Abstract
1. Intra-axonal organelles were detected by darkfield and Nomarski microscopy in isolated myelinated nerve fibres from Xenopus laevis. Nerve fibres from the 8th spinal roots, the sciatic nerve, and identified motor and sensory axons from other hind limb nerves were used. The movement of the organelles was recorded either on motion picture film or by noting the times at which they crossed the lines of an ocular grid.
2. Three groups of organelles were detected in all fibres. A group of particles with round profiles 0·2-0·5 μm in diameter moved somatopetally. Another group of round particles moved somatofugally. The ratio of the number of somatopetally travelling particles to the number of somatofugally travelling particles was about 10:1. The third group of organelles consisted of rod-shaped bodies about 0·2-0·3 μm in diameter and 1-8 μm in length; these were usually stationary.
3. All the round particles appeared to move independently of each other with a saltatory motion. The somatopetally and somatofugally travelling particles had statistically different mean velocities of 0·98 and 1·32 μm/sec respectively.
4. Round particles often crossed the node of Ranvier with no appreciable change in velocity. Some, however, were temporarily arrested at the entrance to the node.
5. While the rod-shaped organelles were usually stationary, they occasionally moved rapidly lengthwise for distances of up to 10 μm. Rarely a rod-shaped organelle exhibited a continuous saltatory motion.
6. Round particles often travelled in either direction along the edge of rod-shaped organelles. One rod was observed to move along the path previously taken by a round particle.
7. The findings are discussed with respect to (a) the normality of the preparations, (b) the numbers of particles travelling in each direction, (c) the nature of the organelles, and (d) the mechanisms underlying the motion.
8. We suggest that particles move along microtubules which have specific directionalities and particle affinities. The microtubules are in bundles and are closely associated with rod-shaped mitochondria.
Full text
PDF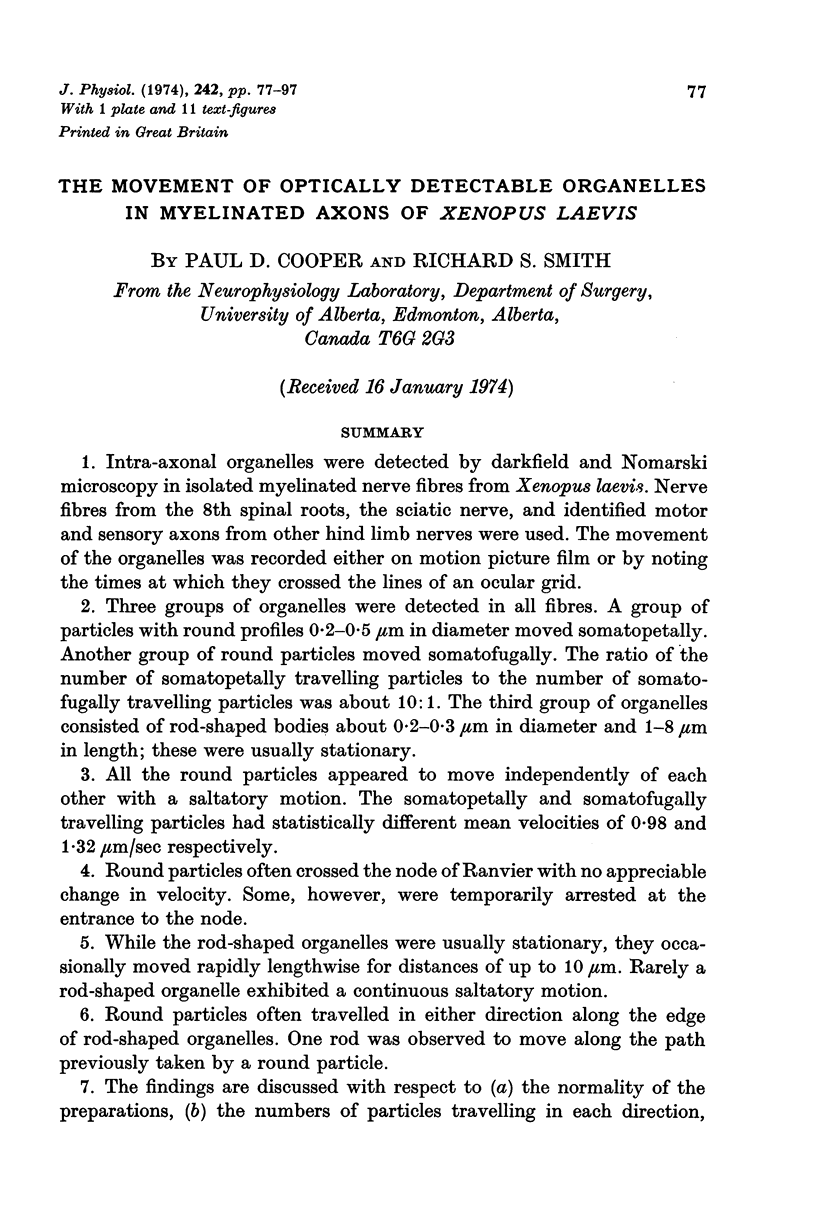
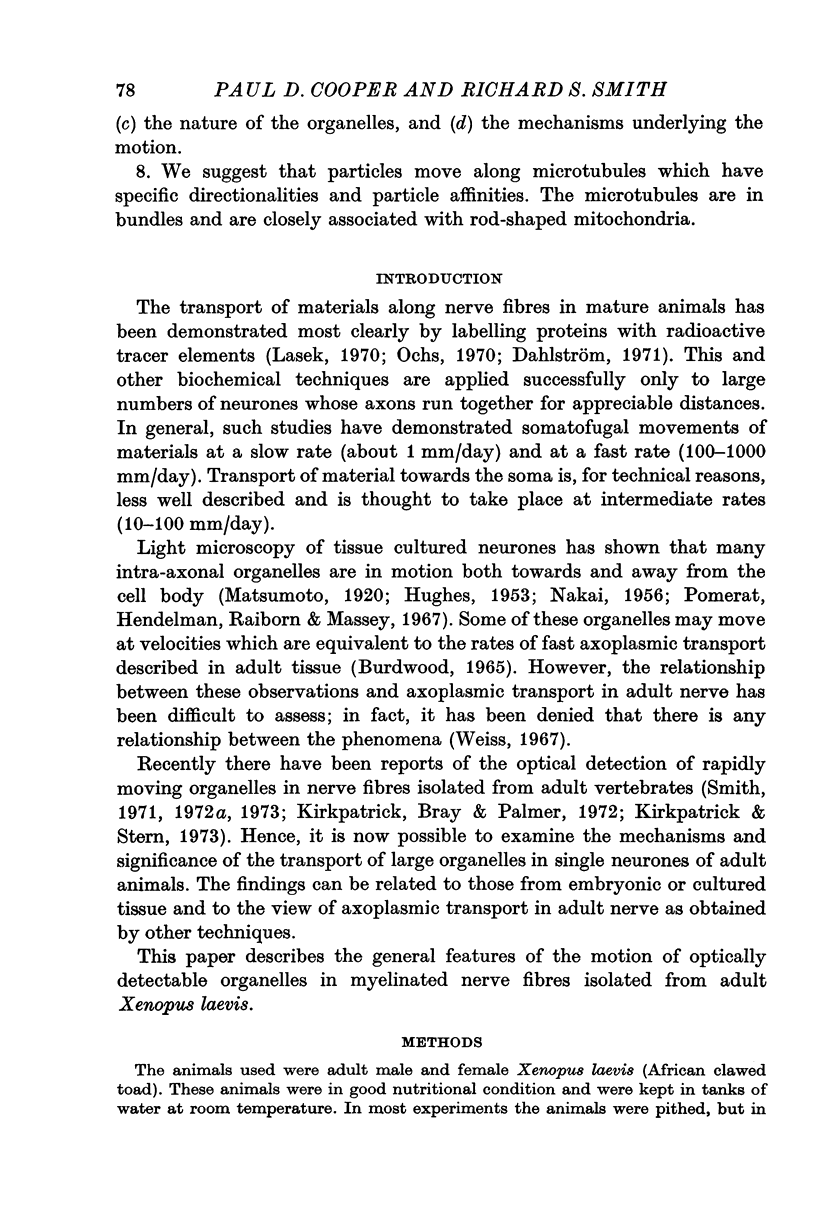
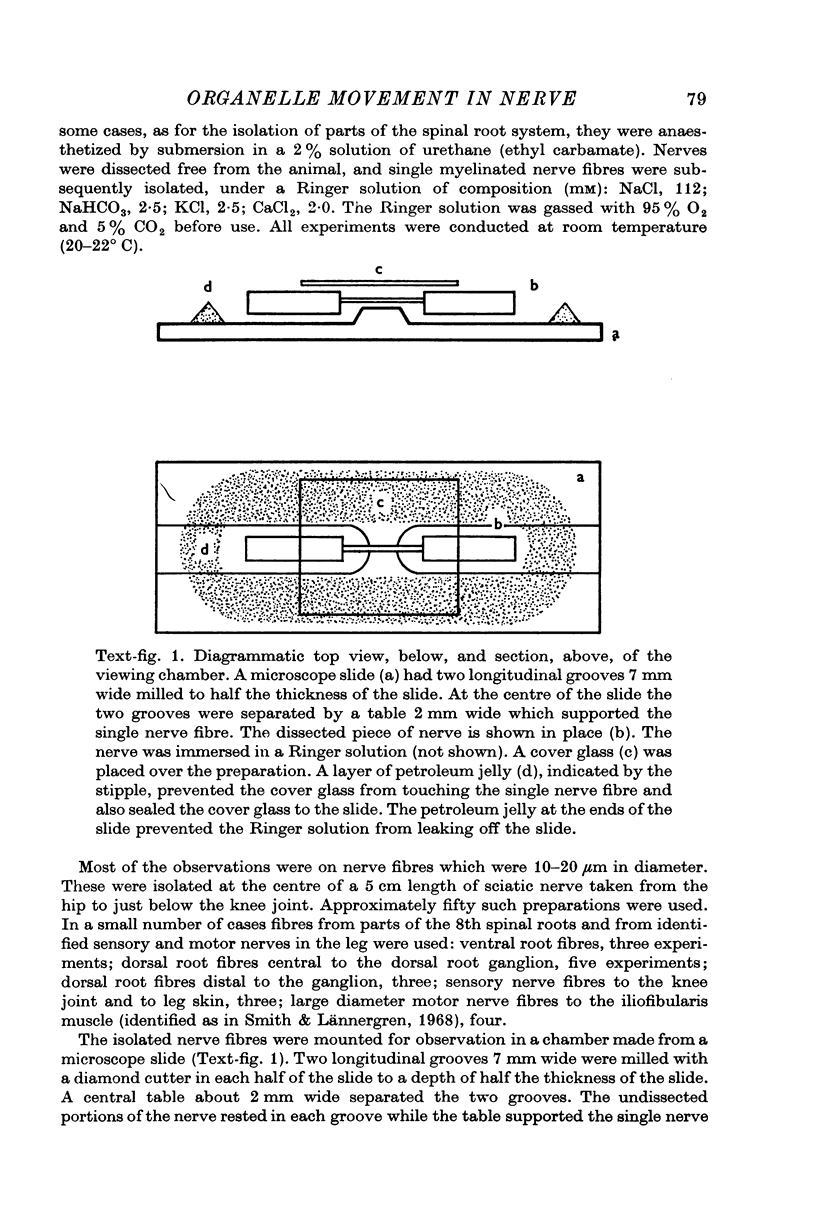
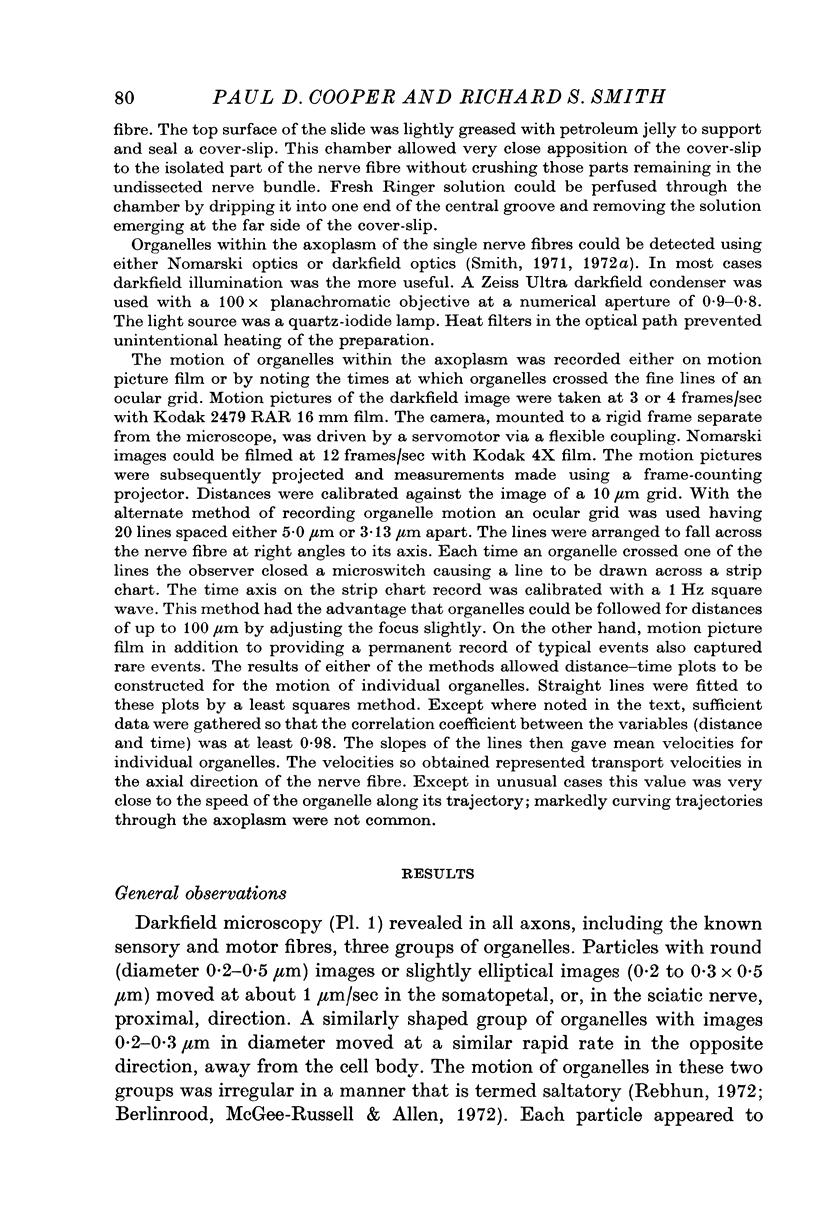
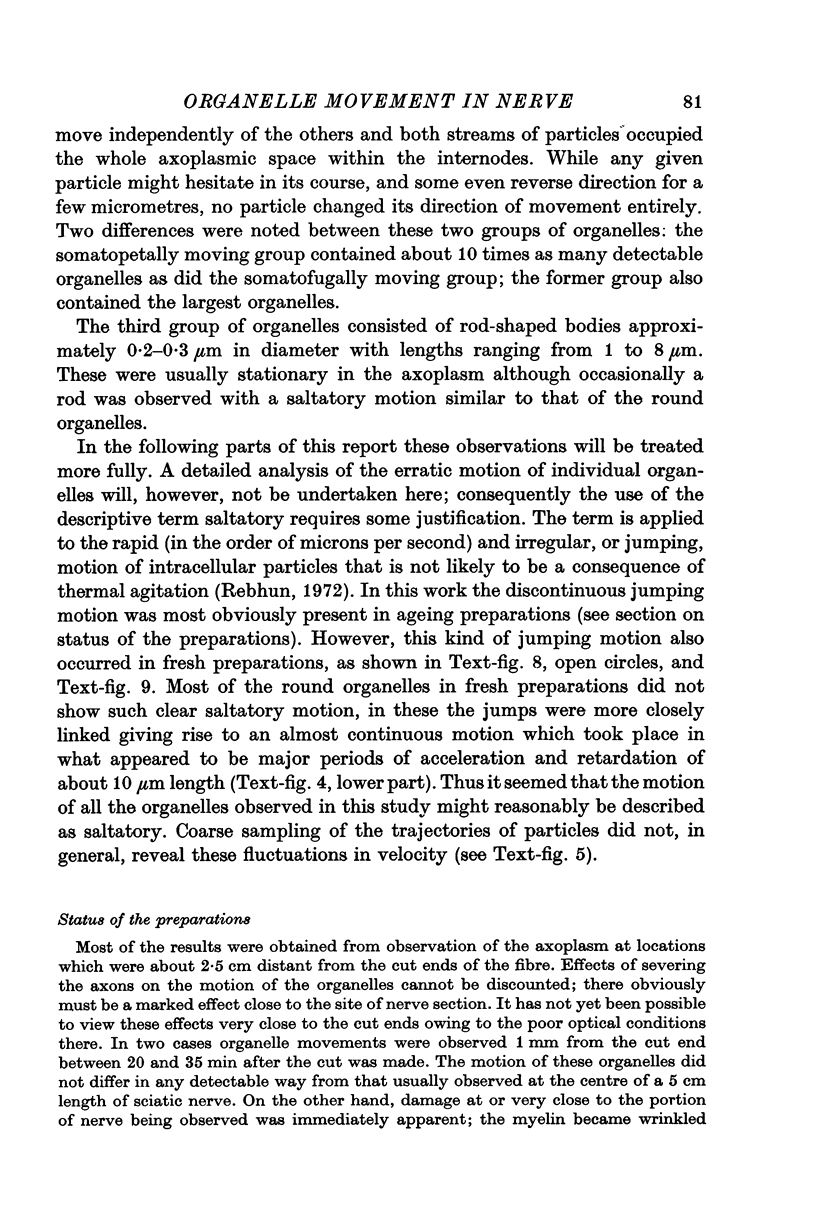
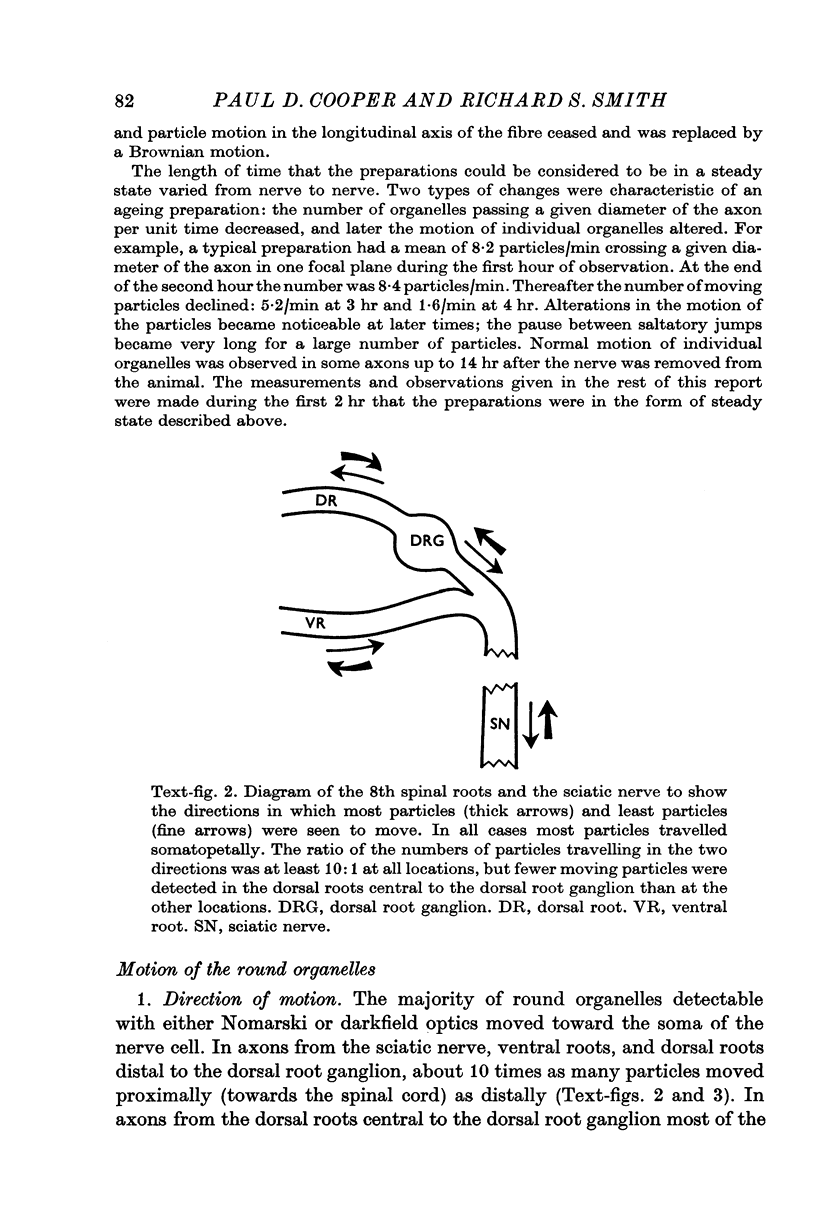
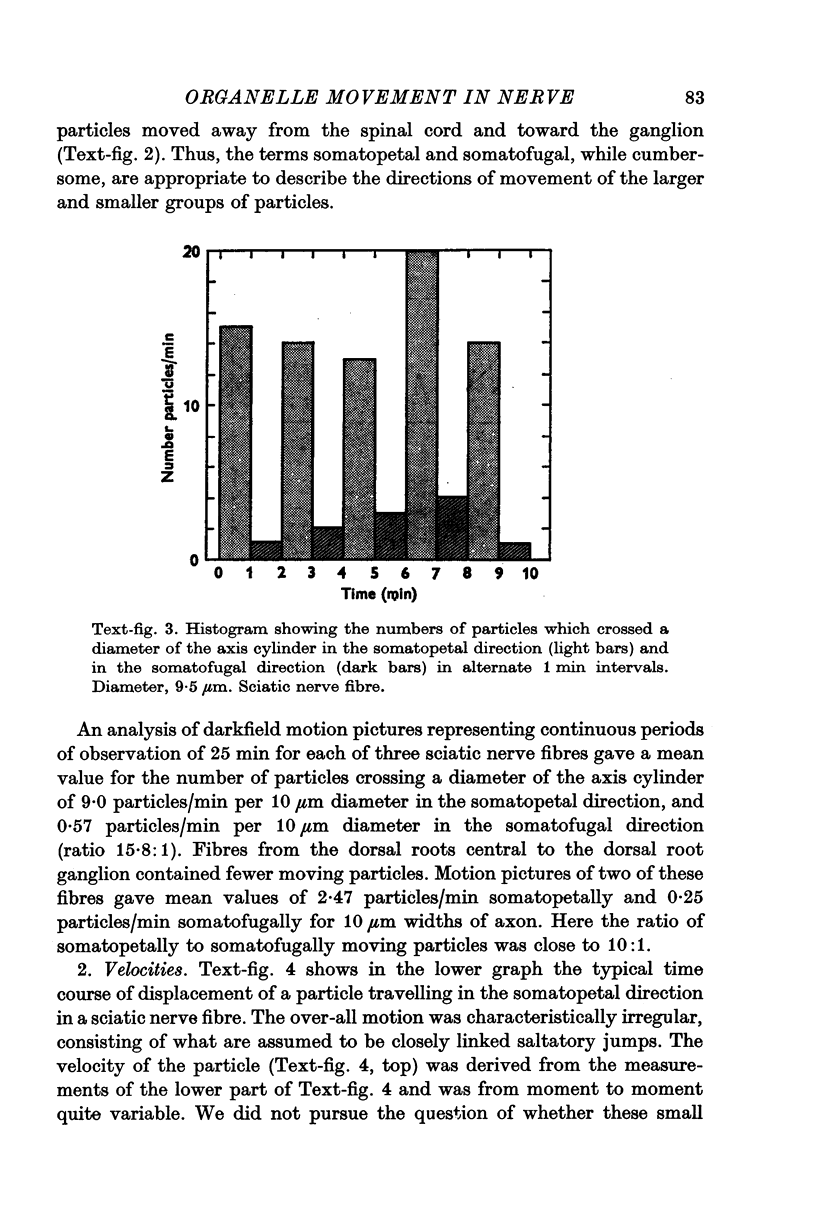
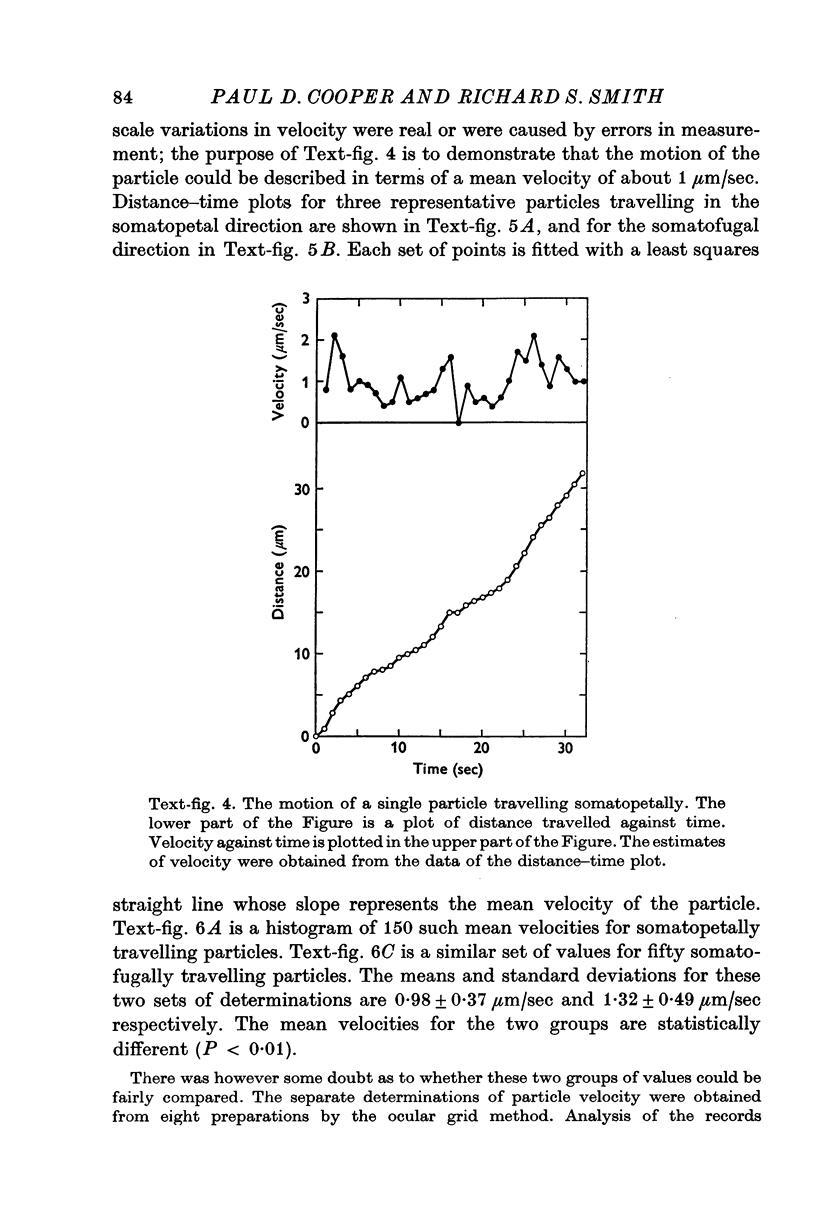
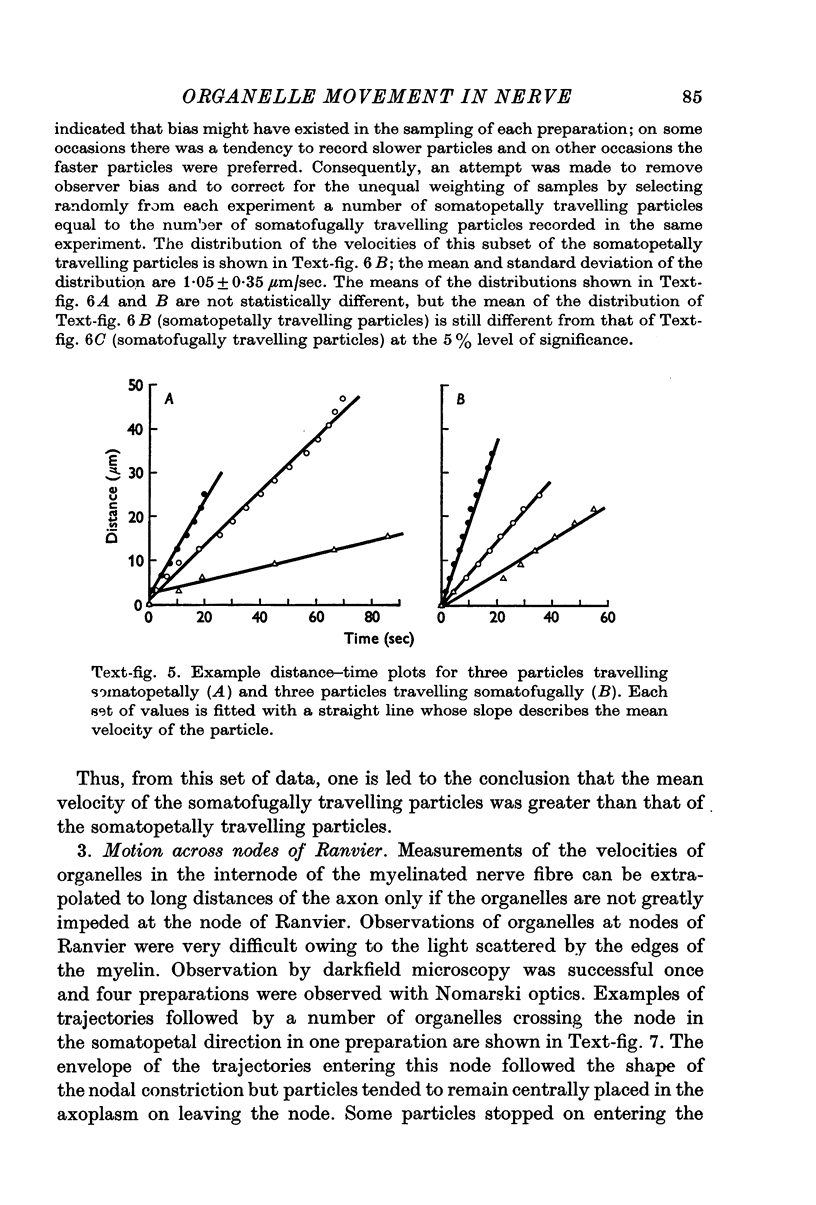
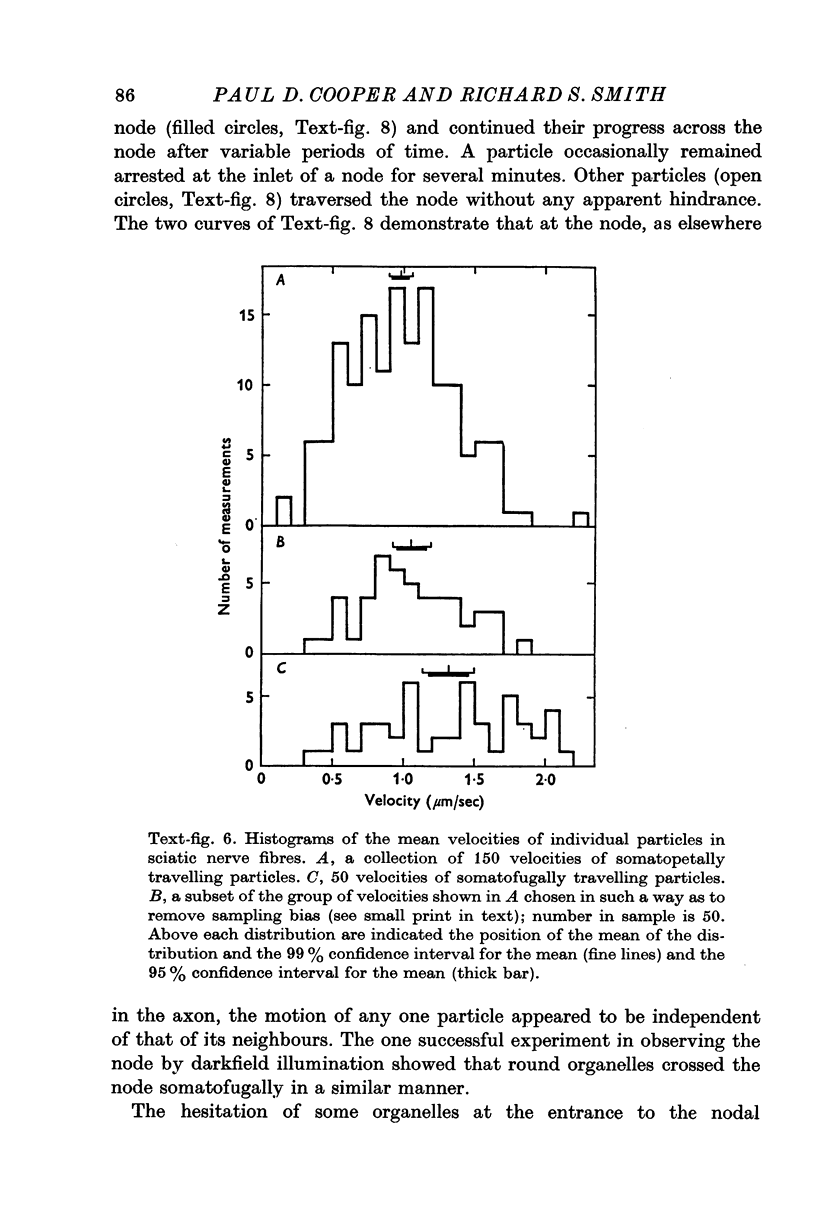
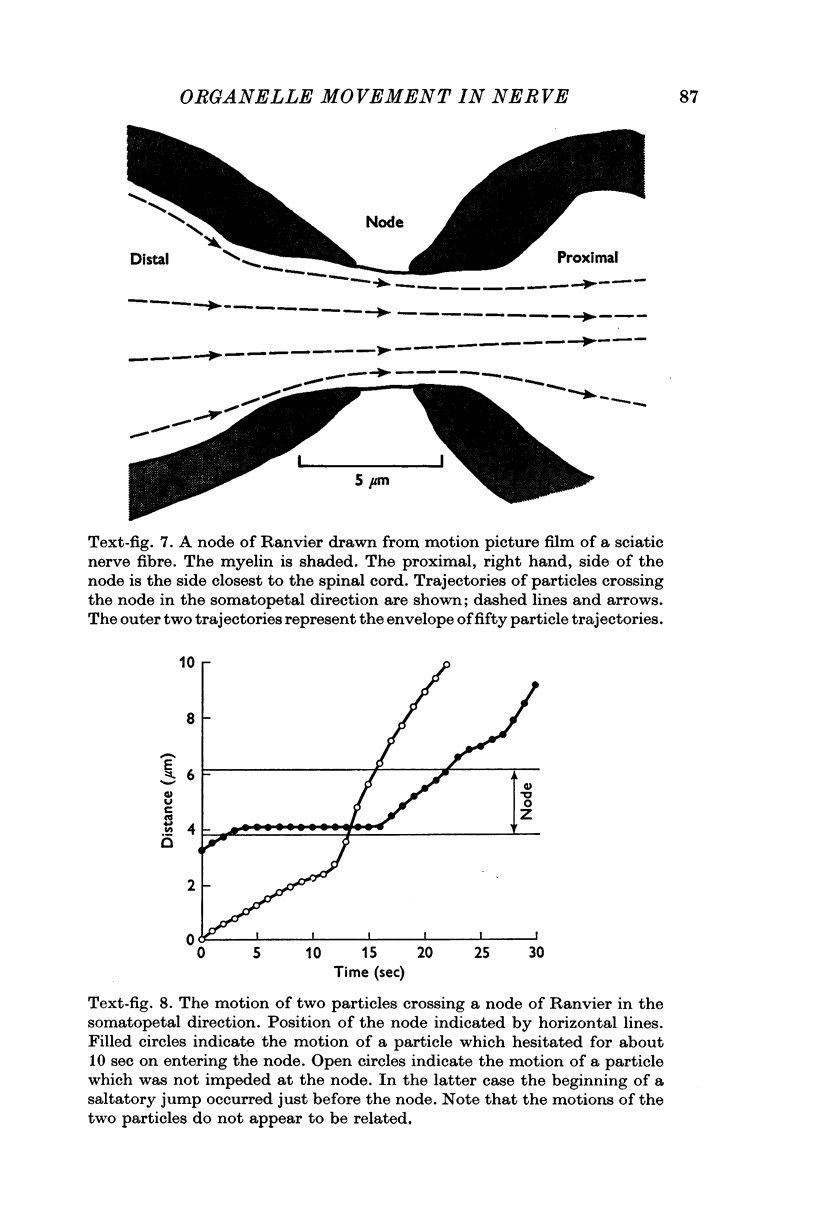
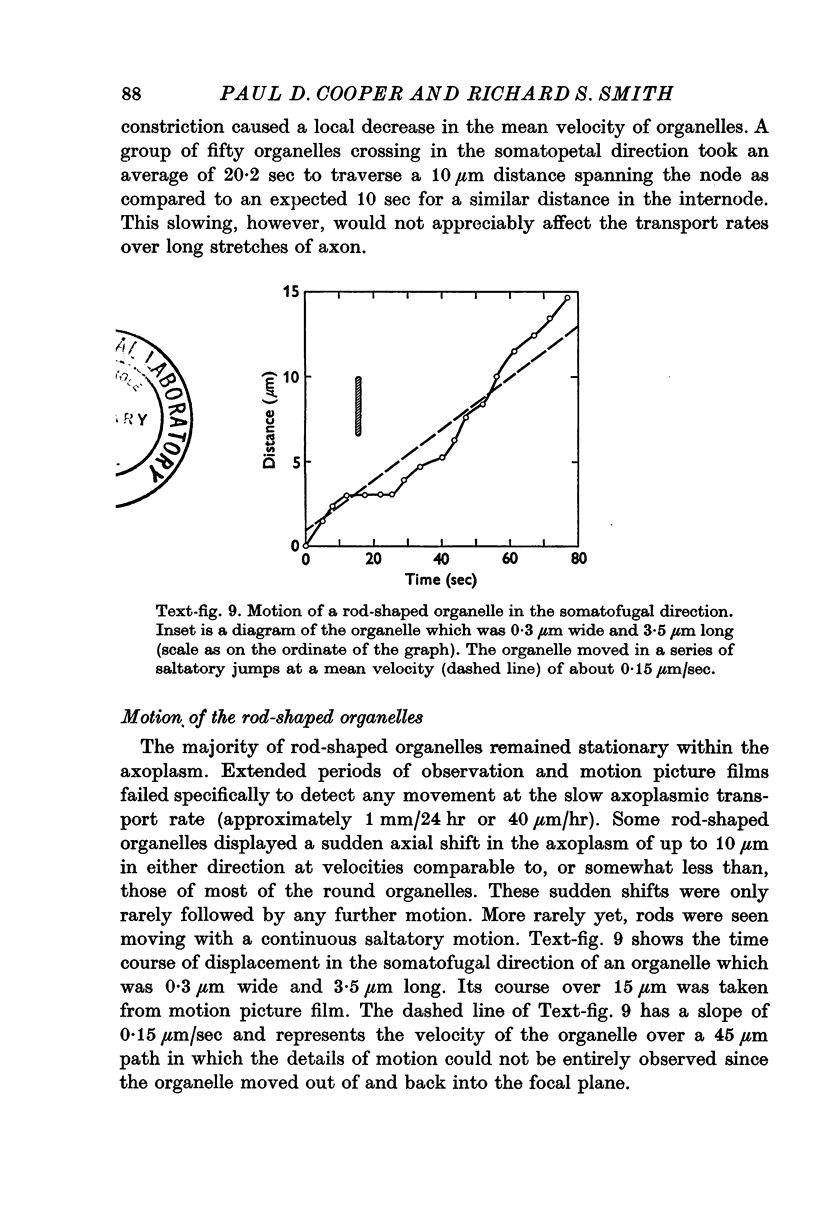
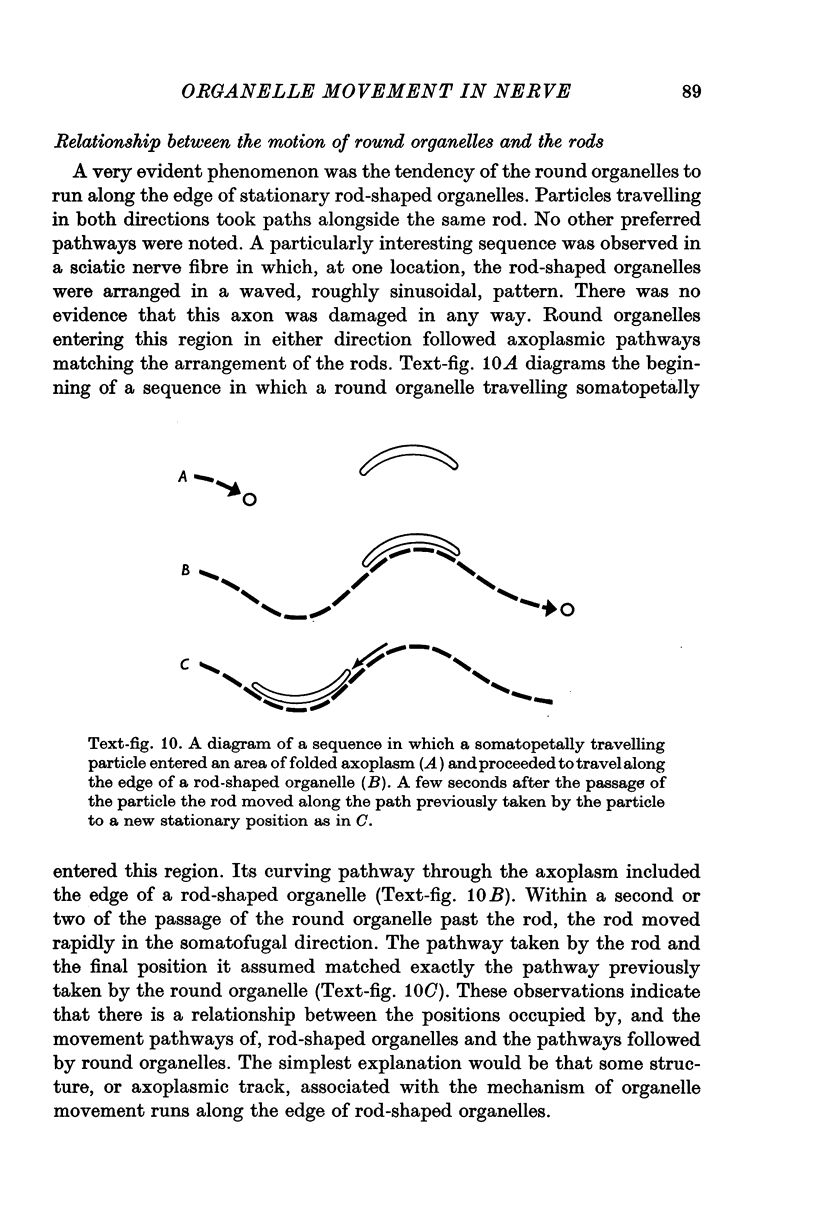
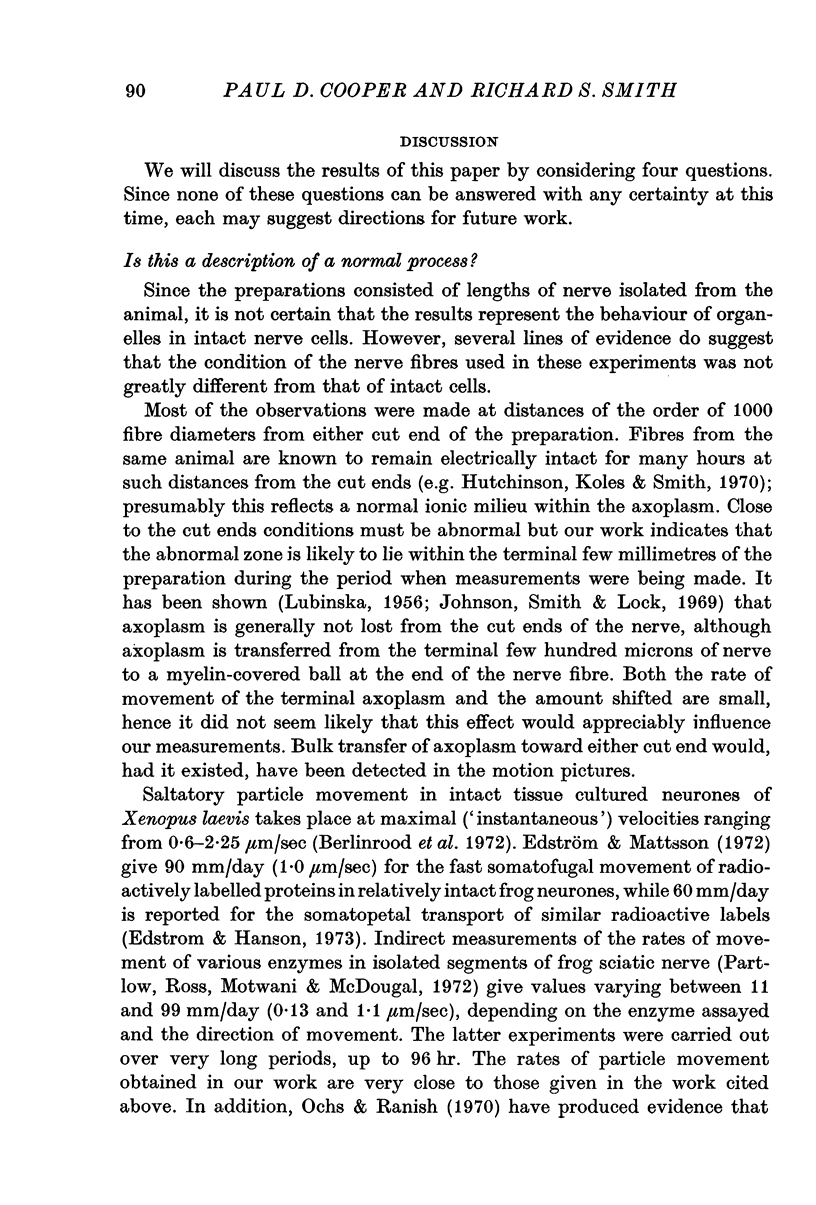
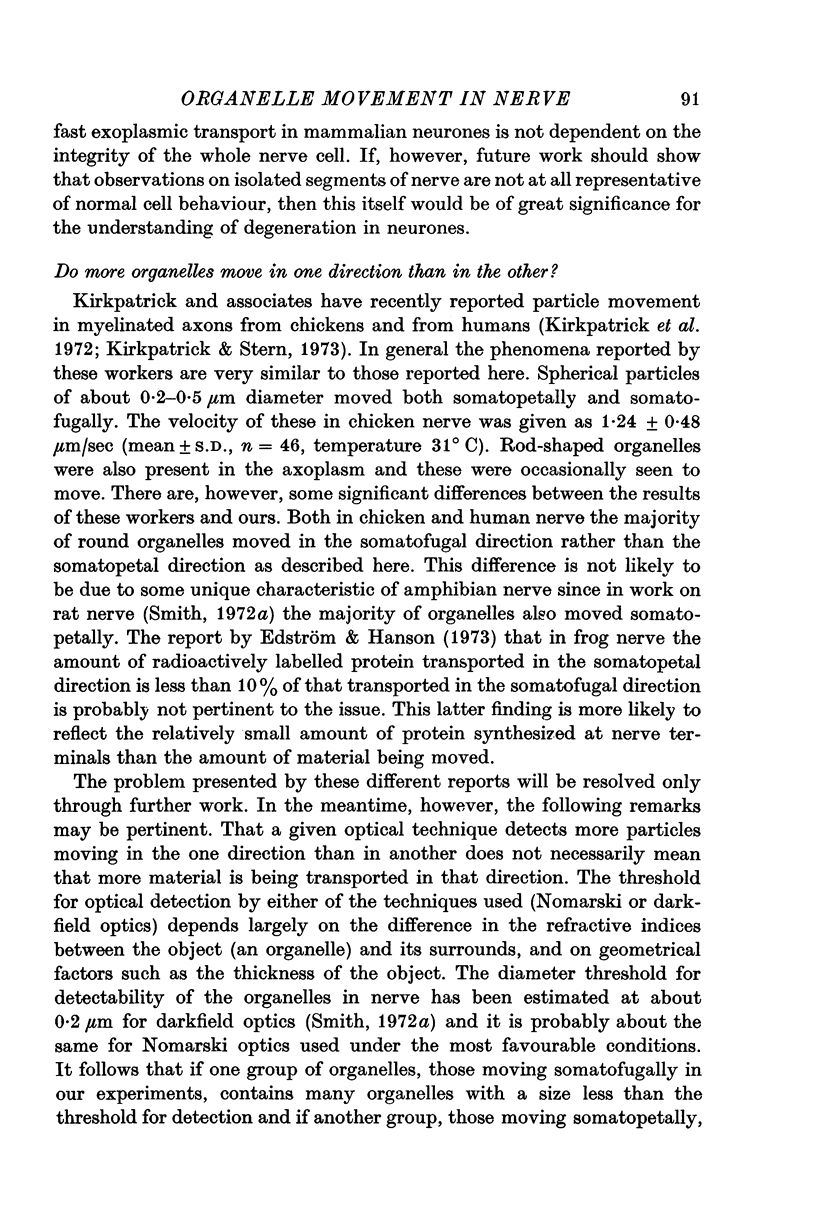
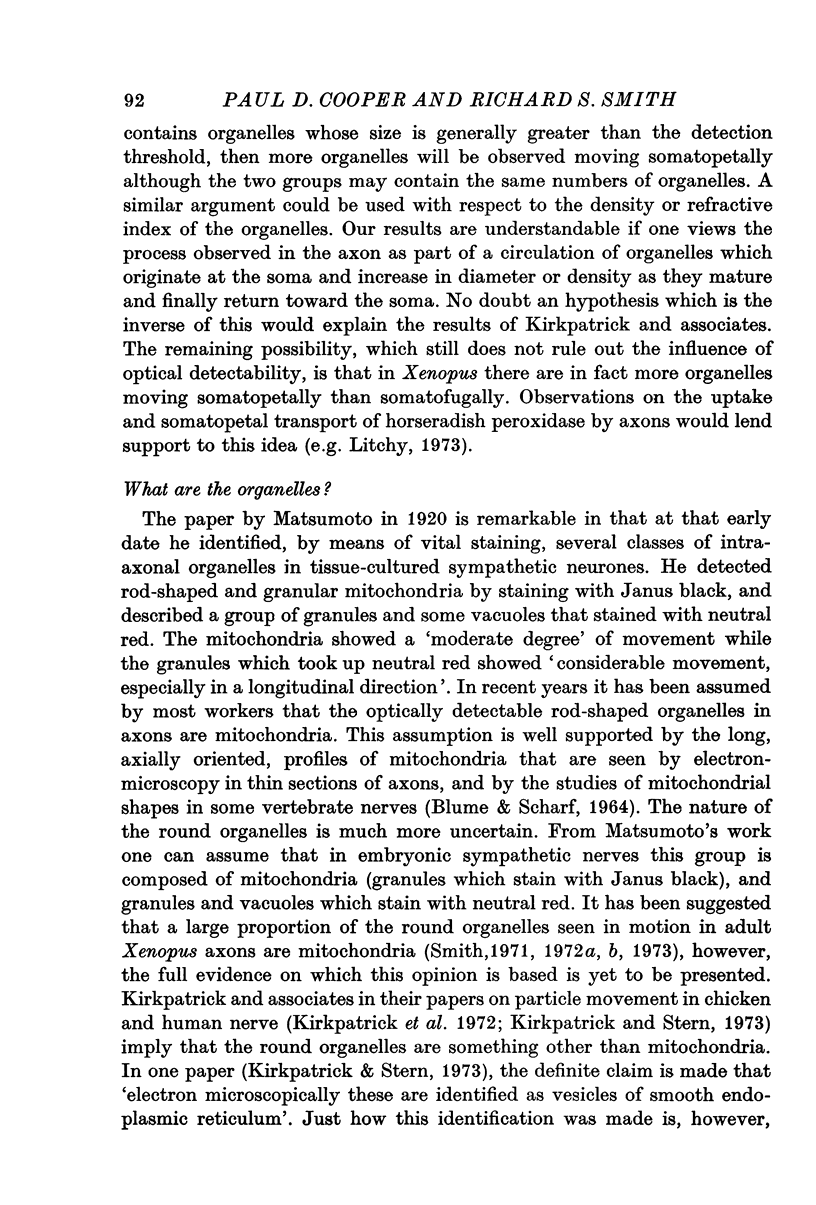
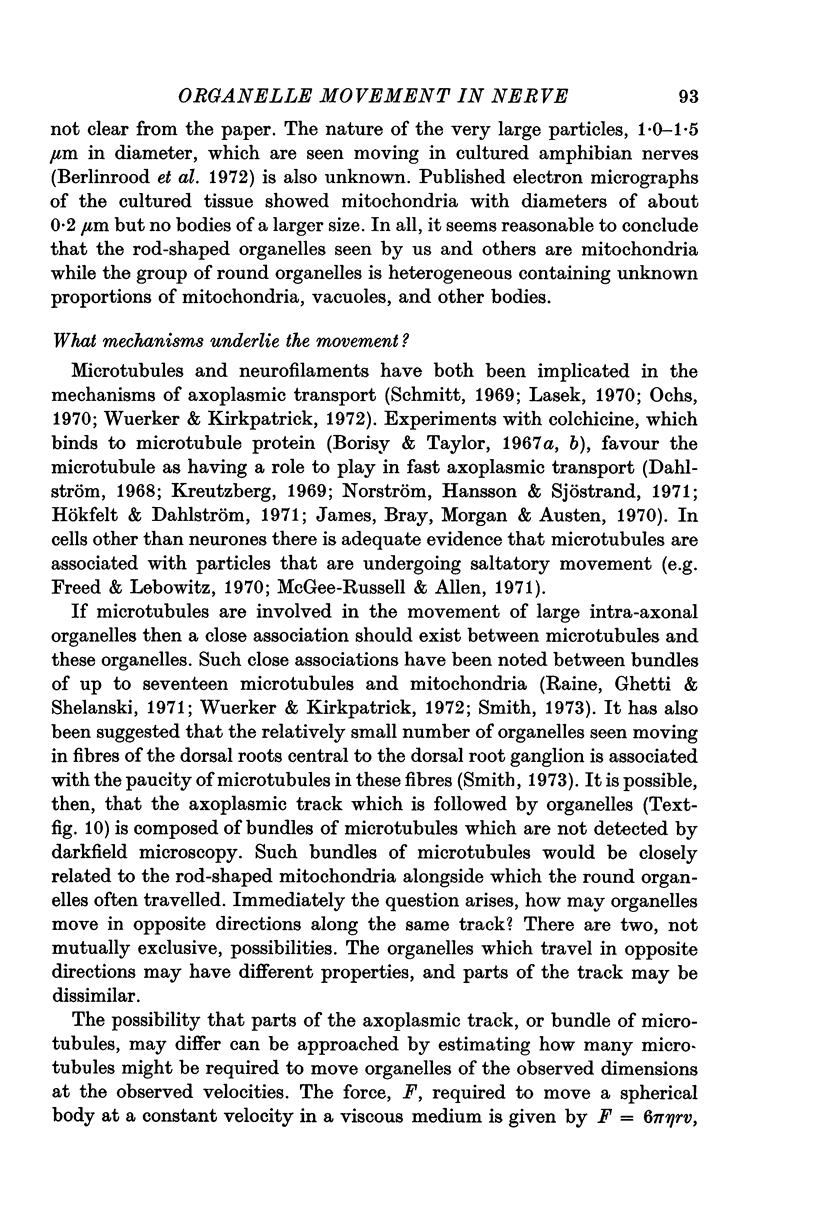
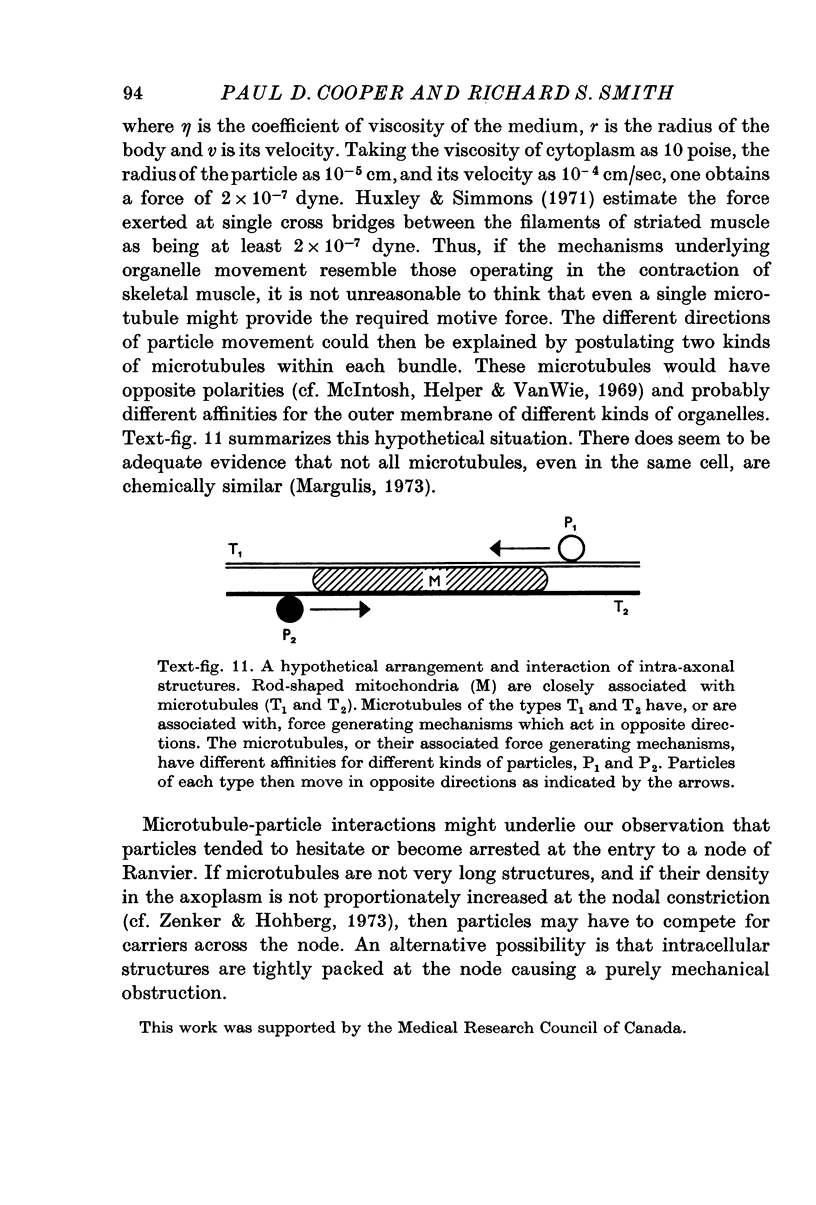
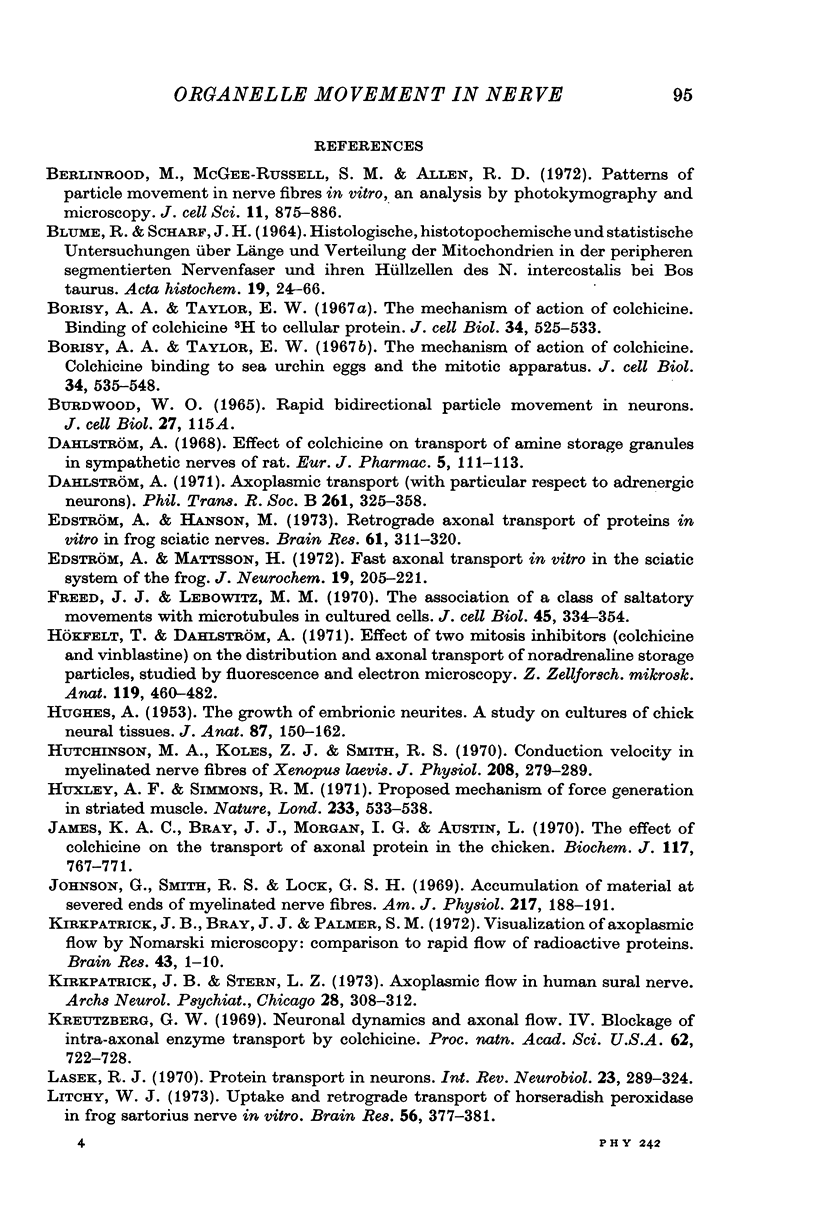
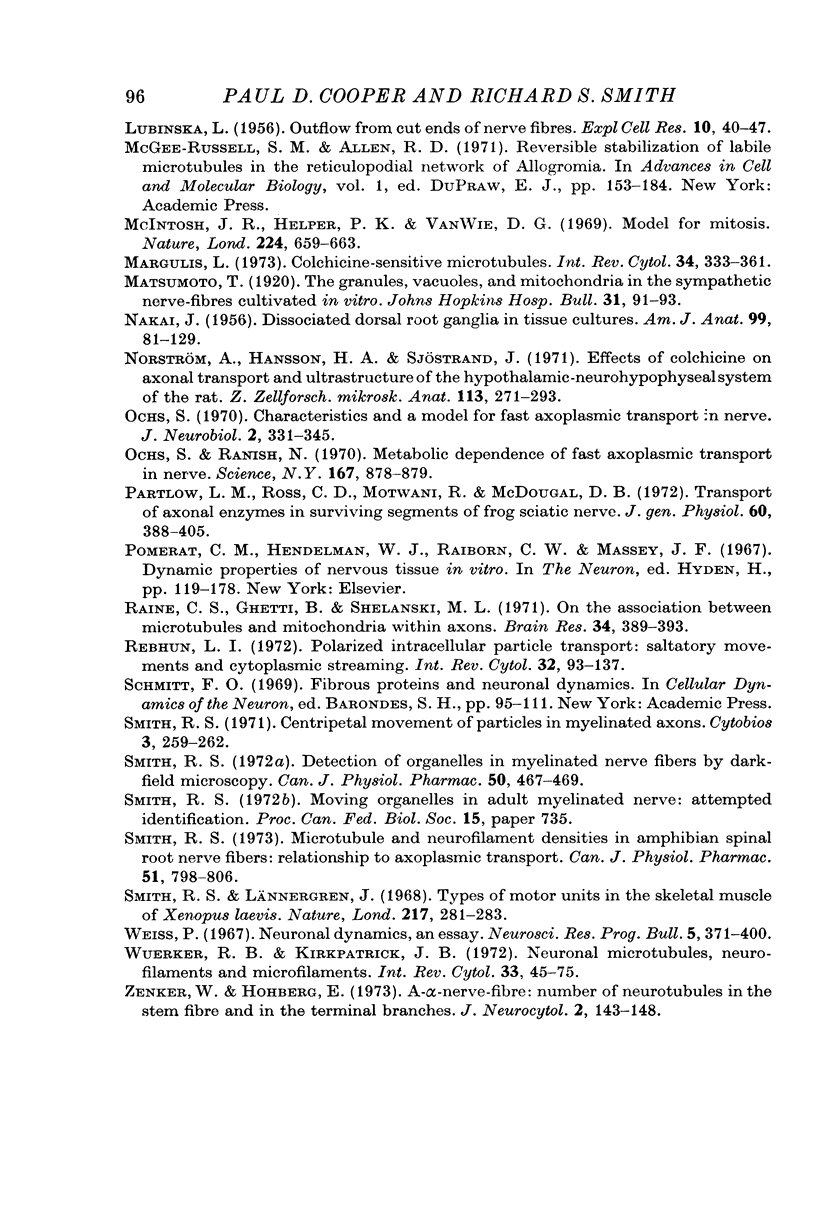
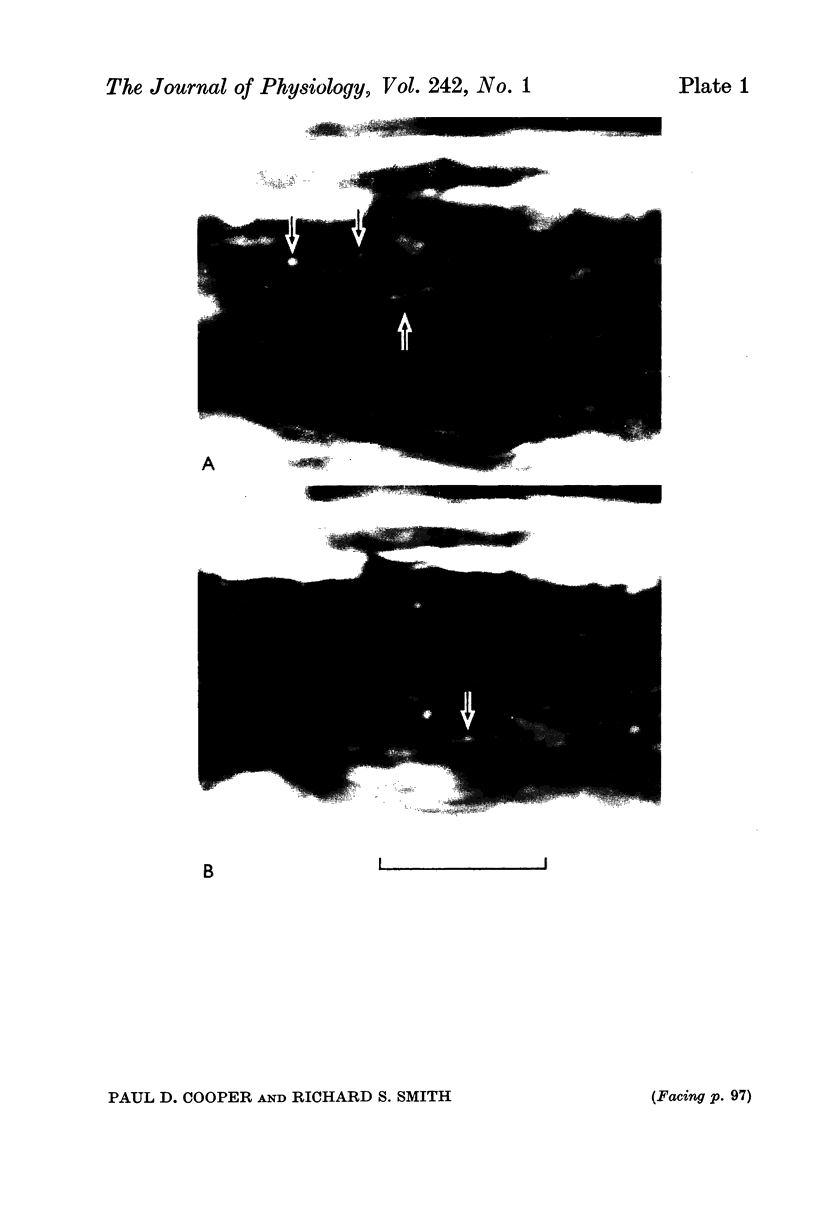
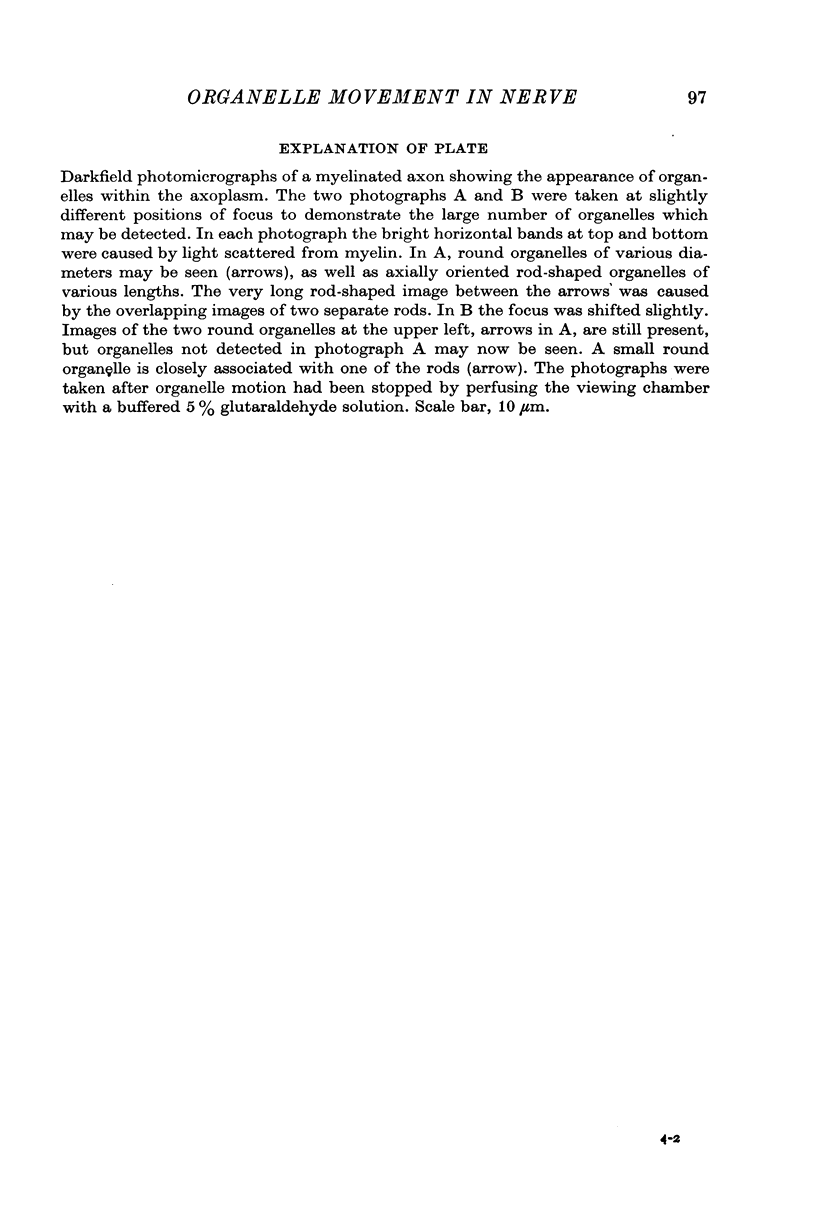
Images in this article
Selected References
These references are in PubMed. This may not be the complete list of references from this article.
- Berlinrood M., McGee-Russell S. M., Allen R. D. Patterns of particle movement in nerve fibres in vitro. An analysis by photokymography and microscopy. J Cell Sci. 1972 Nov;11(3):875–886. doi: 10.1242/jcs.11.3.875. [DOI] [PubMed] [Google Scholar]
- Blume R., Scharf J. H. Histologische, histotopochemische und statistische Untersuchungen über Länge und Verteilung der Mitochondrien in der peripheren segmentierten Nervenfaser und ihren Hüllzellen des N. intercostalis bei Bos taurus) Acta Histochem. 1964 Oct 13;19(1):24–66. [PubMed] [Google Scholar]
- Borisy G. G., Taylor E. W. The mechanism of action of colchicine. Binding of colchincine-3H to cellular protein. J Cell Biol. 1967 Aug;34(2):525–533. doi: 10.1083/jcb.34.2.525. [DOI] [PMC free article] [PubMed] [Google Scholar]
- Borisy G. G., Taylor E. W. The mechanism of action of colchicine. Colchicine binding to sea urchin eggs and the mitotic apparatus. J Cell Biol. 1967 Aug;34(2):535–548. doi: 10.1083/jcb.34.2.535. [DOI] [PMC free article] [PubMed] [Google Scholar]
- Dahlström A. Effect of colchicine on transport of amine storage granules in sympathetic nerves of rat. Eur J Pharmacol. 1968 Dec;5(1):111–113. doi: 10.1016/0014-2999(68)90165-9. [DOI] [PubMed] [Google Scholar]
- Edstrom A., Mattsson H. Fast axonal transport in vitro in the sciatic system of the frog. J Neurochem. 1972 Jan;19(1):205–221. doi: 10.1111/j.1471-4159.1972.tb01270.x. [DOI] [PubMed] [Google Scholar]
- Edström A., Hanson M. Retrograde axonal transport of proteins in vitro in frog sciatic nerves. Brain Res. 1973 Oct 26;61:311–320. doi: 10.1016/0006-8993(73)90535-0. [DOI] [PubMed] [Google Scholar]
- Freed J. J., Lebowitz M. M. The association of a class of saltatory movements with microtubules in cultured cells. J Cell Biol. 1970 May;45(2):334–354. doi: 10.1083/jcb.45.2.334. [DOI] [PMC free article] [PubMed] [Google Scholar]
- HUGHES A. The growth of embryonic neurites; a study of cultures of chick neural tissues. J Anat. 1953 Apr;87(2):150–162. [PMC free article] [PubMed] [Google Scholar]
- Hutchinson N. A., Koles Z. J., Smith R. S. Conduction velocity in myelinated nerve fibres of Xenopus laevis. J Physiol. 1970 Jun;208(2):279–289. doi: 10.1113/jphysiol.1970.sp009119. [DOI] [PMC free article] [PubMed] [Google Scholar]
- Huxley A. F., Simmons R. M. Proposed mechanism of force generation in striated muscle. Nature. 1971 Oct 22;233(5321):533–538. doi: 10.1038/233533a0. [DOI] [PubMed] [Google Scholar]
- Hökfelt T., Dahlström A. Effects of two mitosis inhibitors (colchicine and vinblastine) on the distribution and axonal transport of noradrenaline storage particles, studied by fluorescence and electron microscopy. Z Zellforsch Mikrosk Anat. 1971;119(4):460–482. doi: 10.1007/BF00455243. [DOI] [PubMed] [Google Scholar]
- James K. A., Bray J. J., Morgan I. G., Austin L. The effect of colchicine on the transport of axonal protein in the chicken. Biochem J. 1970 May;117(4):767–771. doi: 10.1042/bj1170767. [DOI] [PMC free article] [PubMed] [Google Scholar]
- Johnson G., Smith R. S., Lock G. S. Accumulation of material at severed ends of myelinated nerve fibers. Am J Physiol. 1969 Jul;217(1):188–191. doi: 10.1152/ajplegacy.1969.217.1.188. [DOI] [PubMed] [Google Scholar]
- Kirkpatrick J. B., Bray J. J., Palmer S. M. Visualization of axoplasmic flow in vitro by Nomarski microscopy. Comparison to rapid flow of radioactive proteins. Brain Res. 1972 Aug 11;43(1):1–10. doi: 10.1016/0006-8993(72)90270-3. [DOI] [PubMed] [Google Scholar]
- Kirkpatrick J. B., Stern L. Z. Axoplasmic flow in human sural nerve. Arch Neurol. 1973 May;28(5):308–312. doi: 10.1001/archneur.1973.00490230044005. [DOI] [PubMed] [Google Scholar]
- Kreutzberg G. W. Neuronal dynamics and axonal flow. IV. Blockage of intra-axonal enzyme transport by colchicine. Proc Natl Acad Sci U S A. 1969 Mar;62(3):722–728. doi: 10.1073/pnas.62.3.722. [DOI] [PMC free article] [PubMed] [Google Scholar]
- LUBINSKA L. Outflow from cut ends of nerve fibres. Exp Cell Res. 1956 Feb;10(1):40–47. doi: 10.1016/0014-4827(56)90068-4. [DOI] [PubMed] [Google Scholar]
- Litchy W. J. Uptake and retrograde transport of horseradish peroxidase in frog sartorius nerve in vitro. Brain Res. 1973 Jun 29;56:377–381. doi: 10.1016/0006-8993(73)90356-9. [DOI] [PubMed] [Google Scholar]
- Margulis L. Colchicine-sensitive microtubules. Int Rev Cytol. 1973;34:333–361. doi: 10.1016/s0074-7696(08)61939-7. [DOI] [PubMed] [Google Scholar]
- NAKAI J. Dissociated dorsal root ganglia in tissue culture. Am J Anat. 1956 Jul;99(1):81–129. doi: 10.1002/aja.1000990105. [DOI] [PubMed] [Google Scholar]
- Norström A., Hansson H. A., Sjöstrand J. Effects of colchicine on axonal transport and ultrastructure of the hypothalamo-neurohypophyseal system of the rat. Z Zellforsch Mikrosk Anat. 1971;113(2):271–293. doi: 10.1007/BF00339421. [DOI] [PubMed] [Google Scholar]
- Ochs S. Characteristics and a model for fast axoplasmic transport in nerve. J Neurobiol. 1971;2(4):331–345. doi: 10.1002/neu.480020406. [DOI] [PubMed] [Google Scholar]
- Ochs S., Ranish N. Metabolic dependence of fast axoplasmic transport in nerve. Science. 1970 Feb 6;167(3919):878–879. doi: 10.1126/science.167.3919.878. [DOI] [PubMed] [Google Scholar]
- Partlow L. M., Ross C. D., Motwani R., McDougal D. B., Jr Transport of axonal enzymes in surviving segments of frog sciatic nerve. J Gen Physiol. 1972 Oct;60(4):388–405. doi: 10.1085/jgp.60.4.388. [DOI] [PMC free article] [PubMed] [Google Scholar]
- Raine C. S., Ghetti B., Shelanski M. L. On the association between microtubules and mitochondria within axons. Brain Res. 1971 Nov;34(2):389–393. doi: 10.1016/0006-8993(71)90293-9. [DOI] [PubMed] [Google Scholar]
- Rebhun L. I. Polarized intracellular particle transport: saltatory movements and cytoplasmic streaming. Int Rev Cytol. 1972;32:93–137. doi: 10.1016/s0074-7696(08)60339-3. [DOI] [PubMed] [Google Scholar]
- Smith R. S. Detection of organelles in myelinated nerve fibers by dark-field microscopy. Can J Physiol Pharmacol. 1972 May;50(5):467–469. doi: 10.1139/y72-071. [DOI] [PubMed] [Google Scholar]
- Smith R. S., Lännergren J. Types of motor units in the skeletal muscle of Xenopus laevis. Nature. 1968 Jan 20;217(5125):281–283. doi: 10.1038/217281a0. [DOI] [PubMed] [Google Scholar]
- Smith R. S. Microtubule and neurofilament densities in amphibian spinal root nerve fibers: relationship to axoplasmic transport. Can J Physiol Pharmacol. 1973 Nov;51(11):798–806. doi: 10.1139/y73-123. [DOI] [PubMed] [Google Scholar]
- Wuerker R. B., Kirkpatrick J. B. Neuronal microtubules, neurofilaments, and microfilaments. Int Rev Cytol. 1972;33:45–75. doi: 10.1016/s0074-7696(08)61448-5. [DOI] [PubMed] [Google Scholar]
- Zenker W., Hohberg E. A-alpha-nerve-fiber: number of neurotubules in the stem fibre and in the terminal branches. J Neurocytol. 1973 Jun;2(2):143–148. doi: 10.1007/BF01474716. [DOI] [PubMed] [Google Scholar]