Abstract
Two independent methods, induced osmosis and solvent drag, were used to determine the reflection coefficients for NaCl (sigma NaCl) in brush border and basolateral membrane vesicles isolated from rabbit proximal tubule. In the induced osmosis method, vesicles loaded with sucrose were subjected to varying inward NaCl gradients in a stopped-flow apparatus. sigma NaCl was determined from the osmolality of the NaCl solution required to cause no initial osmotic water flux as measured by light scattering (null point). By this method sigma NaCl was greater than 0.92 for both apical and basolateral membranes with best estimates of 1.0. sigma NaCl was determined by the solvent drag method using the Cl-sensitive fluorescent indicator, 6-methoxy-N-[3-sulfopropyl]quinolinium (SPQ), to detect the drag of Cl into vesicles by inward osmotic water movement caused by an outward osmotic gradient. sigma NaCl was determined by comparing experimental data with theoretical curves generated using the coupled flux equations of Kedem and Katchalsky. By this method we found that sigma NaCl was greater than 0.96 for apical and greater than 0.98 for basolateral membrane vesicles, with best estimates of 1.0 for both membranes. These results demonstrate that sigma NaCl for proximal tubule apical and basolateral membranes are near unity. Taken together with previous results, these data suggest that proximal tubule water channels are long narrow pores that exclude NaCl.
Full text
PDF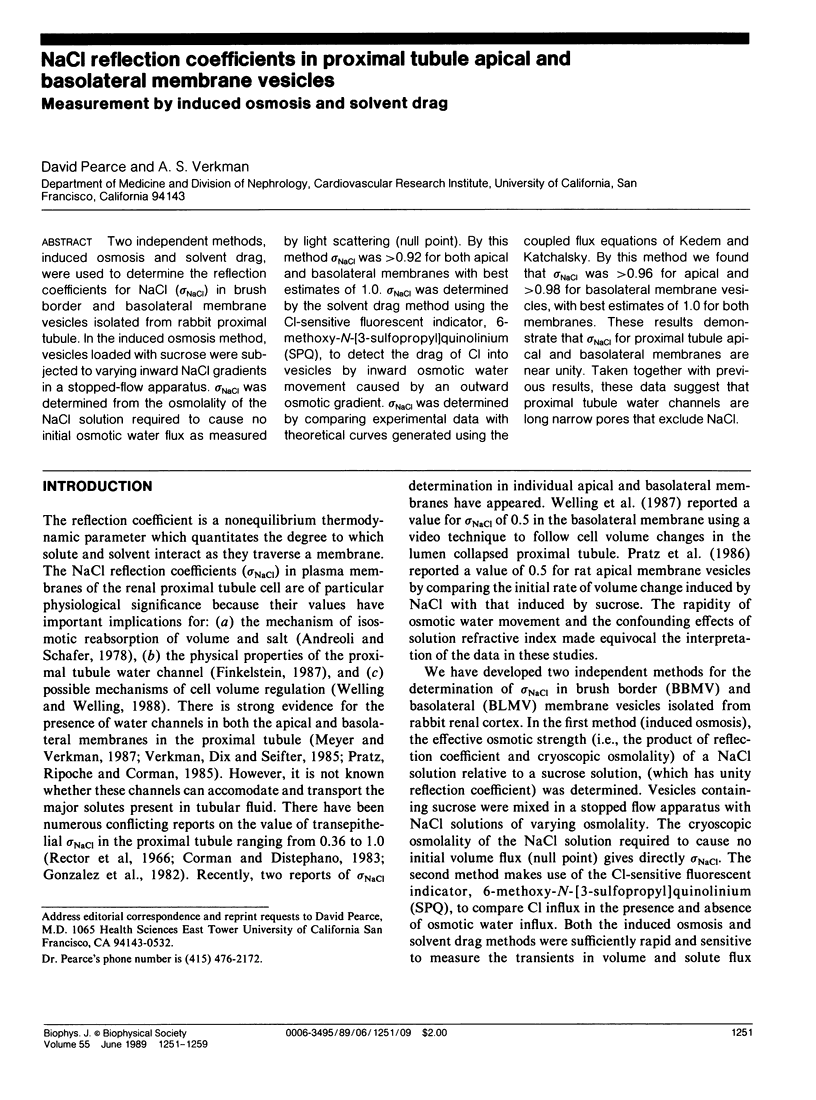
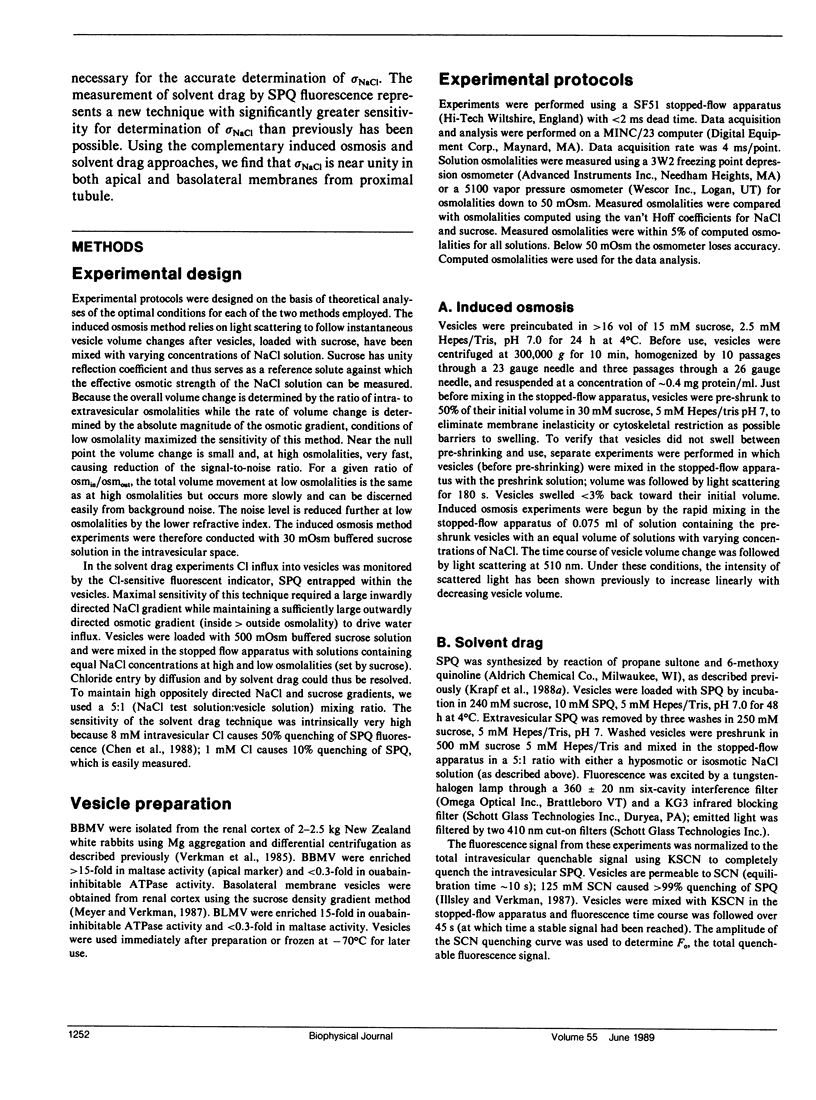
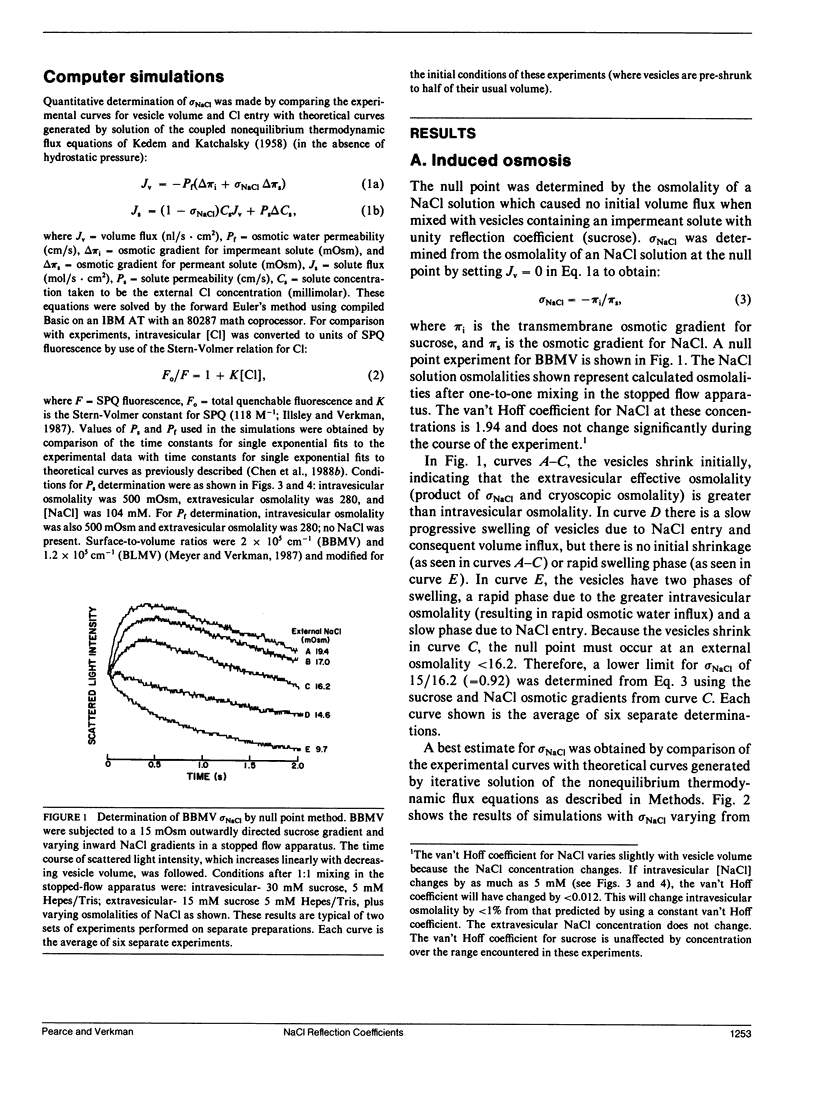
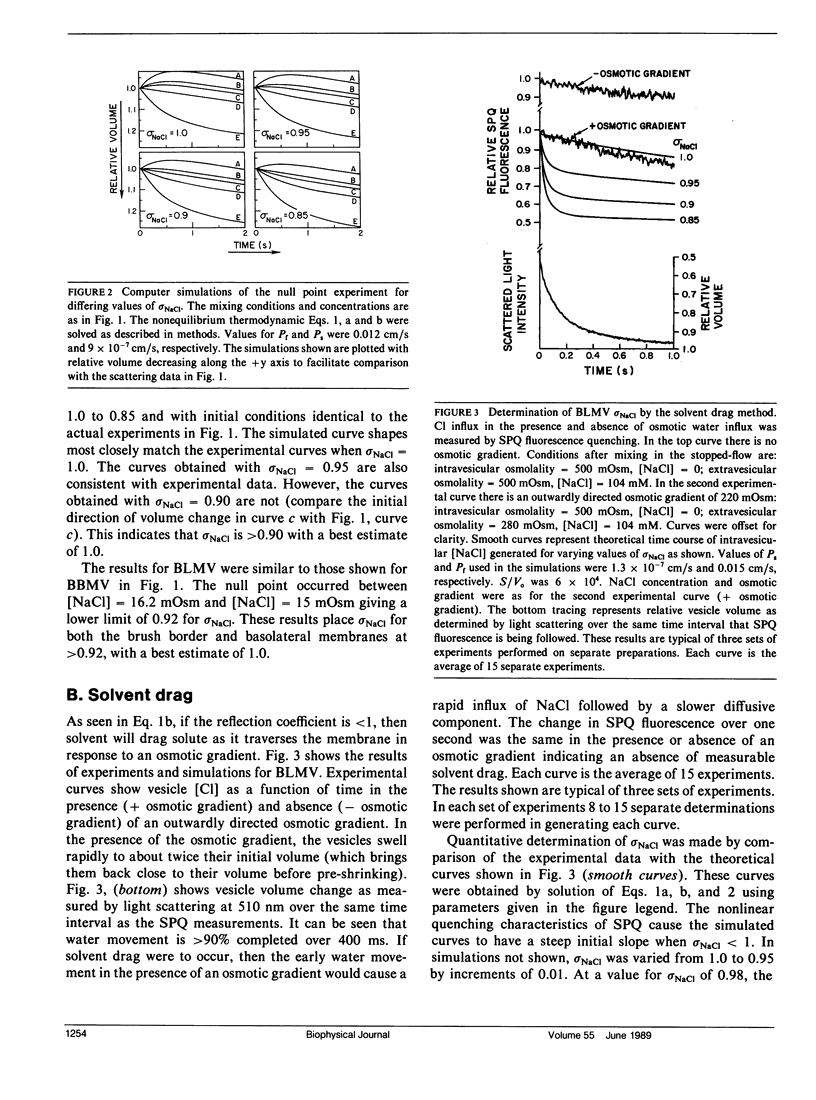
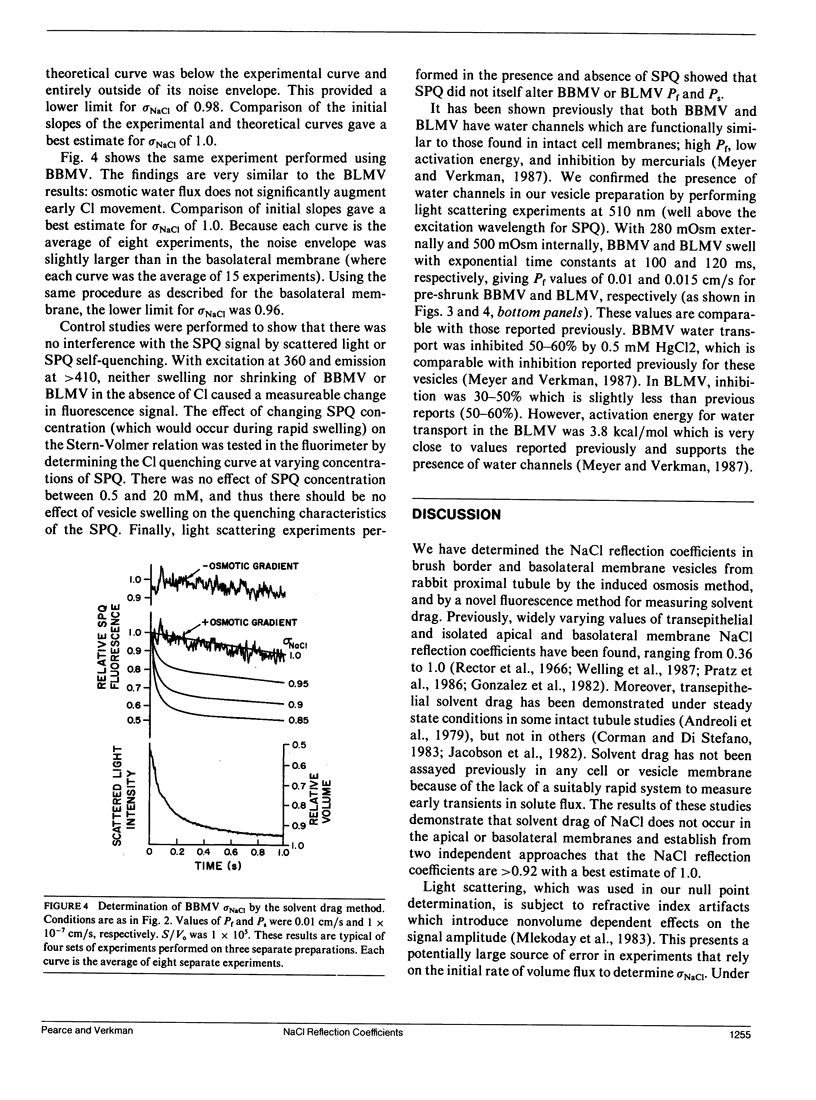
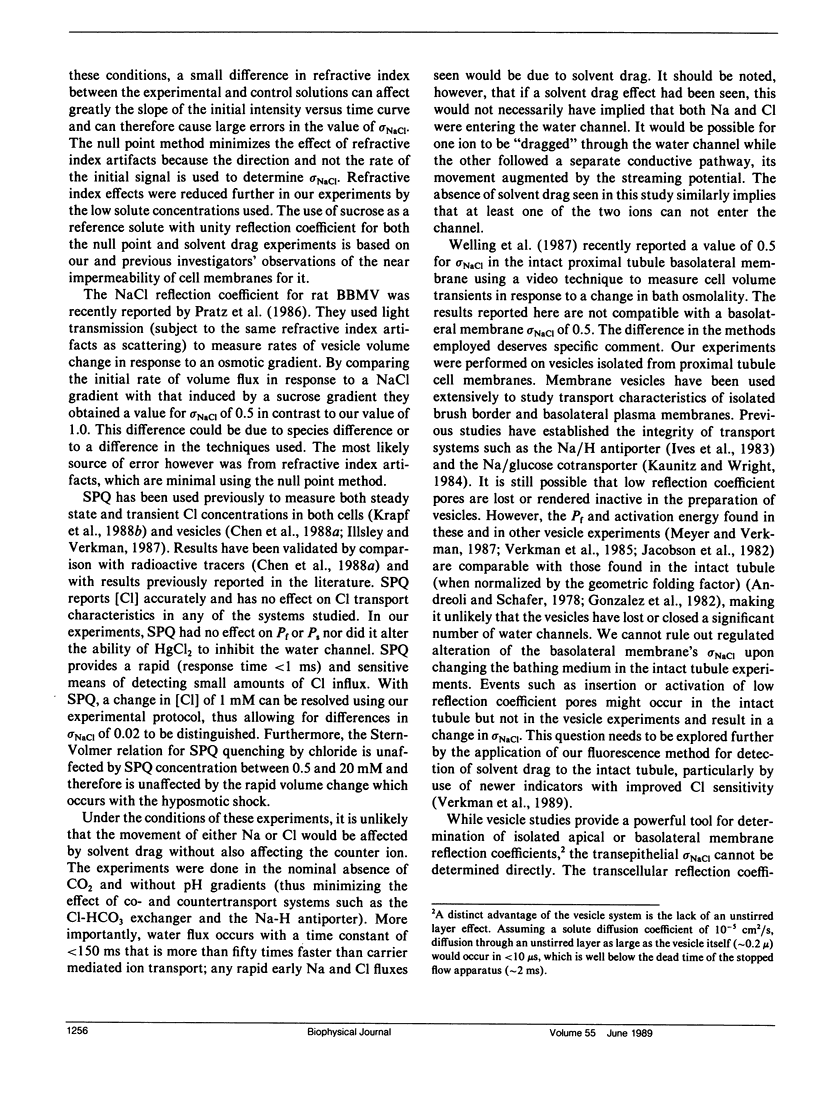
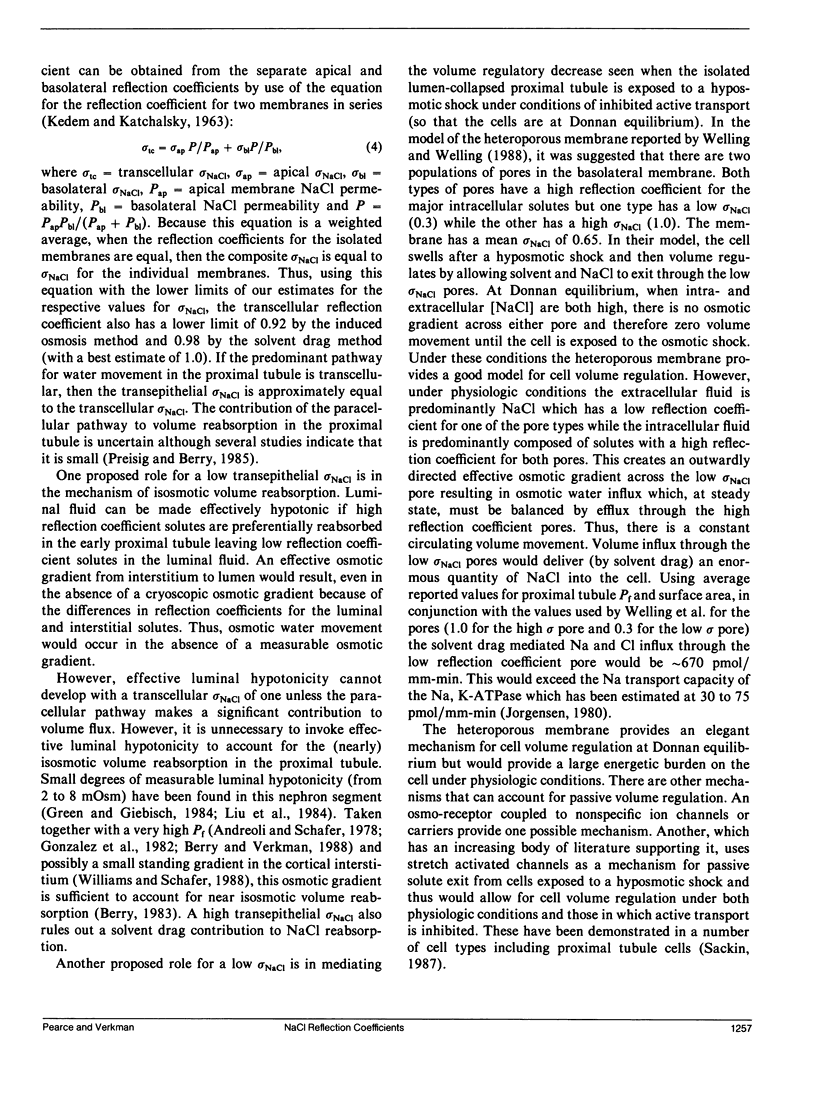
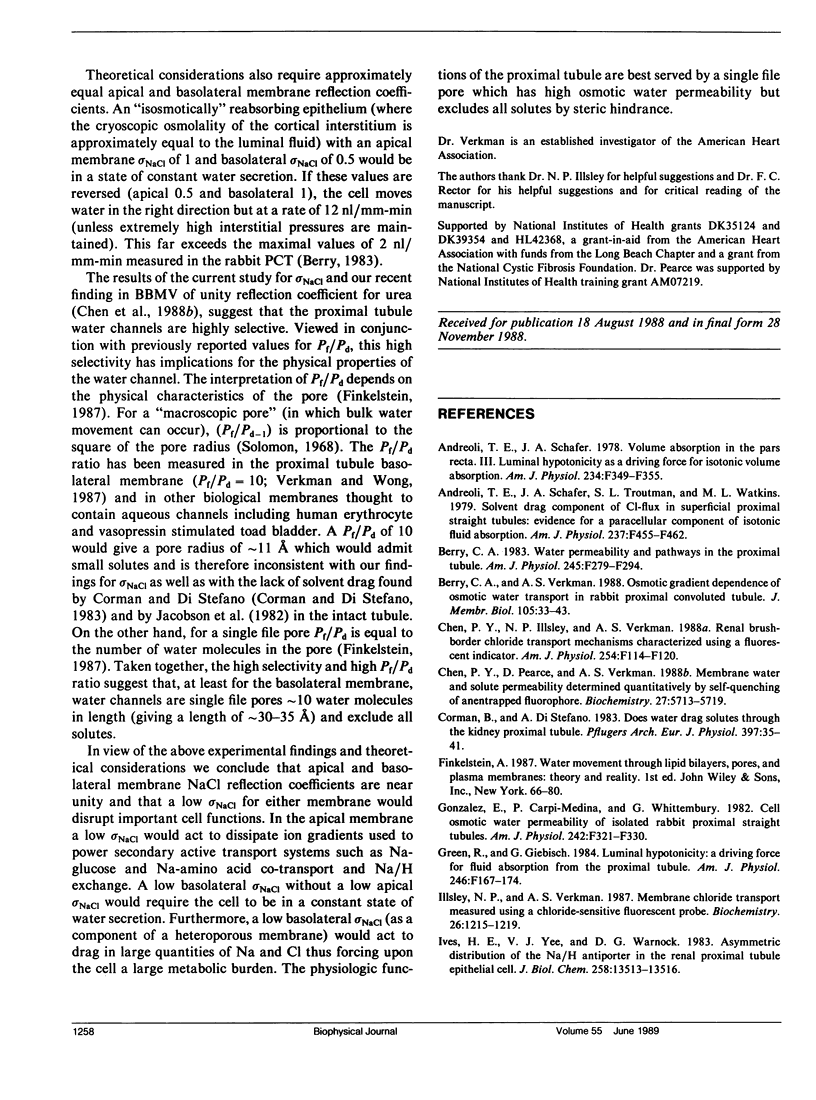
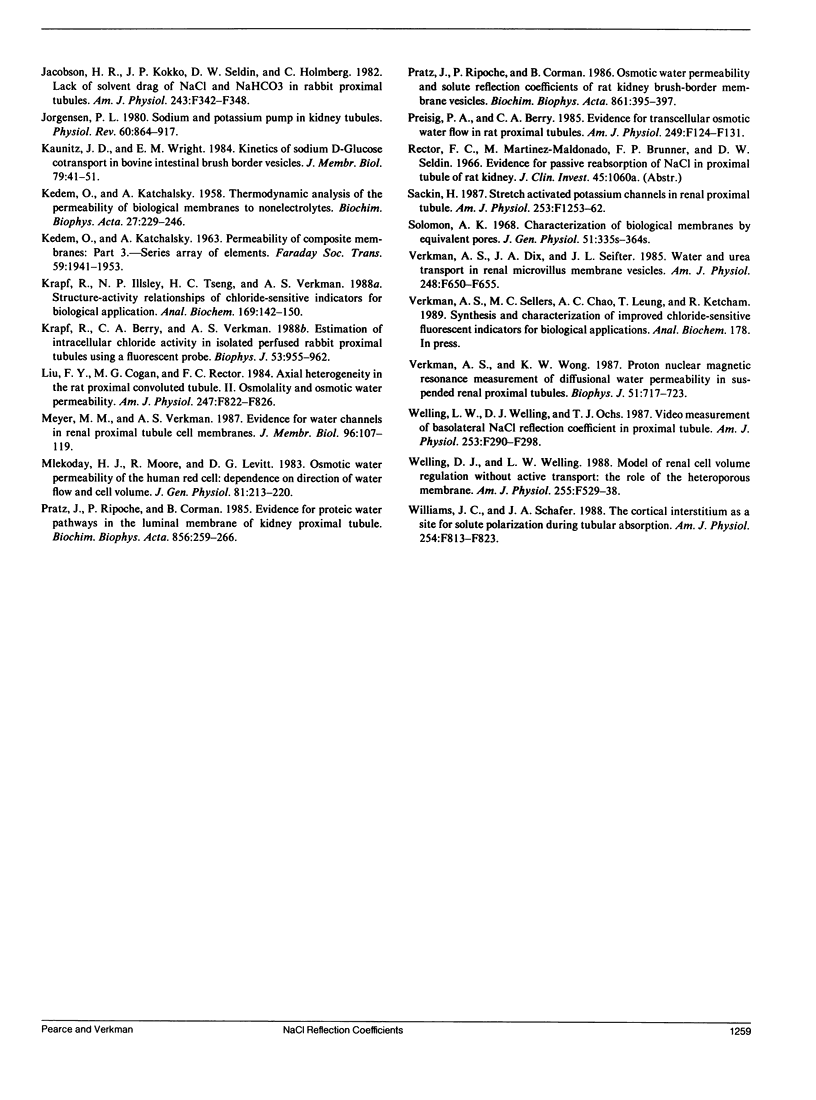
Selected References
These references are in PubMed. This may not be the complete list of references from this article.
- Andreoli T. E., Schafer J. A., Troutman S. L., Watkins M. L. Solvent drag component of Cl- flux in superficial proximal straight tubules: evidence for a paracellular component of isotonic fluid absorption. Am J Physiol. 1979 Dec;237(6):F455–F462. doi: 10.1152/ajprenal.1979.237.6.F455. [DOI] [PubMed] [Google Scholar]
- Andreoli T. E., Schafer J. A. Volume absorption in the pars recta. III. Luminal hypotonicity as a driving force for isotonic volume absorption. Am J Physiol. 1978 Apr;234(4):F349–F355. doi: 10.1152/ajprenal.1978.234.4.F349. [DOI] [PubMed] [Google Scholar]
- Berry C. A., Verkman A. S. Osmotic gradient dependence of osmotic water permeability in rabbit proximal convoluted tubule. J Membr Biol. 1988 Oct;105(1):33–43. doi: 10.1007/BF01871104. [DOI] [PubMed] [Google Scholar]
- Berry C. A. Water permeability and pathways in the proximal tubule. Am J Physiol. 1983 Sep;245(3):F279–F294. doi: 10.1152/ajprenal.1983.245.3.F279. [DOI] [PubMed] [Google Scholar]
- Chen P. Y., Illsley N. P., Verkman A. S. Renal brush-border chloride transport mechanisms characterized using a fluorescent indicator. Am J Physiol. 1988 Jan;254(1 Pt 2):F114–F120. doi: 10.1152/ajprenal.1988.254.1.F114. [DOI] [PubMed] [Google Scholar]
- Chen P. Y., Pearce D., Verkman A. S. Membrane water and solute permeability determined quantitatively by self-quenching of an entrapped fluorophore. Biochemistry. 1988 Jul 26;27(15):5713–5718. doi: 10.1021/bi00415a048. [DOI] [PubMed] [Google Scholar]
- Corman B., Di Stefano A. Does water drag solutes through kidney proximal tubule? Pflugers Arch. 1983 Apr;397(1):35–41. doi: 10.1007/BF00585165. [DOI] [PubMed] [Google Scholar]
- González E., Carpi-Medina P., Whittembury G. Cell osmotic water permeability of isolated rabbit proximal straight tubules. Am J Physiol. 1982 Apr;242(4):F321–F330. doi: 10.1152/ajprenal.1982.242.4.F321. [DOI] [PubMed] [Google Scholar]
- Green R., Giebisch G. Luminal hypotonicity: a driving force for fluid absorption from the proximal tubule. Am J Physiol. 1984 Feb;246(2 Pt 2):F167–F174. doi: 10.1152/ajprenal.1984.246.2.F167. [DOI] [PubMed] [Google Scholar]
- Illsley N. P., Verkman A. S. Membrane chloride transport measured using a chloride-sensitive fluorescent probe. Biochemistry. 1987 Mar 10;26(5):1215–1219. doi: 10.1021/bi00379a002. [DOI] [PubMed] [Google Scholar]
- Ives H. E., Yee V. J., Warnock D. G. Asymmetric distribution of the Na+/H+ antiporter in the renal proximal tubule epithelial cell. J Biol Chem. 1983 Nov 25;258(22):13513–13516. [PubMed] [Google Scholar]
- Jacobson H. R., Kokko J. P., Seldin D. W., Holmberg C. Lack of solvent drag of NaCl and NaHCO3 in rabbit proximal tubules. Am J Physiol. 1982 Oct;243(4):F342–F348. doi: 10.1152/ajprenal.1982.243.4.F342. [DOI] [PubMed] [Google Scholar]
- Jørgensen P. L. Sodium and potassium ion pump in kidney tubules. Physiol Rev. 1980 Jul;60(3):864–917. doi: 10.1152/physrev.1980.60.3.864. [DOI] [PubMed] [Google Scholar]
- KEDEM O., KATCHALSKY A. Thermodynamic analysis of the permeability of biological membranes to non-electrolytes. Biochim Biophys Acta. 1958 Feb;27(2):229–246. doi: 10.1016/0006-3002(58)90330-5. [DOI] [PubMed] [Google Scholar]
- Kaunitz J. D., Wright E. M. Kinetics of sodium D-glucose cotransport in bovine intestinal brush border vesicles. J Membr Biol. 1984;79(1):41–51. doi: 10.1007/BF01868525. [DOI] [PubMed] [Google Scholar]
- Krapf R., Berry C. A., Verkman A. S. Estimation of intracellular chloride activity in isolated perfused rabbit proximal convoluted tubules using a fluorescent indicator. Biophys J. 1988 Jun;53(6):955–962. doi: 10.1016/S0006-3495(88)83176-X. [DOI] [PMC free article] [PubMed] [Google Scholar]
- Krapf R., Illsley N. P., Tseng H. C., Verkman A. S. Structure-activity relationships of chloride-sensitive fluorescent indicators for biological application. Anal Biochem. 1988 Feb 15;169(1):142–150. doi: 10.1016/0003-2697(88)90265-5. [DOI] [PubMed] [Google Scholar]
- Liu F. Y., Cogan M. G., Rector F. C., Jr Axial heterogeneity in the rat proximal convoluted tubule. II. Osmolality and osmotic water permeability. Am J Physiol. 1984 Nov;247(5 Pt 2):F822–F826. doi: 10.1152/ajprenal.1984.247.5.F822. [DOI] [PubMed] [Google Scholar]
- Meyer M. M., Verkman A. S. Evidence for water channels in renal proximal tubule cell membranes. J Membr Biol. 1987;96(2):107–119. doi: 10.1007/BF01869237. [DOI] [PubMed] [Google Scholar]
- Mlekoday H. J., Moore R., Levitt D. G. Osmotic water permeability of the human red cell. Dependence on direction of water flow and cell volume. J Gen Physiol. 1983 Feb;81(2):213–220. doi: 10.1085/jgp.81.2.213. [DOI] [PMC free article] [PubMed] [Google Scholar]
- Pratz J., Ripoche P., Corman B. Evidence for proteic water pathways in the luminal membrane of kidney proximal tubule. Biochim Biophys Acta. 1986 Apr 14;856(2):259–266. doi: 10.1016/0005-2736(86)90035-0. [DOI] [PubMed] [Google Scholar]
- Pratz J., Ripoche P., Corman B. Osmotic water permeability and solute reflection coefficients of rat kidney brush-border membrane vesicles. Biochim Biophys Acta. 1986 Oct 9;861(2):395–397. doi: 10.1016/0005-2736(86)90446-3. [DOI] [PubMed] [Google Scholar]
- Preisig P. A., Berry C. A. Evidence for transcellular osmotic water flow in rat proximal tubules. Am J Physiol. 1985 Jul;249(1 Pt 2):F124–F131. doi: 10.1152/ajprenal.1985.249.1.F124. [DOI] [PubMed] [Google Scholar]
- Sackin H. Stretch-activated potassium channels in renal proximal tubule. Am J Physiol. 1987 Dec;253(6 Pt 2):F1253–F1262. doi: 10.1152/ajprenal.1987.253.6.F1253. [DOI] [PubMed] [Google Scholar]
- Solomon A. K. Characterization of biological membranes by equivalent pores. J Gen Physiol. 1968 May;51(5 Suppl):335S+–335S+. [PubMed] [Google Scholar]
- Verkman A. S., Dix J. A., Seifter J. L. Water and urea transport in renal microvillus membrane vesicles. Am J Physiol. 1985 May;248(5 Pt 2):F650–F655. doi: 10.1152/ajprenal.1985.248.5.F650. [DOI] [PubMed] [Google Scholar]
- Verkman A. S., Wong K. R. Proton nuclear magnetic resonance measurement of diffusional water permeability in suspended renal proximal tubules. Biophys J. 1987 May;51(5):717–723. doi: 10.1016/S0006-3495(87)83398-2. [DOI] [PMC free article] [PubMed] [Google Scholar]
- Welling D. J., Welling L. W. Model of renal cell volume regulation without active transport: role of a heteroporous membrane. Am J Physiol. 1988 Sep;255(3 Pt 2):F529–F538. doi: 10.1152/ajprenal.1988.255.3.F529. [DOI] [PubMed] [Google Scholar]
- Welling L. W., Welling D. J., Ochs T. J. Video measurement of basolateral NaCl reflection coefficient in proximal tubule. Am J Physiol. 1987 Aug;253(2 Pt 2):F290–F298. doi: 10.1152/ajprenal.1987.253.2.F290. [DOI] [PubMed] [Google Scholar]
- Williams J. C., Jr, Schafer J. A. Cortical interstitium as a site for solute polarization during tubular absorption. Am J Physiol. 1988 Jun;254(6 Pt 2):F813–F823. doi: 10.1152/ajprenal.1988.254.6.F813. [DOI] [PubMed] [Google Scholar]