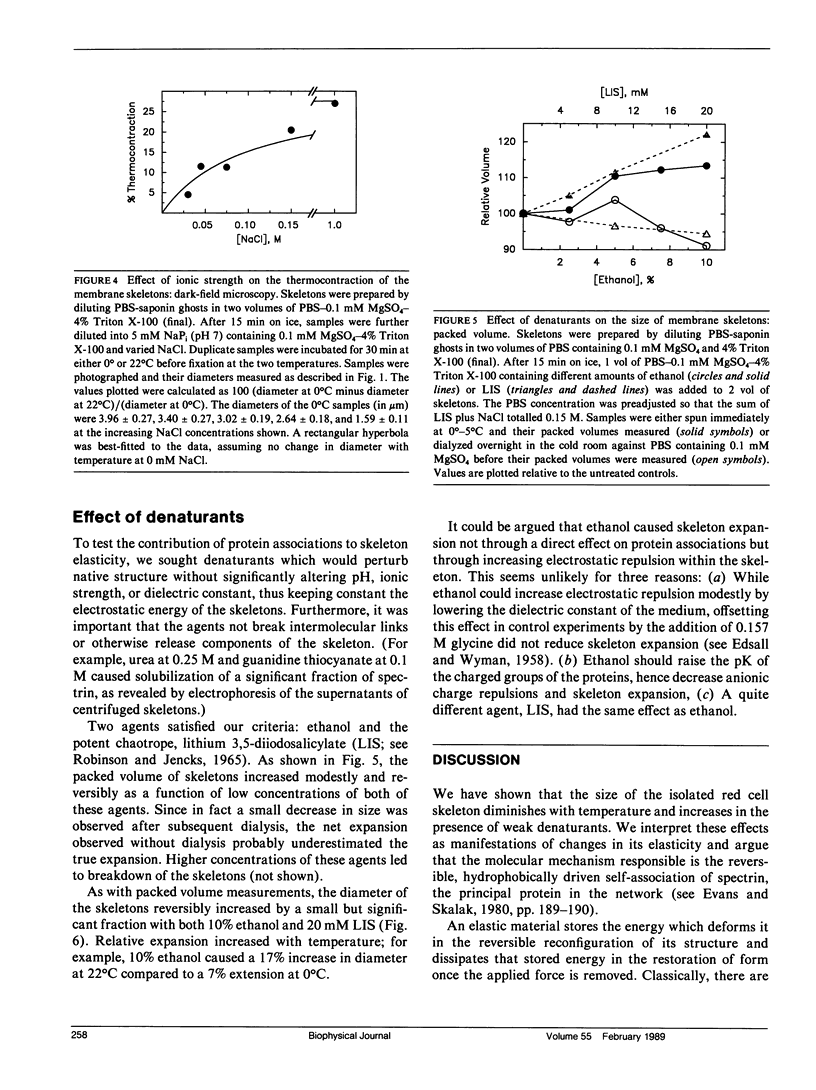
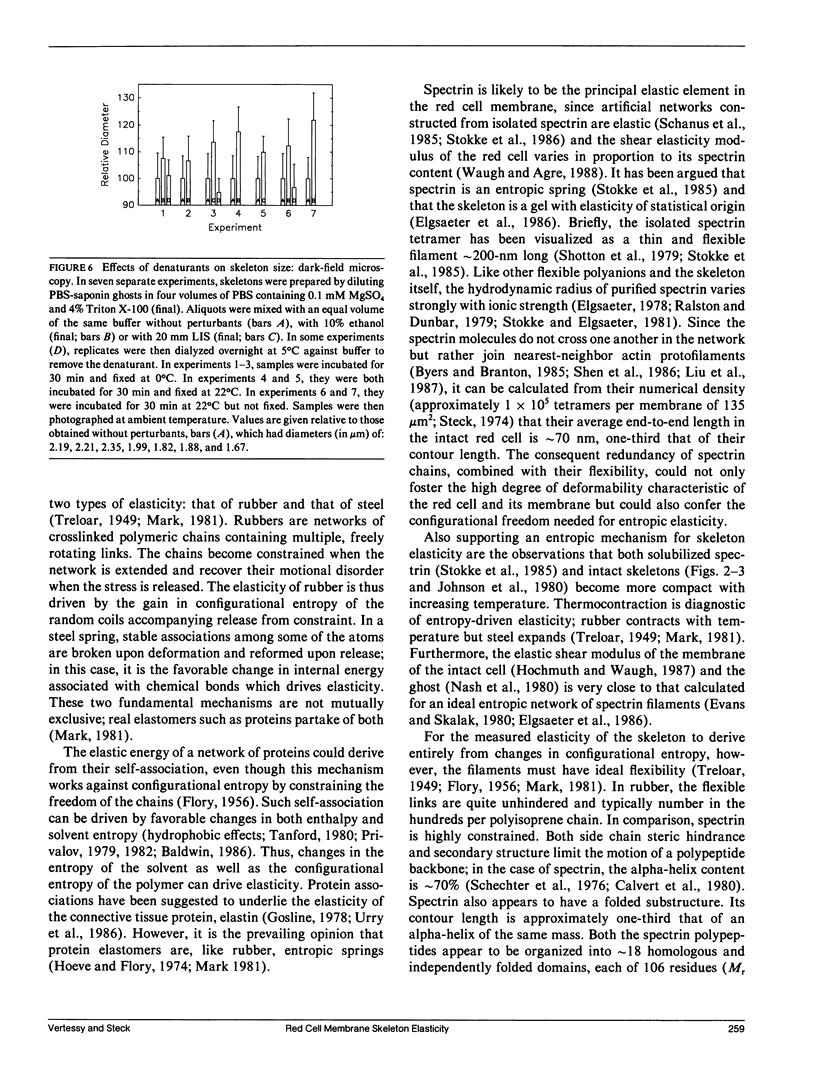
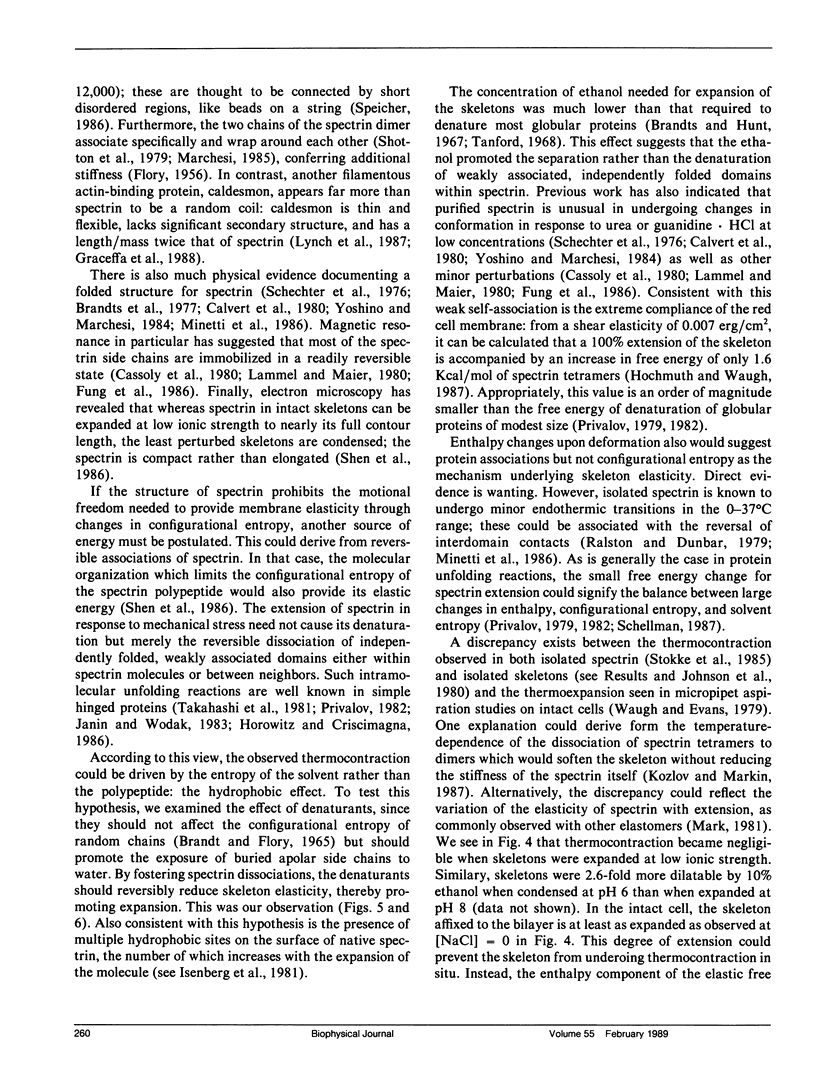
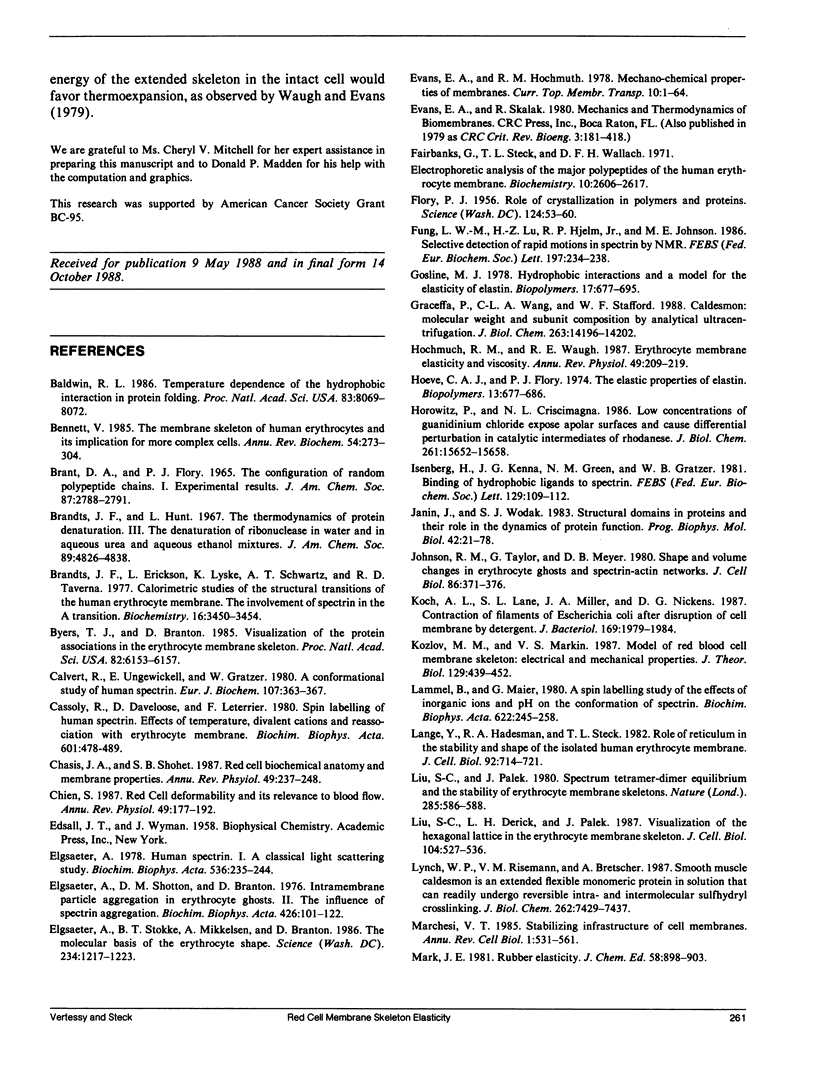
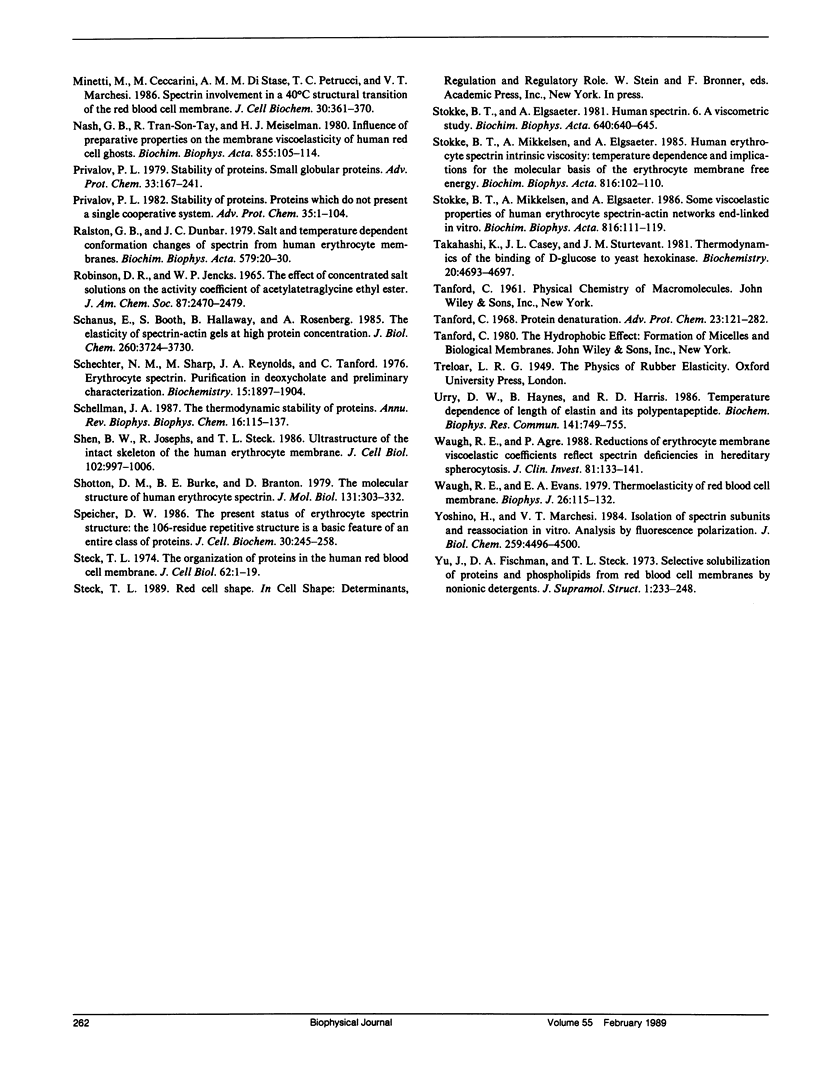
Selected References
These references are in PubMed. This may not be the complete list of references from this article.
- Baldwin R. L. Temperature dependence of the hydrophobic interaction in protein folding. Proc Natl Acad Sci U S A. 1986 Nov;83(21):8069–8072. doi: 10.1073/pnas.83.21.8069. [DOI] [PMC free article] [PubMed] [Google Scholar]
- Bennett V. The membrane skeleton of human erythrocytes and its implications for more complex cells. Annu Rev Biochem. 1985;54:273–304. doi: 10.1146/annurev.bi.54.070185.001421. [DOI] [PubMed] [Google Scholar]
- Brandts J. F., Erickson L., Lysko K., Schwartz A. T., Taverna R. D. Calorimetric studies of the structural transitions of the human erythrocyte membrane. The involvement of spectrin in the A transition. Biochemistry. 1977 Jul 26;16(15):3450–3454. doi: 10.1021/bi00634a024. [DOI] [PubMed] [Google Scholar]
- Brandts J. F., Hunt L. The thermodynamics of protein denaturation. 3. The denaturation of ribonuclease in water and in aqueous urea and aqueous ethanol mixtures. J Am Chem Soc. 1967 Sep 13;89(19):4826–4838. doi: 10.1021/ja00995a002. [DOI] [PubMed] [Google Scholar]
- Byers T. J., Branton D. Visualization of the protein associations in the erythrocyte membrane skeleton. Proc Natl Acad Sci U S A. 1985 Sep;82(18):6153–6157. doi: 10.1073/pnas.82.18.6153. [DOI] [PMC free article] [PubMed] [Google Scholar]
- Calvert R., Ungewickell E., Gratzer W. A conformational study of human spectrin. Eur J Biochem. 1980 Jun;107(2):363–367. doi: 10.1111/j.1432-1033.1980.tb06037.x. [DOI] [PubMed] [Google Scholar]
- Cassoly R., Daveloose D., Leterrier F. Spin labeling of human spectrin. Effects of temperature, divalent cations and reassociation with erythrocyte membrane. Biochim Biophys Acta. 1980 Oct 2;601(3):478–489. doi: 10.1016/0005-2736(80)90551-9. [DOI] [PubMed] [Google Scholar]
- Chasis J. A., Shohet S. B. Red cell biochemical anatomy and membrane properties. Annu Rev Physiol. 1987;49:237–248. doi: 10.1146/annurev.ph.49.030187.001321. [DOI] [PubMed] [Google Scholar]
- Chien S. Red cell deformability and its relevance to blood flow. Annu Rev Physiol. 1987;49:177–192. doi: 10.1146/annurev.ph.49.030187.001141. [DOI] [PubMed] [Google Scholar]
- Elgsaeter A. Human spectrin. I. A classical light scattering study. Biochim Biophys Acta. 1978 Sep 26;536(1):235–244. doi: 10.1016/0005-2795(78)90069-7. [DOI] [PubMed] [Google Scholar]
- Elgsaeter A., Shotton D. M., Branton D. Intramembrane particle aggregation in erythrocyte ghosts. II. The influence of spectrin aggregation. Biochim Biophys Acta. 1976 Feb 19;426(1):101–122. doi: 10.1016/0005-2736(76)90433-8. [DOI] [PubMed] [Google Scholar]
- Elgsaeter A., Stokke B. T., Mikkelsen A., Branton D. The molecular basis of erythrocyte shape. Science. 1986 Dec 5;234(4781):1217–1223. doi: 10.1126/science.3775380. [DOI] [PubMed] [Google Scholar]
- Evans E. A., Skalak R. Mechanics and thermodynamics of biomembranes: part 2. CRC Crit Rev Bioeng. 1979 Nov;3(4):331–418. [PubMed] [Google Scholar]
- Fairbanks G., Steck T. L., Wallach D. F. Electrophoretic analysis of the major polypeptides of the human erythrocyte membrane. Biochemistry. 1971 Jun 22;10(13):2606–2617. doi: 10.1021/bi00789a030. [DOI] [PubMed] [Google Scholar]
- Flory P. J. Role of Crystallization in Polymers and Proteins. Science. 1956 Jul 13;124(3211):53–60. doi: 10.1126/science.124.3211.53. [DOI] [PubMed] [Google Scholar]
- Fung L. W., Lu H. Z., Hjelm R. P., Jr, Johnson M. E. Selective detection of rapid motions in spectrin by NMR. FEBS Lett. 1986 Mar 3;197(1-2):234–238. doi: 10.1016/0014-5793(86)80333-7. [DOI] [PubMed] [Google Scholar]
- Gosline J. M. Hydrophobic interaction and a model for the elasticity of elastin. Biopolymers. 1978 Mar;17(3):677–695. doi: 10.1002/bip.1978.360170311. [DOI] [PubMed] [Google Scholar]
- Graceffa P., Wang C. L., Stafford W. F. Caldesmon. Molecular weight and subunit composition by analytical ultracentrifugation. J Biol Chem. 1988 Oct 5;263(28):14196–14202. [PubMed] [Google Scholar]
- Hochmuth R. M., Waugh R. E. Erythrocyte membrane elasticity and viscosity. Annu Rev Physiol. 1987;49:209–219. doi: 10.1146/annurev.ph.49.030187.001233. [DOI] [PubMed] [Google Scholar]
- Hoeve C. A., Flory P. J. The elastic properties of elastin. Biopolymers. 1974 Apr;13(4):677–686. doi: 10.1002/bip.1974.360130404. [DOI] [PubMed] [Google Scholar]
- Horowitz P., Criscimagna N. L. Low concentrations of guanidinium chloride expose apolar surfaces and cause differential perturbation in catalytic intermediates of rhodanese. J Biol Chem. 1986 Nov 25;261(33):15652–15658. [PubMed] [Google Scholar]
- Isenberg H., Kenna J. G., Green N. M., Gratzer W. B. Binding of hydrophobic ligands to spectrin. FEBS Lett. 1981 Jun 29;129(1):109–112. doi: 10.1016/0014-5793(81)80767-3. [DOI] [PubMed] [Google Scholar]
- Janin J., Wodak S. J. Structural domains in proteins and their role in the dynamics of protein function. Prog Biophys Mol Biol. 1983;42(1):21–78. doi: 10.1016/0079-6107(83)90003-2. [DOI] [PubMed] [Google Scholar]
- Johnson R. M., Taylor G., Meyer D. B. Shape and volume changes in erythrocyte ghosts and spectrin-actin networks. J Cell Biol. 1980 Aug;86(2):371–376. doi: 10.1083/jcb.86.2.371. [DOI] [PMC free article] [PubMed] [Google Scholar]
- Koch A. L., Lane S. L., Miller J. A., Nickens D. G. Contraction of filaments of Escherichia coli after disruption of cell membrane by detergent. J Bacteriol. 1987 May;169(5):1979–1984. doi: 10.1128/jb.169.5.1979-1984.1987. [DOI] [PMC free article] [PubMed] [Google Scholar]
- Kozlov M. M., Markin V. S. Model of red blood cell membrane skeleton: electrical and mechanical properties. J Theor Biol. 1987 Dec 21;129(4):439–452. doi: 10.1016/s0022-5193(87)80023-1. [DOI] [PubMed] [Google Scholar]
- Lammel B., Maier G. A spin labeling study of the effects of inorganic ions and pH on the conformation of spectrin. Biochim Biophys Acta. 1980 Apr 25;622(2):245–258. doi: 10.1016/0005-2795(80)90035-5. [DOI] [PubMed] [Google Scholar]
- Lange Y., Hadesman R. A., Steck T. L. Role of the reticulum in the stability and shape of the isolated human erythrocyte membrane. J Cell Biol. 1982 Mar;92(3):714–721. doi: 10.1083/jcb.92.3.714. [DOI] [PMC free article] [PubMed] [Google Scholar]
- Liu S. C., Derick L. H., Palek J. Visualization of the hexagonal lattice in the erythrocyte membrane skeleton. J Cell Biol. 1987 Mar;104(3):527–536. doi: 10.1083/jcb.104.3.527. [DOI] [PMC free article] [PubMed] [Google Scholar]
- Liu S. C., Palek J. Spectrin tetramer-dimer equilibrium and the stability of erythrocyte membrane skeletons. Nature. 1980 Jun 19;285(5766):586–588. doi: 10.1038/285586a0. [DOI] [PubMed] [Google Scholar]
- Lynch W. P., Riseman V. M., Bretscher A. Smooth muscle caldesmon is an extended flexible monomeric protein in solution that can readily undergo reversible intra- and intermolecular sulfhydryl cross-linking. A mechanism for caldesmon's F-actin bundling activity. J Biol Chem. 1987 May 25;262(15):7429–7437. [PubMed] [Google Scholar]
- Marchesi V. T. Stabilizing infrastructure of cell membranes. Annu Rev Cell Biol. 1985;1:531–561. doi: 10.1146/annurev.cb.01.110185.002531. [DOI] [PubMed] [Google Scholar]
- Minetti M., Ceccarini M., Di Stasi A. M., Petrucci T. C., Marchesi V. T. Spectrin involvement in a 40 degrees C structural transition of the red blood cell membrane. J Cell Biochem. 1986;30(4):361–370. doi: 10.1002/jcb.240300409. [DOI] [PubMed] [Google Scholar]
- Nash G. B., Tran-Son-Tay R., Meiselman H. J. Influence of preparative procedures on the membrane viscoelasticity of human red cell ghosts. Biochim Biophys Acta. 1986 Feb 13;855(1):105–114. doi: 10.1016/0005-2736(86)90194-x. [DOI] [PubMed] [Google Scholar]
- Privalov P. L. Stability of proteins. Proteins which do not present a single cooperative system. Adv Protein Chem. 1982;35:1–104. [PubMed] [Google Scholar]
- Privalov P. L. Stability of proteins: small globular proteins. Adv Protein Chem. 1979;33:167–241. doi: 10.1016/s0065-3233(08)60460-x. [DOI] [PubMed] [Google Scholar]
- ROBINSON D. R., JENCKS W. P. THE EFFECT OF CONCENTRATED SALT SOLUTIONS ON THE ACTIVITY COEFFICIENT OF ACETYLTETRAGLYCINE ETHYL ESTER. J Am Chem Soc. 1965 Jun 5;87:2470–2479. doi: 10.1021/ja01089a029. [DOI] [PubMed] [Google Scholar]
- Ralston G. B., Dunbar J. C. Salt and temperature-dependent conformation changes in spectrin from human erythrocyte membranes. Biochim Biophys Acta. 1979 Jul 25;579(1):20–30. doi: 10.1016/0005-2795(79)90083-7. [DOI] [PubMed] [Google Scholar]
- Schanus E., Booth S., Hallaway B., Rosenberg A. The elasticity of spectrin-actin gels at high protein concentration. J Biol Chem. 1985 Mar 25;260(6):3724–3730. [PubMed] [Google Scholar]
- Schechter N. M., Sharp M., Reynolds J. A., Tanford C. Erythrocyte spectrin. Purification in deoxycholate and preliminary characterization. Biochemistry. 1976 May 4;15(9):1897–1904. doi: 10.1021/bi00654a016. [DOI] [PubMed] [Google Scholar]
- Schellman J. A. The thermodynamic stability of proteins. Annu Rev Biophys Biophys Chem. 1987;16:115–137. doi: 10.1146/annurev.bb.16.060187.000555. [DOI] [PubMed] [Google Scholar]
- Shen B. W., Josephs R., Steck T. L. Ultrastructure of the intact skeleton of the human erythrocyte membrane. J Cell Biol. 1986 Mar;102(3):997–1006. doi: 10.1083/jcb.102.3.997. [DOI] [PMC free article] [PubMed] [Google Scholar]
- Shotton D. M., Burke B. E., Branton D. The molecular structure of human erythrocyte spectrin. Biophysical and electron microscopic studies. J Mol Biol. 1979 Jun 25;131(2):303–329. doi: 10.1016/0022-2836(79)90078-0. [DOI] [PubMed] [Google Scholar]
- Speicher D. W. The present status of erythrocyte spectrin structure: the 106-residue repetitive structure is a basic feature of an entire class of proteins. J Cell Biochem. 1986;30(3):245–258. doi: 10.1002/jcb.240300306. [DOI] [PubMed] [Google Scholar]
- Steck T. L. The organization of proteins in the human red blood cell membrane. A review. J Cell Biol. 1974 Jul;62(1):1–19. doi: 10.1083/jcb.62.1.1. [DOI] [PMC free article] [PubMed] [Google Scholar]
- Stokke B. T., Elgsaeter A. Human spectrin. VI. A viscometric study. Biochim Biophys Acta. 1981 Feb 6;640(3):640–645. doi: 10.1016/0005-2736(81)90094-8. [DOI] [PubMed] [Google Scholar]
- Stokke B. T., Mikkelsen A., Elgsaeter A. Human erythrocyte spectrin dimer intrinsic viscosity: temperature dependence and implications for the molecular basis of the erythrocyte membrane free energy. Biochim Biophys Acta. 1985 Jun 11;816(1):102–110. doi: 10.1016/0005-2736(85)90398-0. [DOI] [PubMed] [Google Scholar]
- Stokke B. T., Mikkelsen A., Elgsaeter A. Some viscoelastic properties of human erythrocyte spectrin networks end-linked in vitro. Biochim Biophys Acta. 1985 Jun 11;816(1):111–121. doi: 10.1016/0005-2736(85)90399-2. [DOI] [PubMed] [Google Scholar]
- Takahashi K., Casey J. L., Sturtevant J. M. Thermodynamics of the binding of D-glucose to yeast hexokinase. Biochemistry. 1981 Aug 4;20(16):4693–4697. doi: 10.1021/bi00519a026. [DOI] [PubMed] [Google Scholar]
- Tanford C. Protein denaturation. Adv Protein Chem. 1968;23:121–282. doi: 10.1016/s0065-3233(08)60401-5. [DOI] [PubMed] [Google Scholar]
- Urry D. W., Haynes B., Harris R. D. Temperature dependence of length of elastin and its polypentapeptide. Biochem Biophys Res Commun. 1986 Dec 15;141(2):749–755. doi: 10.1016/s0006-291x(86)80236-4. [DOI] [PubMed] [Google Scholar]
- Waugh R. E., Agre P. Reductions of erythrocyte membrane viscoelastic coefficients reflect spectrin deficiencies in hereditary spherocytosis. J Clin Invest. 1988 Jan;81(1):133–141. doi: 10.1172/JCI113284. [DOI] [PMC free article] [PubMed] [Google Scholar]
- Waugh R., Evans E. A. Thermoelasticity of red blood cell membrane. Biophys J. 1979 Apr;26(1):115–131. doi: 10.1016/S0006-3495(79)85239-X. [DOI] [PMC free article] [PubMed] [Google Scholar]
- Yoshino H., Marchesi V. T. Isolation of spectrin subunits and reassociation in vitro. Analysis by fluorescence polarization. J Biol Chem. 1984 Apr 10;259(7):4496–4500. [PubMed] [Google Scholar]
- Yu J., Fischman D. A., Steck T. L. Selective solubilization of proteins and phospholipids from red blood cell membranes by nonionic detergents. J Supramol Struct. 1973;1(3):233–248. doi: 10.1002/jss.400010308. [DOI] [PubMed] [Google Scholar]