Abstract
We investigated the interaction of tetanus toxin with small unilamellar vesicles composed of different phospholipids as a function of pH, toxin concentration, temperature, and ionic strength of the solution. Tetanus toxin increased the permeability of the vesicles to fluorescent markers of molecular weight up to 700. The time course of the permeabilization was described as the sum of two exponential components of which the faster accounts for more than 70% of the total effect. Both time constants decreased when the pH of the solution was lowered and when vesicles contained negative lipids. These results can be explained in terms of a phenomenological model based on reaction rate theory. The model assumes that tetanus toxin, after equilibrating with the local pH existing at the surface of the vesicles, inserts into the lipid bilayer forming an ionic channel through which solutes can diffuse. Trigger event for the insertion of the toxin is the protonation, and consequent neutralization of one charged group which makes the molecule more hydrophobic. The intrinsic pK of this group was found to be 3.4 +/- 0.2, suggesting that it may be a carboxyl group. Since the toxin equilibrates with the local pH, the enhancing effect of acidic phospholipids is merely explained by the creation of a negative surface potential which increases the local proton concentration. This was confirmed by the inhibitory effect of high Na+ concentration which reduced the surface charge by screening and specific binding. We found still small differences between the lipids tested and the following order of sensitivity to the action of the toxin: phosphatidylinositol greater than phosphatidylserine greater than phosphatidylcholine approximately cholesterol. The activation energy for the two time constants was found to be 19.8 and 14.8 kcal/mol, fast and slow component, respectively, i.e., slightly larger than that for pure diffusion through the bilayer. The permeabilization induced by tetanus toxin is a voltage-dependent process because vesicles bearing an inner negative potential were depolarized very quickly whereas those bearing an inner positive voltage were barely depolarized at all.
Full text
PDF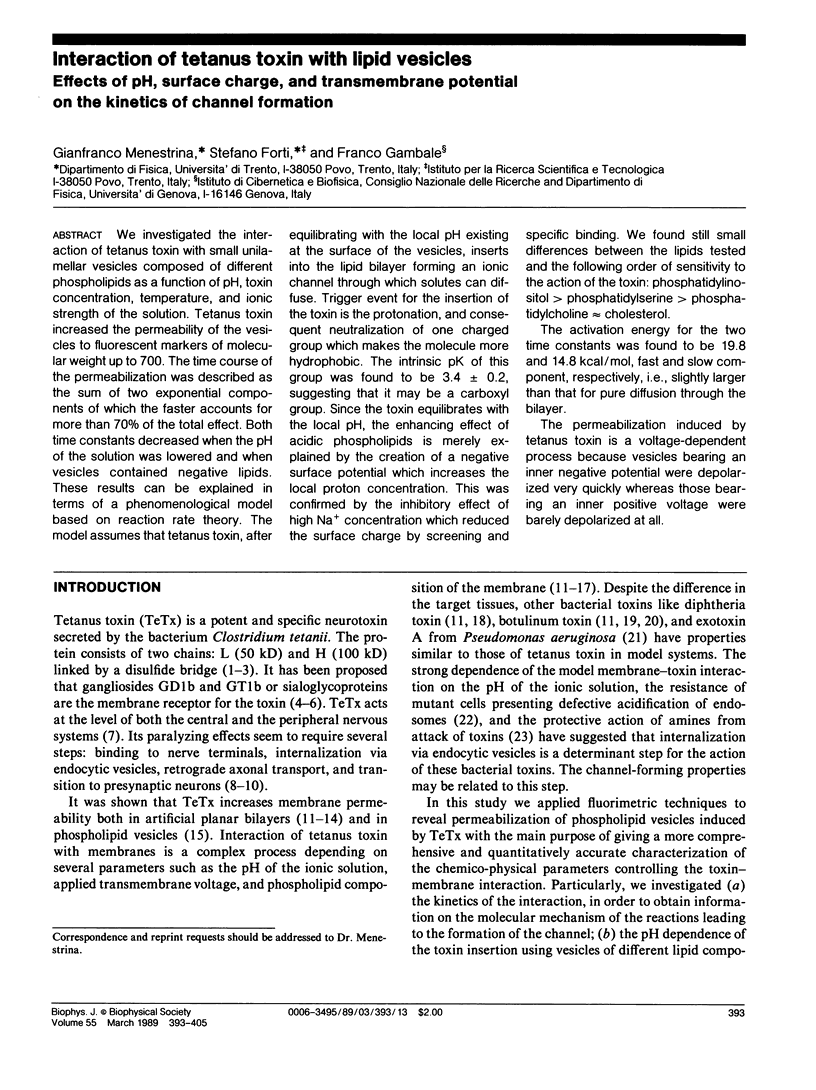
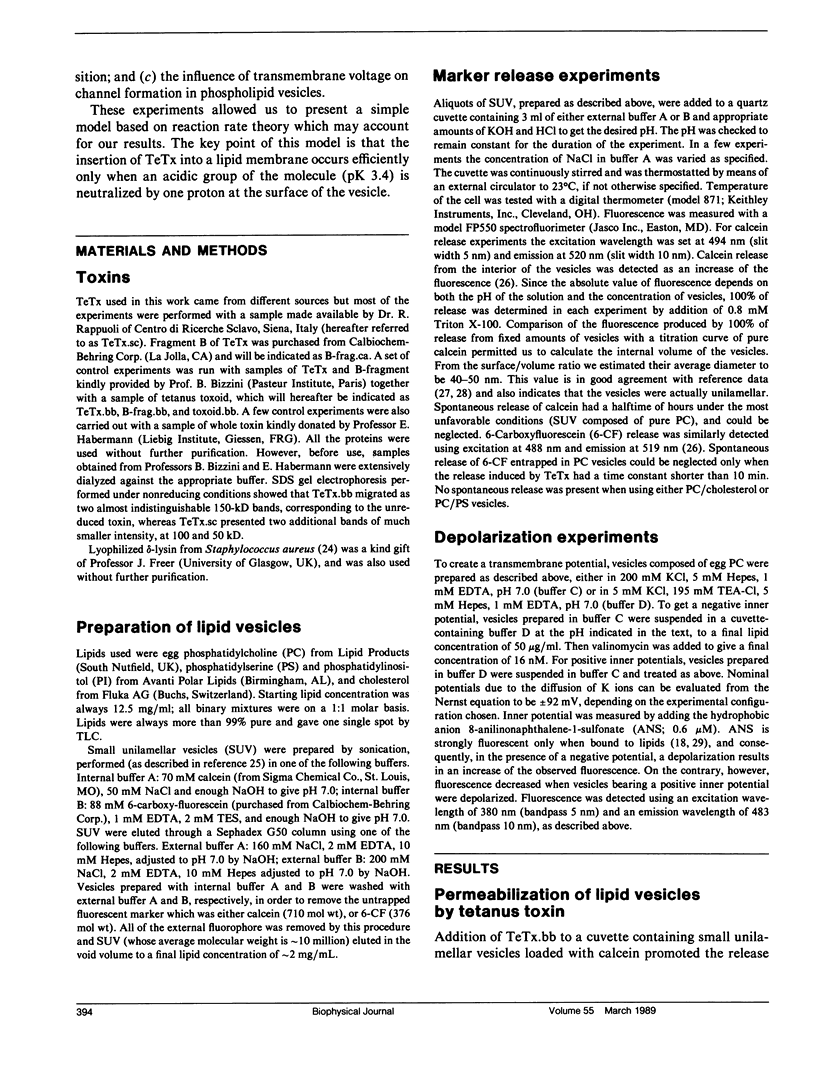
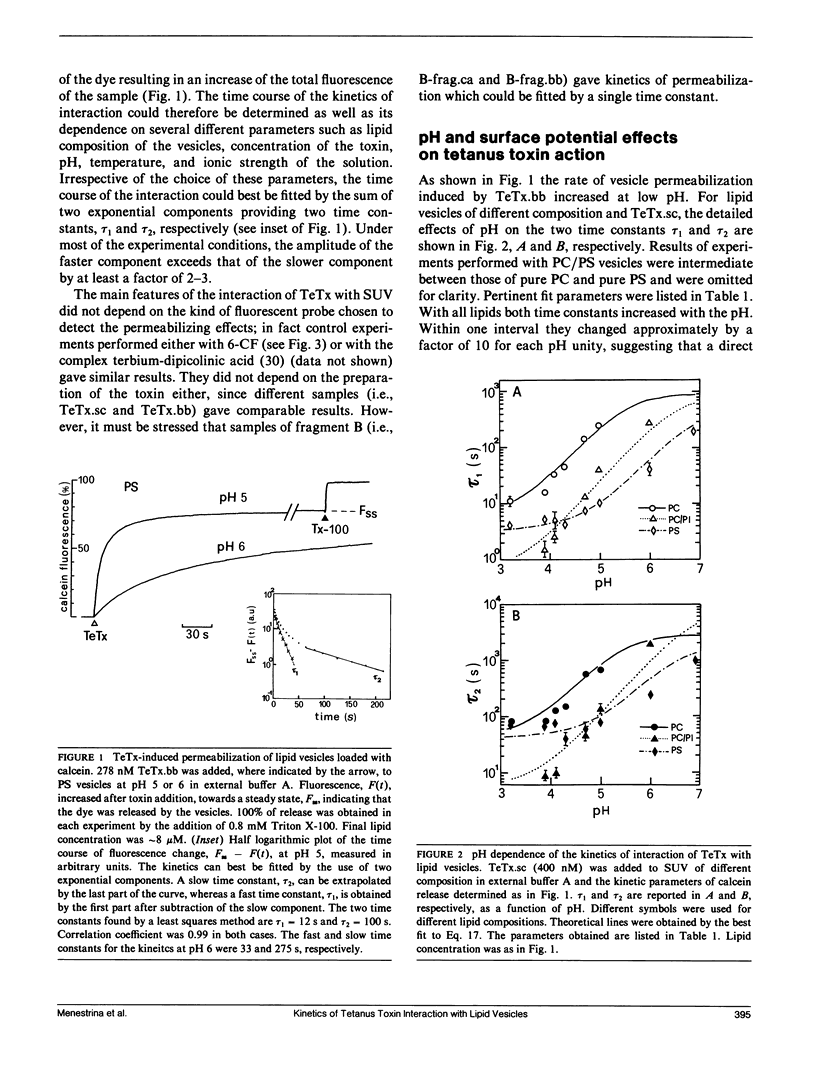
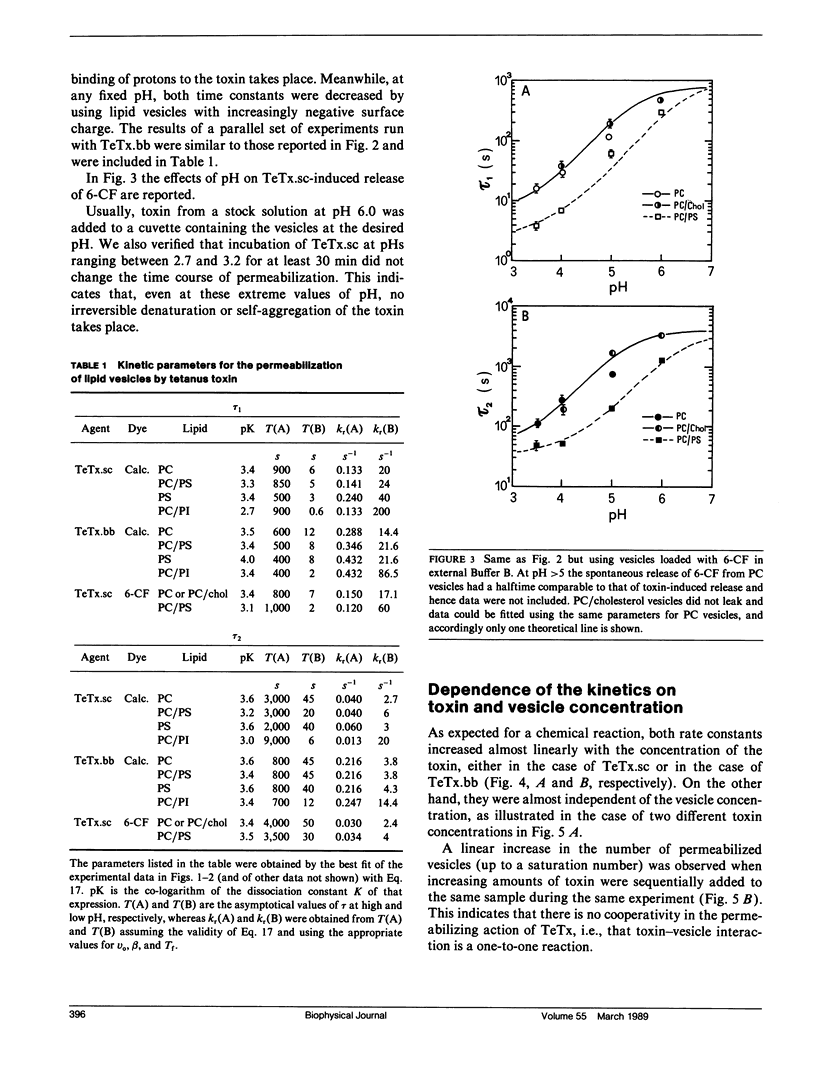
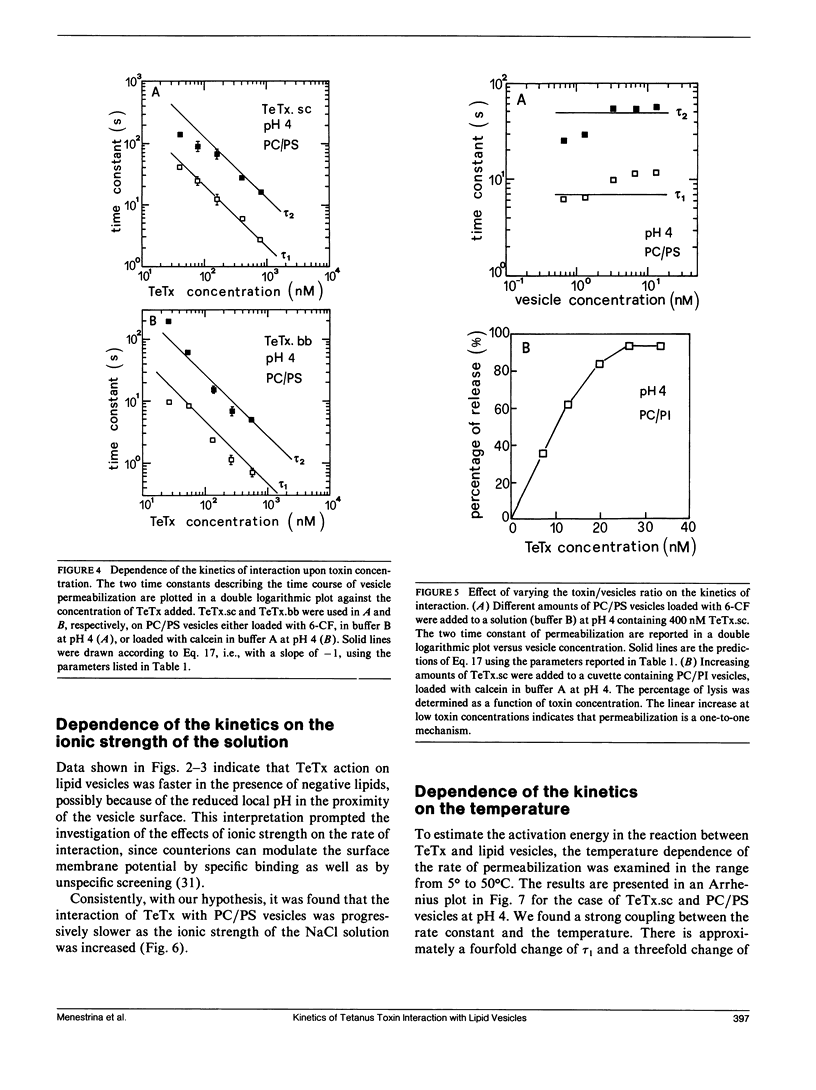
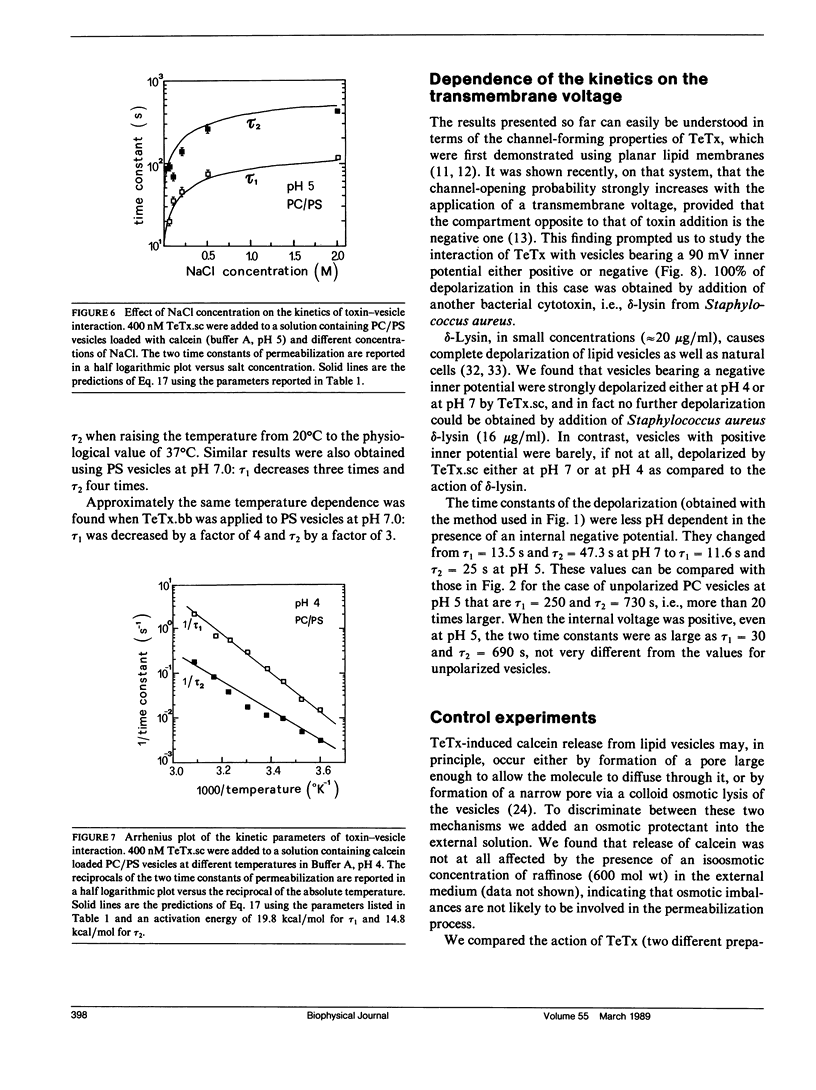
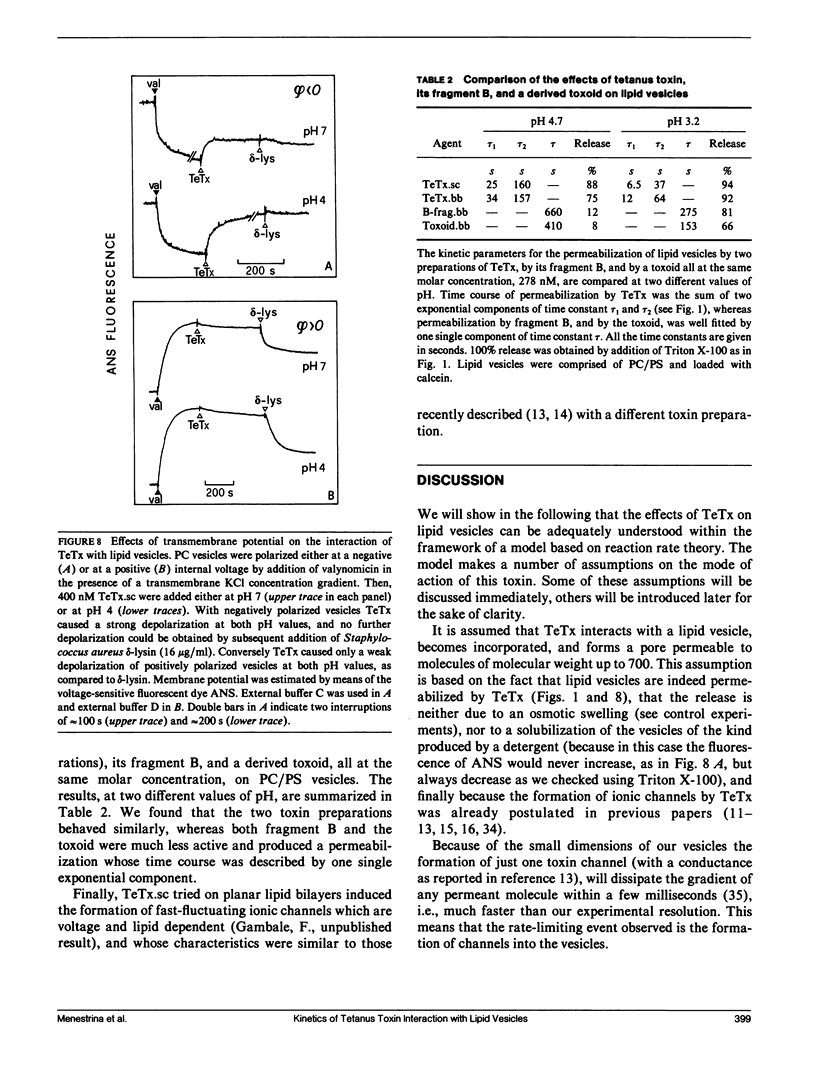
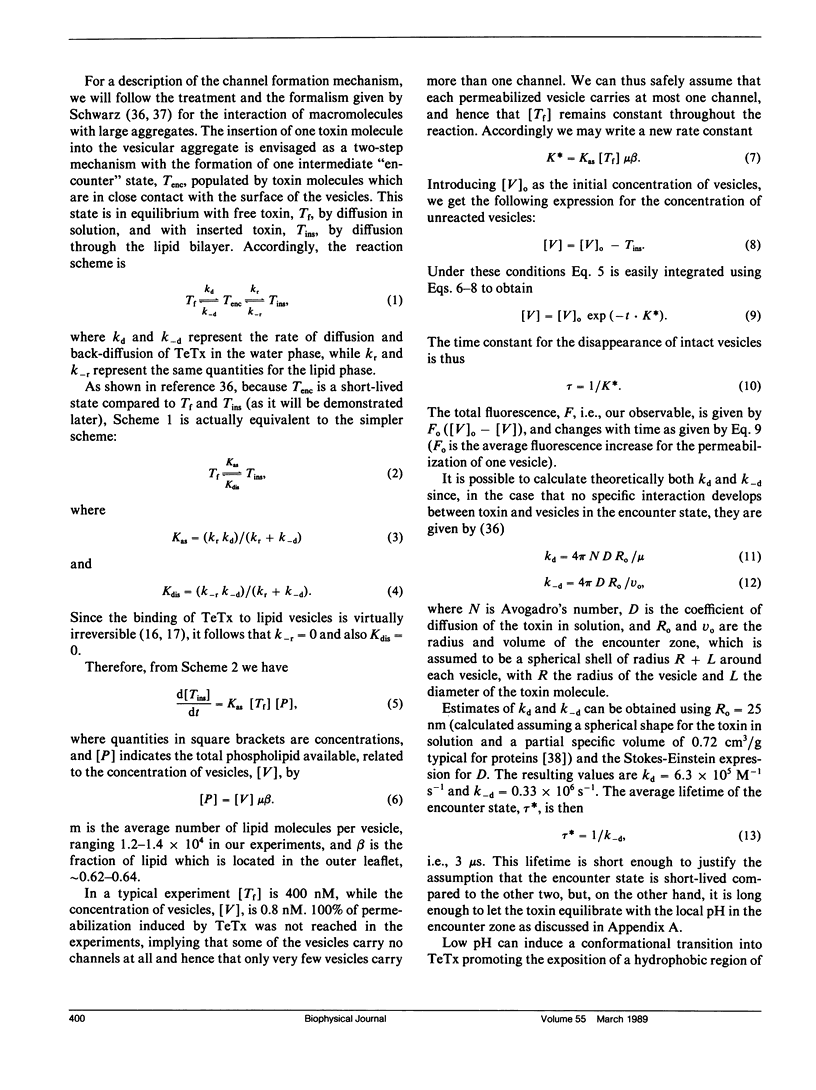
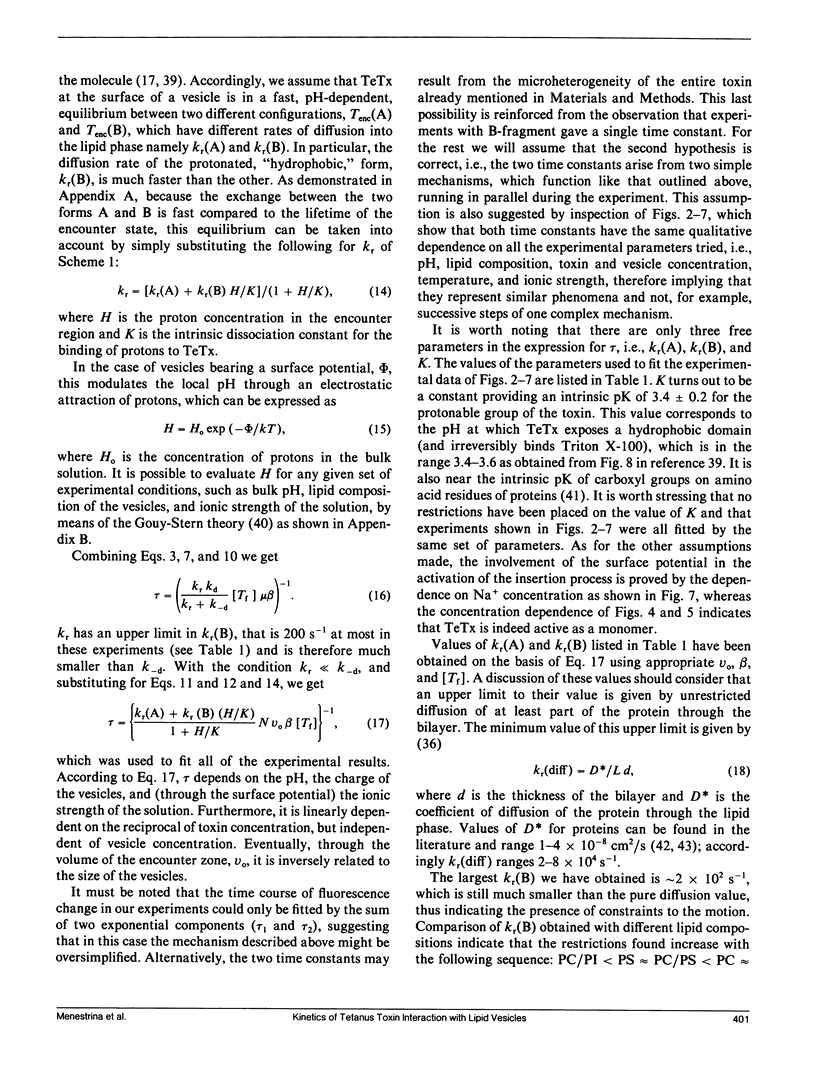
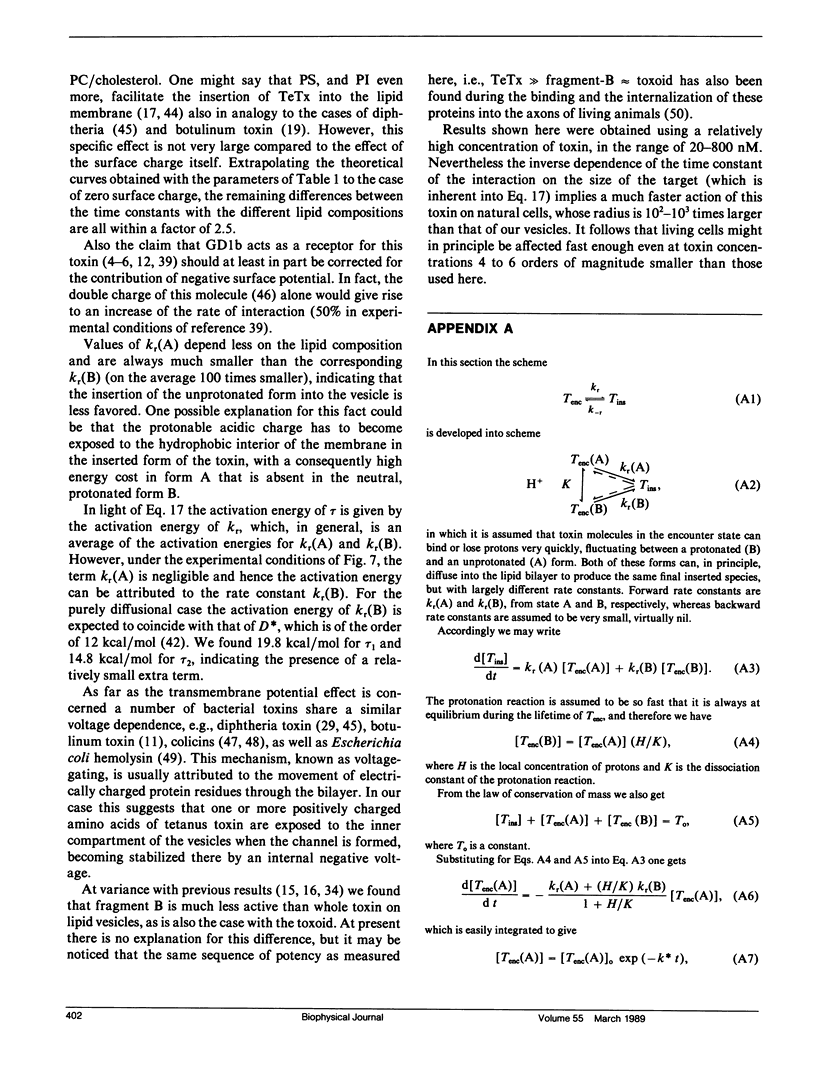
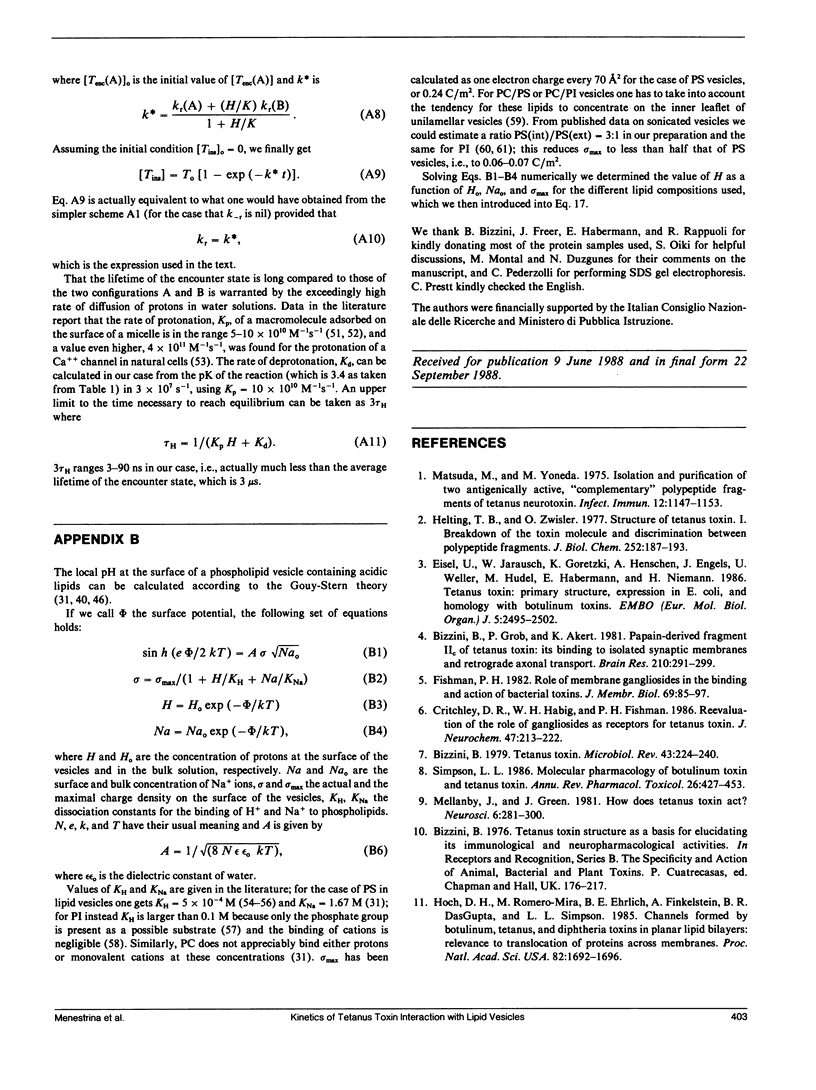
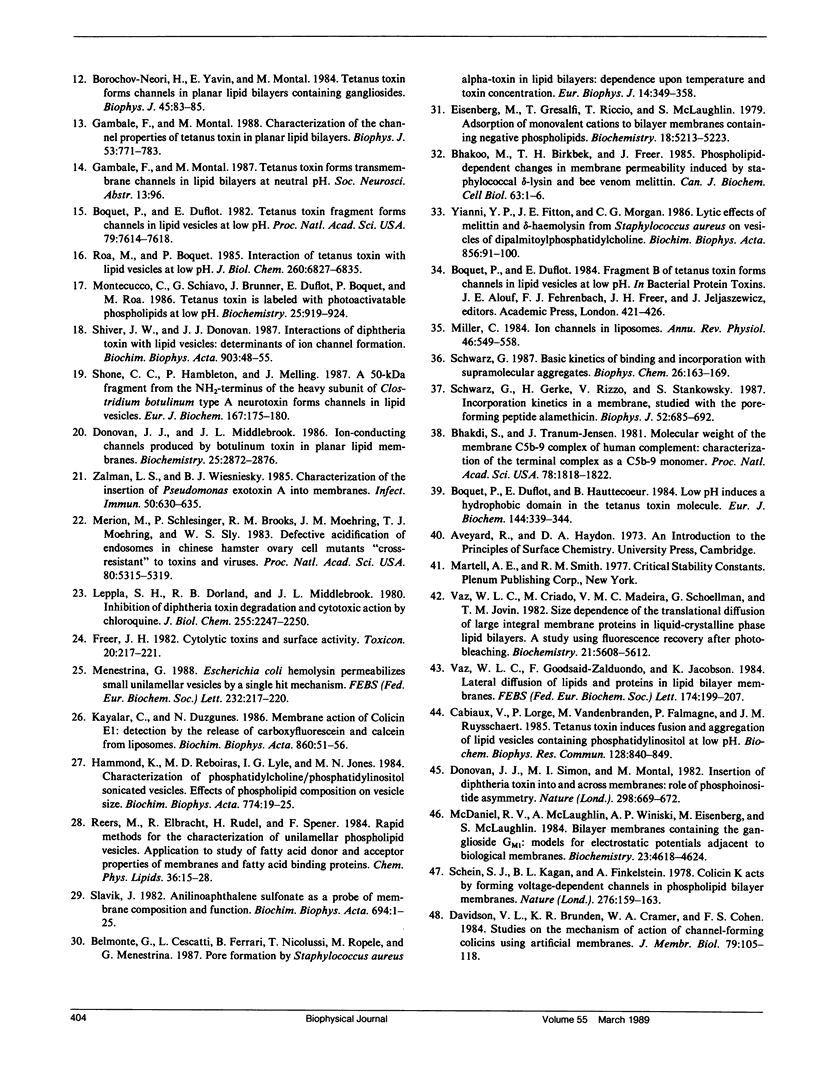
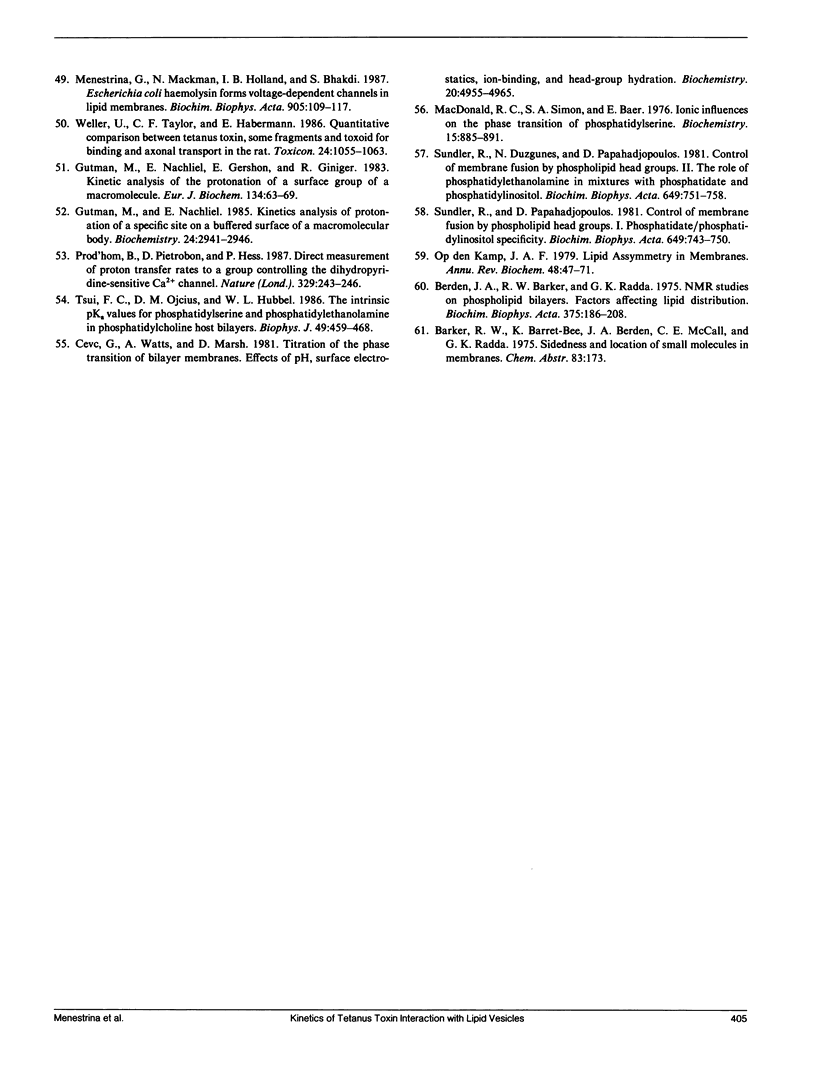
Selected References
These references are in PubMed. This may not be the complete list of references from this article.
- Belmonte G., Cescatti L., Ferrari B., Nicolussi T., Ropele M., Menestrina G. Pore formation by Staphylococcus aureus alpha-toxin in lipid bilayers. Dependence upon temperature and toxin concentration. Eur Biophys J. 1987;14(6):349–358. doi: 10.1007/BF00262320. [DOI] [PubMed] [Google Scholar]
- Berden J. A., Barker R. W., Radda G. K. NMR studies on phospholipid bilayers. Some factors affecting lipid distribution. Biochim Biophys Acta. 1975 Jan 28;375(2):186–208. doi: 10.1016/0005-2736(75)90188-1. [DOI] [PubMed] [Google Scholar]
- Bhakdi S., Tranum-Jensen J. Molecular weight of the membrane C5b-9 complex of human complement: characterization of the terminal complex as a C5b-9 monomer. Proc Natl Acad Sci U S A. 1981 Mar;78(3):1818–1822. doi: 10.1073/pnas.78.3.1818. [DOI] [PMC free article] [PubMed] [Google Scholar]
- Bizzini B., Grob P., Akert K. Papain-derived fragment IIc of tetanus toxin: its binding to isolated synaptic membranes and retrograde axonal transport. Brain Res. 1981 Apr 6;210(1-2):291–299. doi: 10.1016/0006-8993(81)90902-1. [DOI] [PubMed] [Google Scholar]
- Bizzini B. Tetanus toxin. Microbiol Rev. 1979 Jun;43(2):224–240. doi: 10.1128/mr.43.2.224-240.1979. [DOI] [PMC free article] [PubMed] [Google Scholar]
- Boquet P., Duflot E., Hauttecoeur B. Low pH induces a hydrophobic domain in the tetanus toxin molecule. Eur J Biochem. 1984 Oct 15;144(2):339–344. doi: 10.1111/j.1432-1033.1984.tb08469.x. [DOI] [PubMed] [Google Scholar]
- Boquet P., Duflot E. Tetanus toxin fragment forms channels in lipid vesicles at low pH. Proc Natl Acad Sci U S A. 1982 Dec;79(24):7614–7618. doi: 10.1073/pnas.79.24.7614. [DOI] [PMC free article] [PubMed] [Google Scholar]
- Borochov-Neori H., Yavin E., Montal M. Tetanus toxin forms channels in planar lipid bilayers containing gangliosides. Biophys J. 1984 Jan;45(1):83–85. doi: 10.1016/S0006-3495(84)84117-X. [DOI] [PMC free article] [PubMed] [Google Scholar]
- Cabiaux V., Lorge P., Vandenbranden M., Falmagne P., Ruysschaert J. M. Tetanus toxin induces fusion and aggregation of lipid vesicles containing phosphatidylinositol at low pH. Biochem Biophys Res Commun. 1985 Apr 30;128(2):840–849. doi: 10.1016/0006-291x(85)90123-8. [DOI] [PubMed] [Google Scholar]
- Cevc G., Watts A., Marsh D. Titration of the phase transition of phosphatidylserine bilayer membranes. Effects of pH, surface electrostatics, ion binding, and head-group hydration. Biochemistry. 1981 Aug 18;20(17):4955–4965. doi: 10.1021/bi00520a023. [DOI] [PubMed] [Google Scholar]
- Critchley D. R., Habig W. H., Fishman P. H. Reevaluation of the role of gangliosides as receptors for tetanus toxin. J Neurochem. 1986 Jul;47(1):213–222. doi: 10.1111/j.1471-4159.1986.tb02852.x. [DOI] [PubMed] [Google Scholar]
- Davidson V. L., Brunden K. R., Cramer W. A., Cohen F. S. Studies on the mechanism of action of channel-forming colicins using artificial membranes. J Membr Biol. 1984;79(2):105–118. doi: 10.1007/BF01872115. [DOI] [PubMed] [Google Scholar]
- Donovan J. J., Middlebrook J. L. Ion-conducting channels produced by botulinum toxin in planar lipid membranes. Biochemistry. 1986 May 20;25(10):2872–2876. doi: 10.1021/bi00358a020. [DOI] [PubMed] [Google Scholar]
- Donovan J. J., Simon M. I., Montal M. Insertion of diphtheria toxin into and across membranes: role of phosphoinositide asymmetry. Nature. 1982 Aug 12;298(5875):669–672. doi: 10.1038/298669a0. [DOI] [PubMed] [Google Scholar]
- Eisel U., Jarausch W., Goretzki K., Henschen A., Engels J., Weller U., Hudel M., Habermann E., Niemann H. Tetanus toxin: primary structure, expression in E. coli, and homology with botulinum toxins. EMBO J. 1986 Oct;5(10):2495–2502. doi: 10.1002/j.1460-2075.1986.tb04527.x. [DOI] [PMC free article] [PubMed] [Google Scholar]
- Eisenberg M., Gresalfi T., Riccio T., McLaughlin S. Adsorption of monovalent cations to bilayer membranes containing negative phospholipids. Biochemistry. 1979 Nov 13;18(23):5213–5223. doi: 10.1021/bi00590a028. [DOI] [PubMed] [Google Scholar]
- Fishman P. H. Role of membrane gangliosides in the binding and action of bacterial toxins. J Membr Biol. 1982;69(2):85–97. doi: 10.1007/BF01872268. [DOI] [PubMed] [Google Scholar]
- Freer J. H. Cytolytic toxins and surface activity. Toxicon. 1982;20(1):217–221. doi: 10.1016/0041-0101(82)90204-5. [DOI] [PubMed] [Google Scholar]
- Gambale F., Montal M. Characterization of the channel properties of tetanus toxin in planar lipid bilayers. Biophys J. 1988 May;53(5):771–783. doi: 10.1016/S0006-3495(88)83157-6. [DOI] [PMC free article] [PubMed] [Google Scholar]
- Gutman M., Nachliel E., Gershon E., Giniger R. Kinetic analysis of the protonation of a surface group of a macromolecule. Eur J Biochem. 1983 Jul 15;134(1):63–69. doi: 10.1111/j.1432-1033.1983.tb07531.x. [DOI] [PubMed] [Google Scholar]
- Gutman M., Nachliel E. Kinetic analysis of protonation of a specific site on a buffered surface of a macromolecular body. Biochemistry. 1985 Jun 4;24(12):2941–2946. doi: 10.1021/bi00333a020. [DOI] [PubMed] [Google Scholar]
- Hammond K., Reboiras M. D., Lyle I. G., Jones M. N. Characterisation of phosphatidylcholine/phosphatidylinositol sonicated vesicles. Effects of phospholipid composition on vesicle size. Biochim Biophys Acta. 1984 Jul 11;774(1):19–25. doi: 10.1016/0005-2736(84)90269-4. [DOI] [PubMed] [Google Scholar]
- Helting T. B., Zwisler O. Structure of tetanus toxin. I. Breakdown of the toxin molecule and discrimination between polypeptide fragments. J Biol Chem. 1977 Jan 10;252(1):187–193. [PubMed] [Google Scholar]
- Hoch D. H., Romero-Mira M., Ehrlich B. E., Finkelstein A., DasGupta B. R., Simpson L. L. Channels formed by botulinum, tetanus, and diphtheria toxins in planar lipid bilayers: relevance to translocation of proteins across membranes. Proc Natl Acad Sci U S A. 1985 Mar;82(6):1692–1696. doi: 10.1073/pnas.82.6.1692. [DOI] [PMC free article] [PubMed] [Google Scholar]
- Kayalar C., Düzgüneş N. Membrane action of colicin E1: detection by the release of carboxyfluorescein and calcein from liposomes. Biochim Biophys Acta. 1986 Aug 7;860(1):51–56. doi: 10.1016/0005-2736(86)90497-9. [DOI] [PubMed] [Google Scholar]
- Leppla S., Dorland R. B., Middlebrook J. L. Inhibition of diphtheria toxin degradation and cytotoxic action by chloroquine. J Biol Chem. 1980 Mar 25;255(6):2247–2250. [PubMed] [Google Scholar]
- MacDonald R. C., Simon S. A., Baer E. Ionic influences on the phase transition of dipalmitoylphosphatidylserine. Biochemistry. 1976 Feb 24;15(4):885–891. doi: 10.1021/bi00649a025. [DOI] [PubMed] [Google Scholar]
- Matsuda M., Yoneda M. Isolation and purification of two antigenically active, "complimentary" polypeptide fragments of tetanus neurotoxin. Infect Immun. 1975 Nov;12(5):1147–1153. doi: 10.1128/iai.12.5.1147-1153.1975. [DOI] [PMC free article] [PubMed] [Google Scholar]
- McDaniel R. V., McLaughlin A., Winiski A. P., Eisenberg M., McLaughlin S. Bilayer membranes containing the ganglioside GM1: models for electrostatic potentials adjacent to biological membranes. Biochemistry. 1984 Sep 25;23(20):4618–4624. doi: 10.1021/bi00315a016. [DOI] [PubMed] [Google Scholar]
- Mellanby J., Green J. How does tetanus toxin act? Neuroscience. 1981;6(3):281–300. doi: 10.1016/0306-4522(81)90123-8. [DOI] [PubMed] [Google Scholar]
- Menestrina G. Escherichia coli hemolysin permeabilizes small unilamellar vesicles loaded with calcein by a single-hit mechanism. FEBS Lett. 1988 May 9;232(1):217–220. doi: 10.1016/0014-5793(88)80420-4. [DOI] [PubMed] [Google Scholar]
- Menestrina G., Mackman N., Holland I. B., Bhakdi S. Escherichia coli haemolysin forms voltage-dependent ion channels in lipid membranes. Biochim Biophys Acta. 1987 Nov 27;905(1):109–117. doi: 10.1016/0005-2736(87)90014-9. [DOI] [PubMed] [Google Scholar]
- Merion M., Schlesinger P., Brooks R. M., Moehring J. M., Moehring T. J., Sly W. S. Defective acidification of endosomes in Chinese hamster ovary cell mutants "cross-resistant" to toxins and viruses. Proc Natl Acad Sci U S A. 1983 Sep;80(17):5315–5319. doi: 10.1073/pnas.80.17.5315. [DOI] [PMC free article] [PubMed] [Google Scholar]
- Miller C. Ion channels in liposomes. Annu Rev Physiol. 1984;46:549–558. doi: 10.1146/annurev.ph.46.030184.003001. [DOI] [PubMed] [Google Scholar]
- Montecucco C., Schiavo G., Brunner J., Duflot E., Boquet P., Roa M. Tetanus toxin is labeled with photoactivatable phospholipids at low pH. Biochemistry. 1986 Feb 25;25(4):919–924. doi: 10.1021/bi00352a027. [DOI] [PubMed] [Google Scholar]
- Op den Kamp J. A. Lipid asymmetry in membranes. Annu Rev Biochem. 1979;48:47–71. doi: 10.1146/annurev.bi.48.070179.000403. [DOI] [PubMed] [Google Scholar]
- Prod'hom B., Pietrobon D., Hess P. Direct measurement of proton transfer rates to a group controlling the dihydropyridine-sensitive Ca2+ channel. Nature. 1987 Sep 17;329(6136):243–246. doi: 10.1038/329243a0. [DOI] [PubMed] [Google Scholar]
- Roa M., Boquet P. Interaction of tetanus toxin with lipid vesicles at low pH. Protection of specific polypeptides against proteolysis. J Biol Chem. 1985 Jun 10;260(11):6827–6835. [PubMed] [Google Scholar]
- Schein S. J., Kagan B. L., Finkelstein A. Colicin K acts by forming voltage-dependent channels in phospholipid bilayer membranes. Nature. 1978 Nov 9;276(5684):159–163. doi: 10.1038/276159a0. [DOI] [PubMed] [Google Scholar]
- Schwarz G. Basic kinetics of binding and incorporation with supramolecular aggregates. Biophys Chem. 1987 May 9;26(2-3):163–169. doi: 10.1016/0301-4622(87)80019-4. [DOI] [PubMed] [Google Scholar]
- Schwarz G., Gerke H., Rizzo V., Stankowski S. Incorporation kinetics in a membrane, studied with the pore-forming peptide alamethicin. Biophys J. 1987 Nov;52(5):685–692. doi: 10.1016/S0006-3495(87)83263-0. [DOI] [PMC free article] [PubMed] [Google Scholar]
- Shiver J. W., Donovan J. J. Interactions of diphtheria toxin with lipid vesicles: determinants of ion channel formation. Biochim Biophys Acta. 1987 Sep 18;903(1):48–55. doi: 10.1016/0005-2736(87)90154-4. [DOI] [PubMed] [Google Scholar]
- Shone C. C., Hambleton P., Melling J. A 50-kDa fragment from the NH2-terminus of the heavy subunit of Clostridium botulinum type A neurotoxin forms channels in lipid vesicles. Eur J Biochem. 1987 Aug 17;167(1):175–180. doi: 10.1111/j.1432-1033.1987.tb13320.x. [DOI] [PubMed] [Google Scholar]
- Simpson L. L. Molecular pharmacology of botulinum toxin and tetanus toxin. Annu Rev Pharmacol Toxicol. 1986;26:427–453. doi: 10.1146/annurev.pa.26.040186.002235. [DOI] [PubMed] [Google Scholar]
- Slavík J. Anilinonaphthalene sulfonate as a probe of membrane composition and function. Biochim Biophys Acta. 1982 Aug 11;694(1):1–25. doi: 10.1016/0304-4157(82)90012-0. [DOI] [PubMed] [Google Scholar]
- Sundler R., Düzgüneş N., Papahadjopoulos D. Control of membrane fusion by phospholipid head groups. II. The role of phosphatidylethanolamine in mixtures with phosphatidate and phosphatidylinositol. Biochim Biophys Acta. 1981 Dec 21;649(3):751–758. doi: 10.1016/0005-2736(81)90180-2. [DOI] [PubMed] [Google Scholar]
- Sundler R., Papahadjopoulos D. Control of membrane fusion by phospholipid head groups. I. Phosphatidate/phosphatidylinositol specificity. Biochim Biophys Acta. 1981 Dec 21;649(3):743–750. doi: 10.1016/0005-2736(81)90179-6. [DOI] [PubMed] [Google Scholar]
- Tsui F. C., Ojcius D. M., Hubbell W. L. The intrinsic pKa values for phosphatidylserine and phosphatidylethanolamine in phosphatidylcholine host bilayers. Biophys J. 1986 Feb;49(2):459–468. doi: 10.1016/S0006-3495(86)83655-4. [DOI] [PMC free article] [PubMed] [Google Scholar]
- Vaz W. L., Criado M., Madeira V. M., Schoellmann G., Jovin T. M. Size dependence of the translational diffusion of large integral membrane proteins in liquid-crystalline phase lipid bilayers. A study using fluorescence recovery after photobleaching. Biochemistry. 1982 Oct 26;21(22):5608–5612. doi: 10.1021/bi00265a034. [DOI] [PubMed] [Google Scholar]
- Weller U., Taylor C. F., Habermann E. Quantitative comparison between tetanus toxin, some fragments and toxoid for binding and axonal transport in the rat. Toxicon. 1986;24(11-12):1055–1063. doi: 10.1016/0041-0101(86)90132-7. [DOI] [PubMed] [Google Scholar]
- Yianni Y. P., Fitton J. E., Morgan C. G. Lytic effects of melittin and delta-haemolysin from Staphylococcus aureus on vesicles of dipalmitoylphosphatidylcholine. Biochim Biophys Acta. 1986 Mar 27;856(1):91–100. doi: 10.1016/0005-2736(86)90014-3. [DOI] [PubMed] [Google Scholar]
- Zalman L. S., Wisnieski B. J. Characterization of the insertion of Pseudomonas exotoxin A into membranes. Infect Immun. 1985 Dec;50(3):630–635. doi: 10.1128/iai.50.3.630-635.1985. [DOI] [PMC free article] [PubMed] [Google Scholar]