Abstract
1. Light and electron micrographs of sections of the gastro-oesophageal giant neurone (G cell) of the nudibranch mollusc, Anisodoris nobilis, show that its somatic and axonal membranes are deeply infolded. The surface and volume of its soma and axon have been calculated from measurements taken at the light and electron microscope on sections of the G cell.
2. The surface of the soma is approximately 7·5 times as large as that of a sphere having the same volume. For a typical cell the soma has a volume of 1·5 × 10-5 cm3 and a surface of 2 × 10-2 cm2; the axon has a volume of 5 × 10-5 cm3 and a surface of 5 × 10-1 cm2.
3. Because the axon is star shaped in cross-section, its geometry cannot be described by a single parameter (diameter or radius). Furthermore, the axon is beaded, and both the area (A) and the perimeter (P) of its cross-section change from point to point.
4. However, in spite of the apparent irregularity of their cross-sections, all axons examined could be characterized by a constant A/P ratio. This ratio also remains constant when the axons are stretched.
5. According to the equations derived in the Appendix, the geometrical factor for the length constant in a folded fibre is H = √(A/P); therefore, in the G cell the length constant (and hence the conduction velocity) should be independent of the stretch applied to the axon.
6. The geometrical factor required to calculate the axonal input conductance is M = √(A.P). M changes in adjacent segments of the same axon; in each segment its value depends on how much the axon is stretched.
7. The input conductance of the whole axon can be calculated by applying a modified form of Rall's equations for dendritic trees. The results suggest that the input conductance of the G cell axon should vary with stretch and should be large in comparison to that of the soma.
Full text
PDF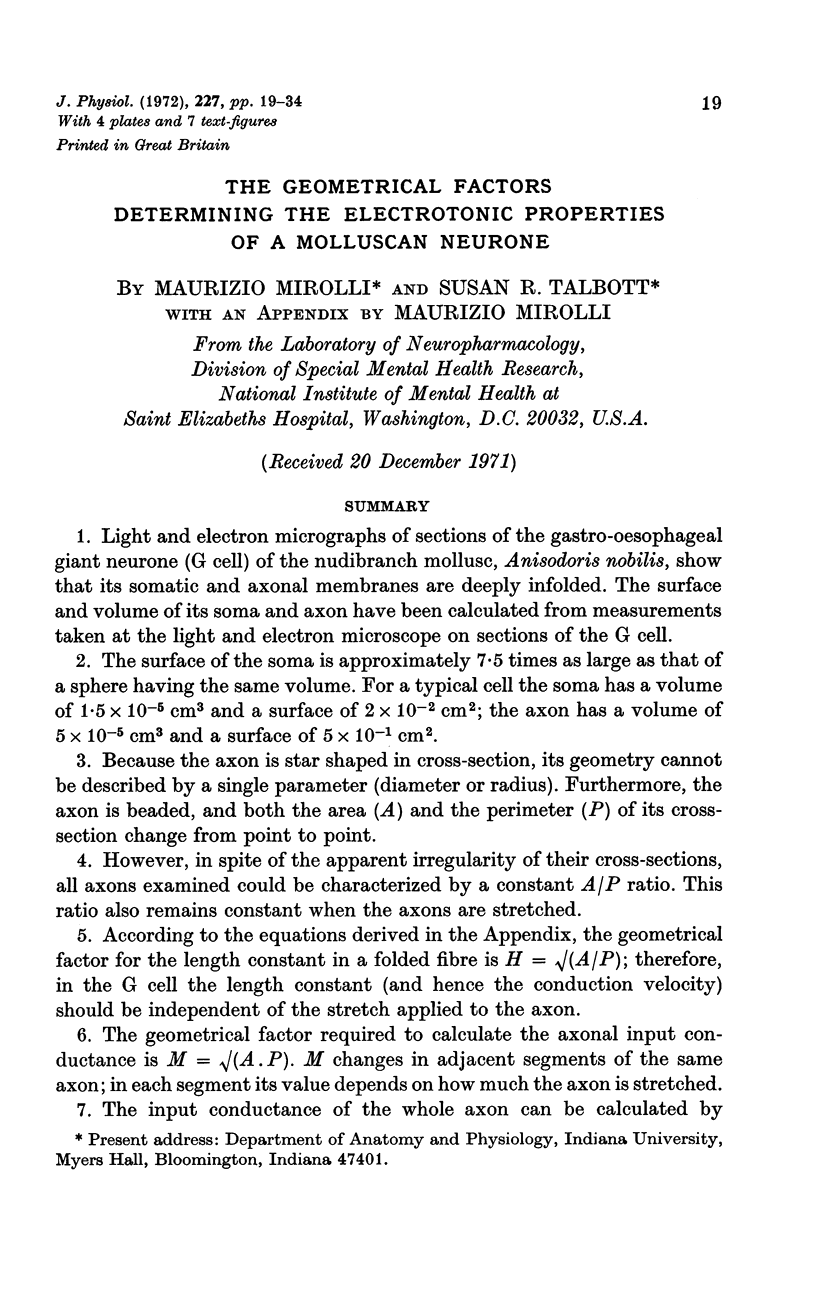
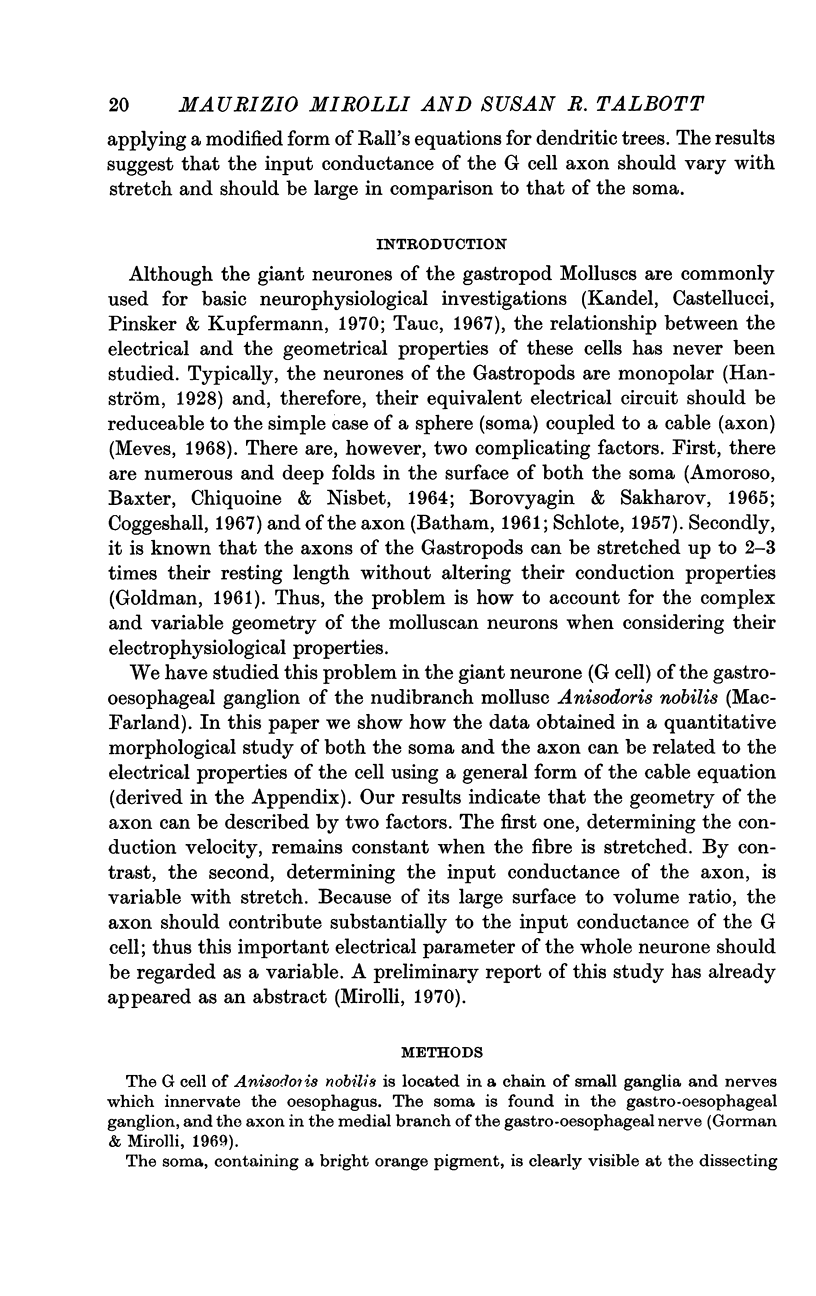
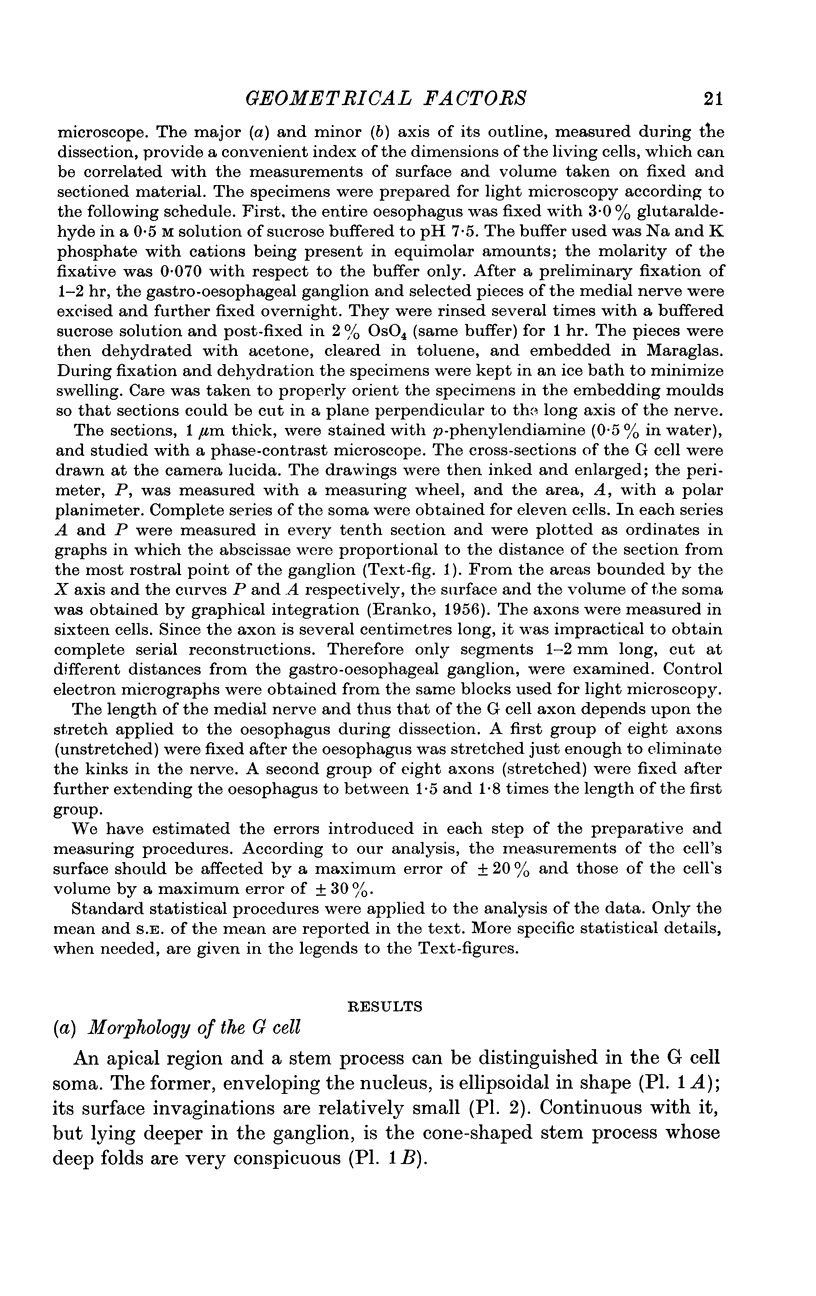
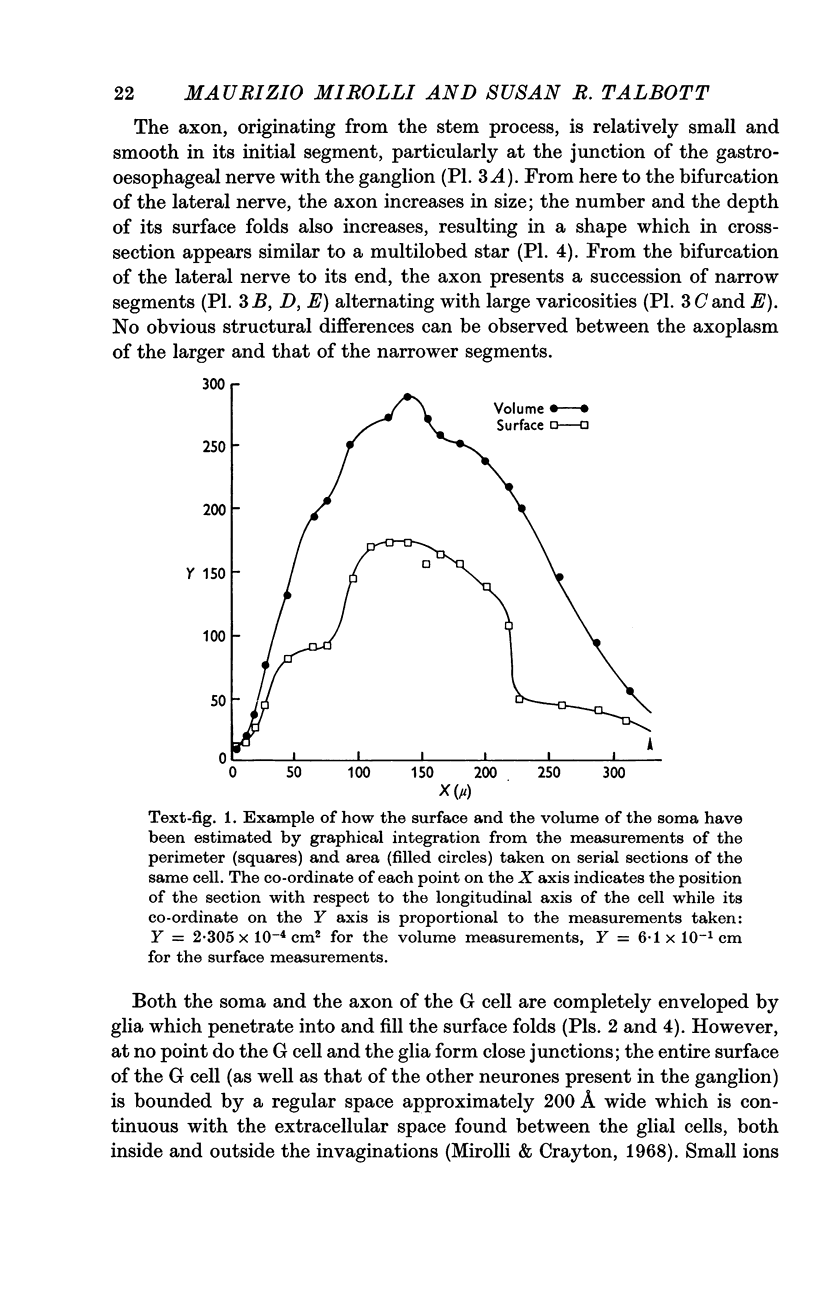
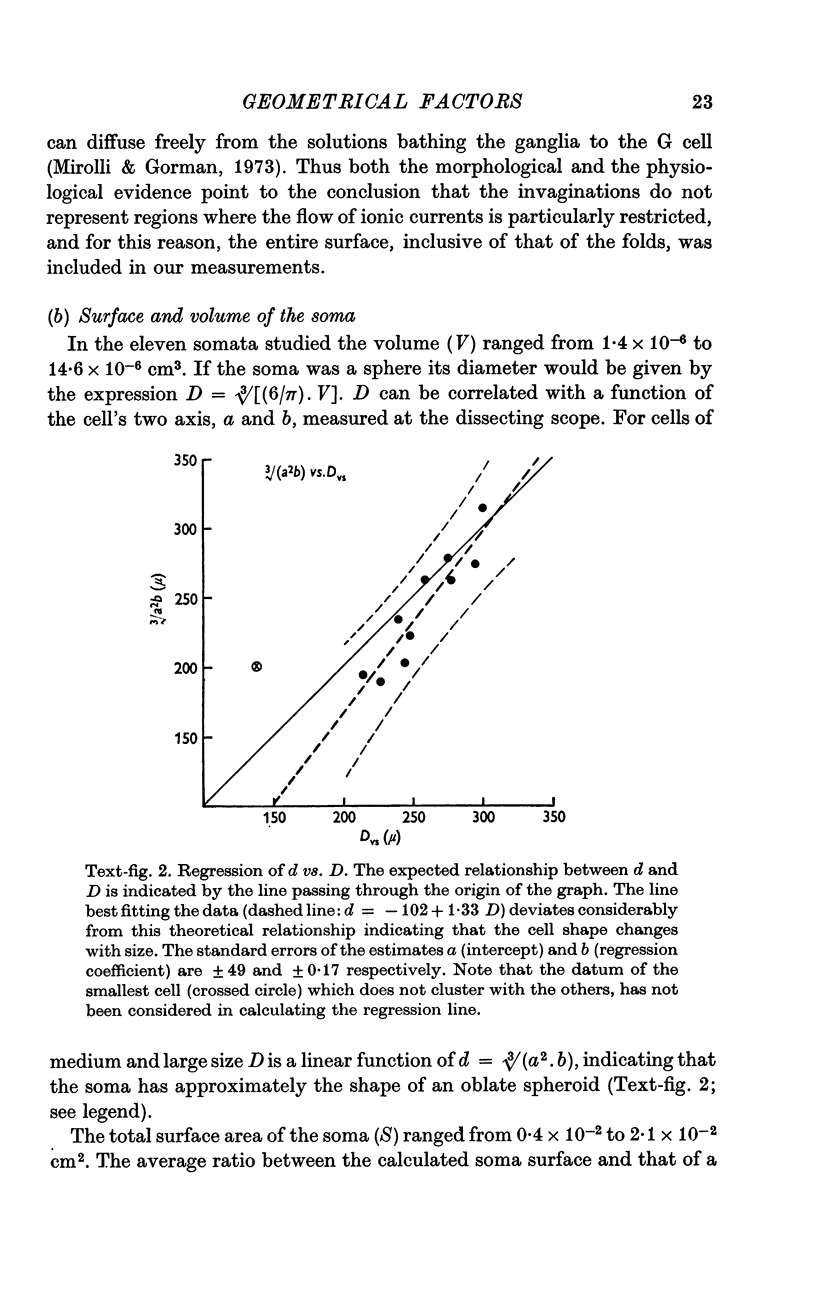
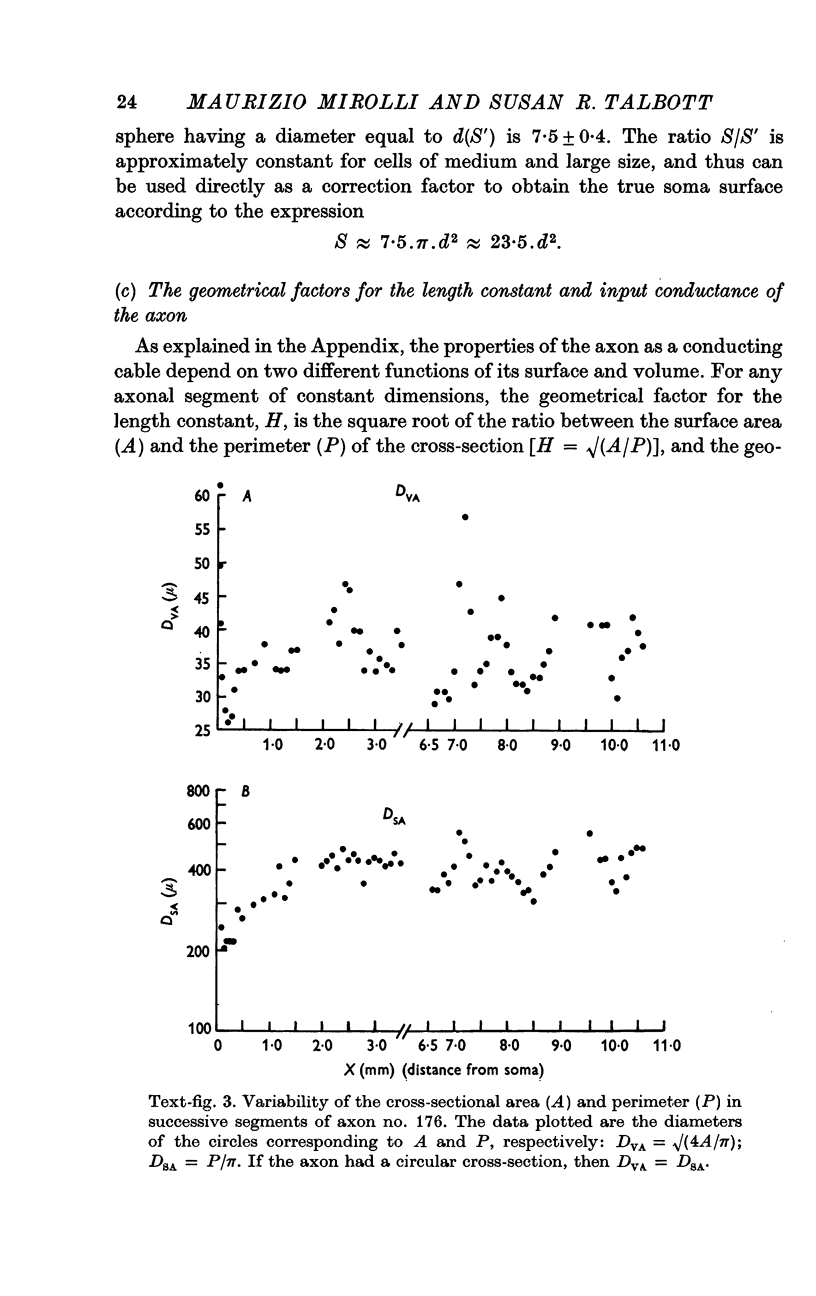
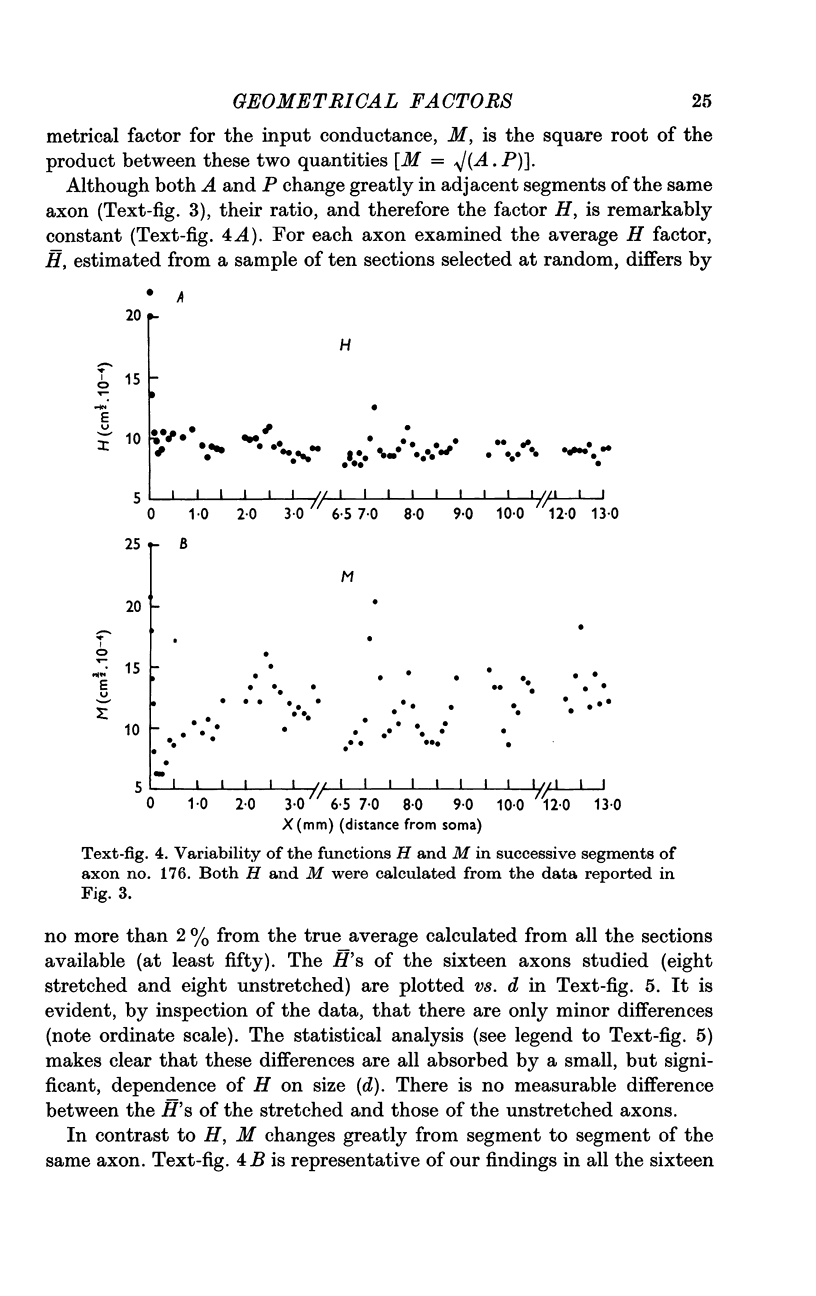
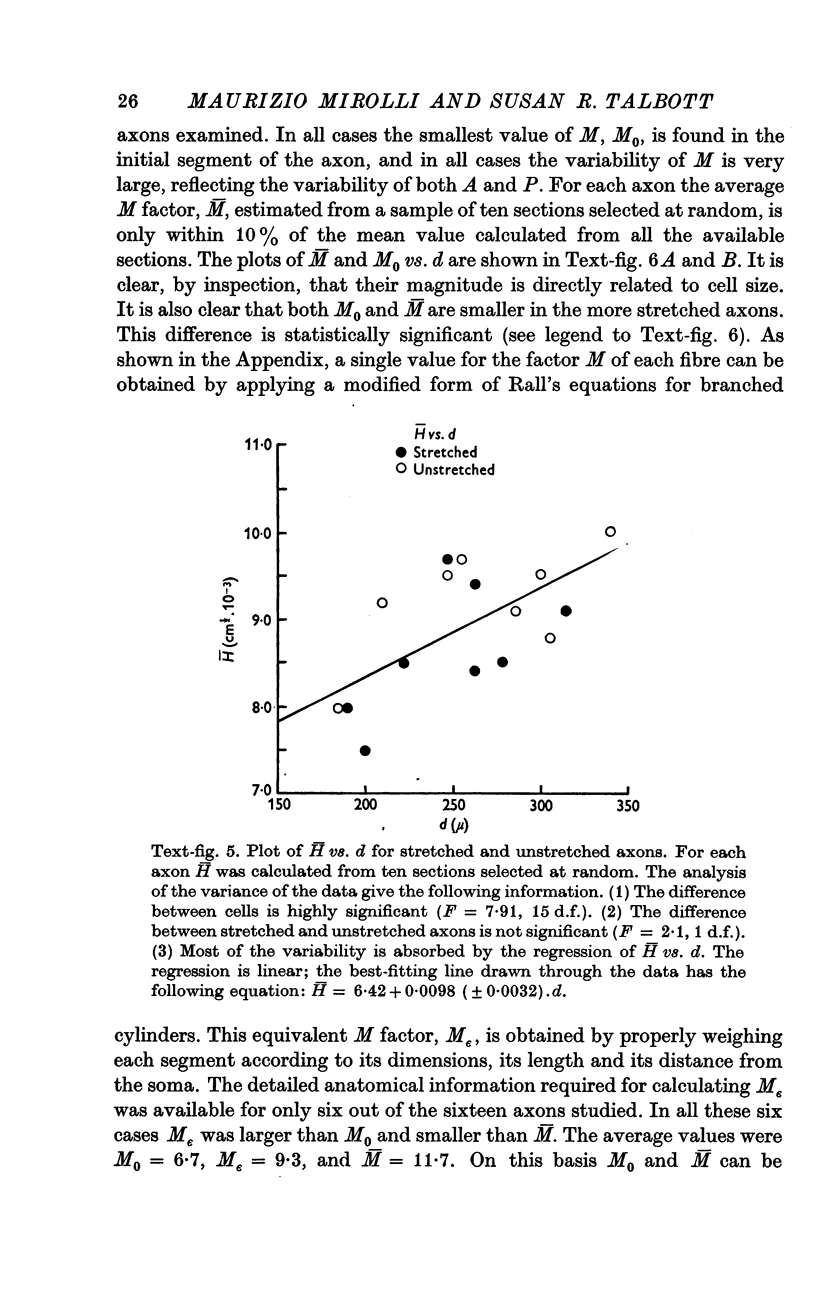
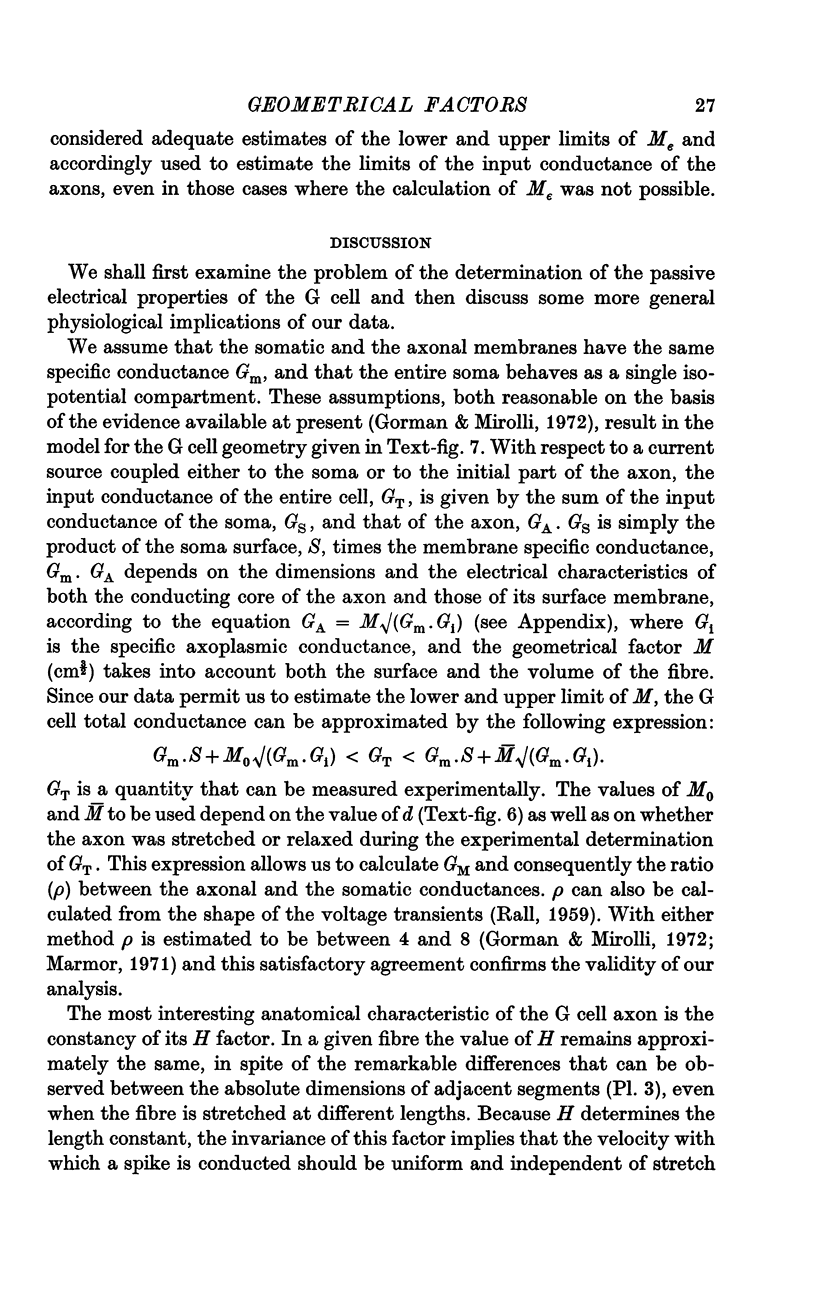
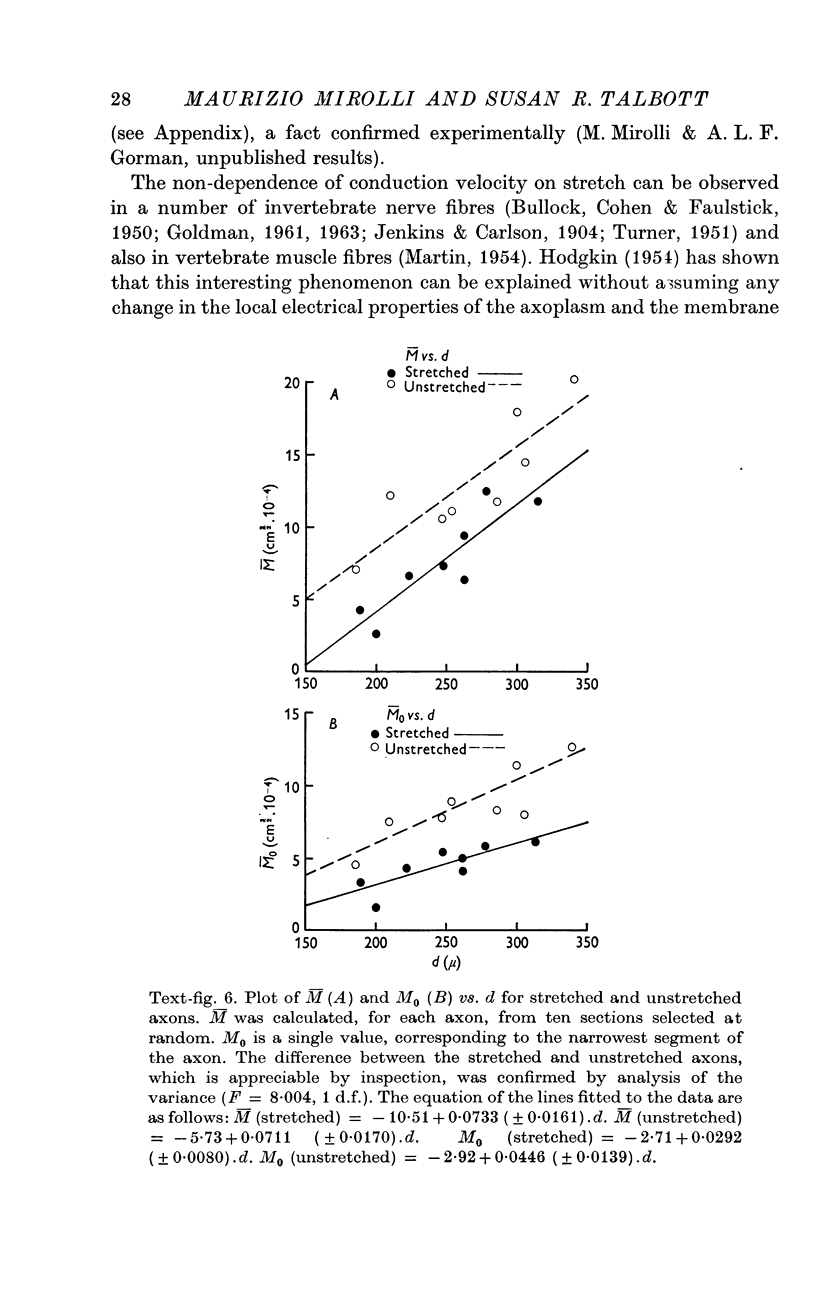
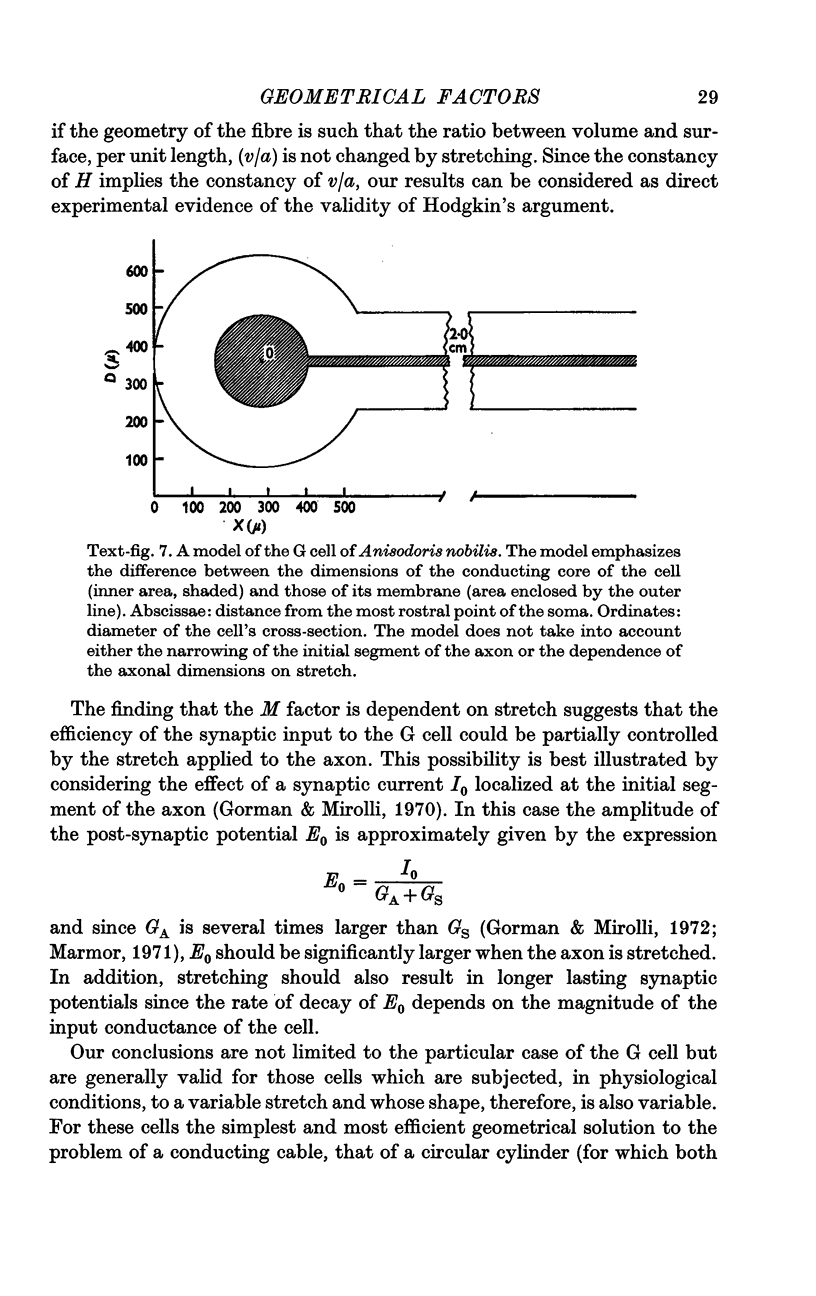
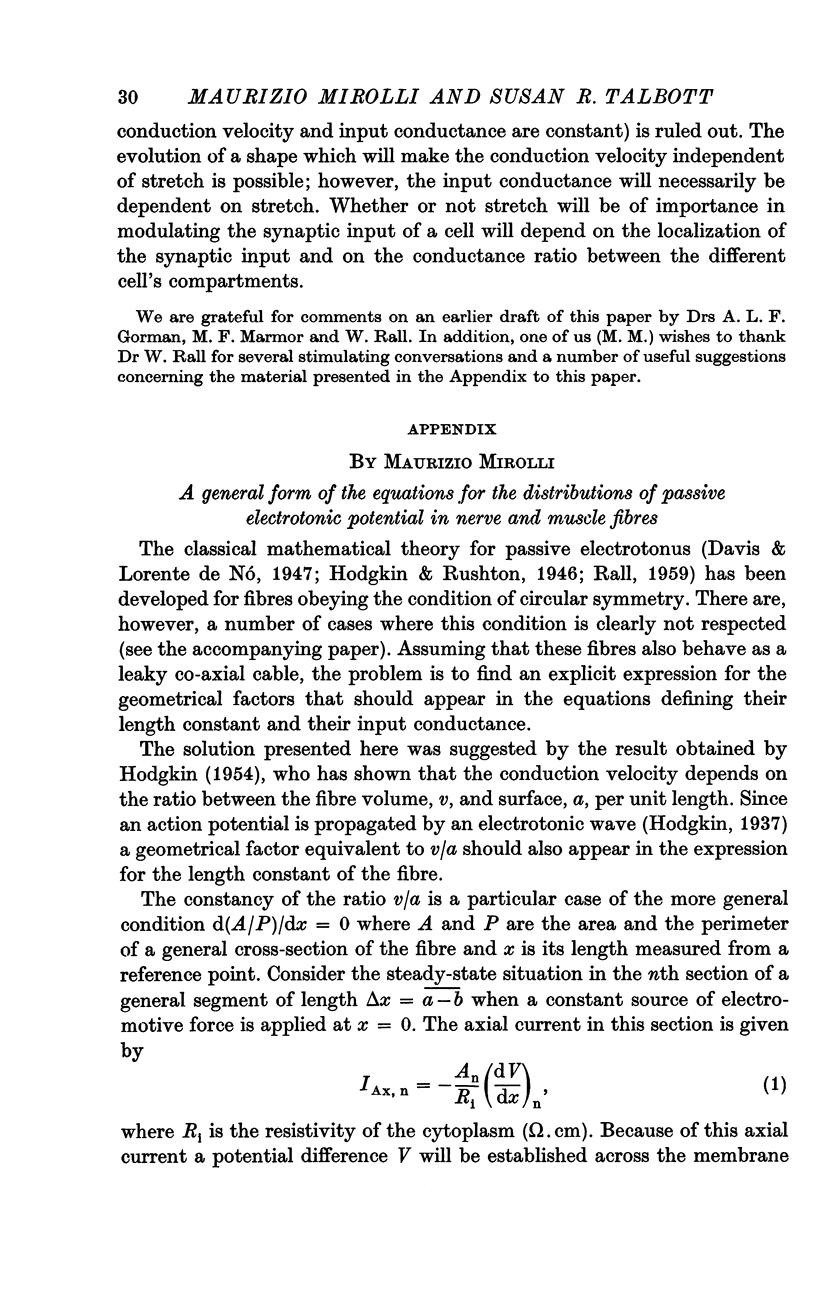
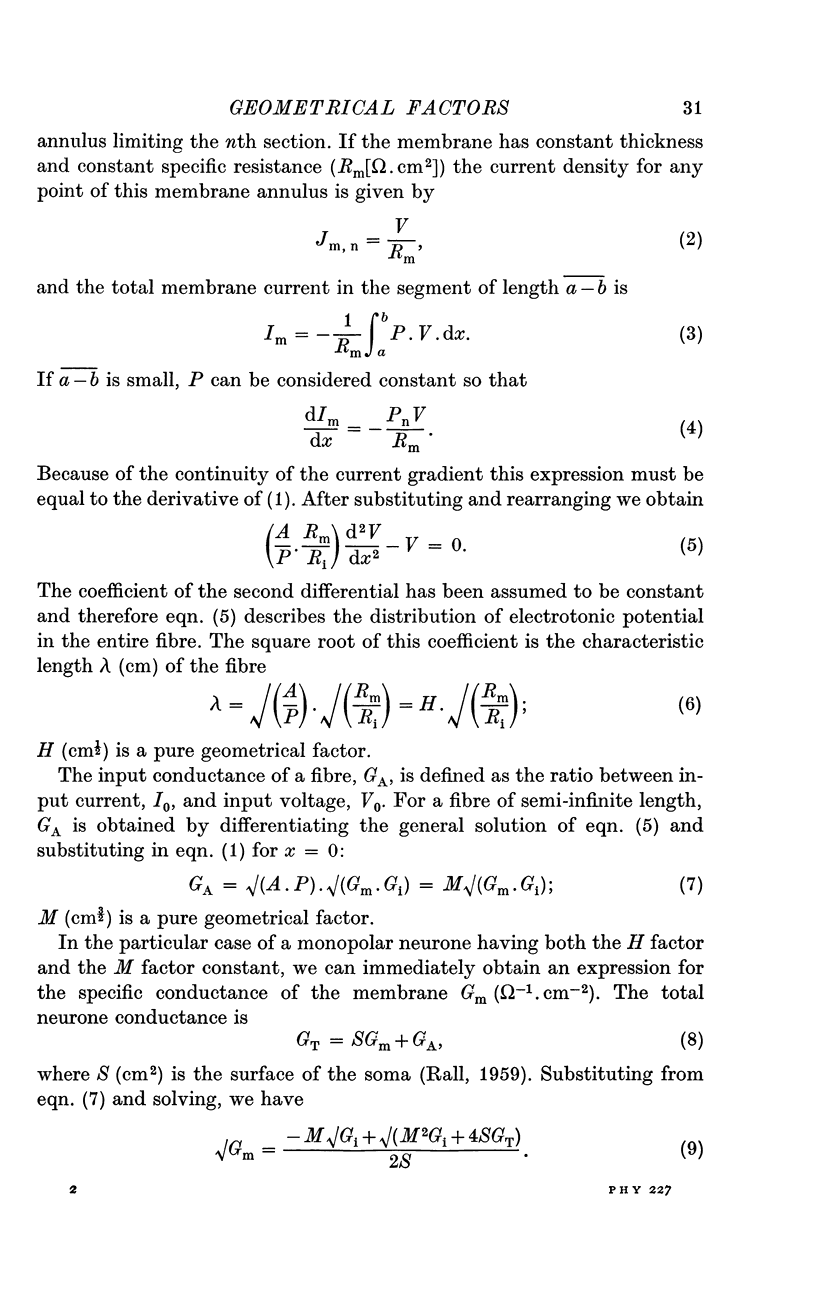
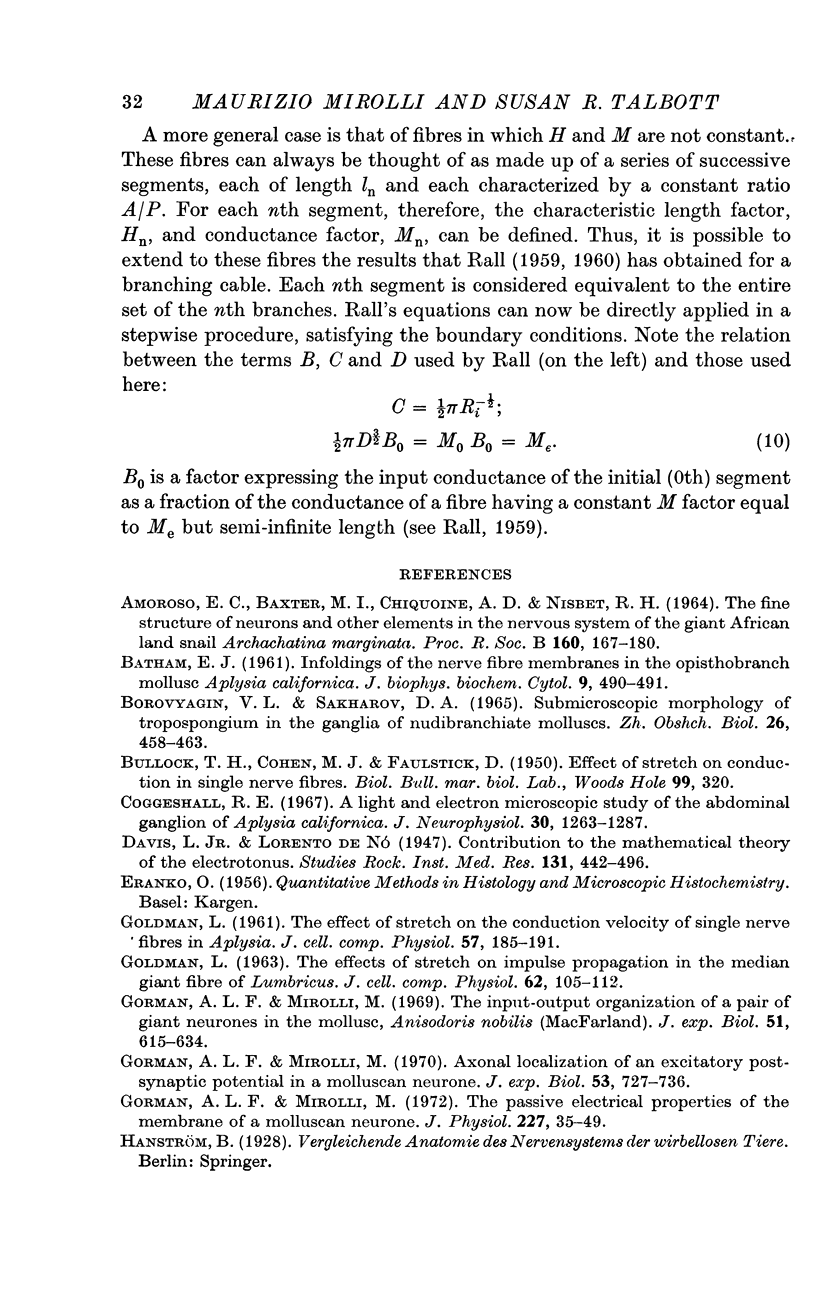
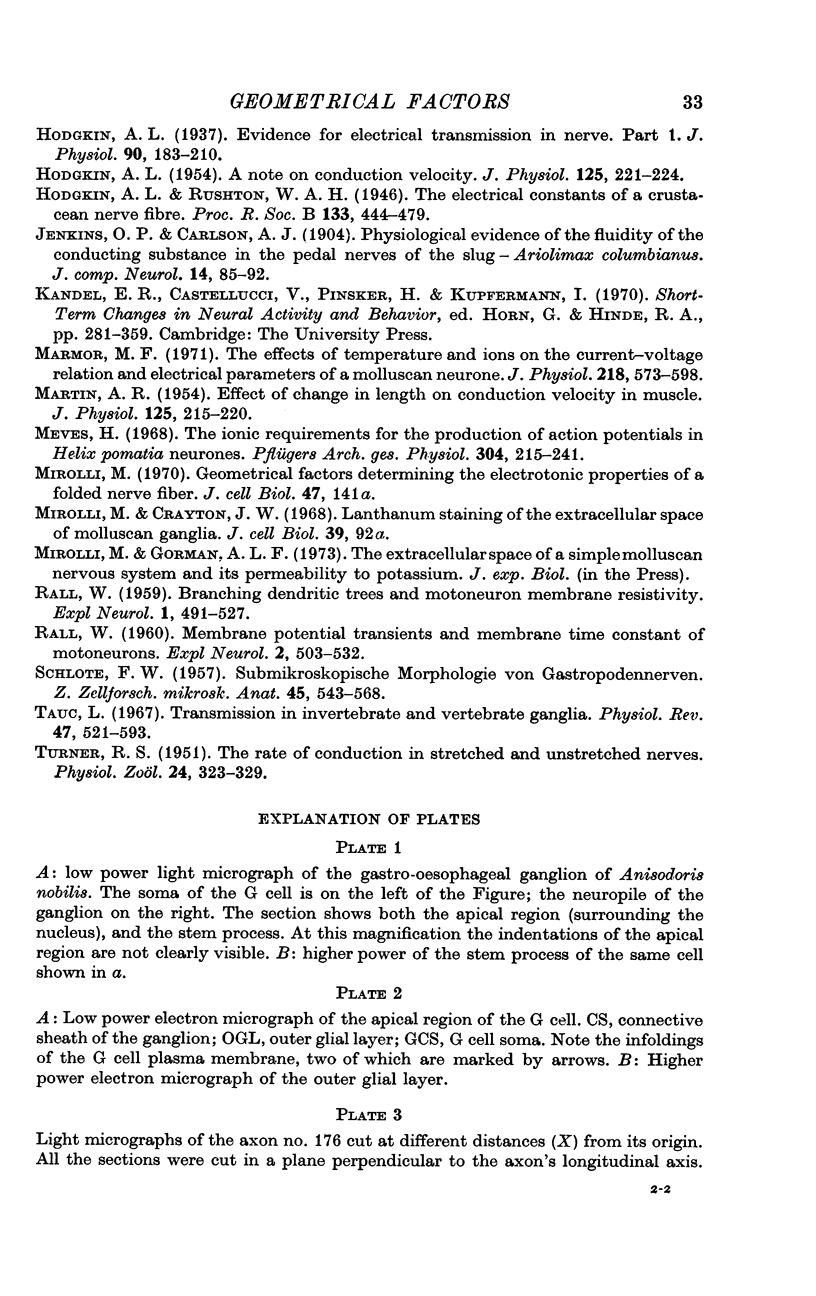
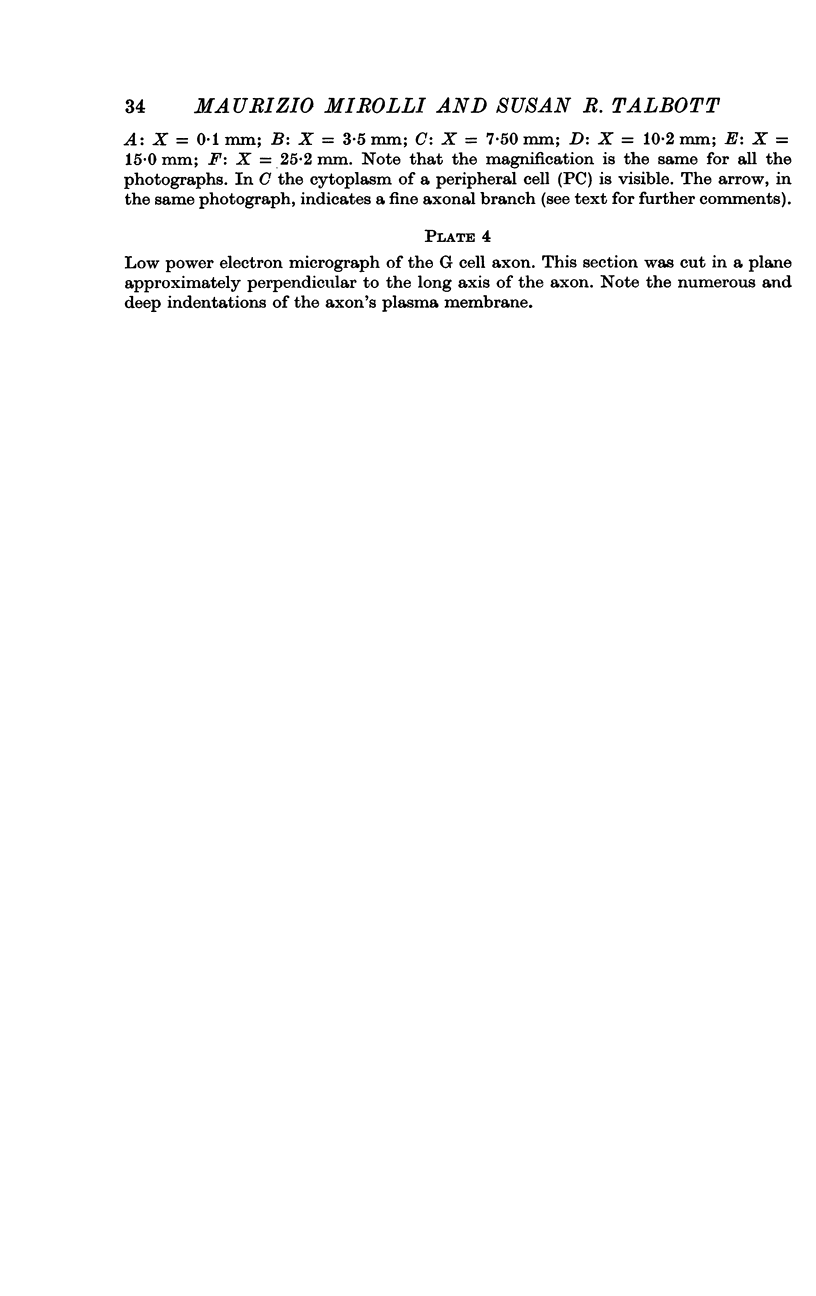
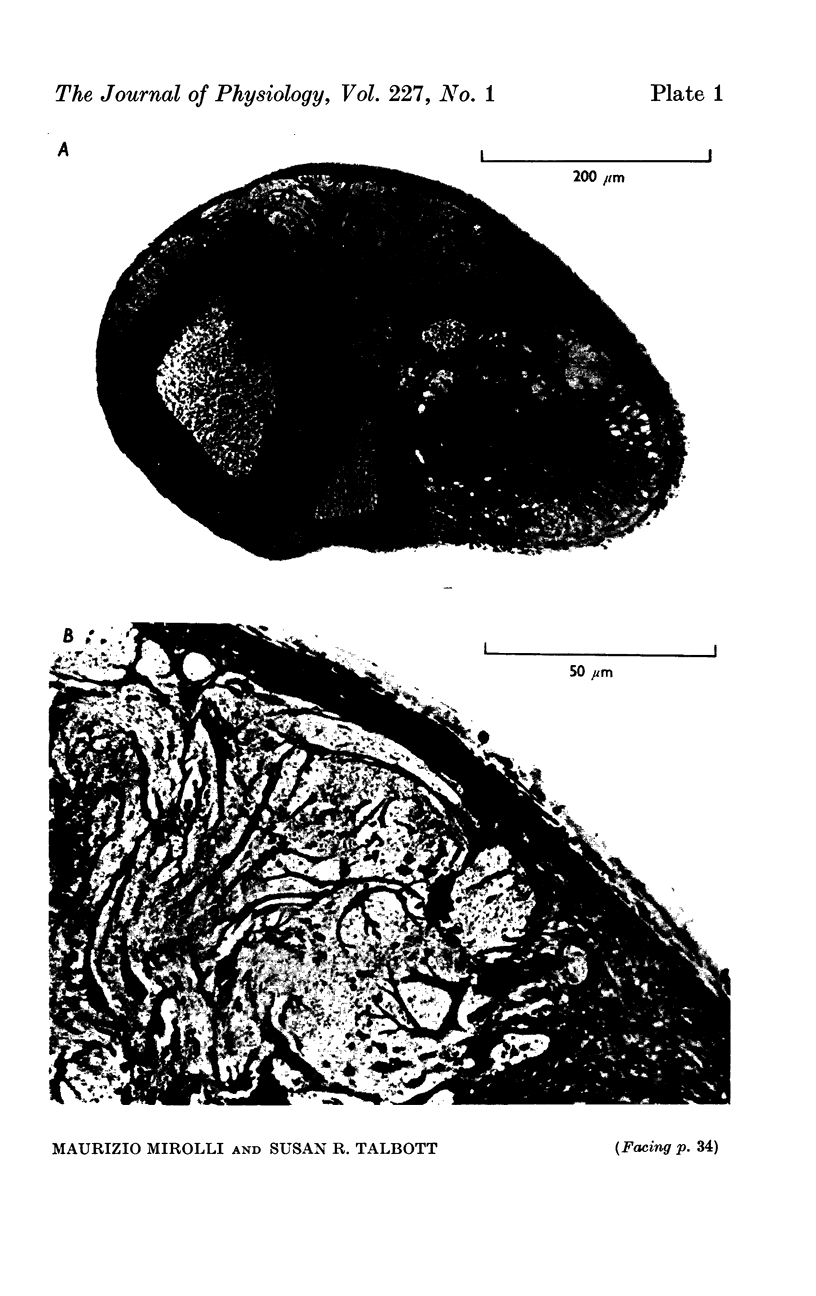
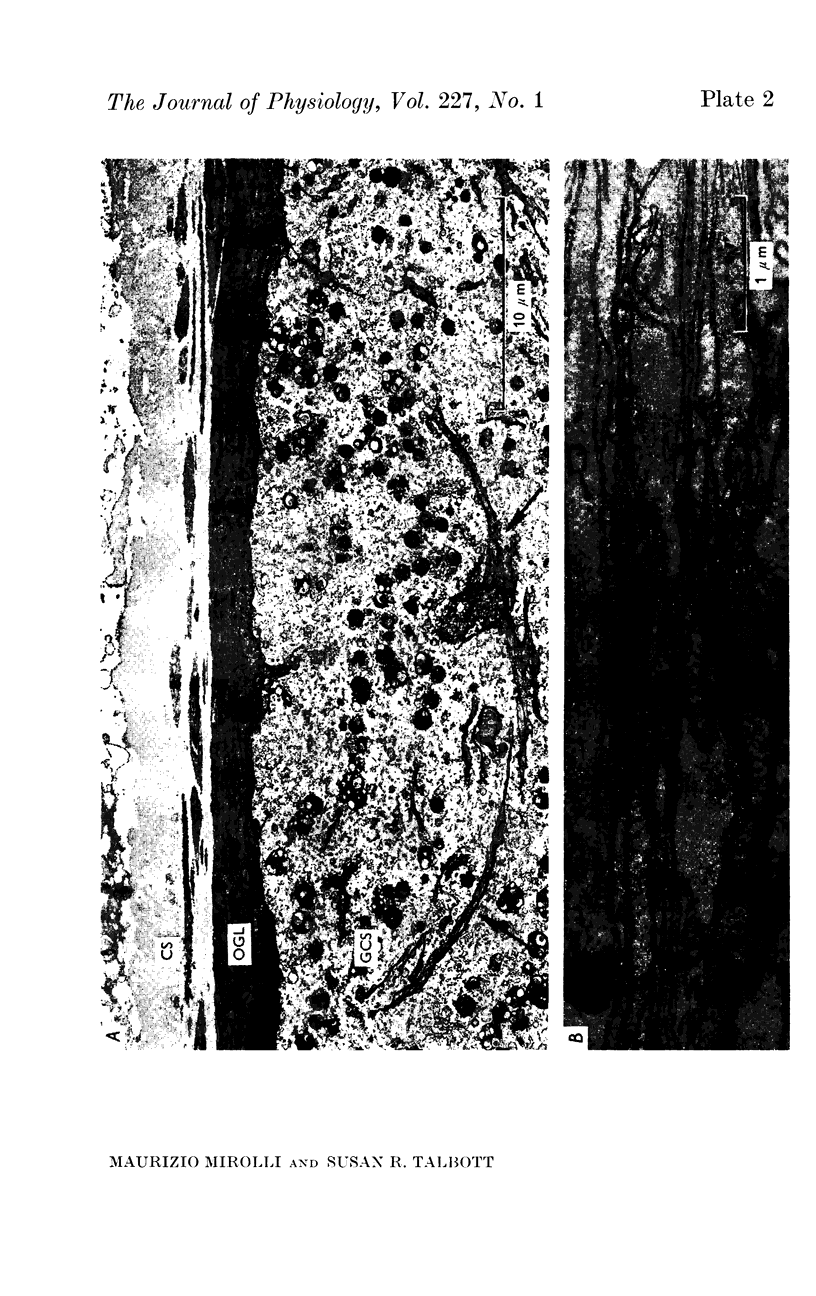
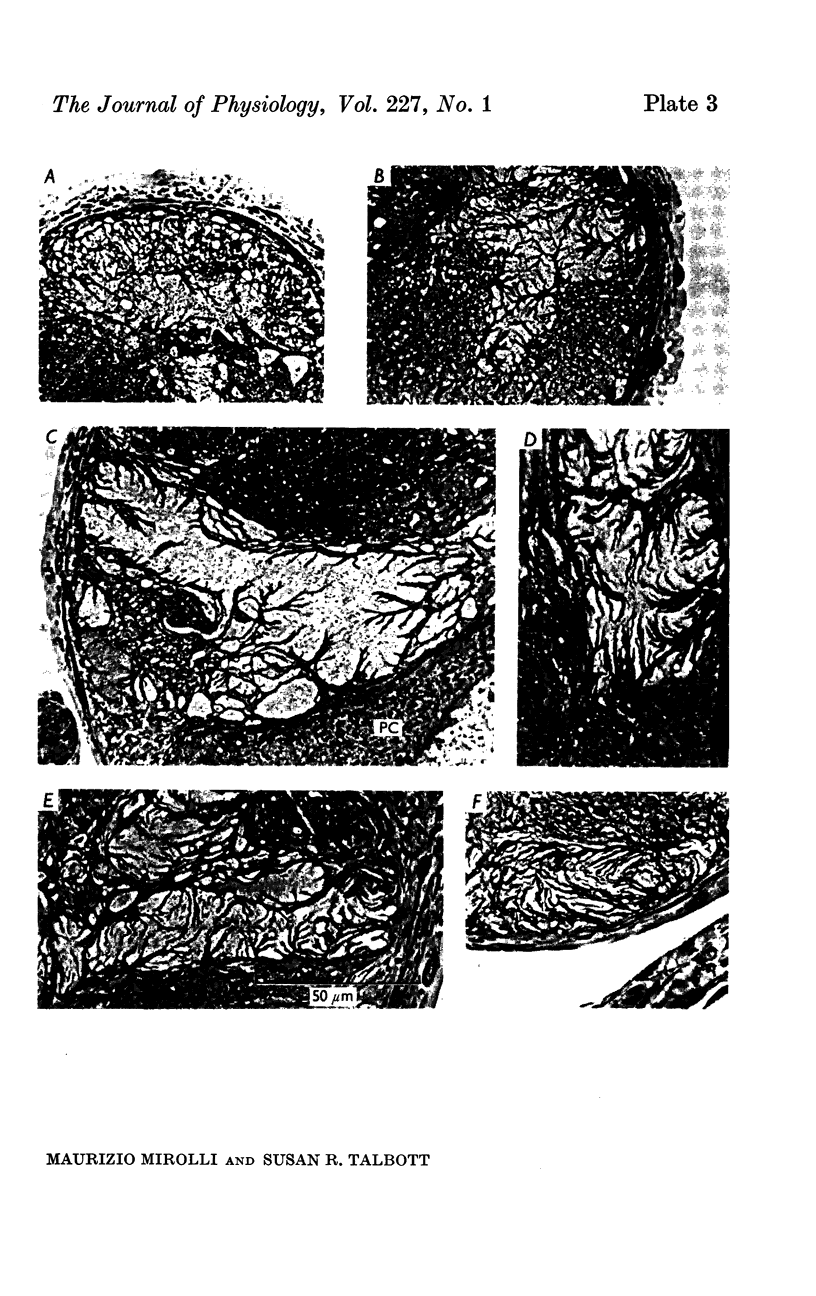
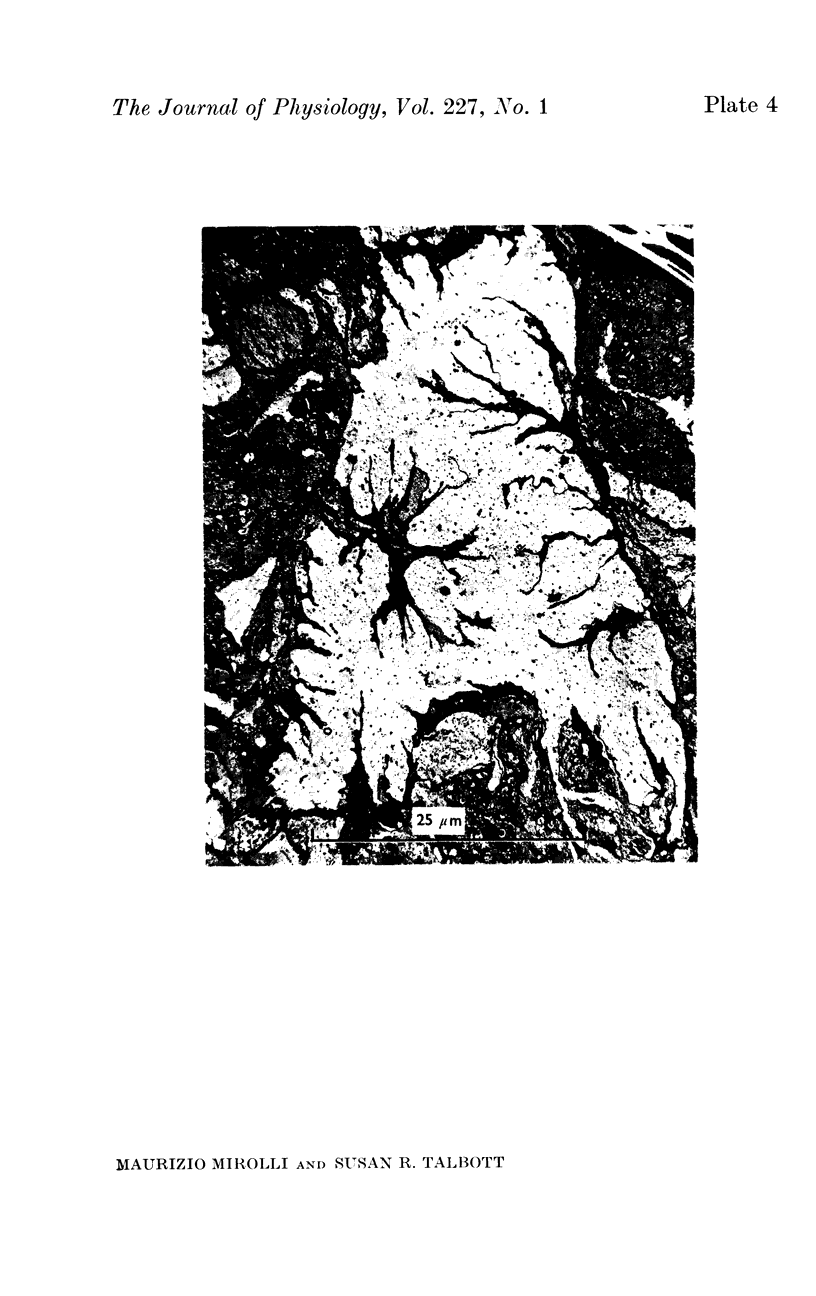
Images in this article
Selected References
These references are in PubMed. This may not be the complete list of references from this article.
- BATHAM E. J. Infoldings of nerve fibre membranes in the opisthobranch mollusc Aplysia californica. J Biophys Biochem Cytol. 1961 Feb;9:490–492. doi: 10.1083/jcb.9.2.490. [DOI] [PMC free article] [PubMed] [Google Scholar]
- BULLOCK T. H., COHEN M. J., FAULSTICK D. Effect of stretch on conduction in single nerve fibers. Biol Bull. 1950 Oct;99(2):320–320. doi: 10.1086/BBLv99n2p308. [DOI] [PubMed] [Google Scholar]
- Boroviagin V. L., Sakharov D. A. Submikroskopicheskaia morfologiia trofospongiia v ganglii golozhabernogo molliuska. Zh Obshch Biol. 1965 Jul-Aug;26(4):458–463. [PubMed] [Google Scholar]
- Coggeshall R. E. A light and electron microscope study of the abdominal ganglion of Aplysia californica. J Neurophysiol. 1967 Nov;30(6):1263–1287. doi: 10.1152/jn.1967.30.6.1263. [DOI] [PubMed] [Google Scholar]
- GOLDMAN L. THE EFFECTS OF STRETCH ON IMPULSE PROPAGATION IN THE MEDIAN GIANT FIBER OF LUMBRICUS. J Cell Physiol. 1963 Aug;62:105–112. doi: 10.1002/jcp.1030620112. [DOI] [PubMed] [Google Scholar]
- GOLDMAN L. The effect of stretch on the conduction velocity of single nerve fibers in Aplysia. J Cell Comp Physiol. 1961 Jun;57:185–191. doi: 10.1002/jcp.1030570306. [DOI] [PubMed] [Google Scholar]
- Gorman A. L., Mirolli M. Axonal localization of an excitatory post-synaptic potential in a molluscan neurone. J Exp Biol. 1970 Dec;53(3):727–736. doi: 10.1242/jeb.53.3.727. [DOI] [PubMed] [Google Scholar]
- Gorman A. L., Mirolli M. The passive electrical properties of the membrane of a molluscan neurone. J Physiol. 1972 Dec;227(1):35–49. doi: 10.1113/jphysiol.1972.sp010018. [DOI] [PMC free article] [PubMed] [Google Scholar]
- HODGKIN A. L. A note on conduction velocity. J Physiol. 1954 Jul 28;125(1):221–224. doi: 10.1113/jphysiol.1954.sp005152. [DOI] [PMC free article] [PubMed] [Google Scholar]
- Hodgkin A. L. Evidence for electrical transmission in nerve: Part I. J Physiol. 1937 Jul 15;90(2):183–210. doi: 10.1113/jphysiol.1937.sp003507. [DOI] [PMC free article] [PubMed] [Google Scholar]
- MARTIN A. R. The effect of change in length on conduction velocity in muscle. J Physiol. 1954 Jul 28;125(1):215–220. doi: 10.1113/jphysiol.1954.sp005151. [DOI] [PMC free article] [PubMed] [Google Scholar]
- Marmor M. F. The effects of temperature and ions on the current-voltage relation and electrical characteristics of a molluscan neurone. J Physiol. 1971 Nov;218(3):573–598. doi: 10.1113/jphysiol.1971.sp009634. [DOI] [PMC free article] [PubMed] [Google Scholar]
- Meves H. The ionic requirements for the production of action potentials in helix pomatia neurones. Pflugers Arch. 1968;304(3):215–241. doi: 10.1007/BF00592126. [DOI] [PubMed] [Google Scholar]
- RALL W. Branching dendritic trees and motoneuron membrane resistivity. Exp Neurol. 1959 Nov;1:491–527. doi: 10.1016/0014-4886(59)90046-9. [DOI] [PubMed] [Google Scholar]
- RALL W. Membrane potential transients and membrane time constant of motoneurons. Exp Neurol. 1960 Oct;2:503–532. doi: 10.1016/0014-4886(60)90029-7. [DOI] [PubMed] [Google Scholar]
- SCHLOTE F. W. Submikroskopische Morphologie von Gastropodennerven. Z Zellforsch Mikrosk Anat. 1957;45(5):543–568. [PubMed] [Google Scholar]
- Tauc L. Transmission in invertebrate and vertebrate ganglia. Physiol Rev. 1967 Jul;47(3):521–593. doi: 10.1152/physrev.1967.47.3.521. [DOI] [PubMed] [Google Scholar]