Abstract
1. Miniature end-plate currents were recorded at neuromuscular junctions of toads, either in voltage-clamped fibres or with extracellular electrodes. The two methods gave similar results. 2. Two types of m.e.p.c.s, differing in their growth times (50-300 musec and 0-5-5 msec) were found. The more frequent had the shorter growth times. 3. The decay of m.e.p.c.s was exponential with a single time constant. The time constant was an exponential function of membrane potential, becoming less as the membrane was depolarized. In contrast, there was little change, or in some cases an increase, in growth times of m.e.p.c.s when the membrane was depolarized. 4. The decay time constant had a Q-10 of 3-13 plus or minus 0-22 (mean plus or minus S.E. of mean) whereas the growth time had a significantly lower Q-10 of about 1-2. The change of decay time constant with temperature followed the Arrhenius equation giving an activation energy of 18 plus or minus 1-0 kcal (mean plus or minus S.E. of mean). The amplitude of m.e.p.c.s increased with temperature and had a Q-10 of 1-5 plus or minus 0-14 (mean plus or minus S.E. of mean) in voltage-clamped fibres. 5. Neostigmine prolonged the decay phase and increased the amplitude of m.e.p.c.s but had little effect on the growth phase. The changes in m.e.p.c.s caused by membrane potential and temperature were not affected by neostigmine. 6. The results show that the growth phase and decay phase of m.e.p.c.s are governed by processes with quite different characteristics. The reaction which limits the decay phase appears first-order, is voltage sensitive and has a Q-10 of about 3, whereas the reaction underlying the growth phase does not appear first-order, is less voltage-sensitive and has a Q-10 of about 1-2. It is suggested that diffusion of transmitter across the synaptic cleft may be the rate-limiting step during the growth phase.
Full text
PDF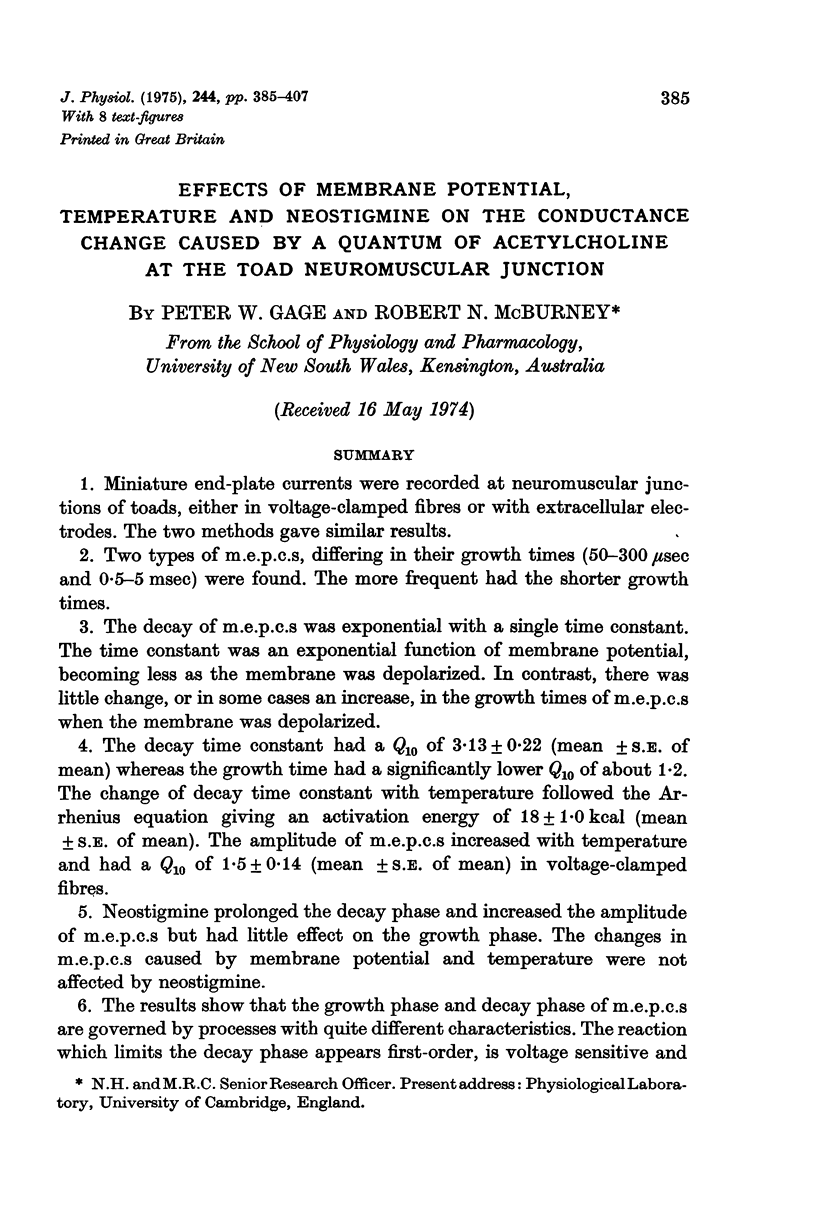
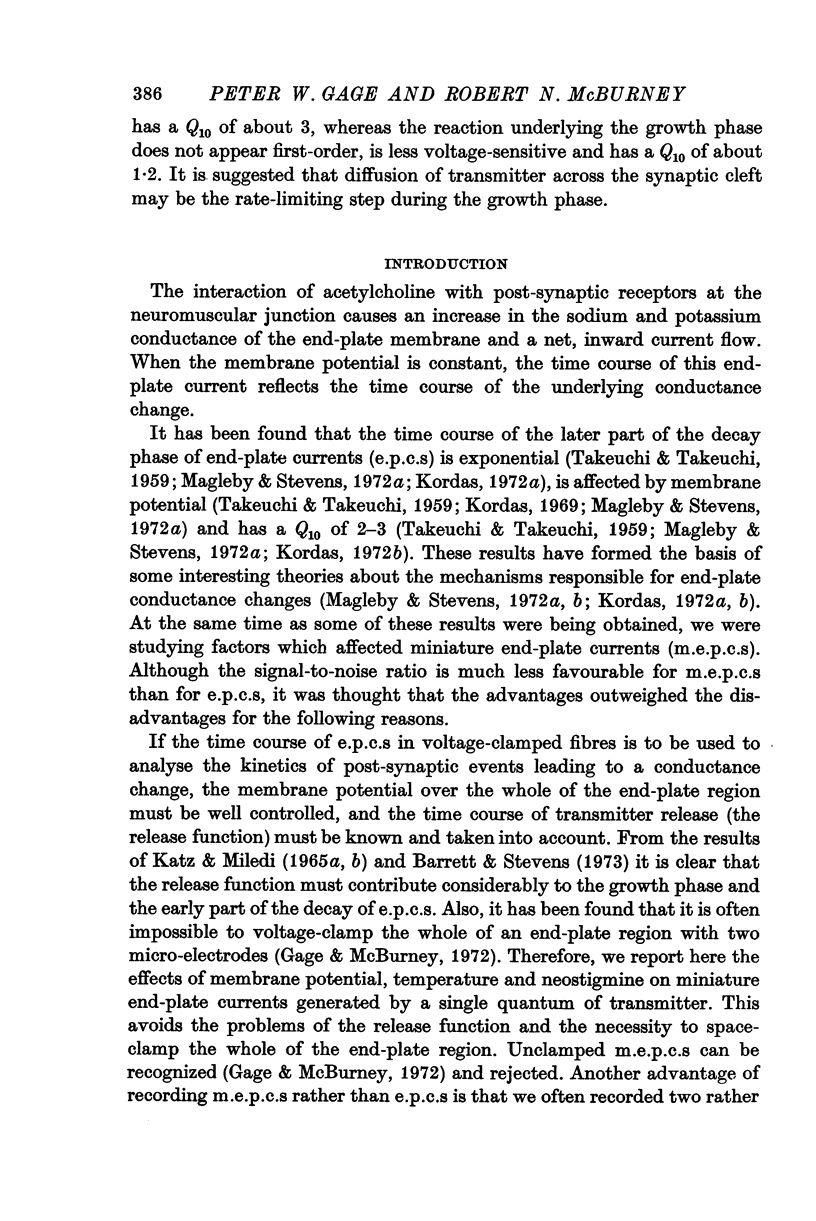
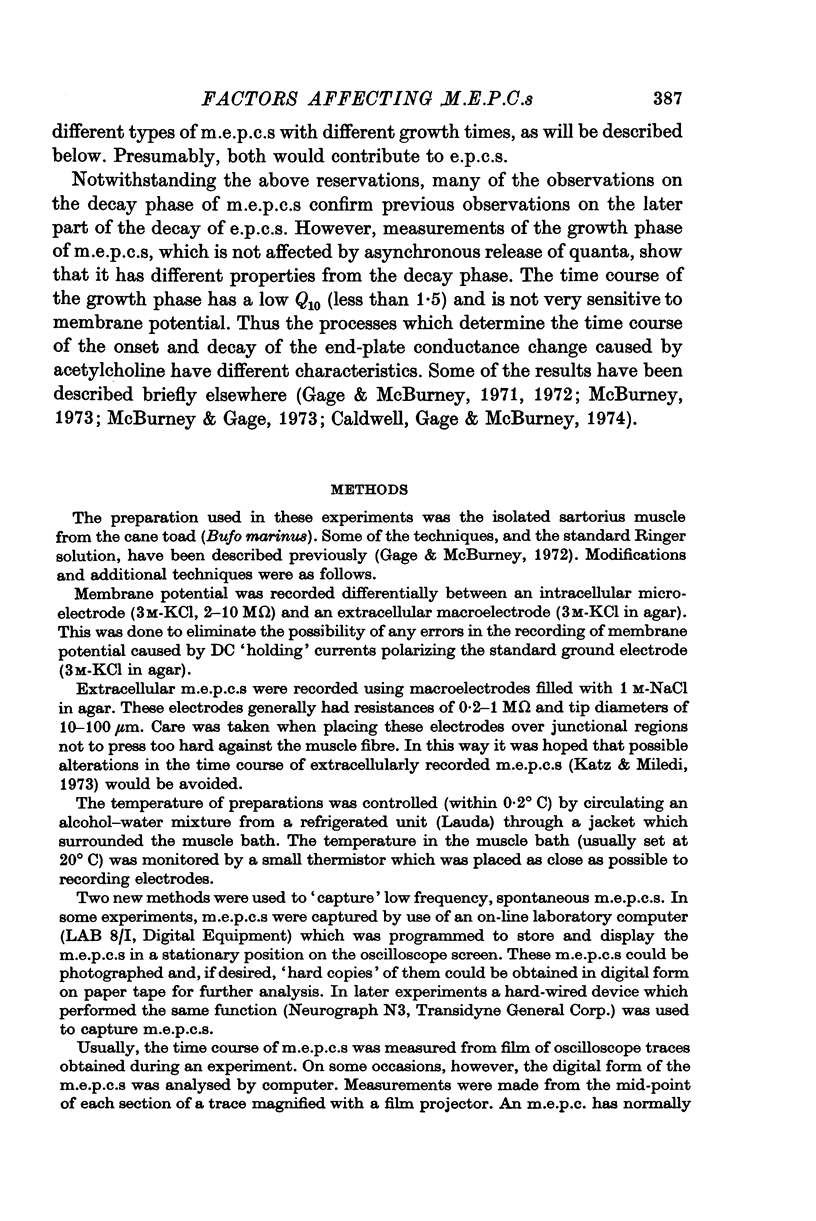
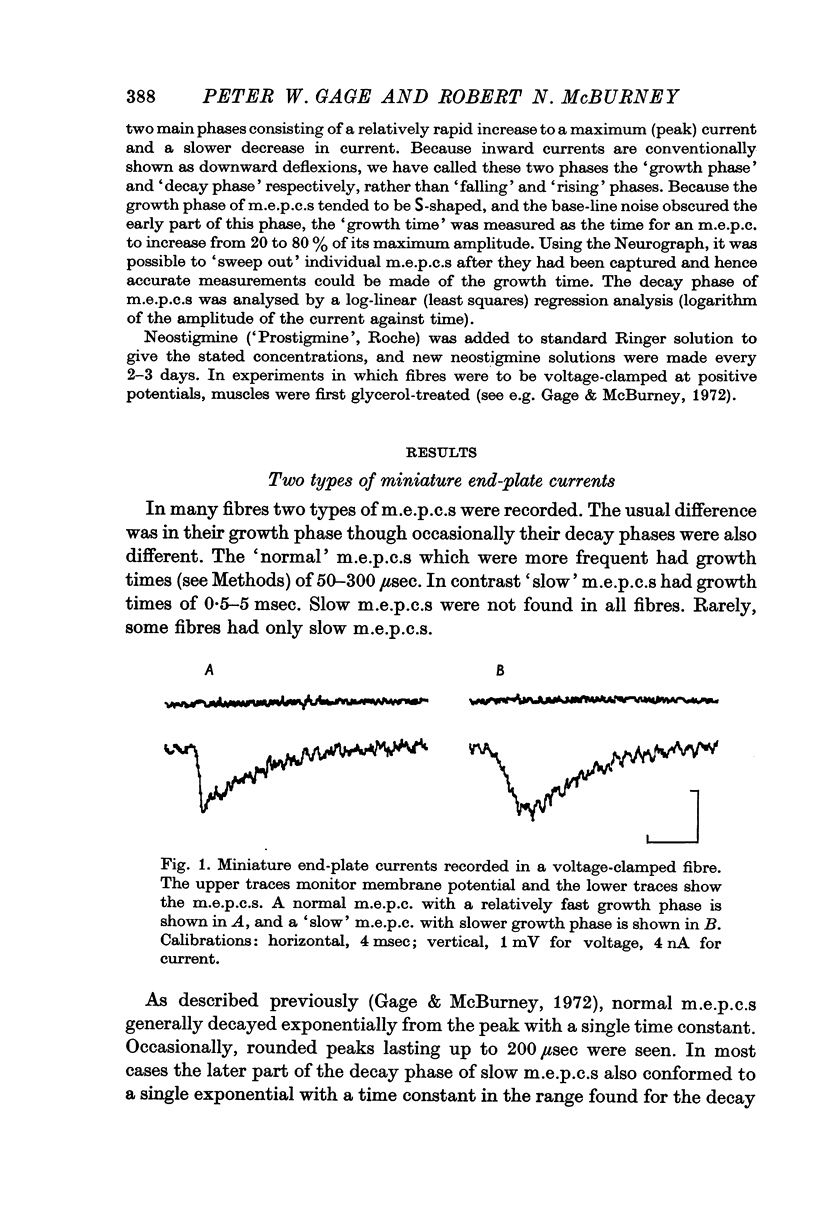
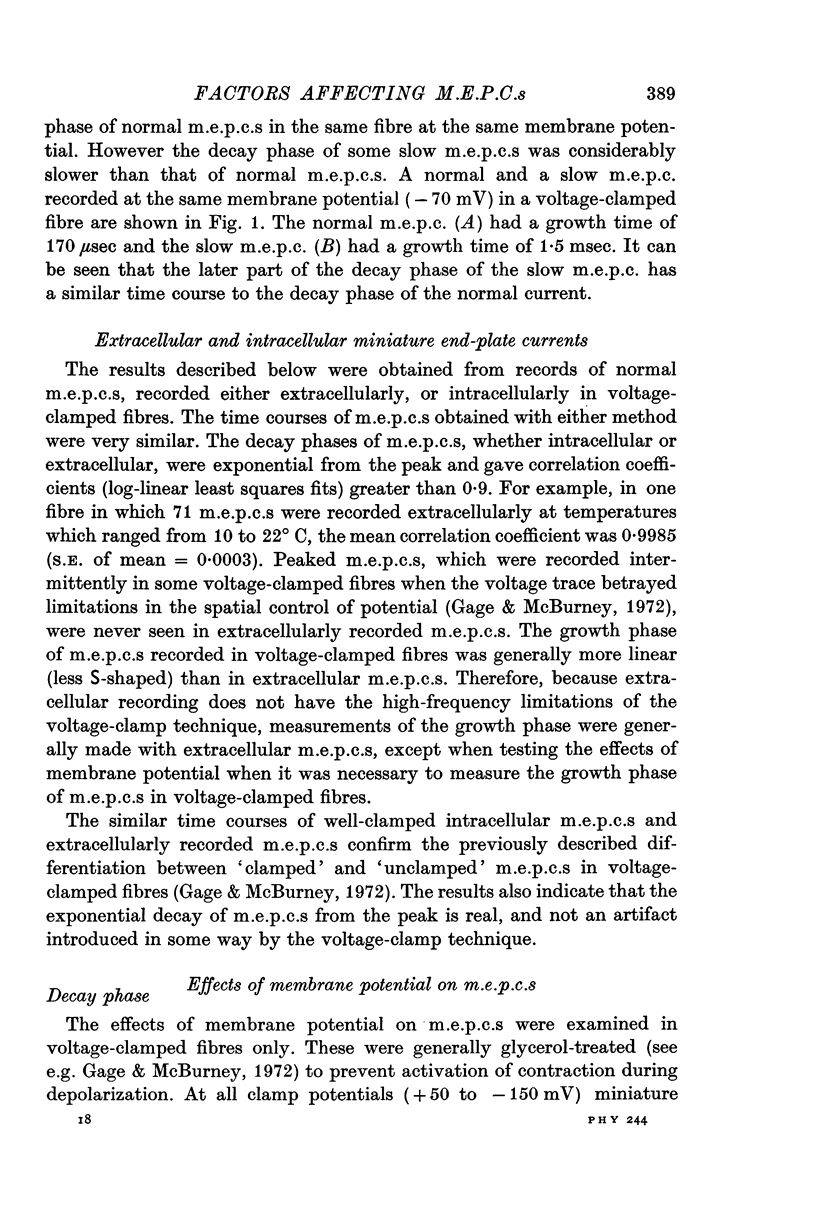
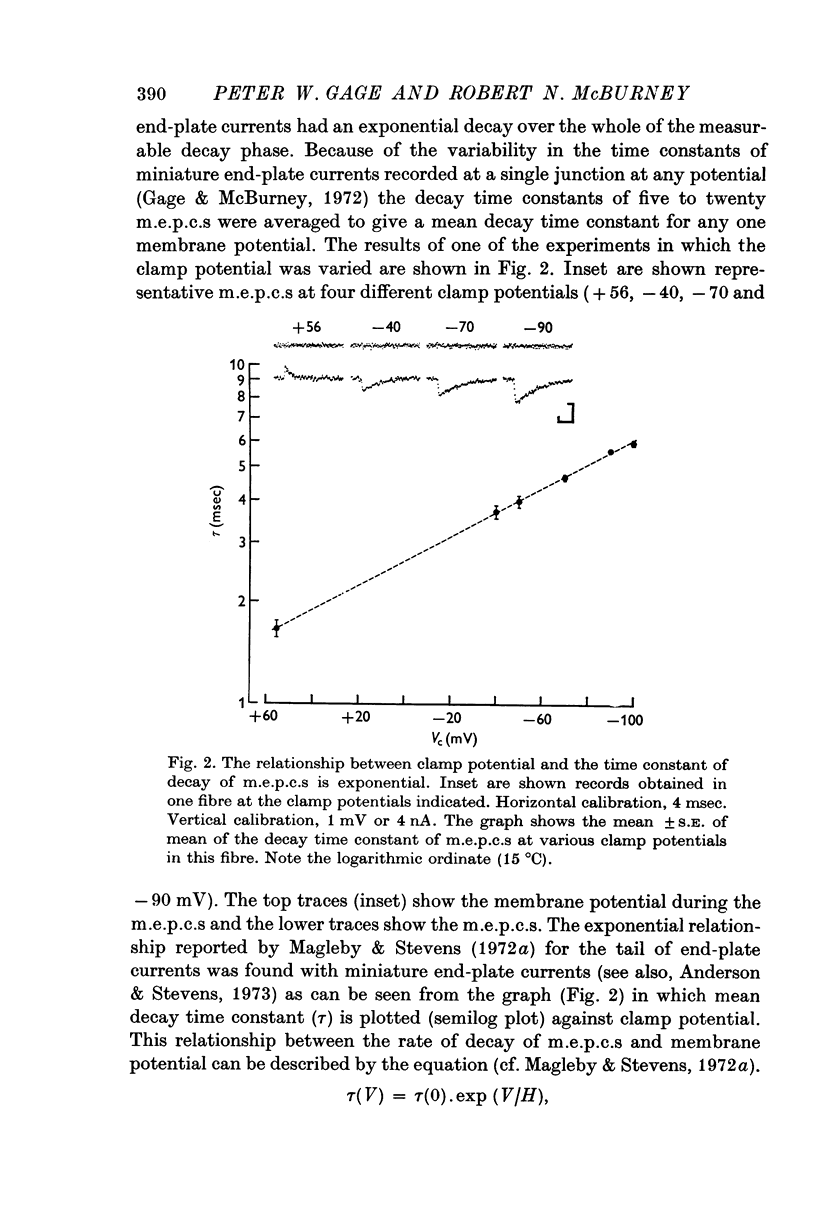
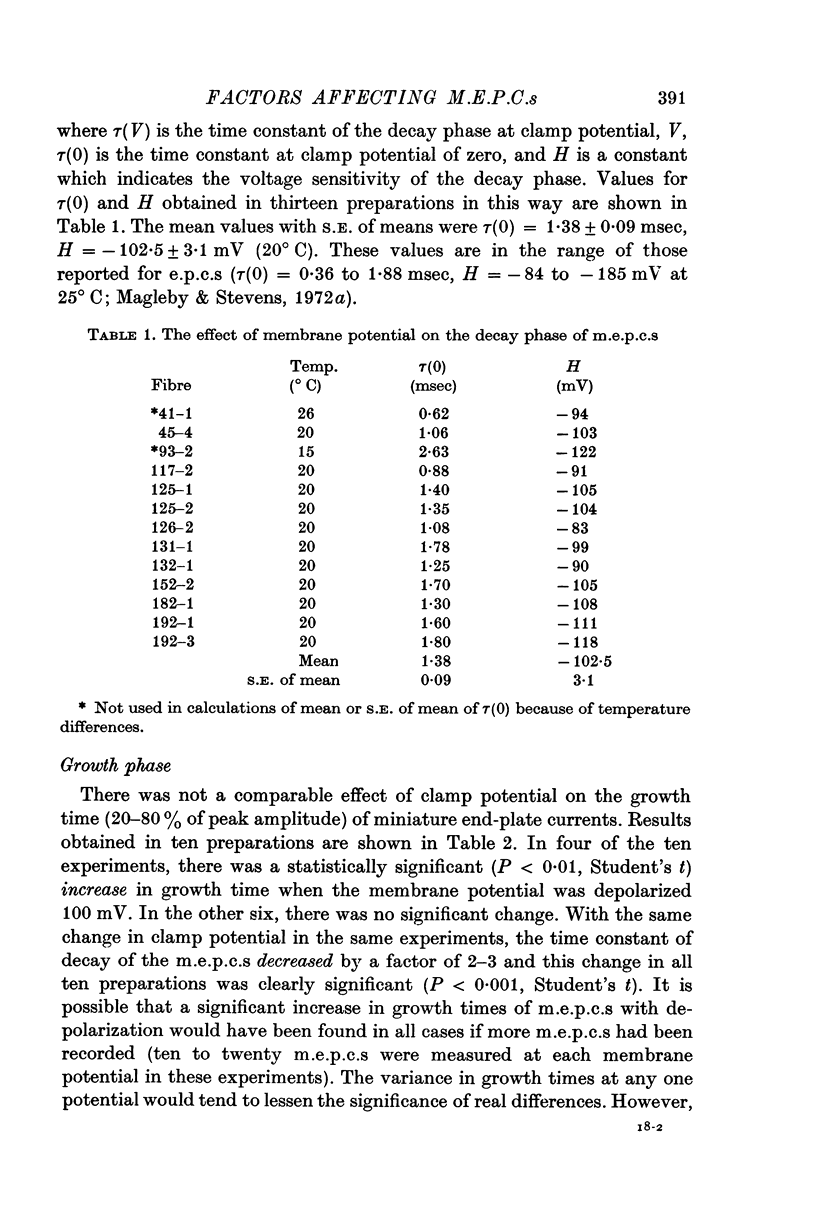
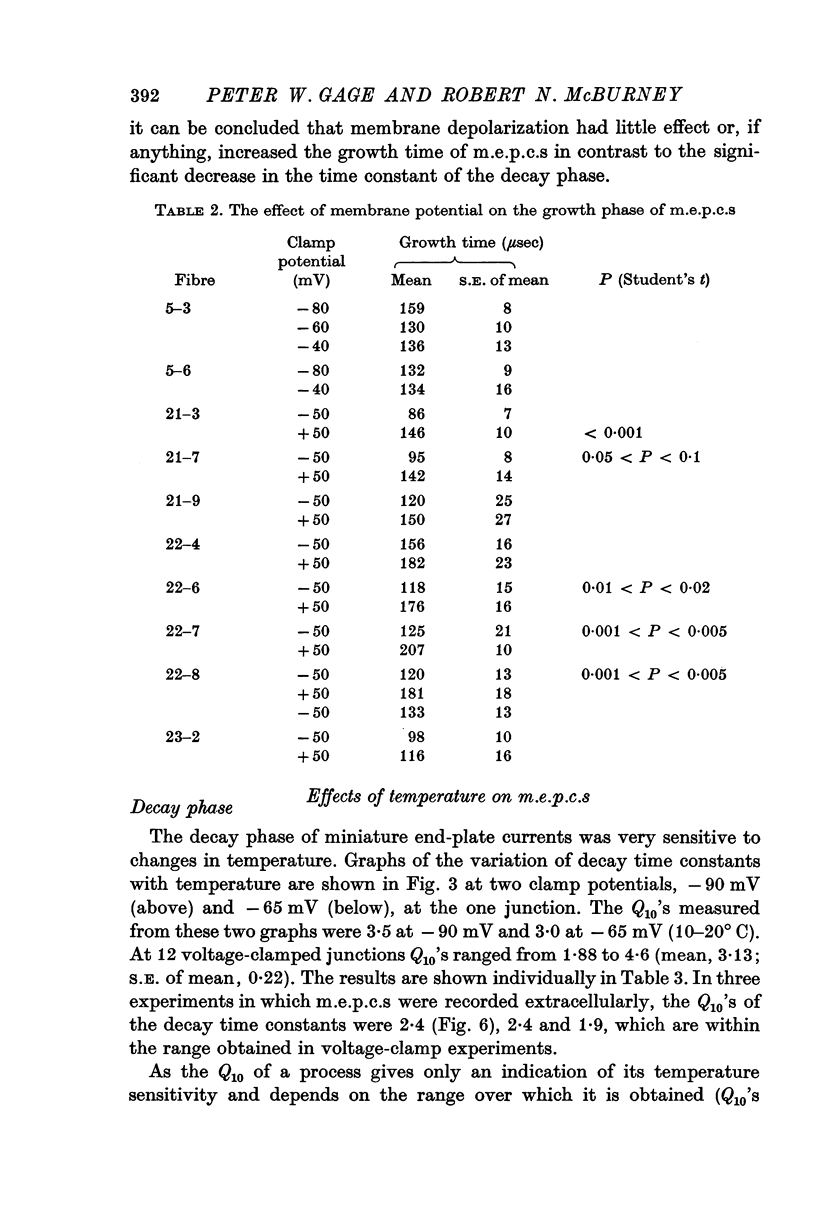
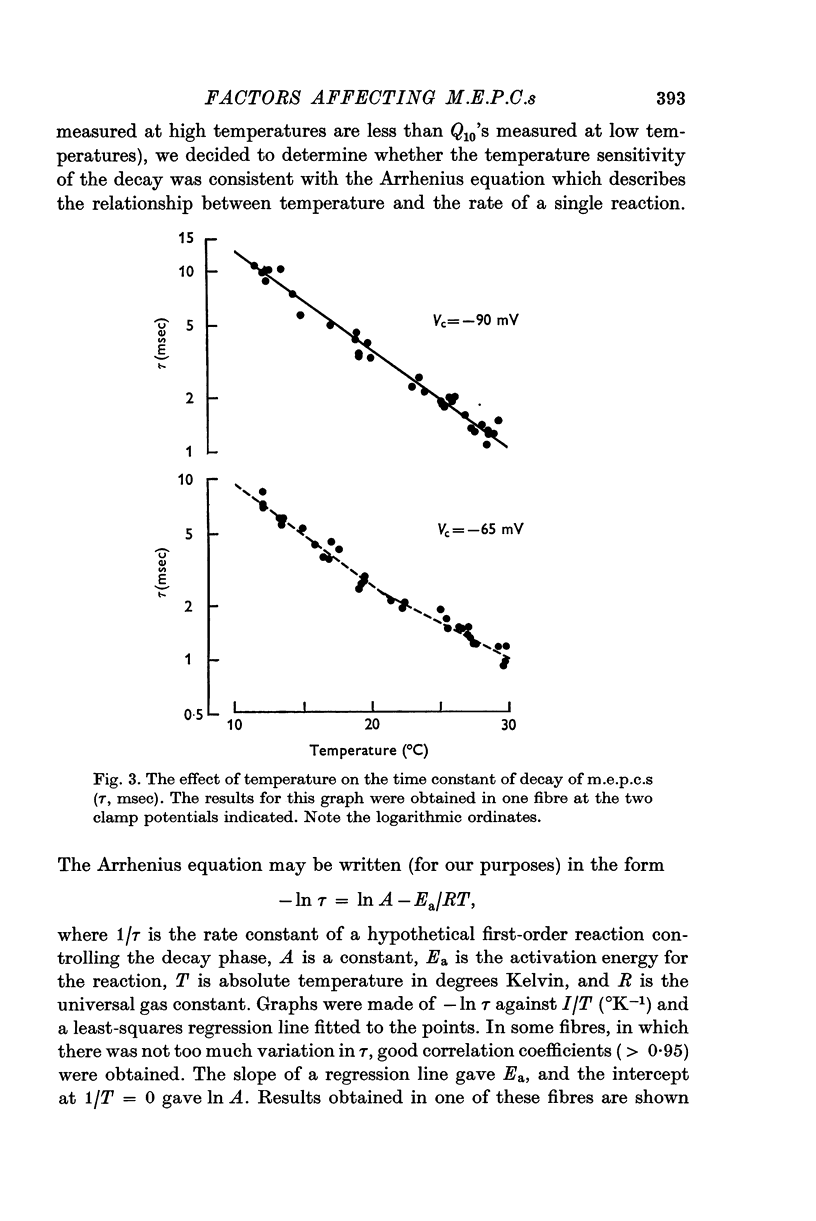
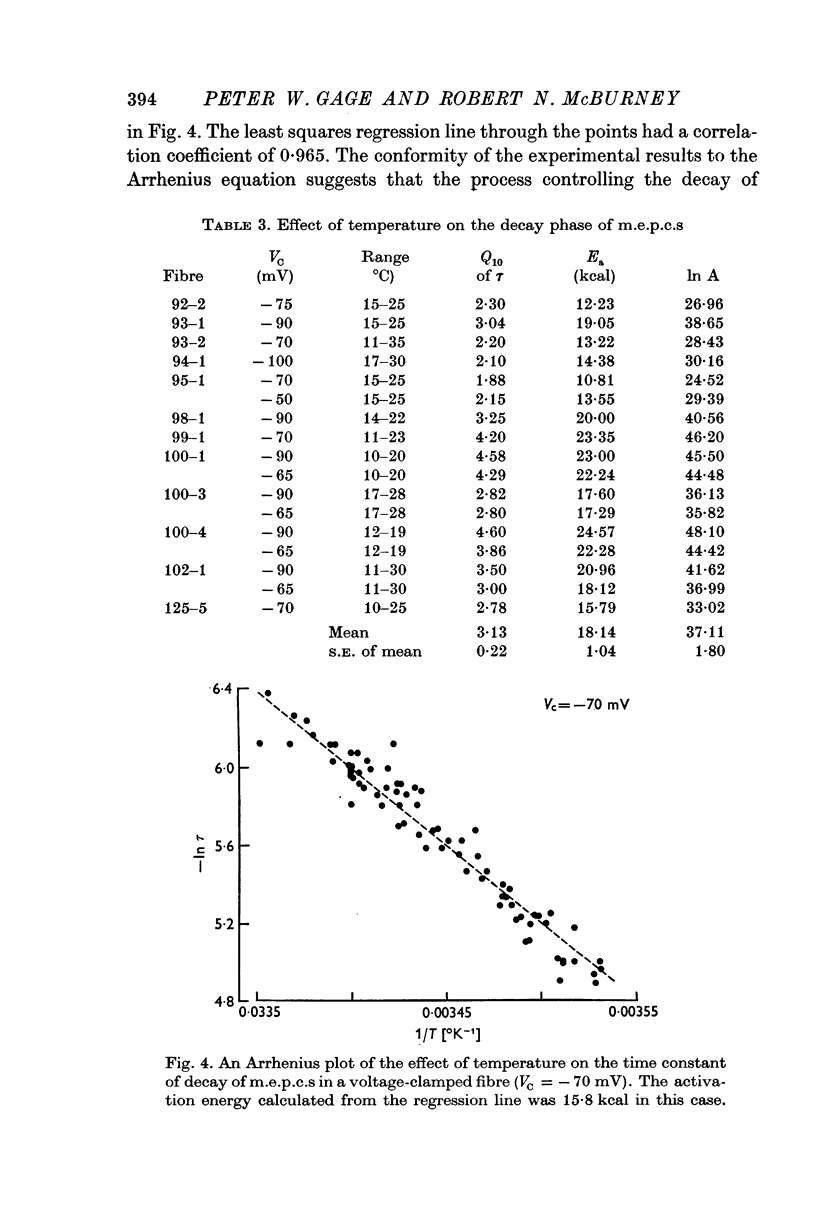
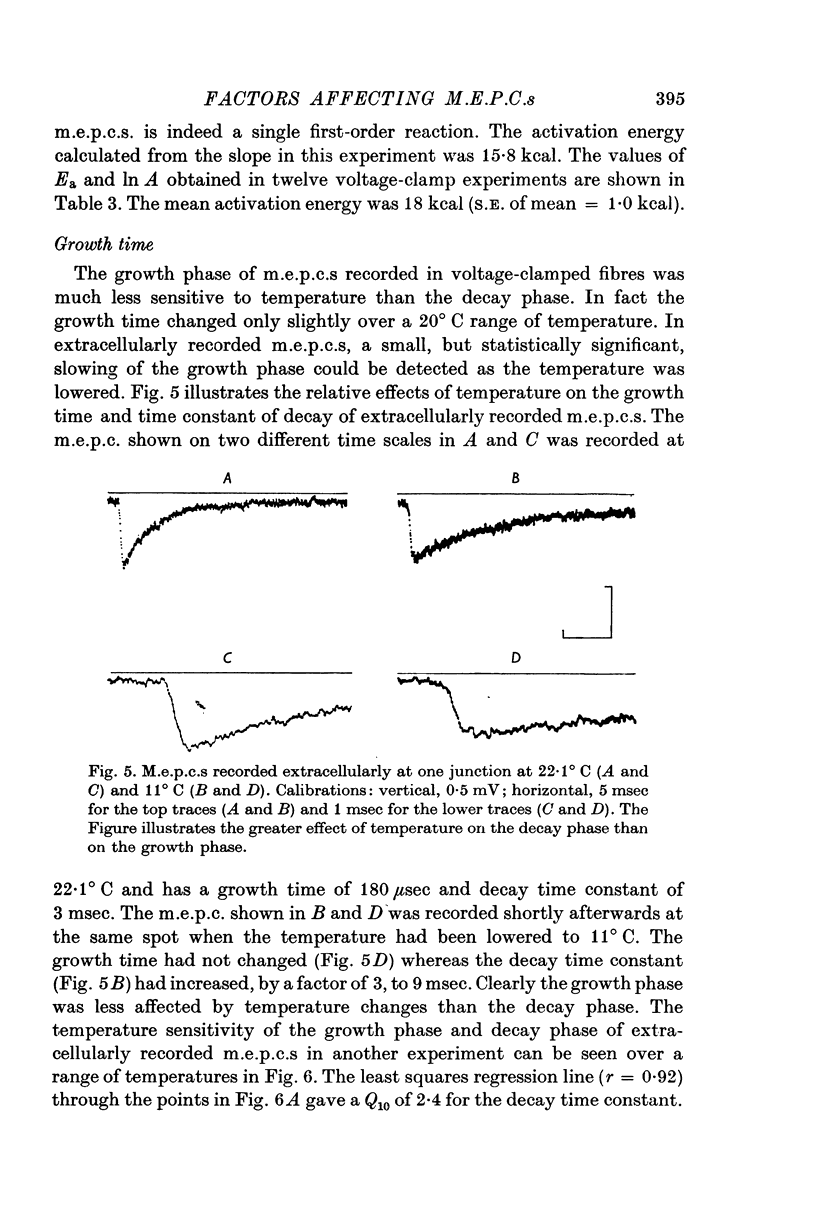
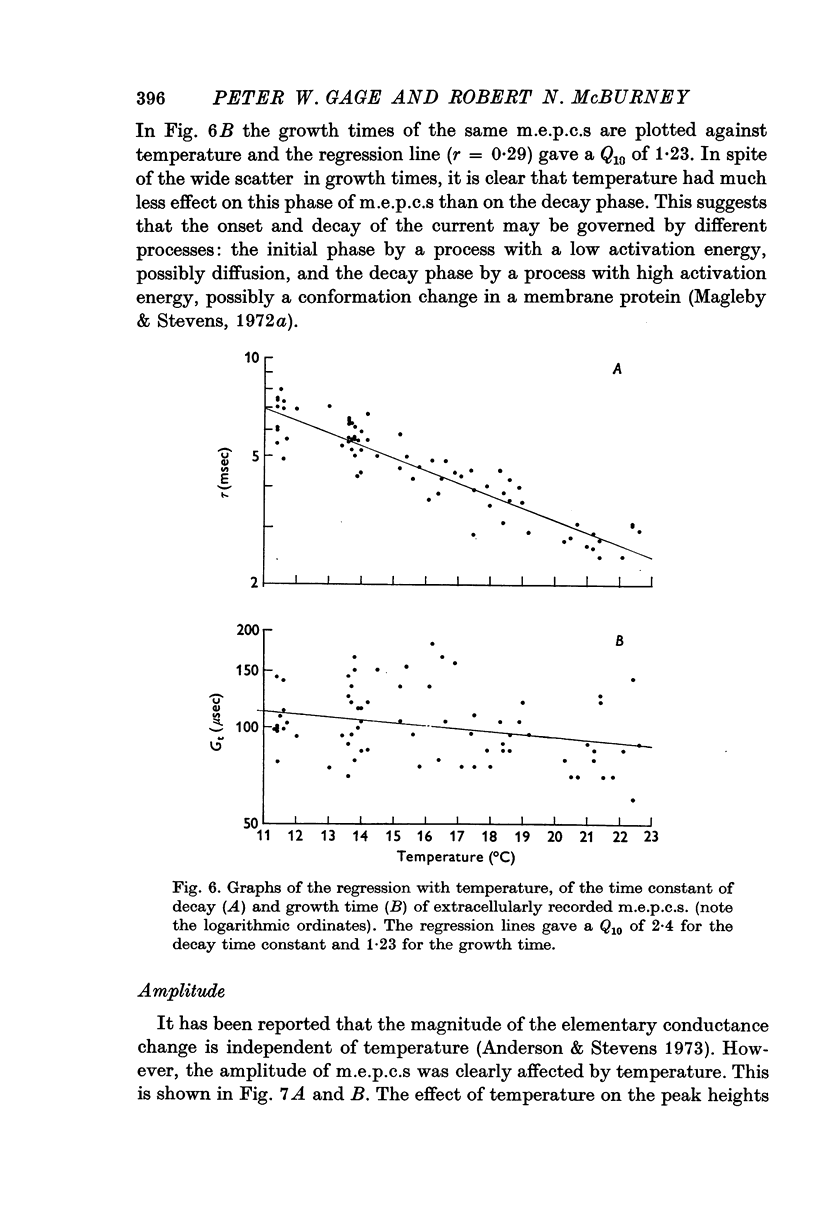
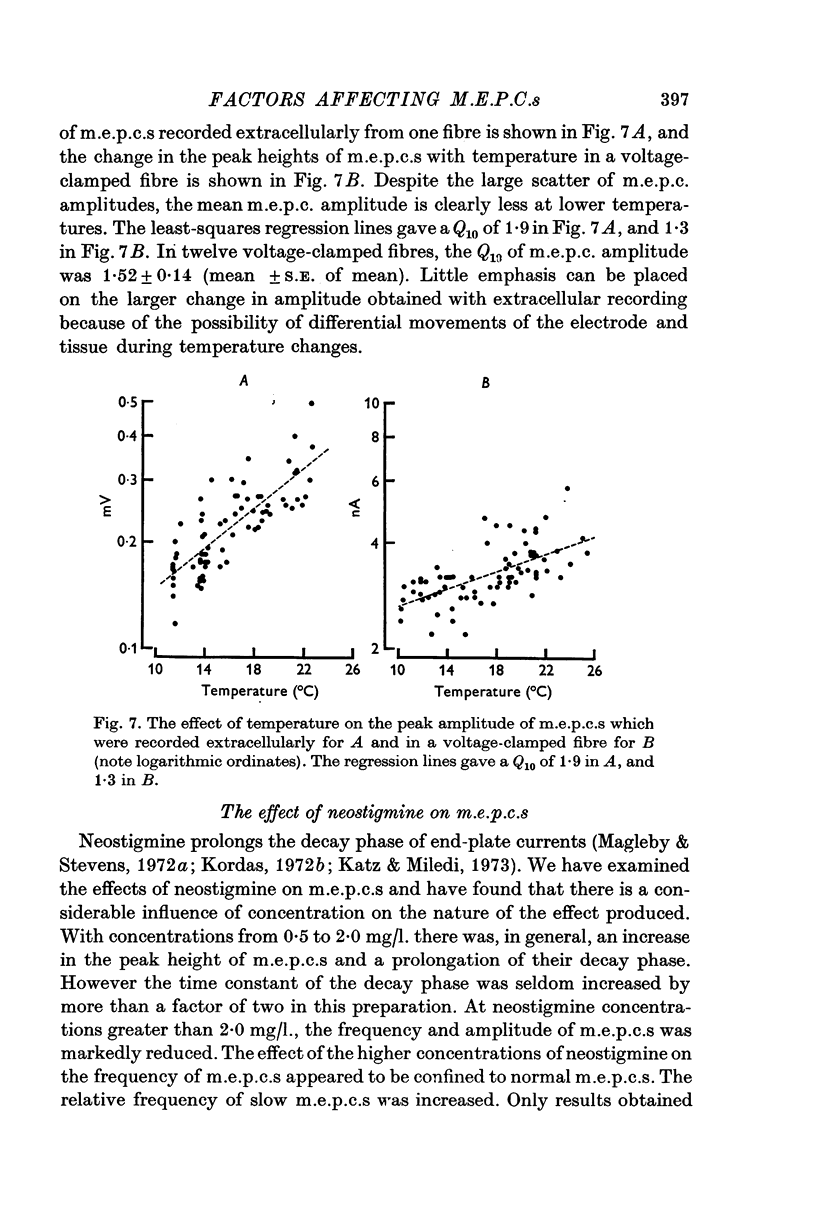
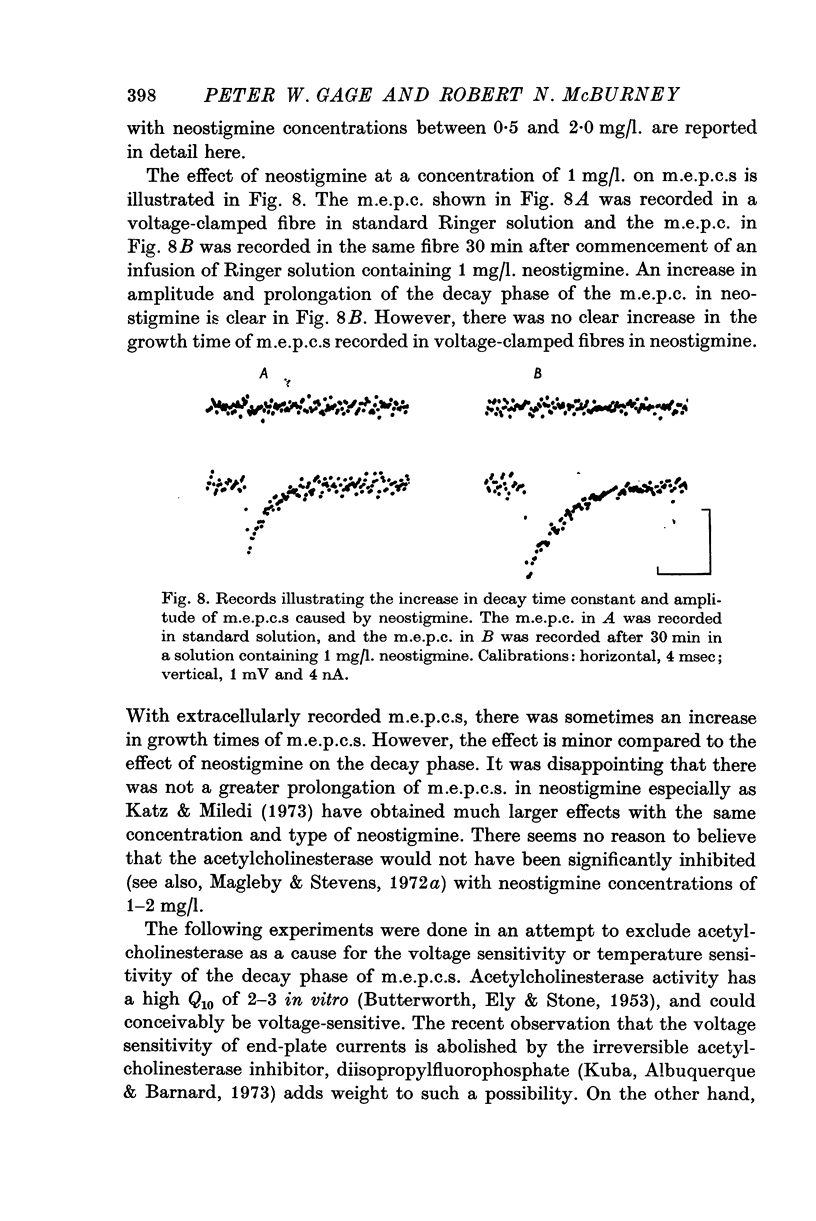
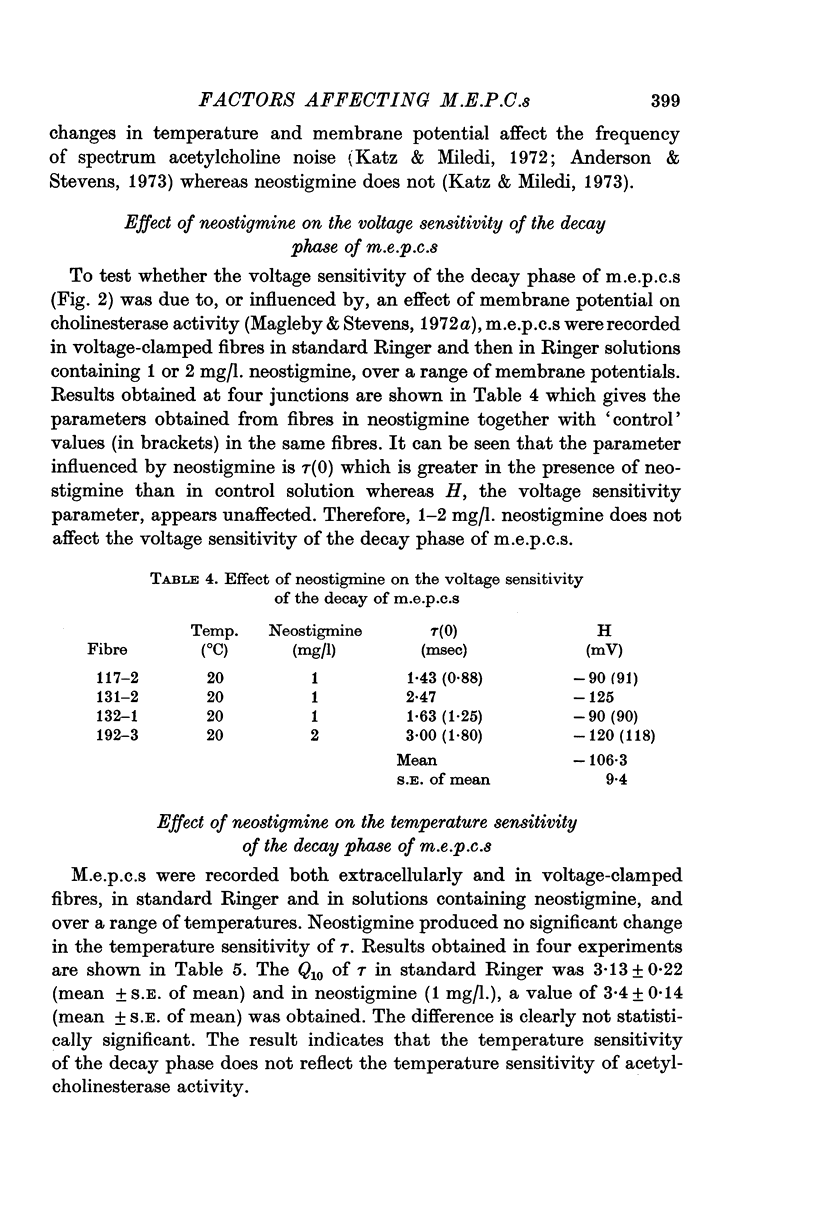
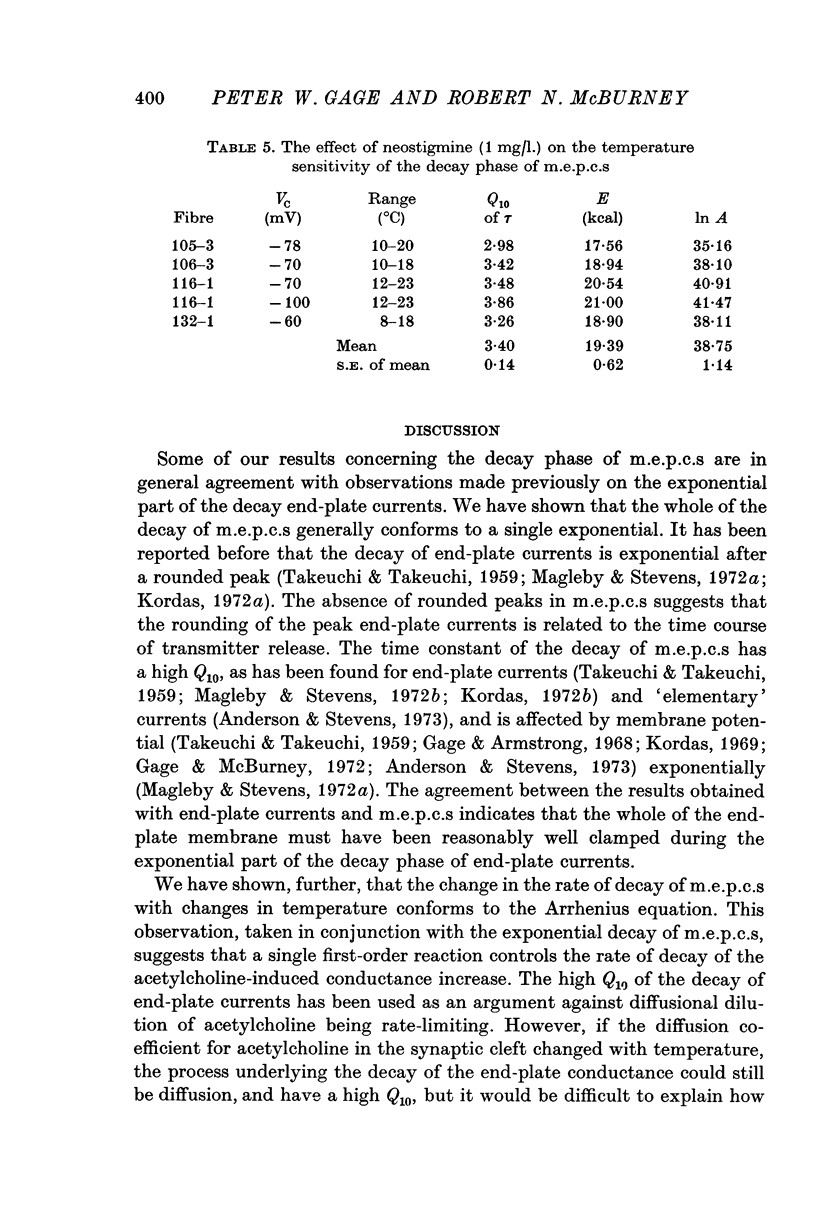
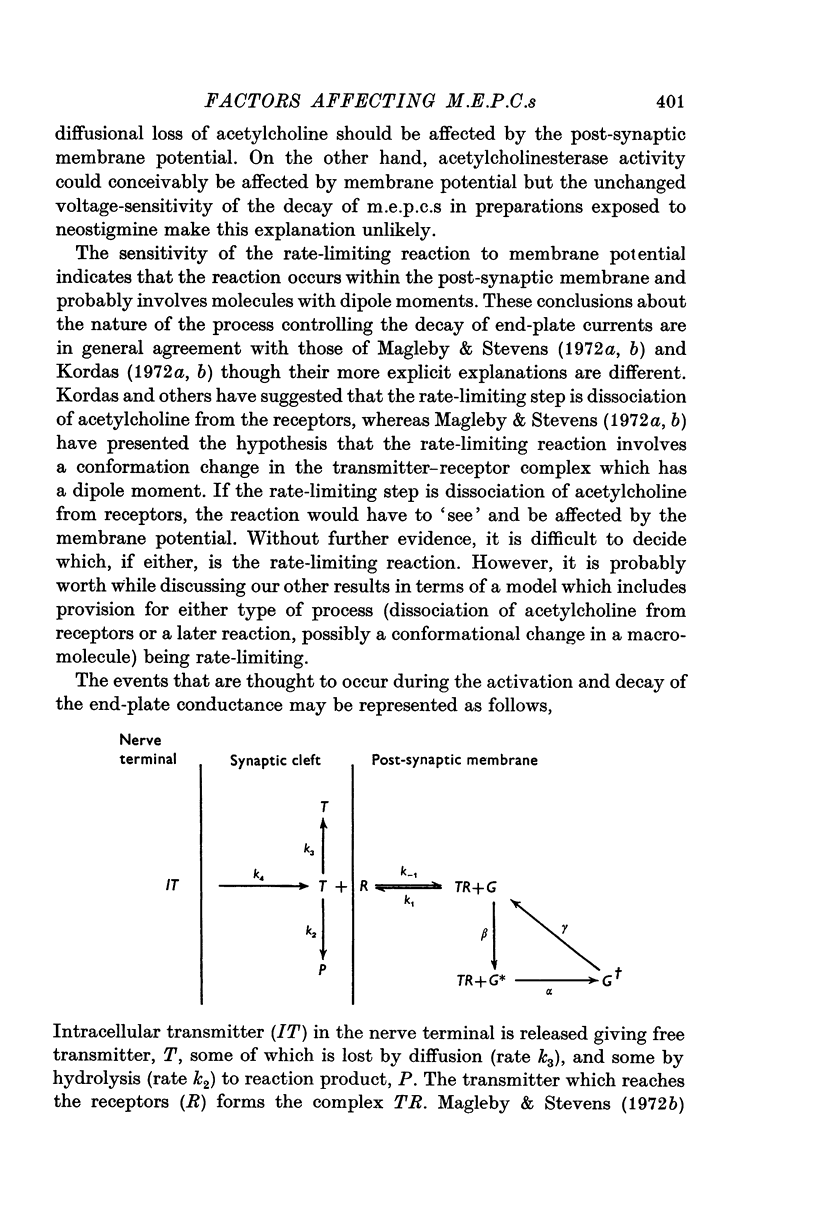
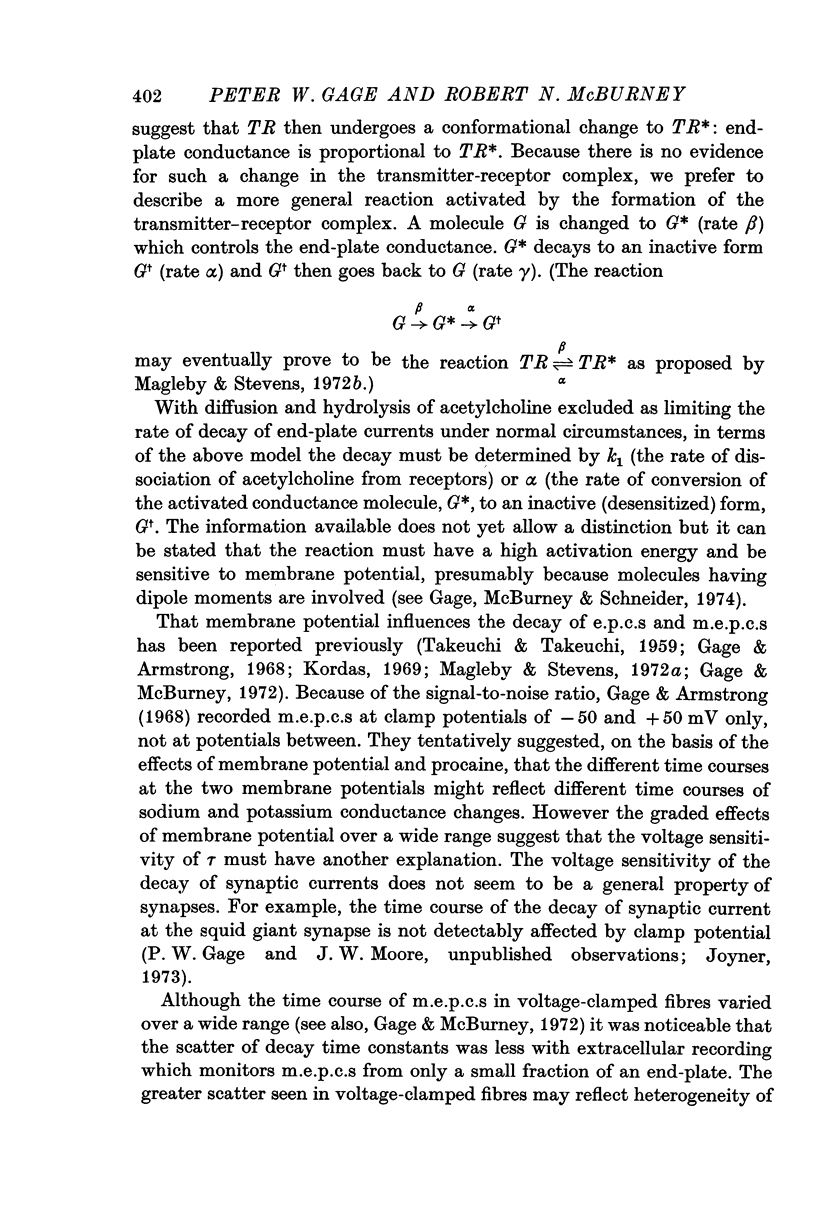
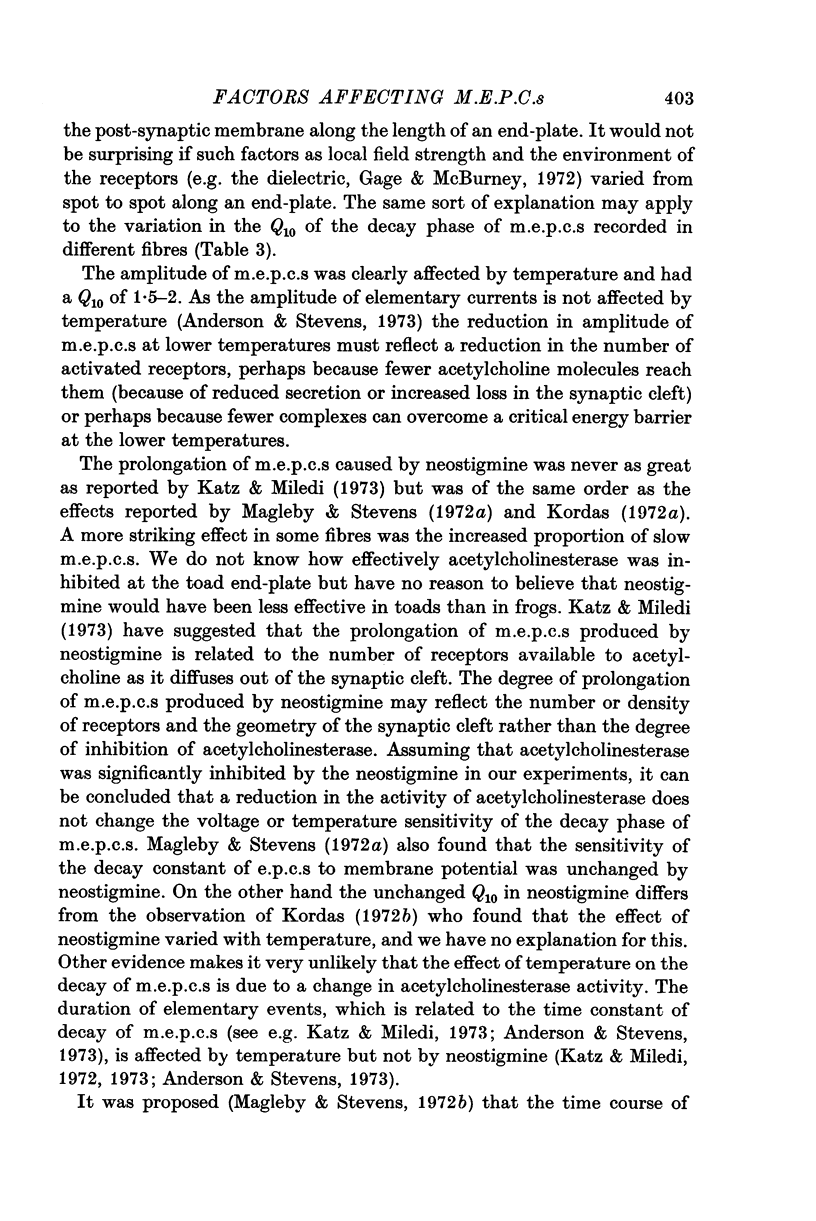
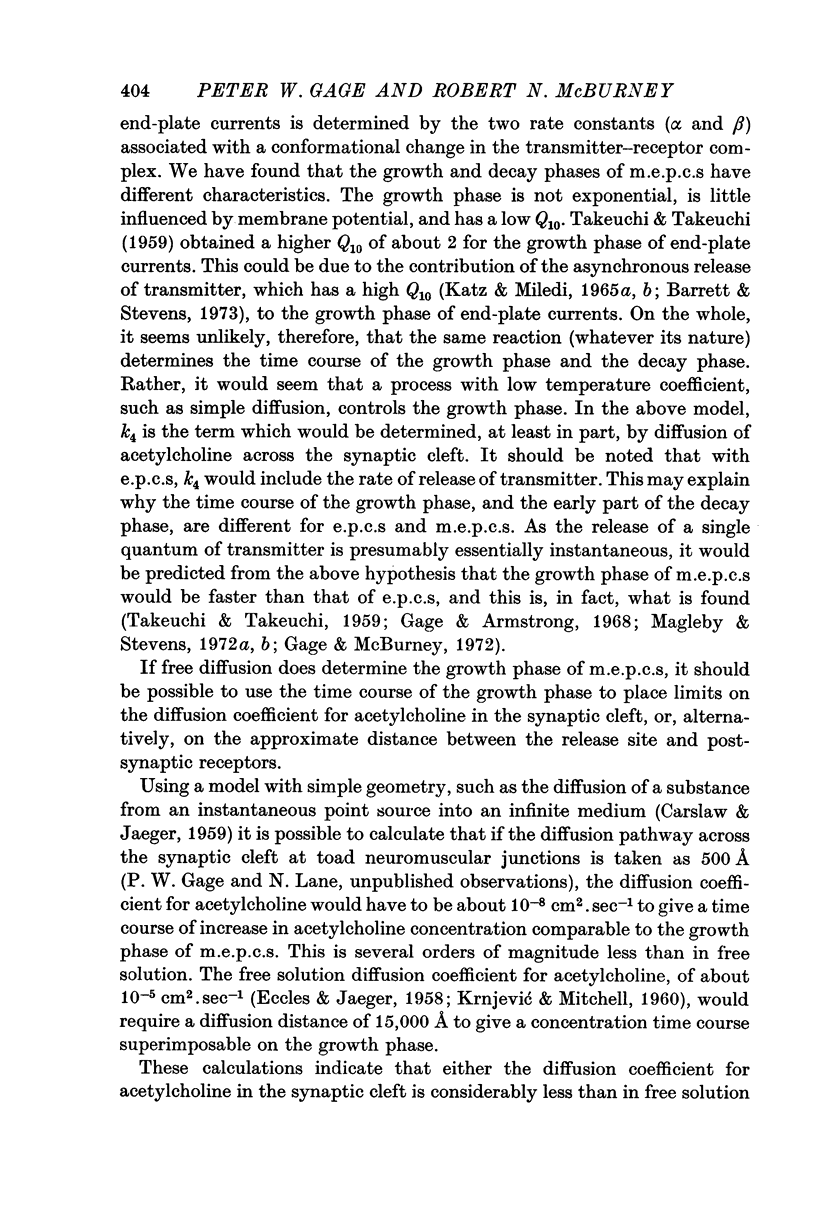
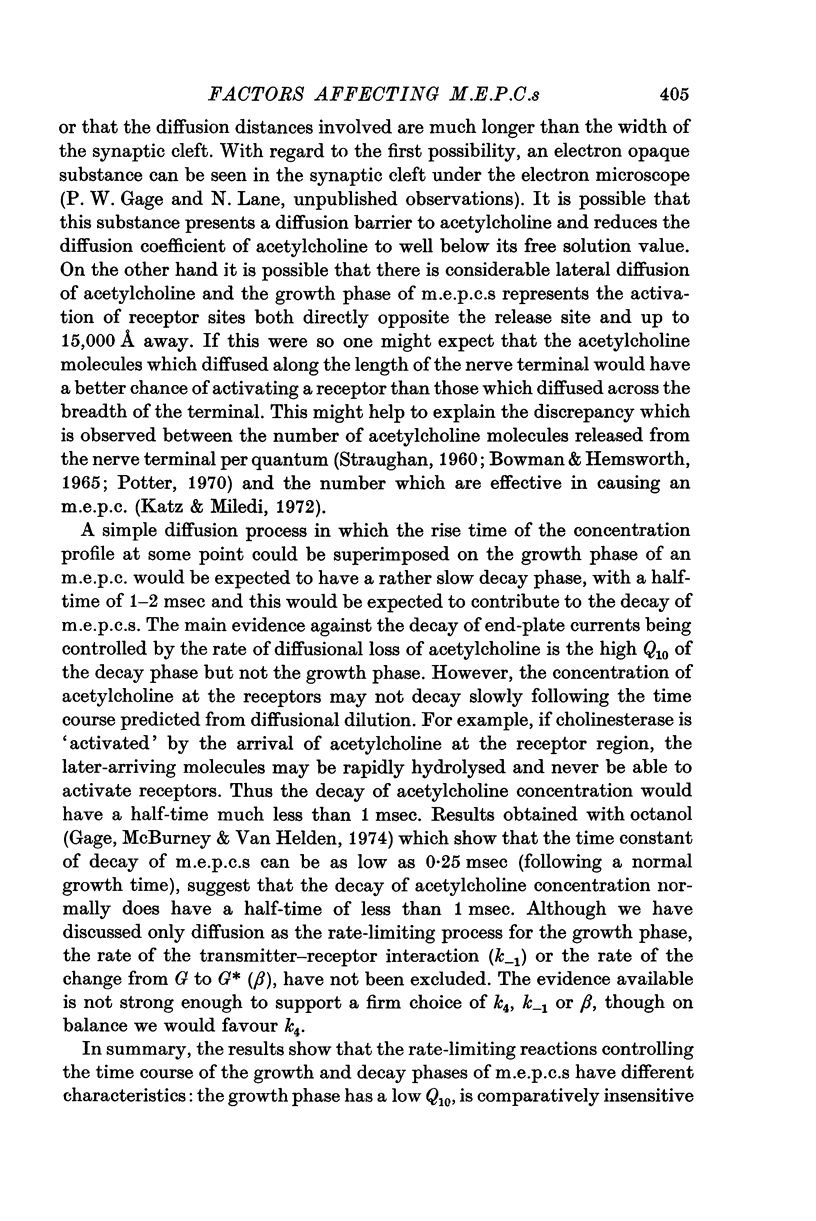
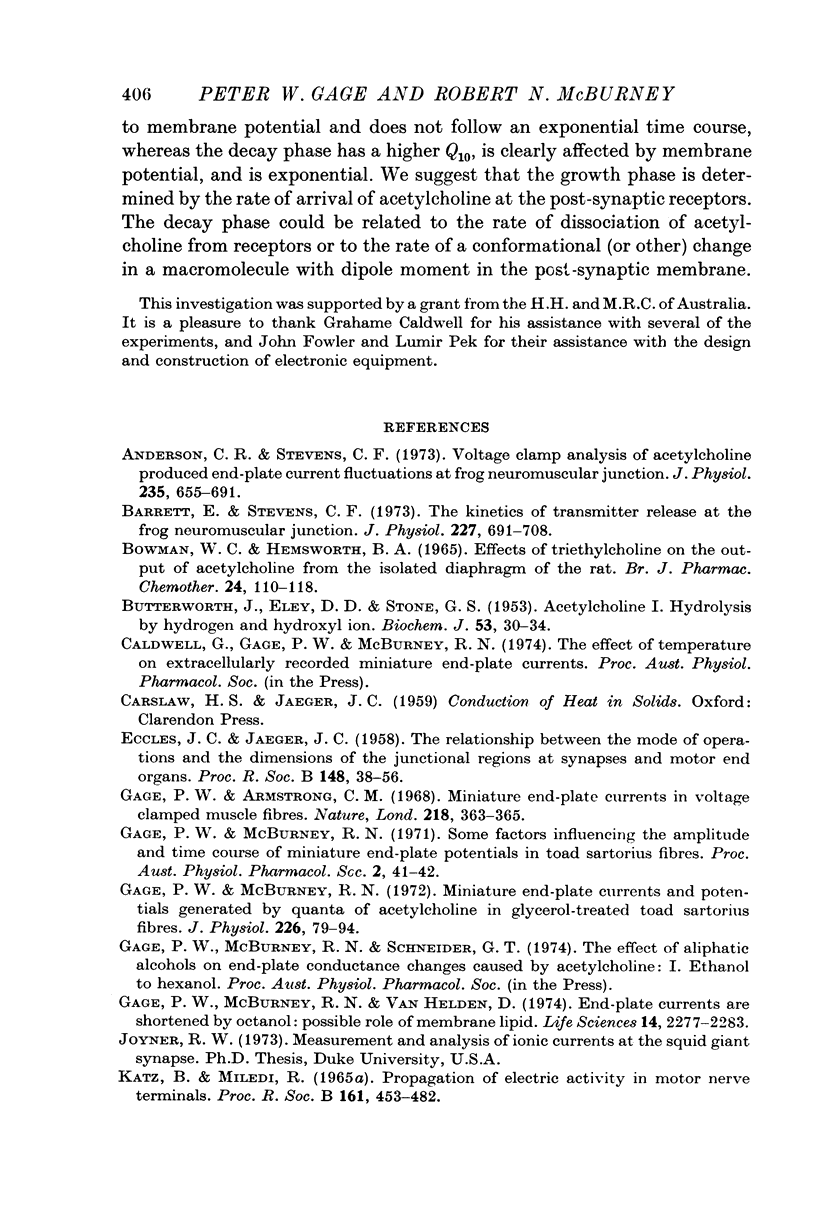
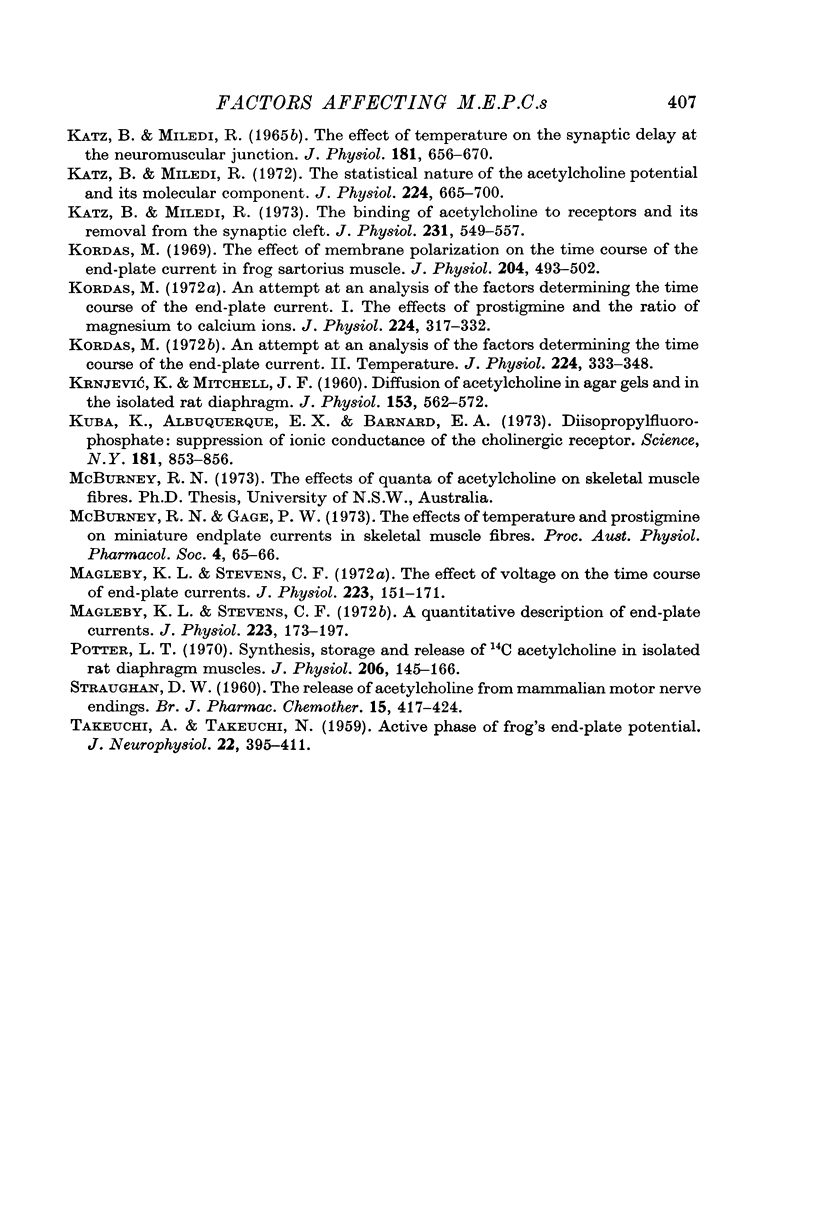
Selected References
These references are in PubMed. This may not be the complete list of references from this article.
- Anderson C. R., Stevens C. F. Voltage clamp analysis of acetylcholine produced end-plate current fluctuations at frog neuromuscular junction. J Physiol. 1973 Dec;235(3):655–691. doi: 10.1113/jphysiol.1973.sp010410. [DOI] [PMC free article] [PubMed] [Google Scholar]
- BOWMAN W. C., HEMSWORTH B. A. EFFECTS OF TRIETHYLCHOLINE ON THE OUTPUT OF ACETYLCHOLINE FROM THE ISOLATED DIAPHRAGM OF THE RAT. Br J Pharmacol Chemother. 1965 Feb;24:110–118. doi: 10.1111/j.1476-5381.1965.tb02084.x. [DOI] [PMC free article] [PubMed] [Google Scholar]
- BUTTERWORTH J., ELEY D. D., STONE G. S. Acetylcholine. I. Hydrolysis by hydrogen and hydroxyl ion. Biochem J. 1953 Jan;53(1):30–34. doi: 10.1042/bj0530030. [DOI] [PMC free article] [PubMed] [Google Scholar]
- Barrett E. F., Stevens C. F. The kinetics of transmitter release at the frog neuromuscular junction. J Physiol. 1972 Dec;227(3):691–708. doi: 10.1113/jphysiol.1972.sp010054. [DOI] [PMC free article] [PubMed] [Google Scholar]
- ECCLES J. C., JAEGER J. C. The relationship between the mode of operation and the dimensions of the junctional regions at synapses and motor end-organs. Proc R Soc Lond B Biol Sci. 1958 Jan 1;148(930):38–56. doi: 10.1098/rspb.1958.0003. [DOI] [PubMed] [Google Scholar]
- Gage P. W., Armstrong C. M. Miniature end-plate currents in voltage-clamped muscle fibre. Nature. 1968 Apr 27;218(5139):363–365. doi: 10.1038/218363b0. [DOI] [PubMed] [Google Scholar]
- Gage P. W., McBurney R. N. Miniature end-plate currents and potentials generated by quanta of acetylcholine in glycerol-treated toad sartorius fibres. J Physiol. 1972 Oct;226(1):79–94. doi: 10.1113/jphysiol.1972.sp009974. [DOI] [PMC free article] [PubMed] [Google Scholar]
- Gage P. W., McBurney R. N., Van Helden D. Endplate currents are shortened by octanol: possible role of membrane lipid. Life Sci. 1974 Jun 1;14(11):2277–2283. doi: 10.1016/0024-3205(74)90109-x. [DOI] [PubMed] [Google Scholar]
- KATZ B., MILEDI R. PROPAGATION OF ELECTRIC ACTIVITY IN MOTOR NERVE TERMINALS. Proc R Soc Lond B Biol Sci. 1965 Feb 16;161:453–482. doi: 10.1098/rspb.1965.0015. [DOI] [PubMed] [Google Scholar]
- KRNJEVIC K., MITCHELL J. F. Diffusion of acetylcholine in agar gels and in the isolated rat diaphragm. J Physiol. 1960 Oct;153:562–572. doi: 10.1113/jphysiol.1960.sp006555. [DOI] [PMC free article] [PubMed] [Google Scholar]
- Katz B., Miledi R. The binding of acetylcholine to receptors and its removal from the synaptic cleft. J Physiol. 1973 Jun;231(3):549–574. doi: 10.1113/jphysiol.1973.sp010248. [DOI] [PMC free article] [PubMed] [Google Scholar]
- Katz B., Miledi R. The effect of temperature on the synaptic delay at the neuromuscular junction. J Physiol. 1965 Dec;181(3):656–670. doi: 10.1113/jphysiol.1965.sp007790. [DOI] [PMC free article] [PubMed] [Google Scholar]
- Katz B., Miledi R. The statistical nature of the acetycholine potential and its molecular components. J Physiol. 1972 Aug;224(3):665–699. doi: 10.1113/jphysiol.1972.sp009918. [DOI] [PMC free article] [PubMed] [Google Scholar]
- Kordas M. An attempt at an analysis of the factors determining the time course of the end-plate current. I. The effects of prostigmine and of the ratio of Mg 2+ to Ca 2+ . J Physiol. 1972 Jul;224(2):317–332. doi: 10.1113/jphysiol.1972.sp009897. [DOI] [PMC free article] [PubMed] [Google Scholar]
- Kordas M. An attempt at an analysis of the factors determining the time course of the end-plate current. II. Temperature. J Physiol. 1972 Jul;224(2):333–348. doi: 10.1113/jphysiol.1972.sp009898. [DOI] [PMC free article] [PubMed] [Google Scholar]
- Kordas M. The effect of membrane polarization on the time course of the end-plate current in frog sartorius muscle. J Physiol. 1969 Oct;204(2):493–502. doi: 10.1113/jphysiol.1969.sp008926. [DOI] [PMC free article] [PubMed] [Google Scholar]
- Kuba K., Albuquerque E. X., Barnard E. A. Diisopropylfluorophosphate: suppression of ionic conductance of the cholinergic receptor. Science. 1973 Aug 31;181(4102):853–856. doi: 10.1126/science.181.4102.853. [DOI] [PubMed] [Google Scholar]
- Magleby K. L., Stevens C. F. A quantitative description of end-plate currents. J Physiol. 1972 May;223(1):173–197. doi: 10.1113/jphysiol.1972.sp009840. [DOI] [PMC free article] [PubMed] [Google Scholar]
- Magleby K. L., Stevens C. F. The effect of voltage on the time course of end-plate currents. J Physiol. 1972 May;223(1):151–171. doi: 10.1113/jphysiol.1972.sp009839. [DOI] [PMC free article] [PubMed] [Google Scholar]
- Potter L. T. Synthesis, storage and release of [14C]acetylcholine in isolated rat diaphragm muscles. J Physiol. 1970 Jan;206(1):145–166. doi: 10.1113/jphysiol.1970.sp009003. [DOI] [PMC free article] [PubMed] [Google Scholar]
- TAKEUCHI A., TAKEUCHI N. Active phase of frog's end-plate potential. J Neurophysiol. 1959 Jul;22(4):395–411. doi: 10.1152/jn.1959.22.4.395. [DOI] [PubMed] [Google Scholar]