Abstract
Our studies are concerned with the frequency tuning that is provided by the electrical resonance of tuberous electroreceptors. Frequency selectivity had previously been measured in the electroreceptor's afferent fibers, and resonant conductances in the electroreceptor cell membrane had been implicated in producing the selectivity. With transdermal application of sinusoidal current, we measured the frequency dependence of the impedance of small areas of the electroreceptor/skin structure of the weakly electric fish Sternopygus and Eigenmannia, and used our data to make a quantitative linear model of the structure. The qualitative form of the model was proposed by Bennett (1). The quantitative model allows us to estimate the frequency selectivity of the voltage across the innervated membrane of the electroreceptor cells. The frequency selectivity of electroreceptor cell voltage derived from our data are as sharp as the neural selectivity at frequencies close to the most sensitive frequency. Many of our measurements supported the linear system model. However, spontaneous electroreceptor voltage oscillations were detected in some of our specimens, suggesting that the electroreceptors can operate in a regime of active nonlinearity. A simple explanation for the observed oscillations is that they arise when damping in the electroreceptor cell's resonant membrane is negative for a limited span of membrane voltage surrounding the resting voltage. The response of oscillating units to sinusoidal current was compatible with this explanation. We report experimental observations bearing on the consequences of active nonlinearity for the frequency tuning of a resonant system.
Full text
PDF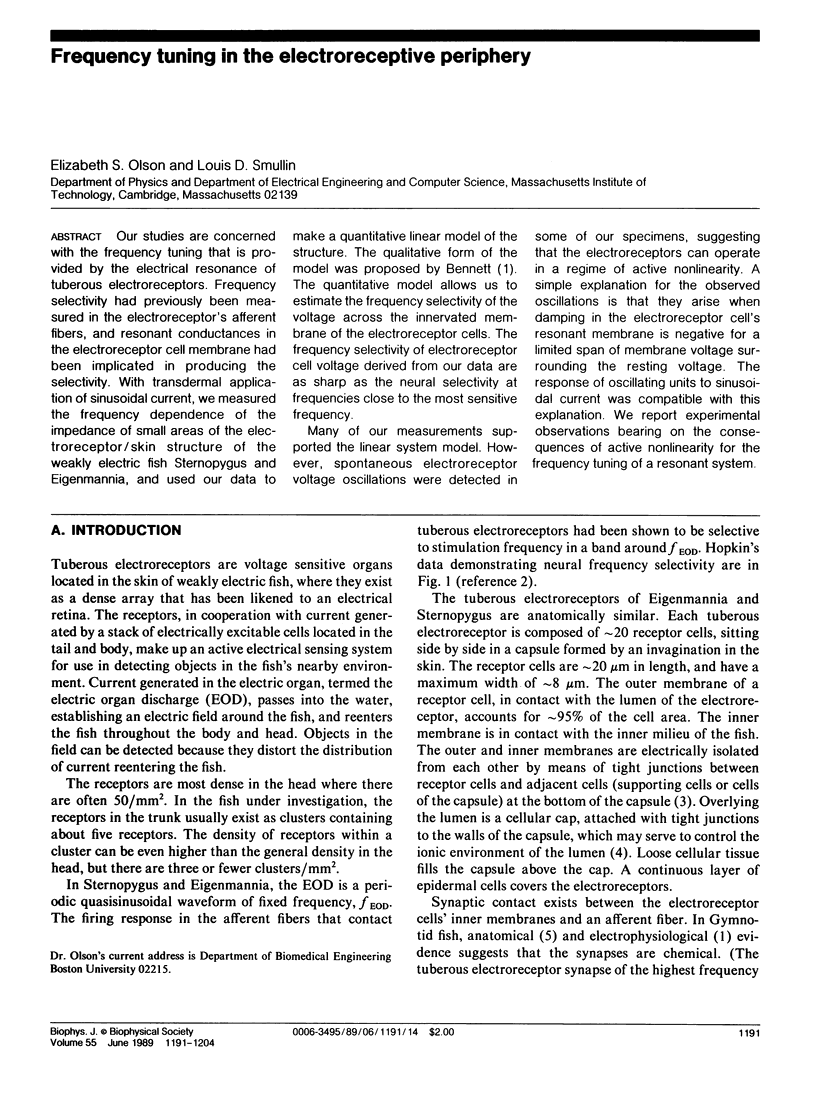
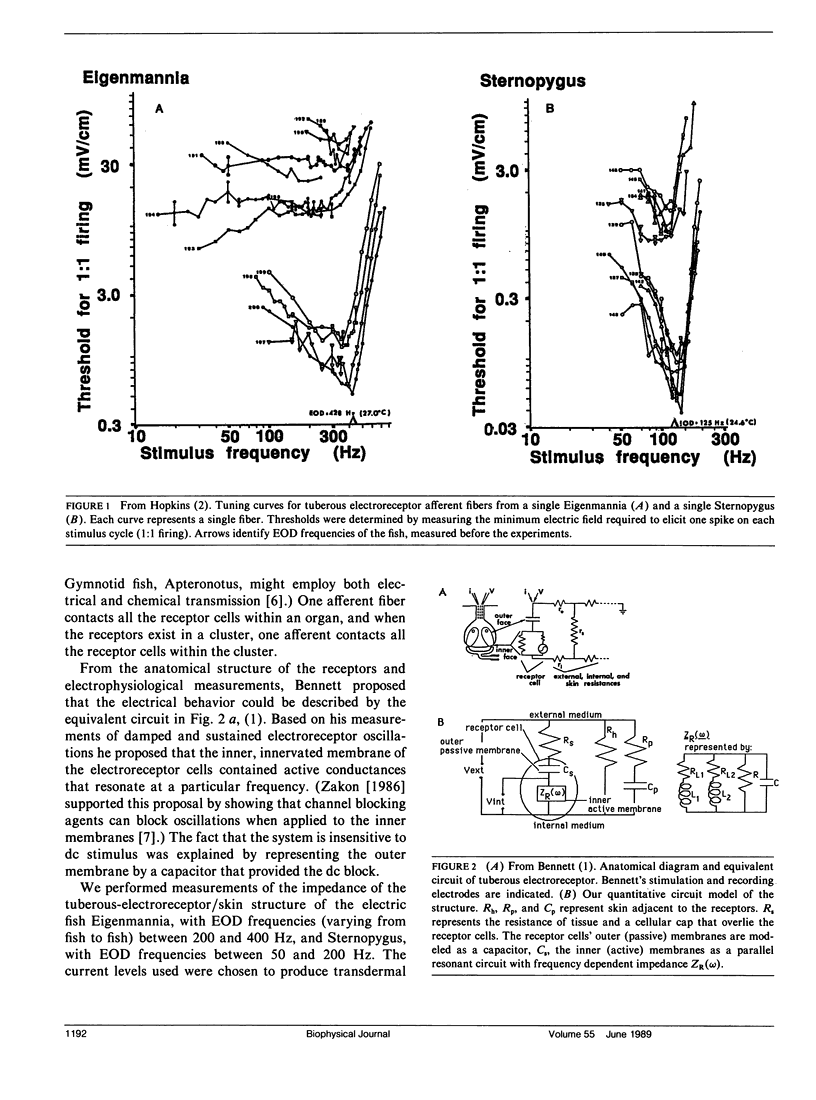
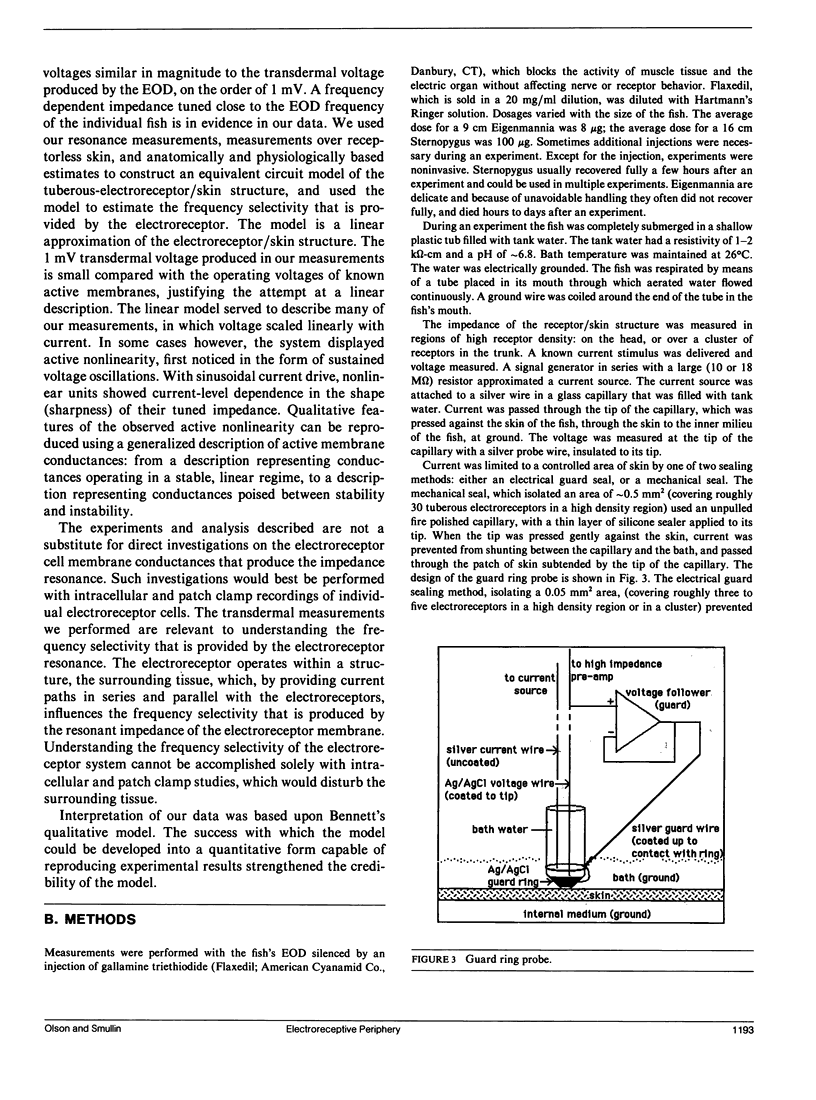
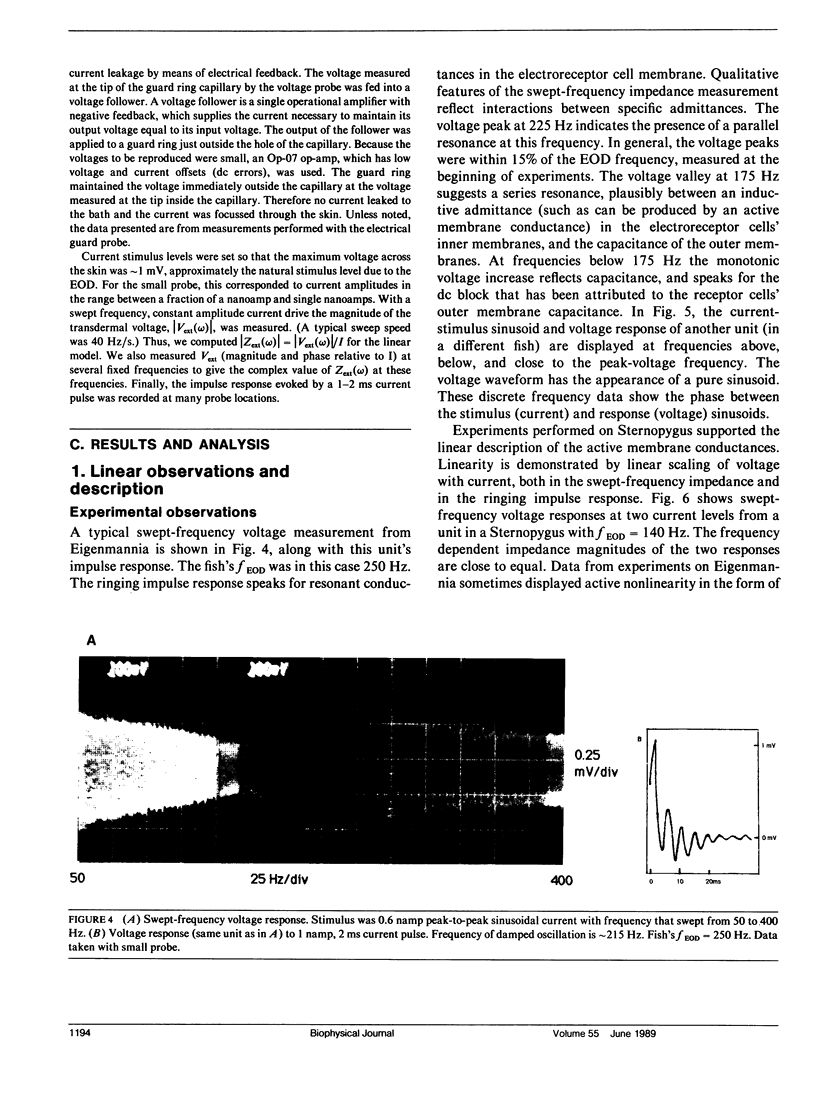
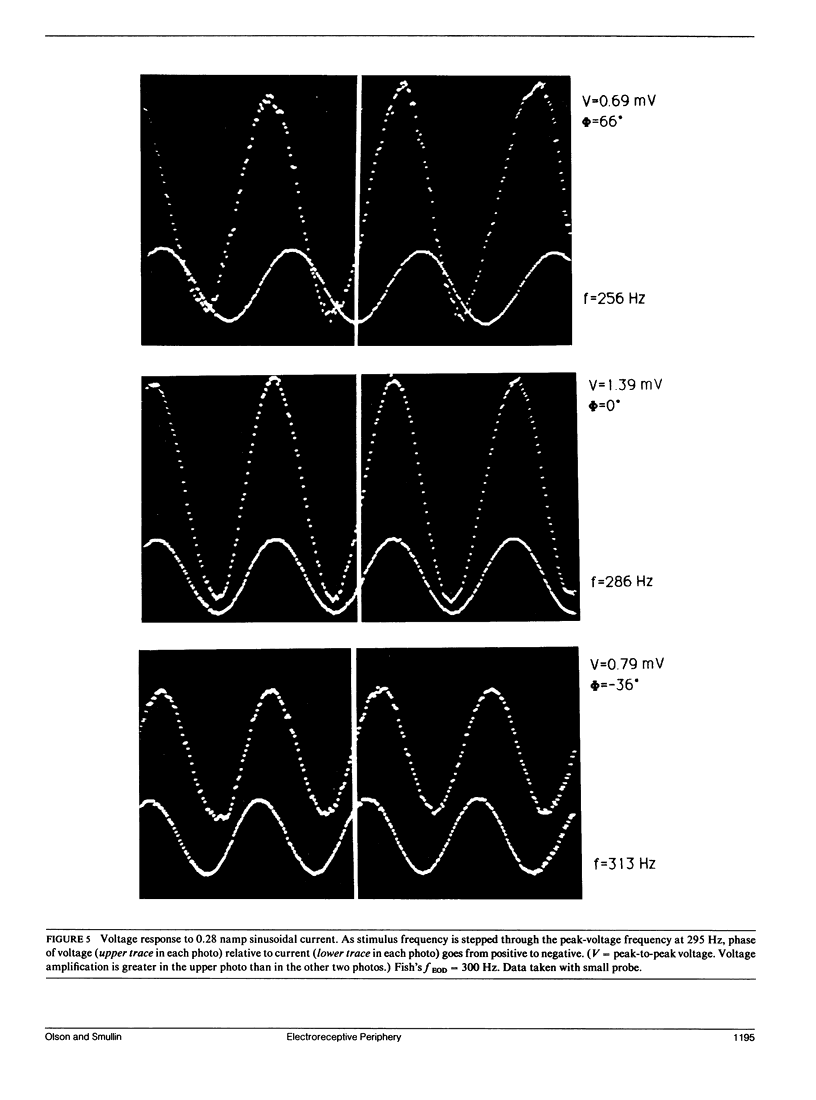
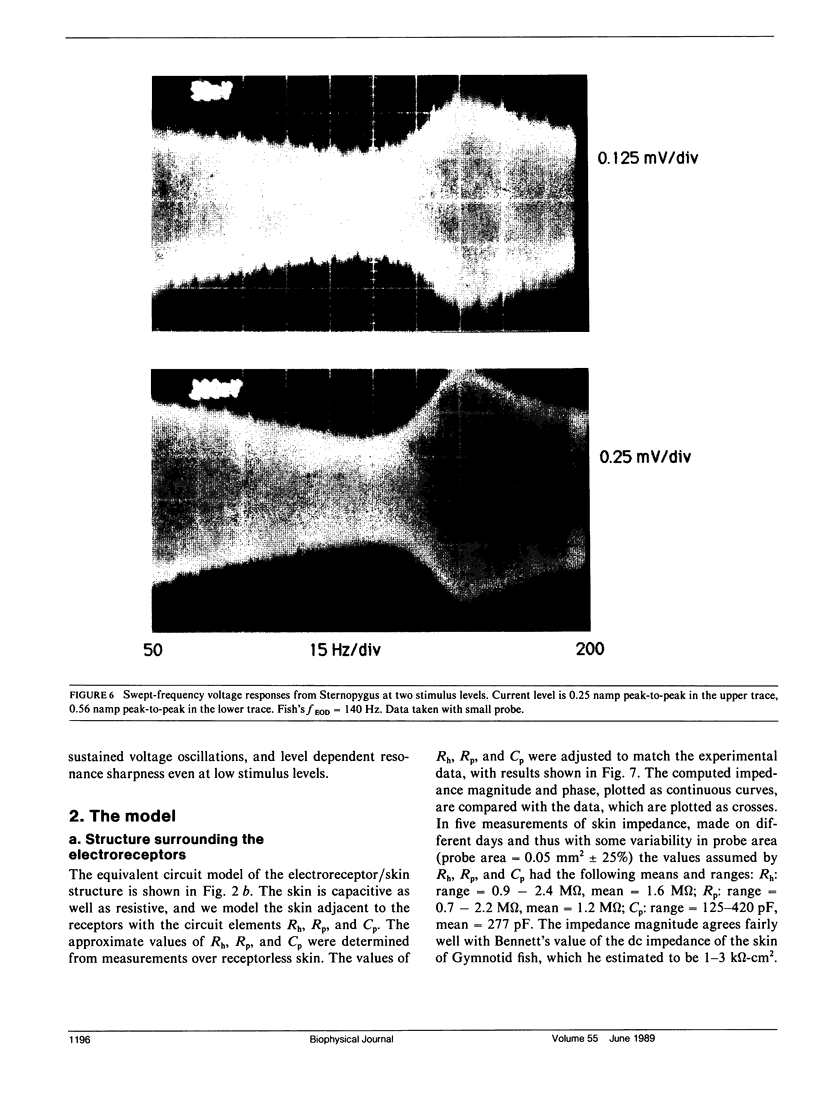
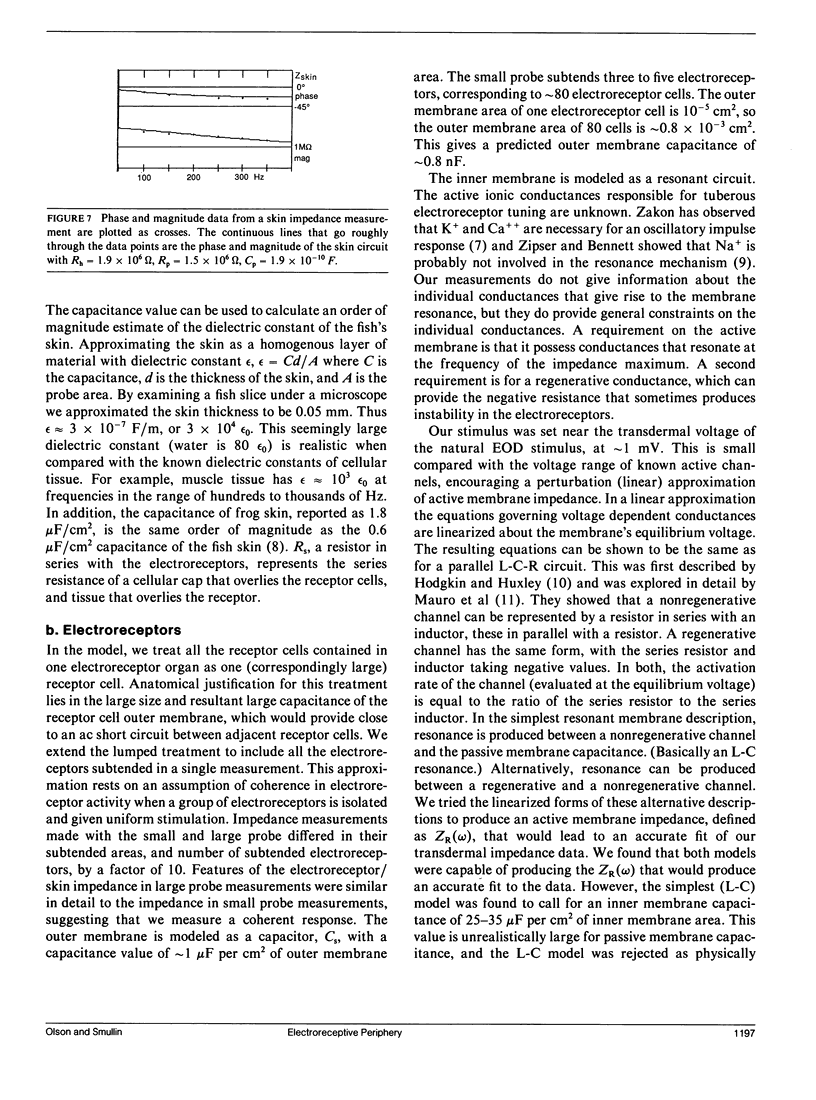
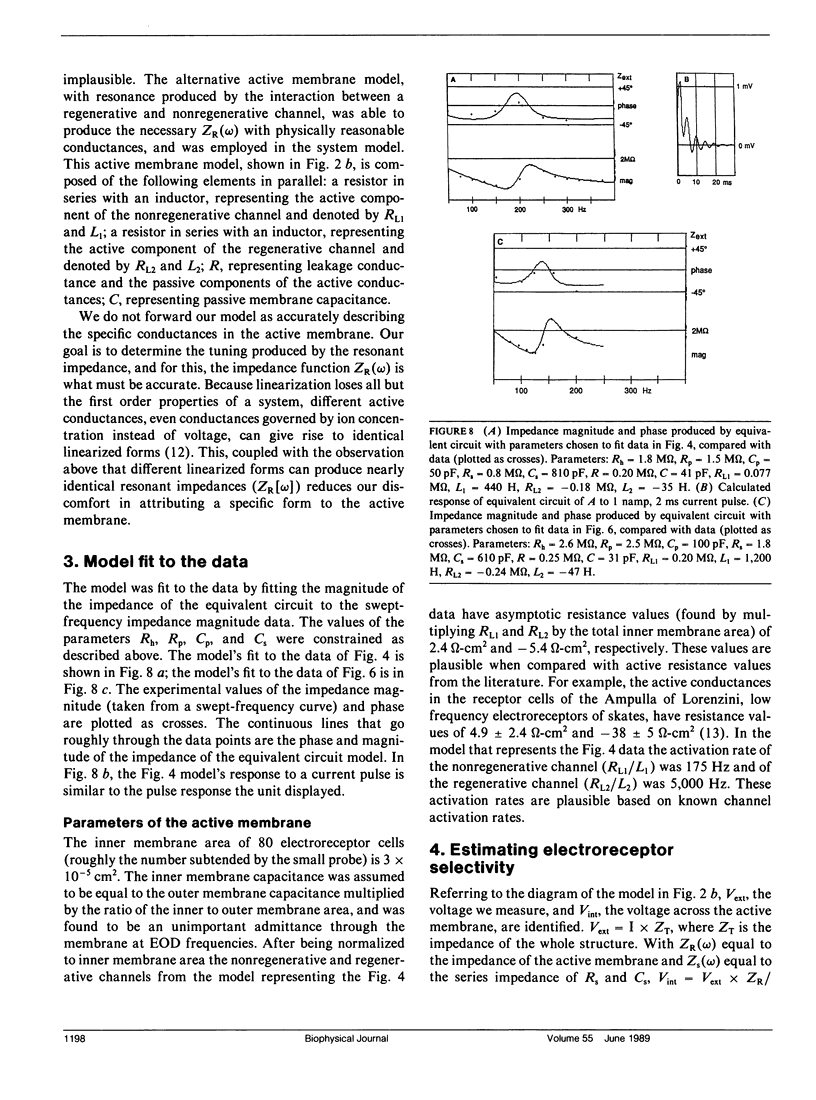
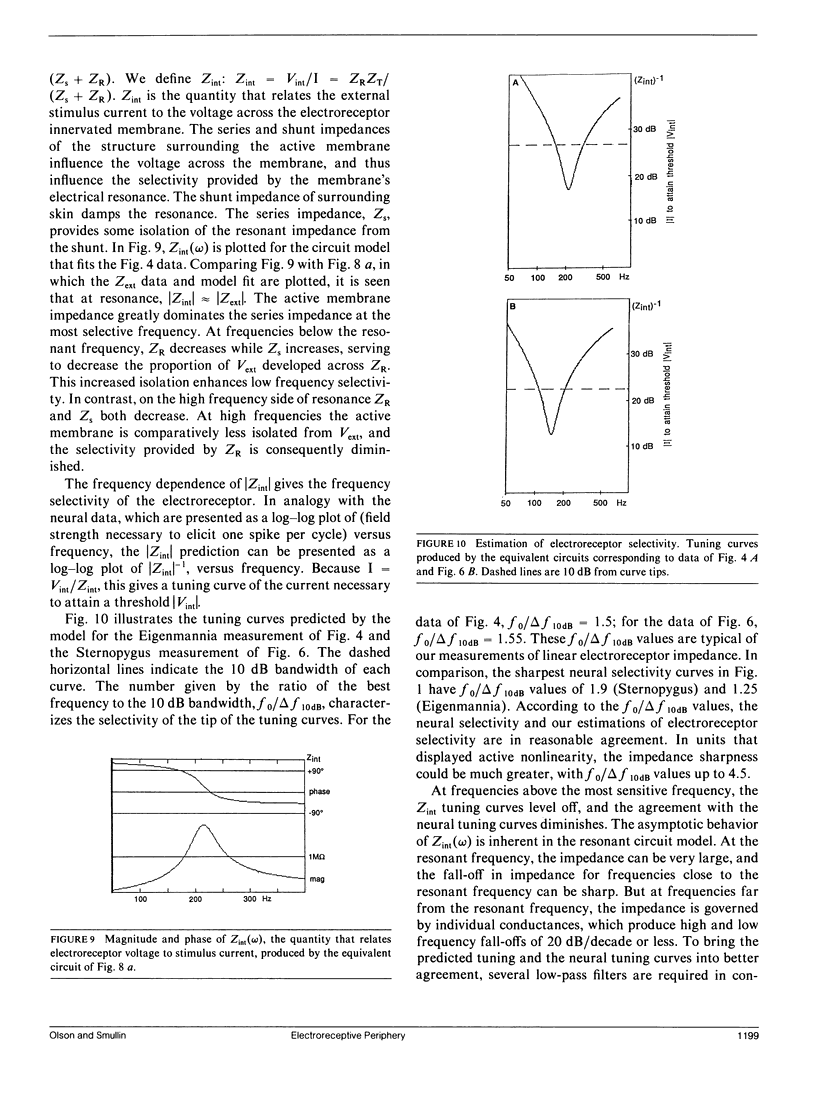
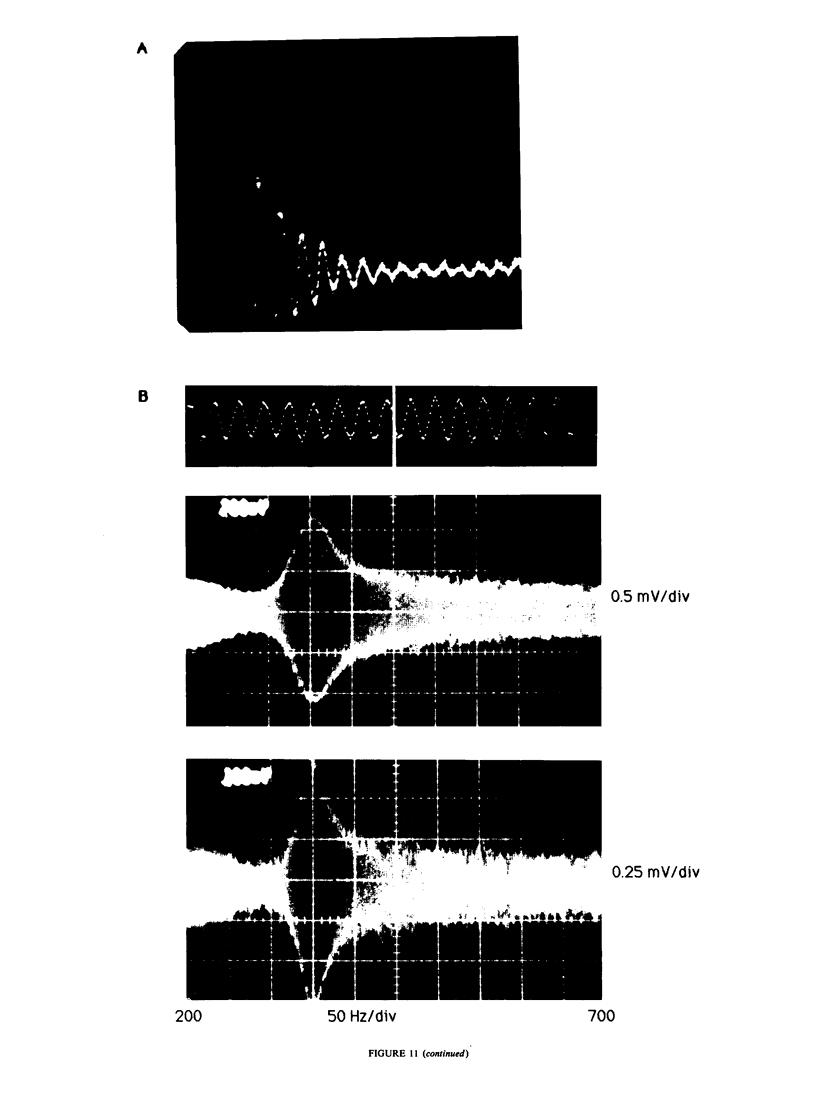
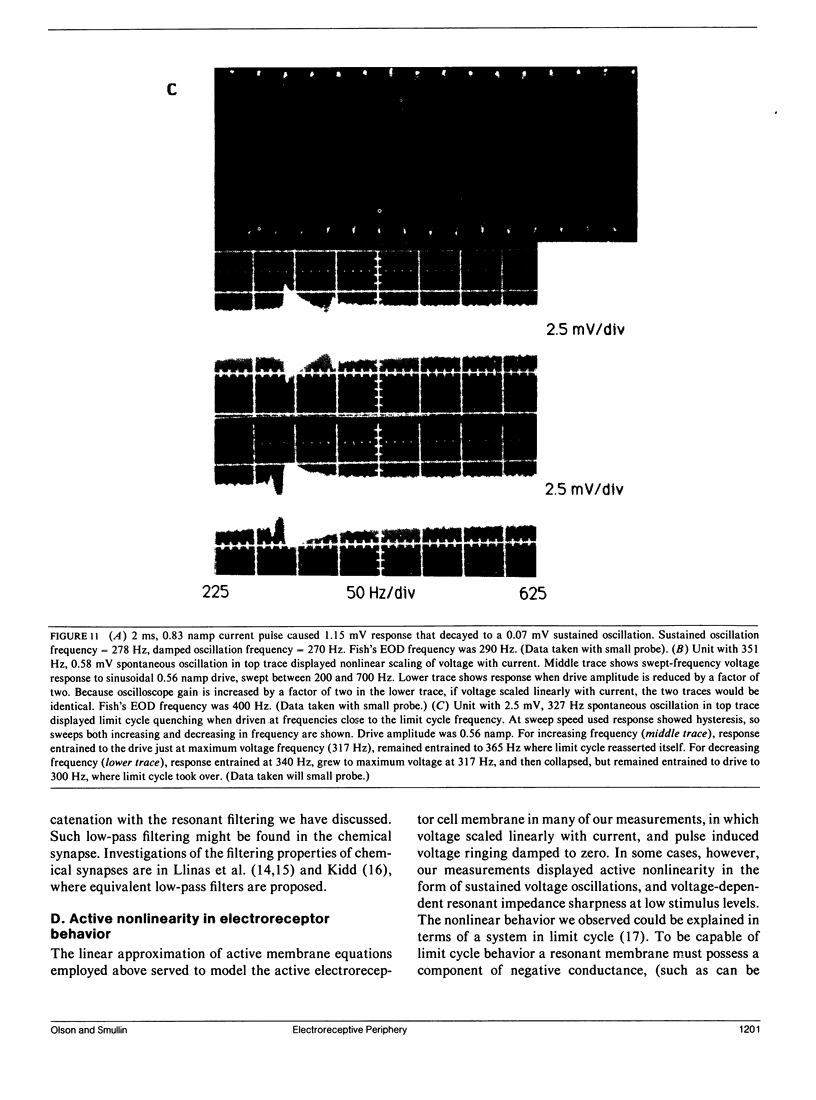
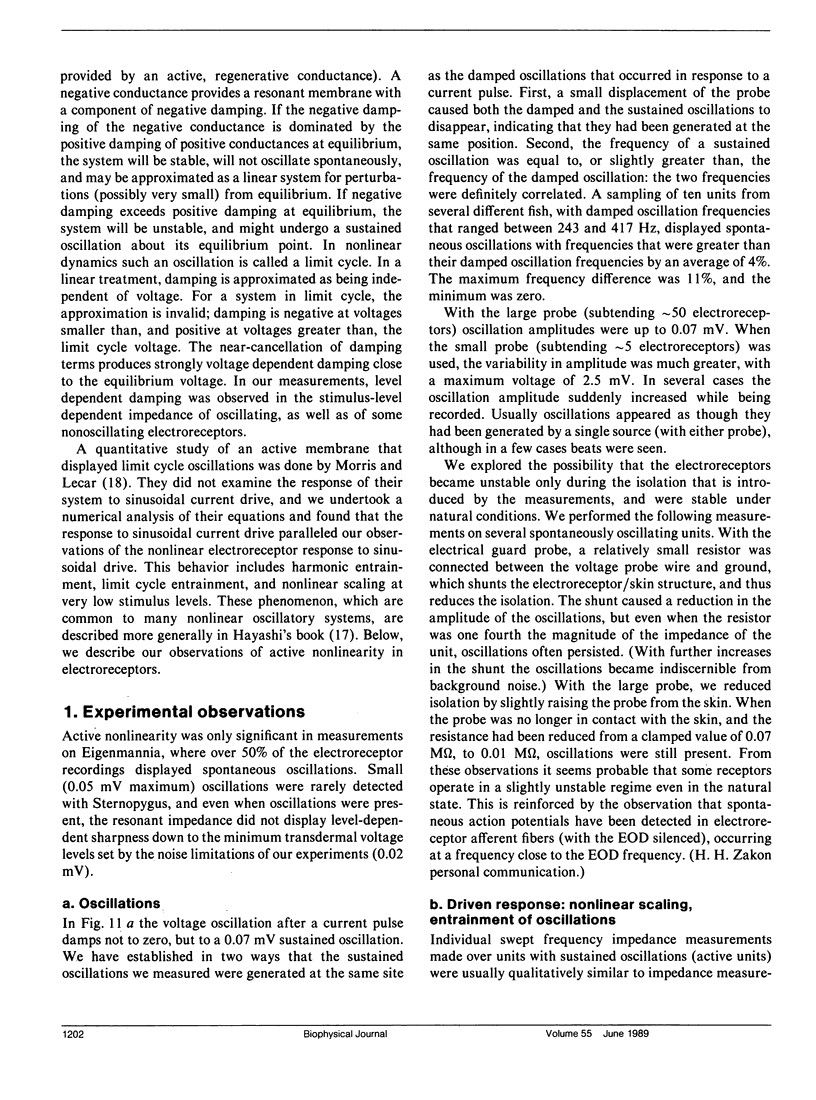
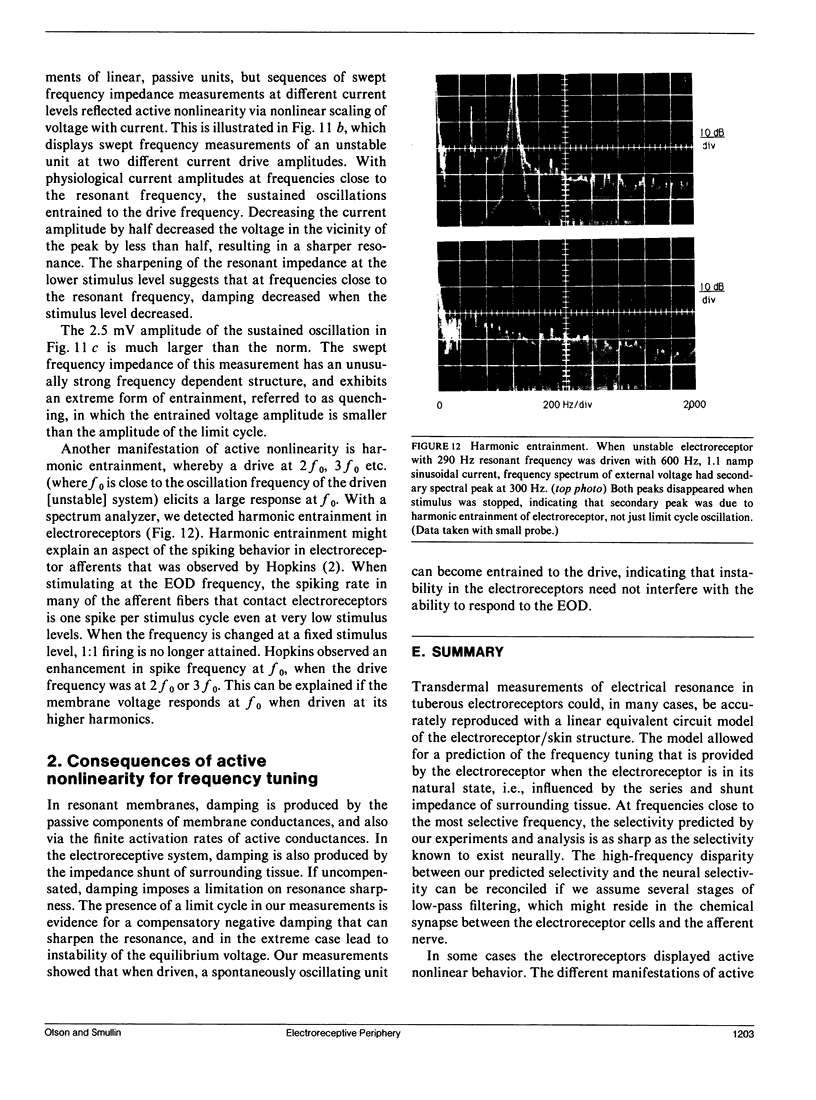
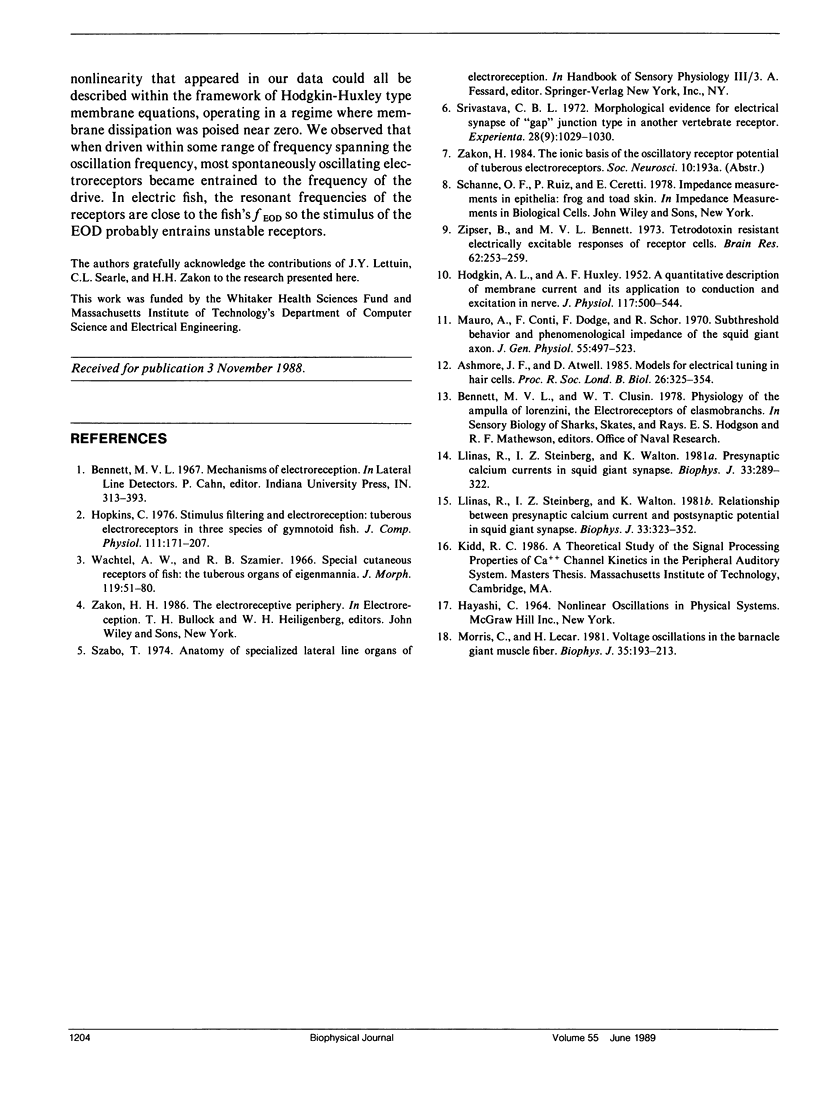
Images in this article
Selected References
These references are in PubMed. This may not be the complete list of references from this article.
- HODGKIN A. L., HUXLEY A. F. A quantitative description of membrane current and its application to conduction and excitation in nerve. J Physiol. 1952 Aug;117(4):500–544. doi: 10.1113/jphysiol.1952.sp004764. [DOI] [PMC free article] [PubMed] [Google Scholar]
- Llinás R., Steinberg I. Z., Walton K. Presynaptic calcium currents in squid giant synapse. Biophys J. 1981 Mar;33(3):289–321. doi: 10.1016/S0006-3495(81)84898-9. [DOI] [PMC free article] [PubMed] [Google Scholar]
- Llinás R., Steinberg I. Z., Walton K. Relationship between presynaptic calcium current and postsynaptic potential in squid giant synapse. Biophys J. 1981 Mar;33(3):323–351. doi: 10.1016/S0006-3495(81)84899-0. [DOI] [PMC free article] [PubMed] [Google Scholar]
- Mauro A., Conti F., Dodge F., Schor R. Subthreshold behavior and phenomenological impedance of the squid giant axon. J Gen Physiol. 1970 Apr;55(4):497–523. doi: 10.1085/jgp.55.4.497. [DOI] [PMC free article] [PubMed] [Google Scholar]
- Morris C., Lecar H. Voltage oscillations in the barnacle giant muscle fiber. Biophys J. 1981 Jul;35(1):193–213. doi: 10.1016/S0006-3495(81)84782-0. [DOI] [PMC free article] [PubMed] [Google Scholar]
- Srivastava C. B. Morphological evidence for electrical synapse of 'gap' junction type in another vertebrate receptor. Experientia. 1972 Sep 15;28(9):1029–1030. doi: 10.1007/BF01918652. [DOI] [PubMed] [Google Scholar]
- Zipser B., Bennett M. V. Tetrodotoxin resistant electrically excitable responses of receptor cells. Brain Res. 1973 Nov 9;62(1):253–259. doi: 10.1016/0006-8993(73)90637-9. [DOI] [PubMed] [Google Scholar]