Abstract
1. When light scattering was measured during hyperpolarizing and depolarizing voltage-clamp steps, relatively large scattering changes were found during the depolarizing steps. These large changes were found to depend on the time integral of the ionic current and not on the changes in conductance or potential.
2. The current-dependent changes were examined at several scattering angles, and three distinct time courses were found. At 30-120°, the main change occurred after the current when steps of 2-5 msec duration were used. This change was called I-90°. At 15-30°, the change occurred with the same time course as the time integral of the current. This change was called I-25°. At 5-15° the scattering change occurred with a time course intermediate between that of I-90° and I-25°. This change was called I-10°.
3. In all experiments, outward potassium and outward sodium currents led to similar light scattering changes indicating that specific effects of the cation carrying the current across the membrane were not involved.
4. The size of I-90° was reduced by 29% when an isethionate artificial sea water was substituted for the normal chloride artificial sea water. This reduction equalled the reduction predicted for a transport number effect at the membrane-solution interface. The time course of I-90° was similar to the predicted time course for a volume change in the periaxonal space, and such volume changes were tentatively identified as the origin of I-90°.
5. Because of difficulties in measuring the time course of I-25°, it was not possible to distinguish between a water of hydration effect and a transport number effect as the cause of this change. Similarly, the origins of I-10° were not identified. Only I-10° was altered in size and time course when the external refractive index was increased with bovine albumin.
6. When the scattering changes during the action potential were examined in light of the voltage-clamp experiments, we concluded that the forward-angle change was potential-dependent and that the long-lasting change at right angles probably represented a swelling of the periaxonal space resulting from the fact that chloride carried a significant fraction of the outward current during the action potential.
Full text
PDF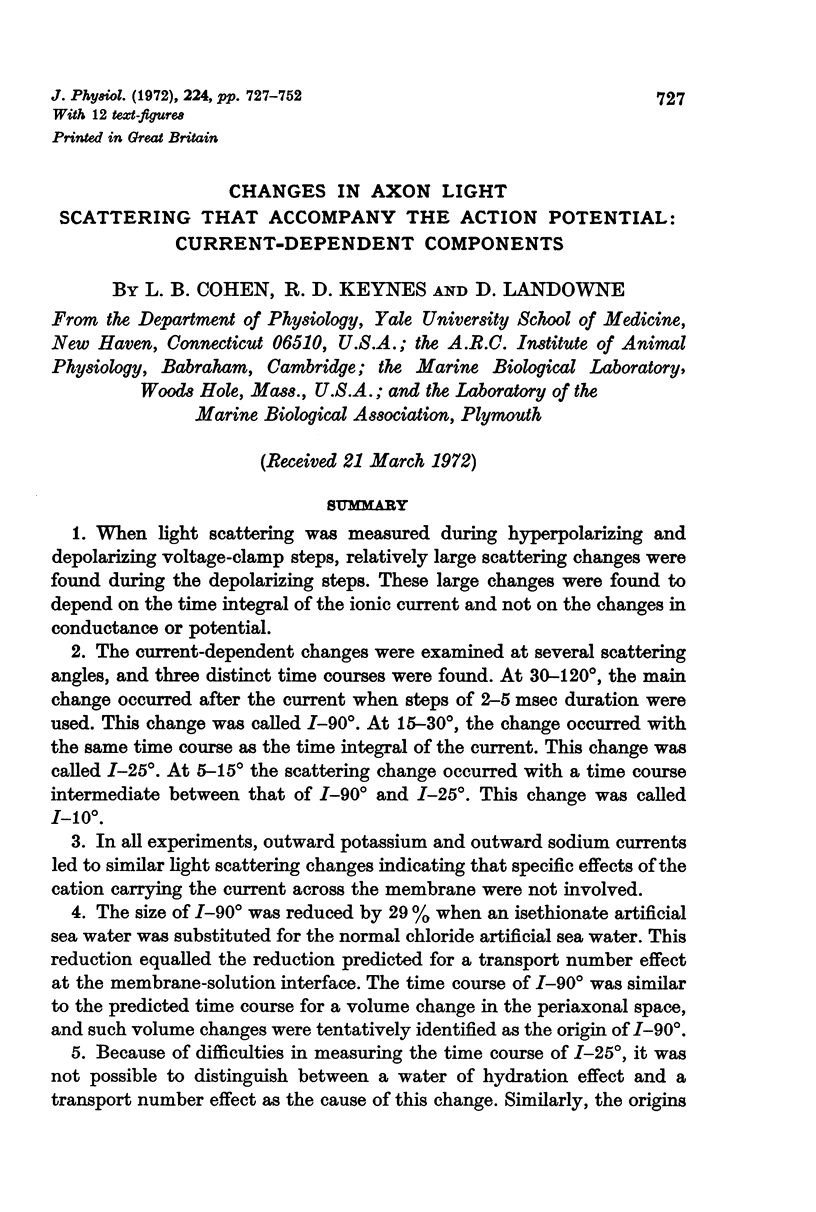
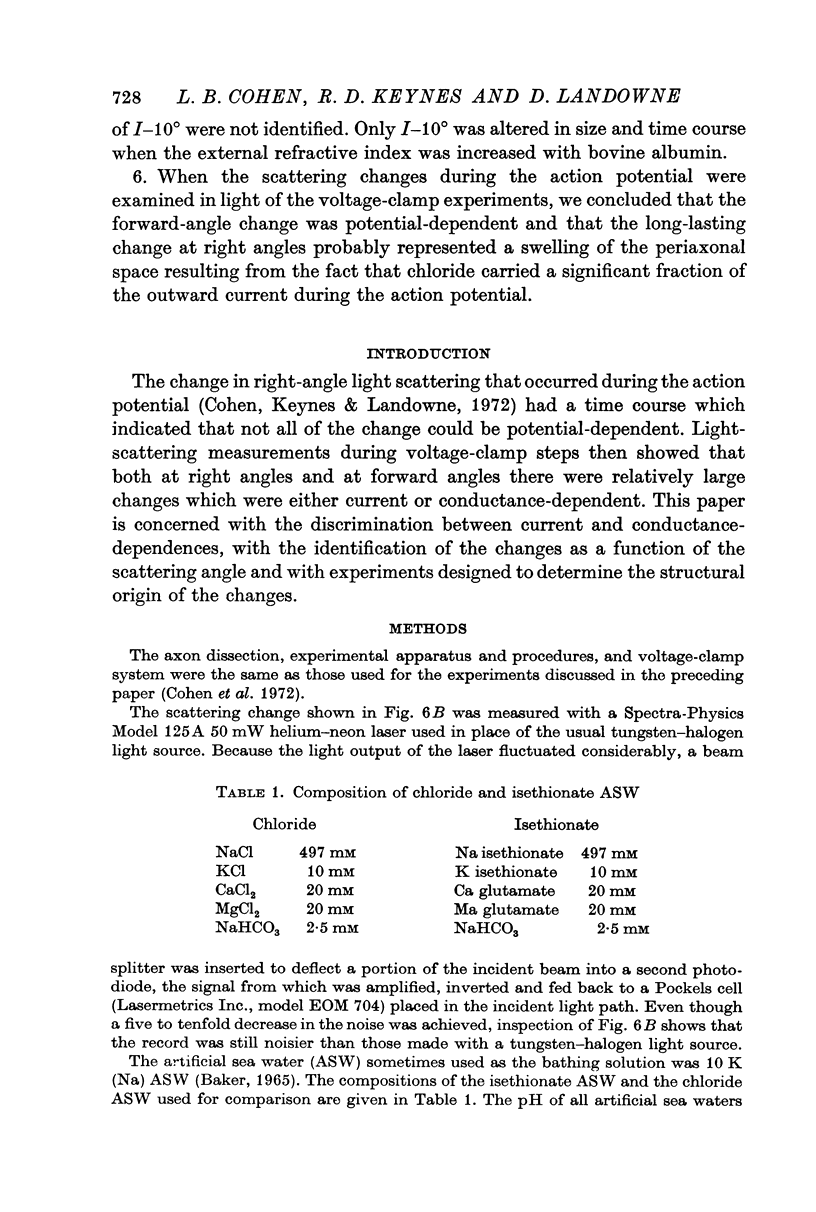
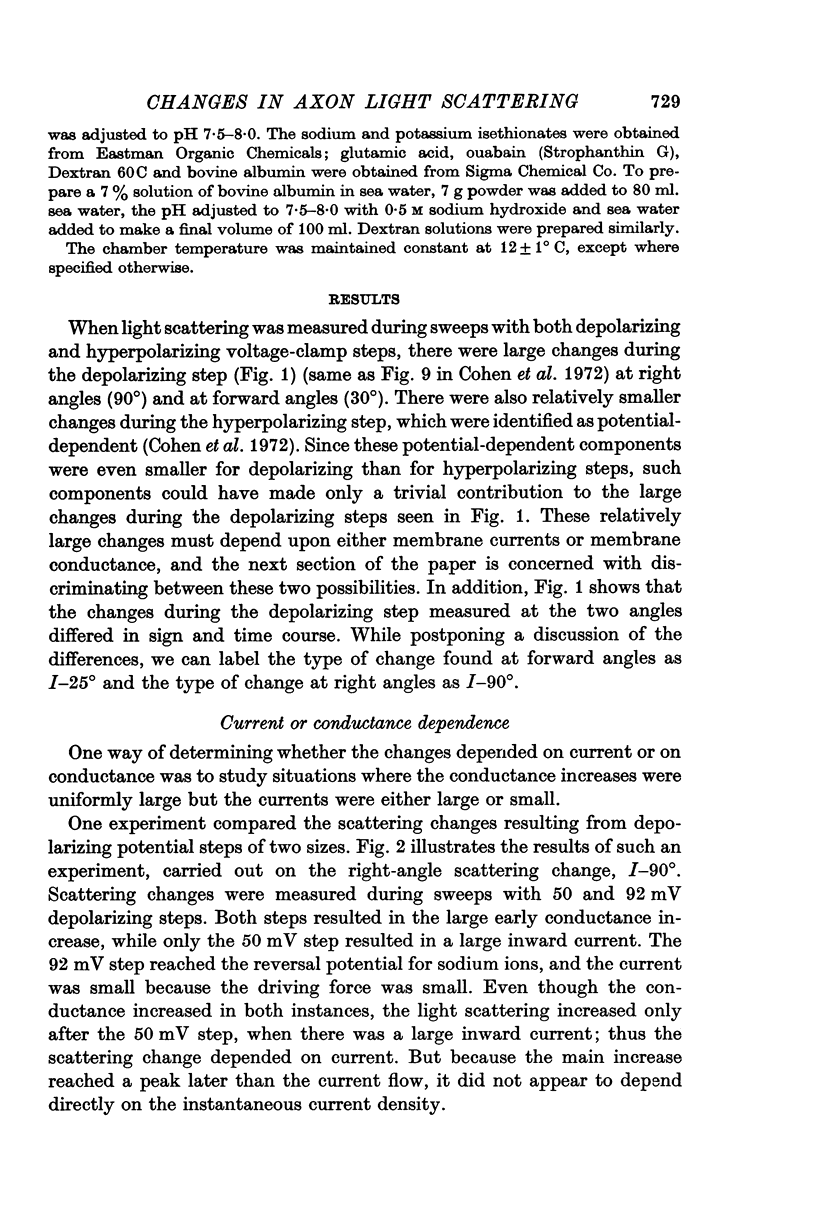
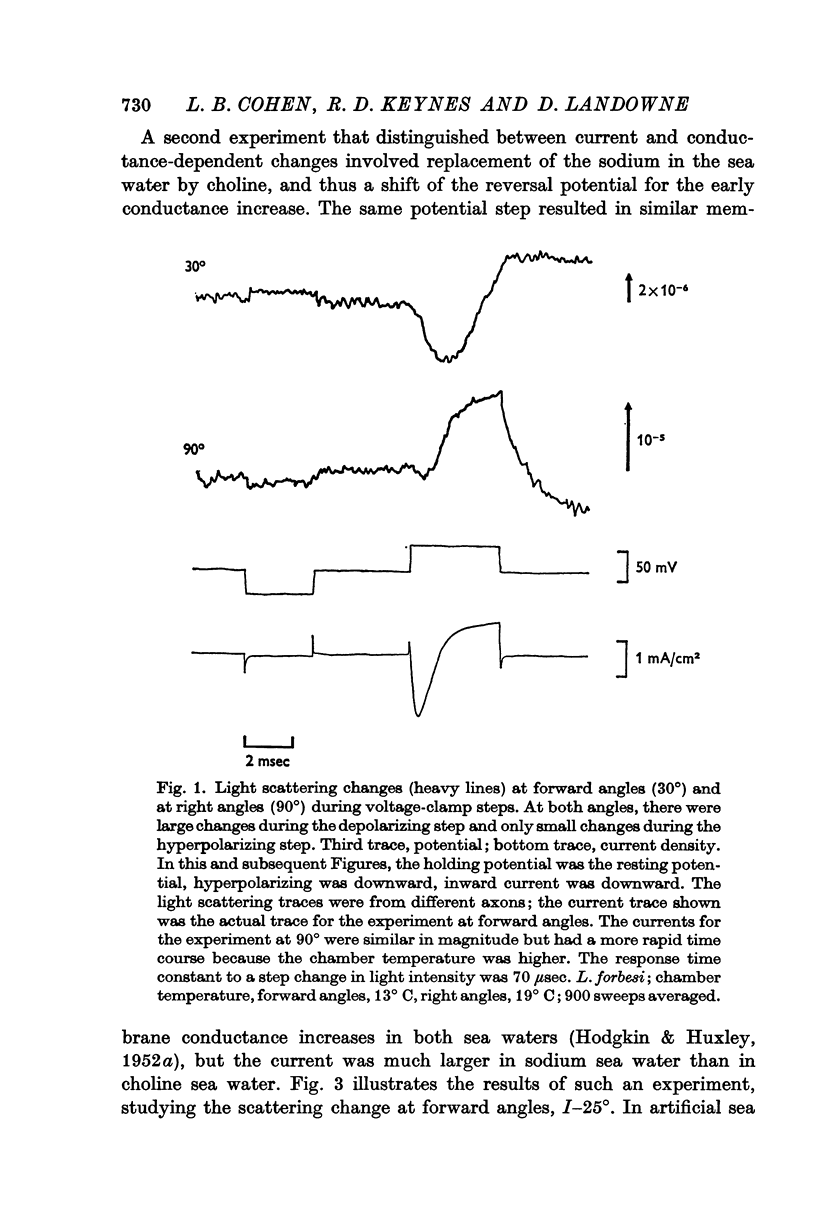
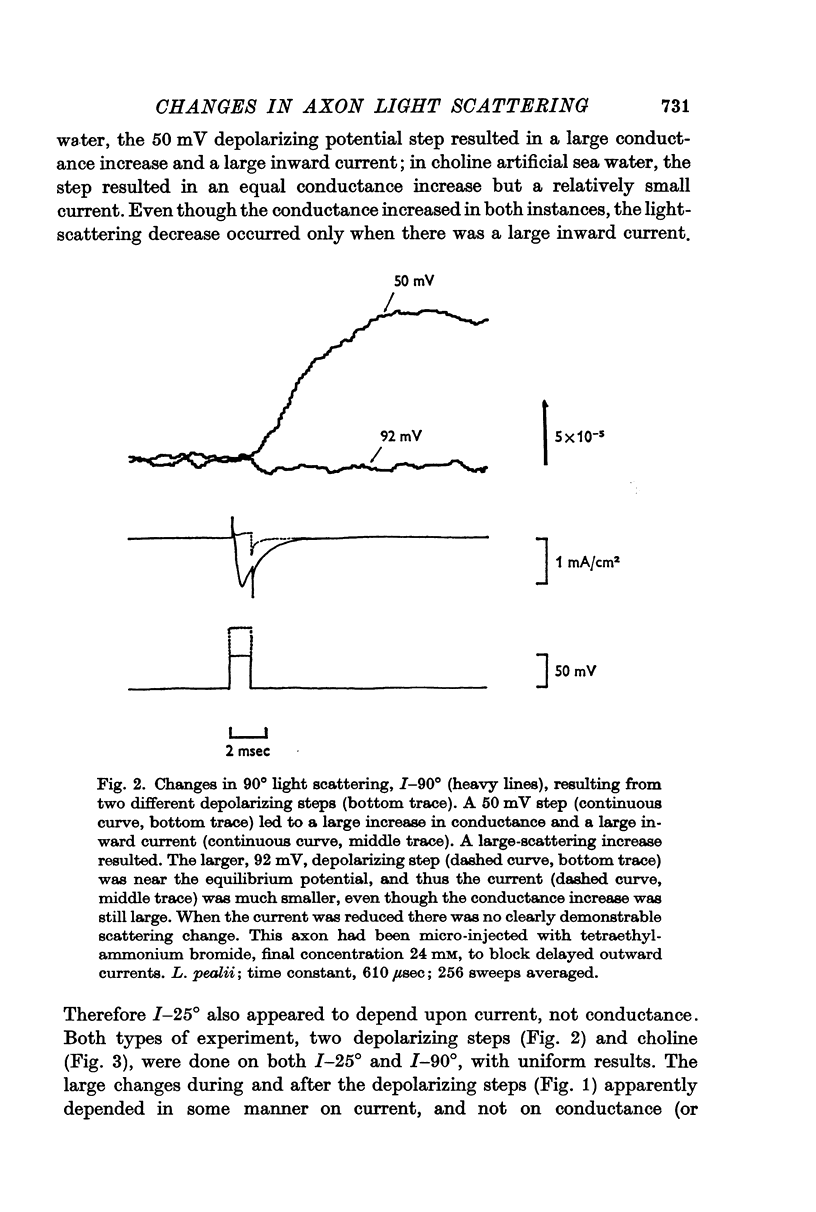
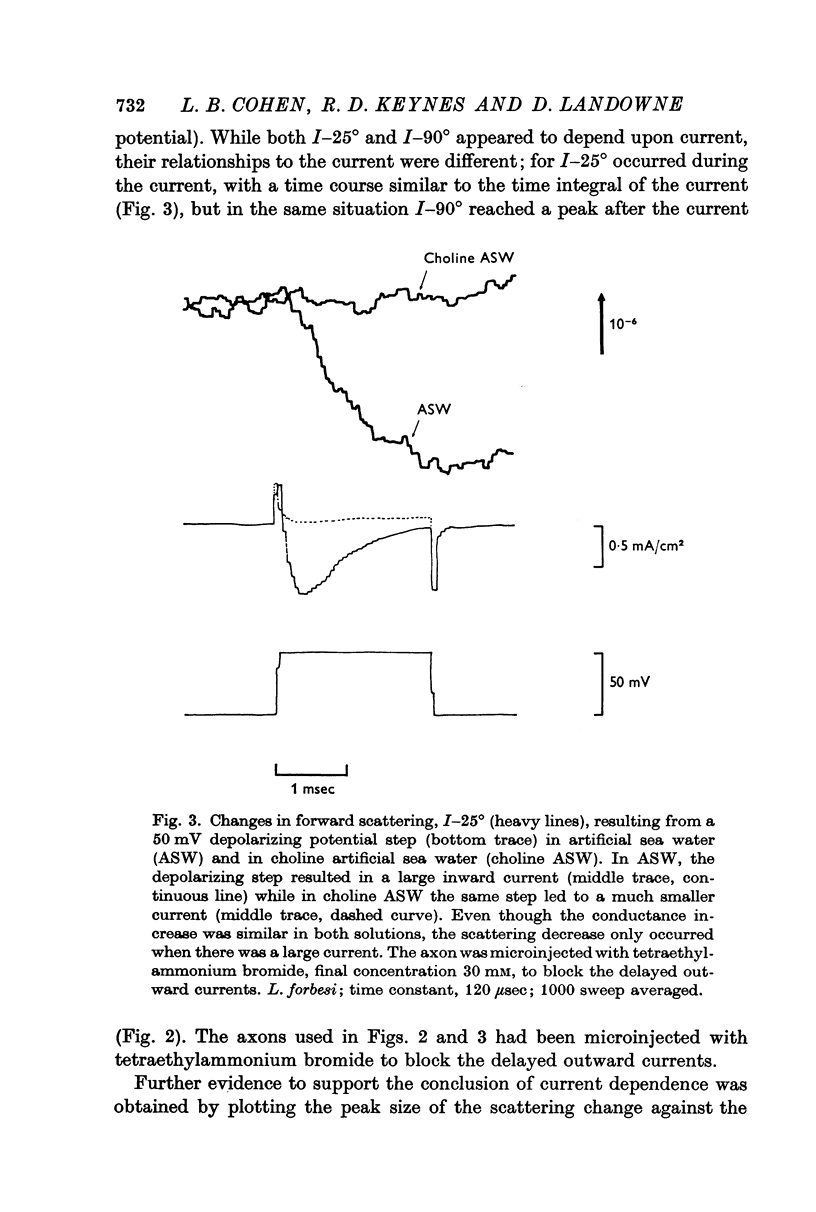
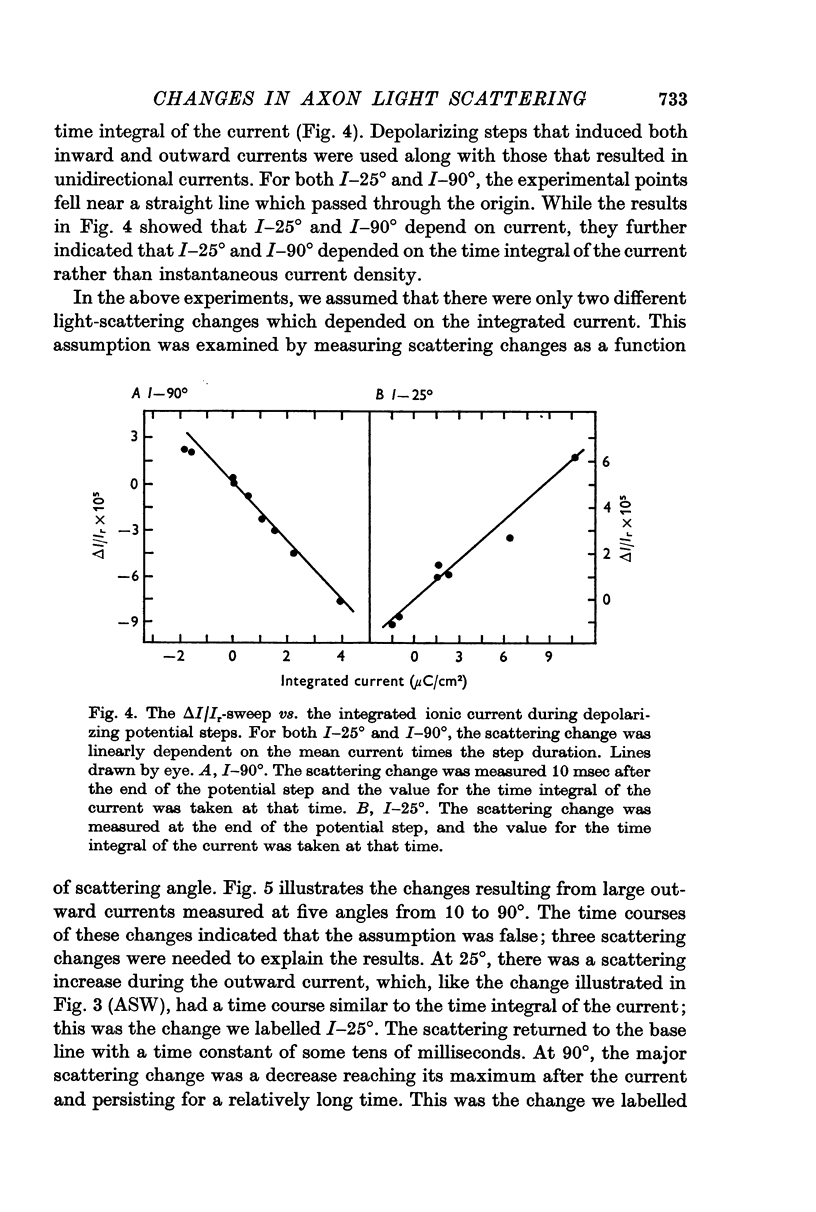
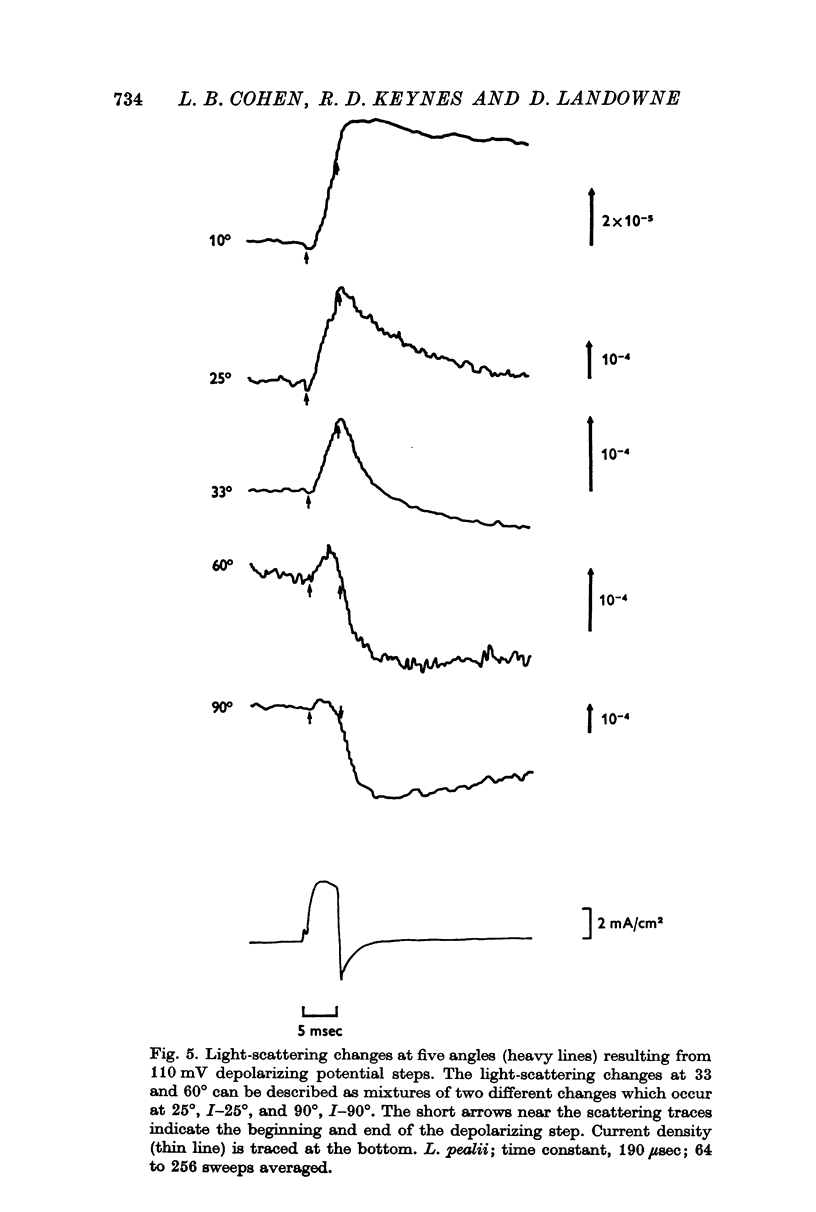
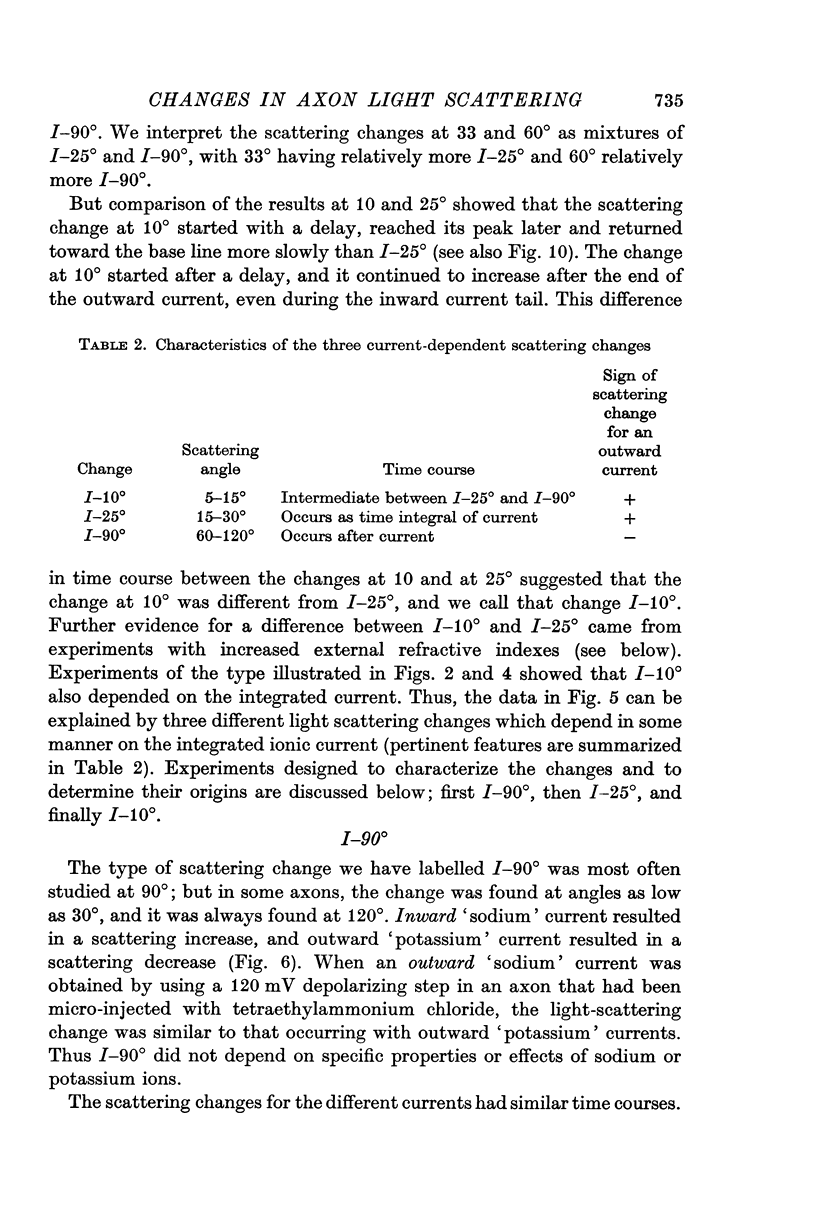
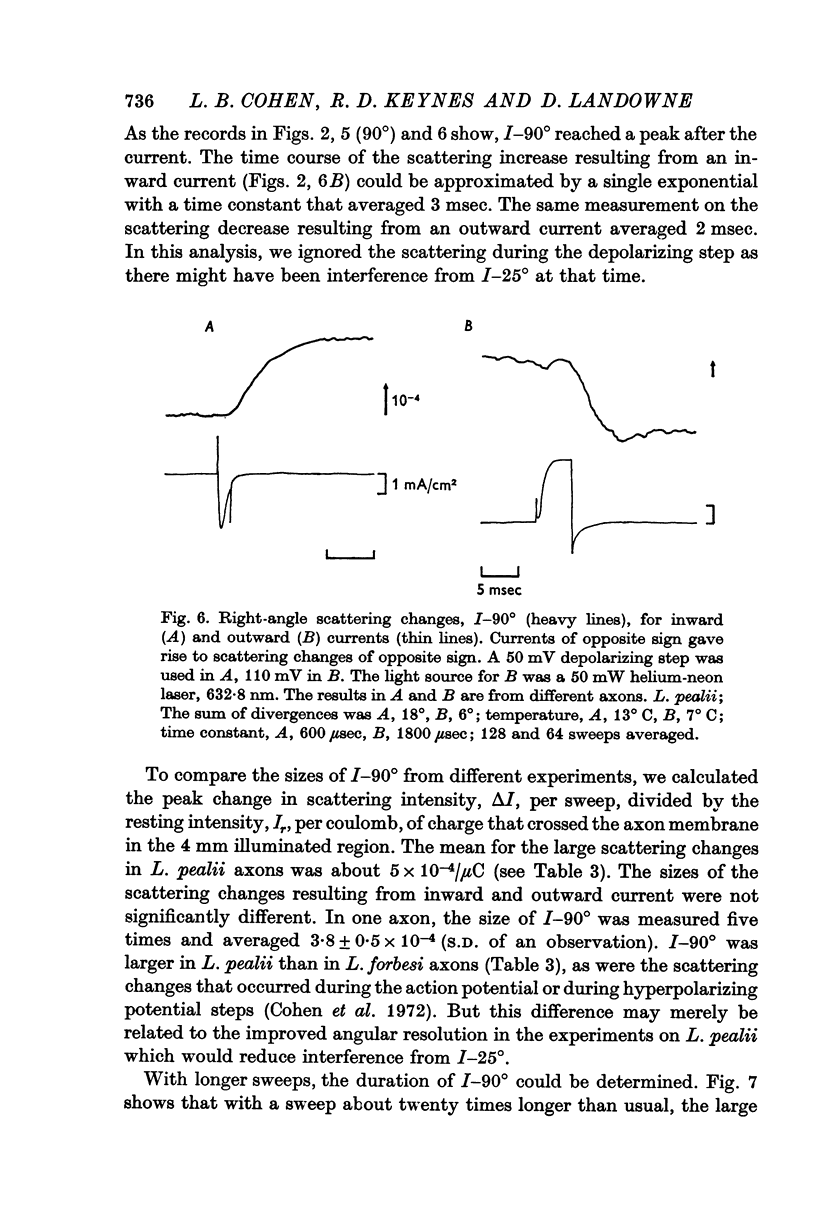
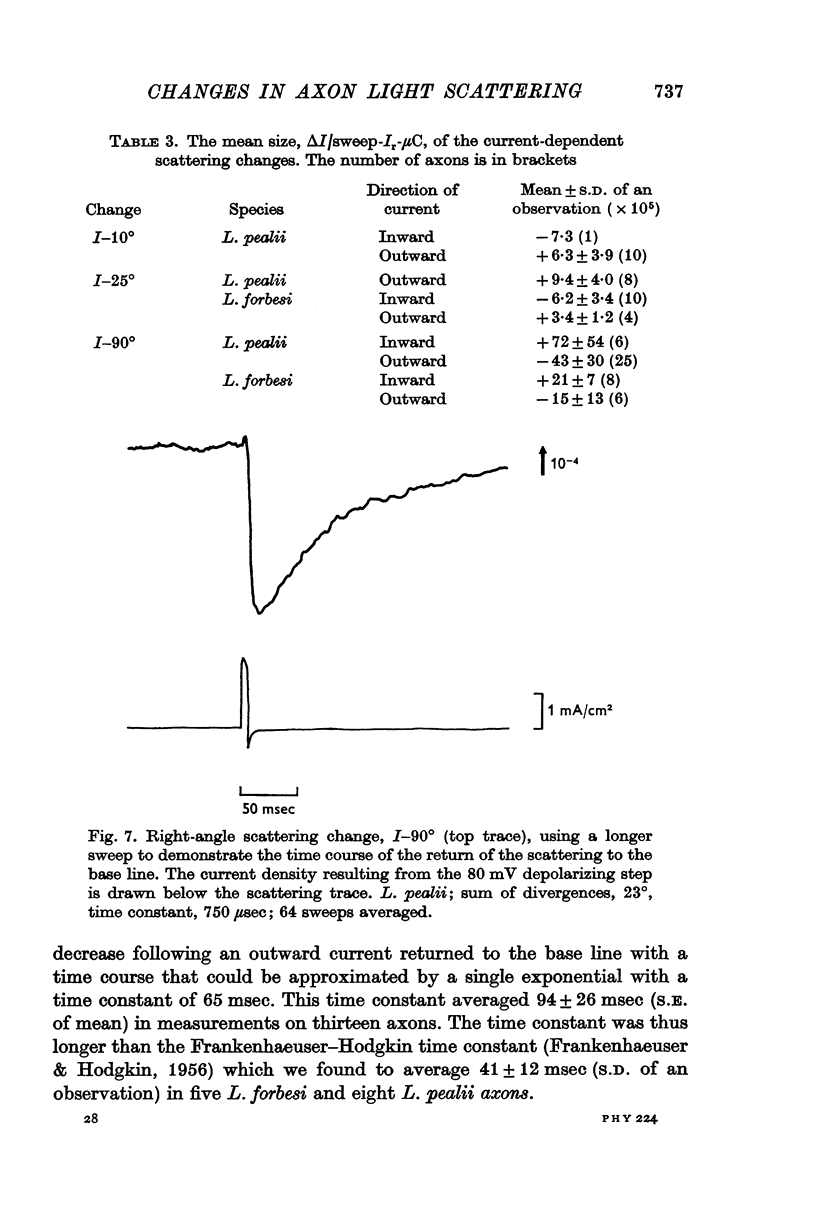
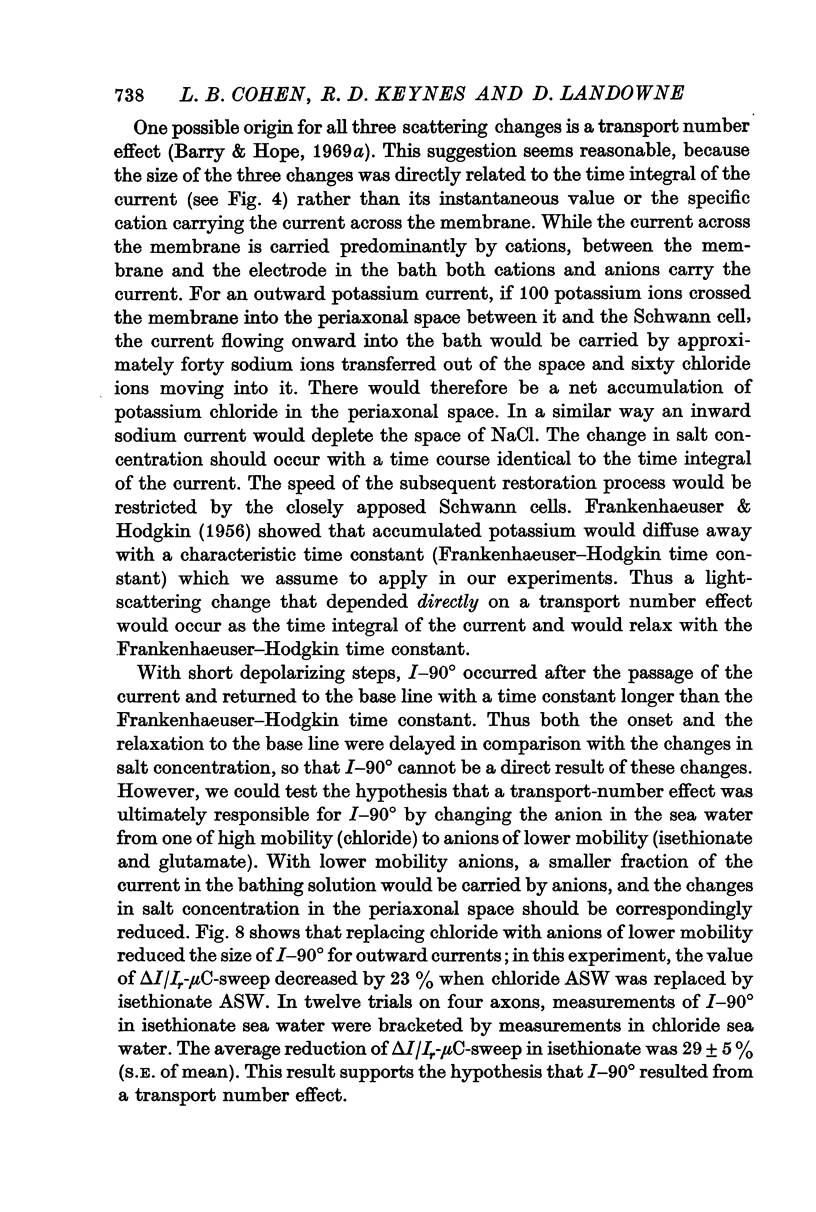
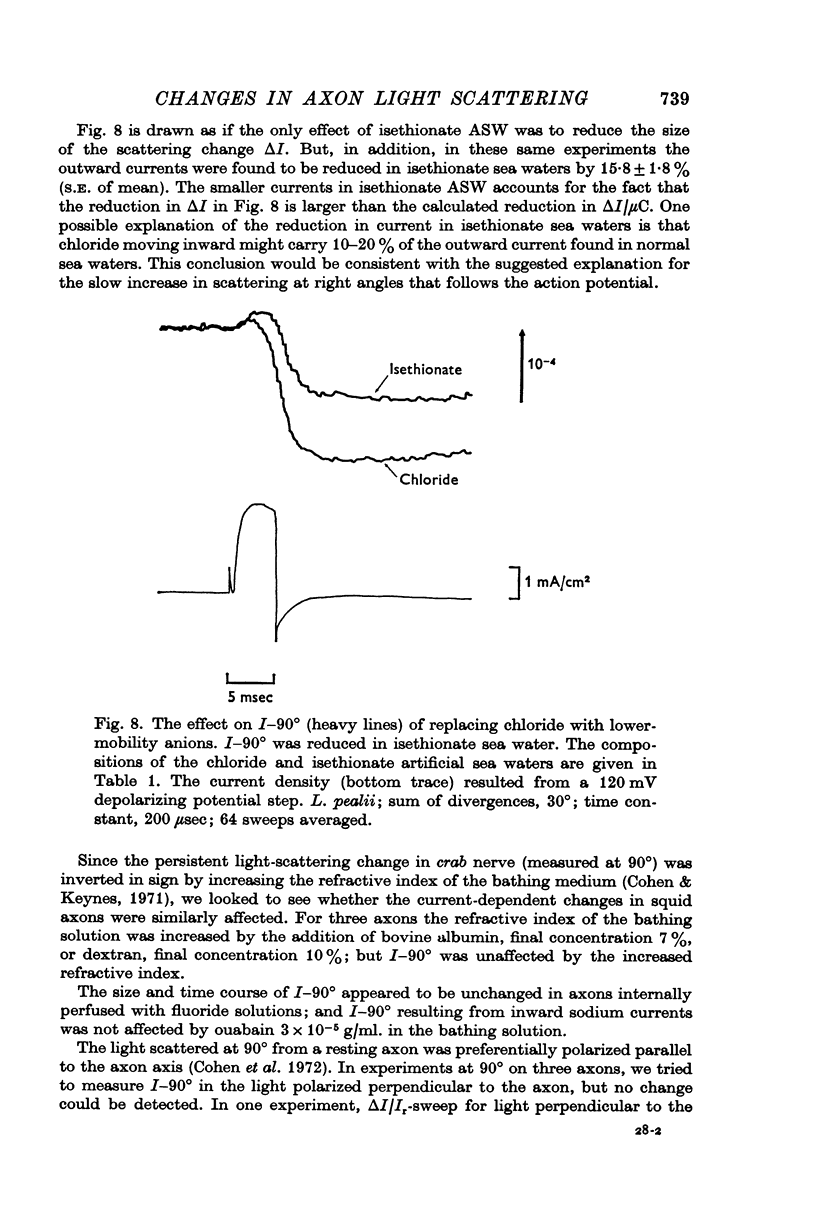
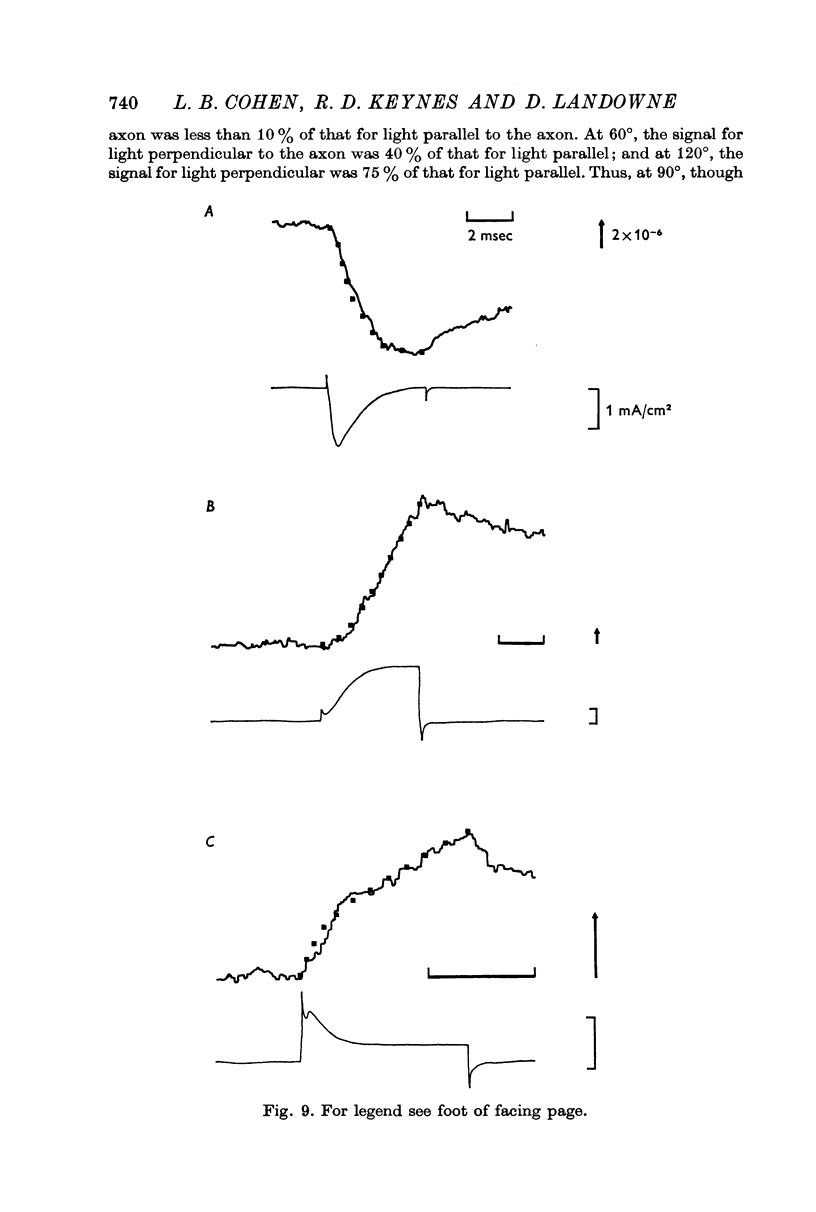
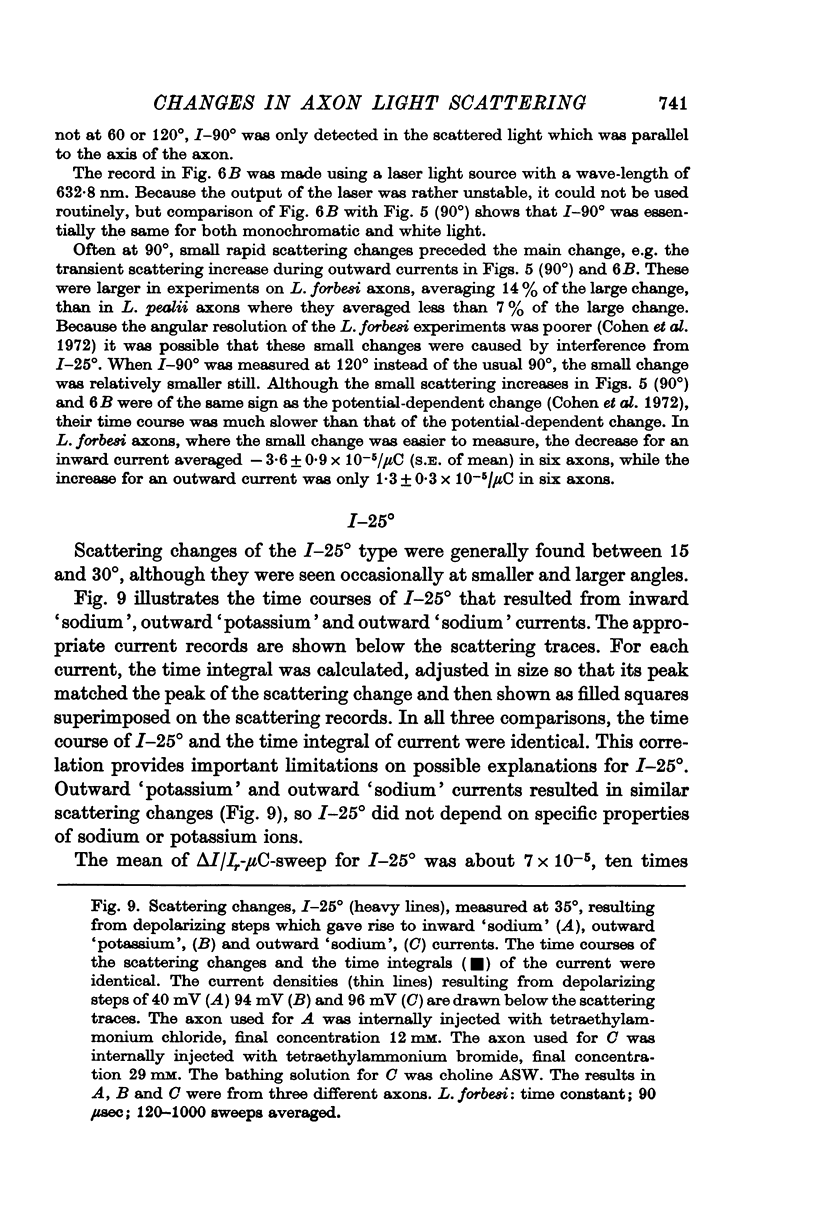
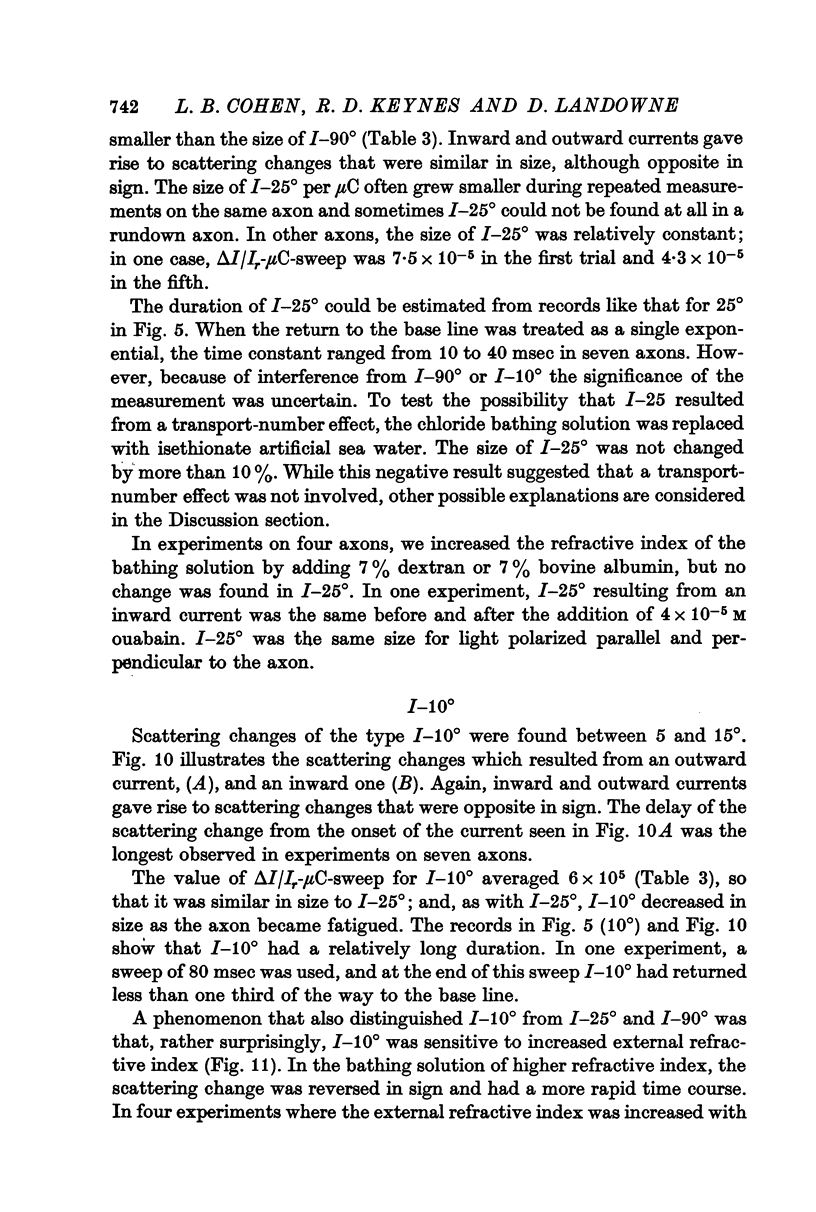
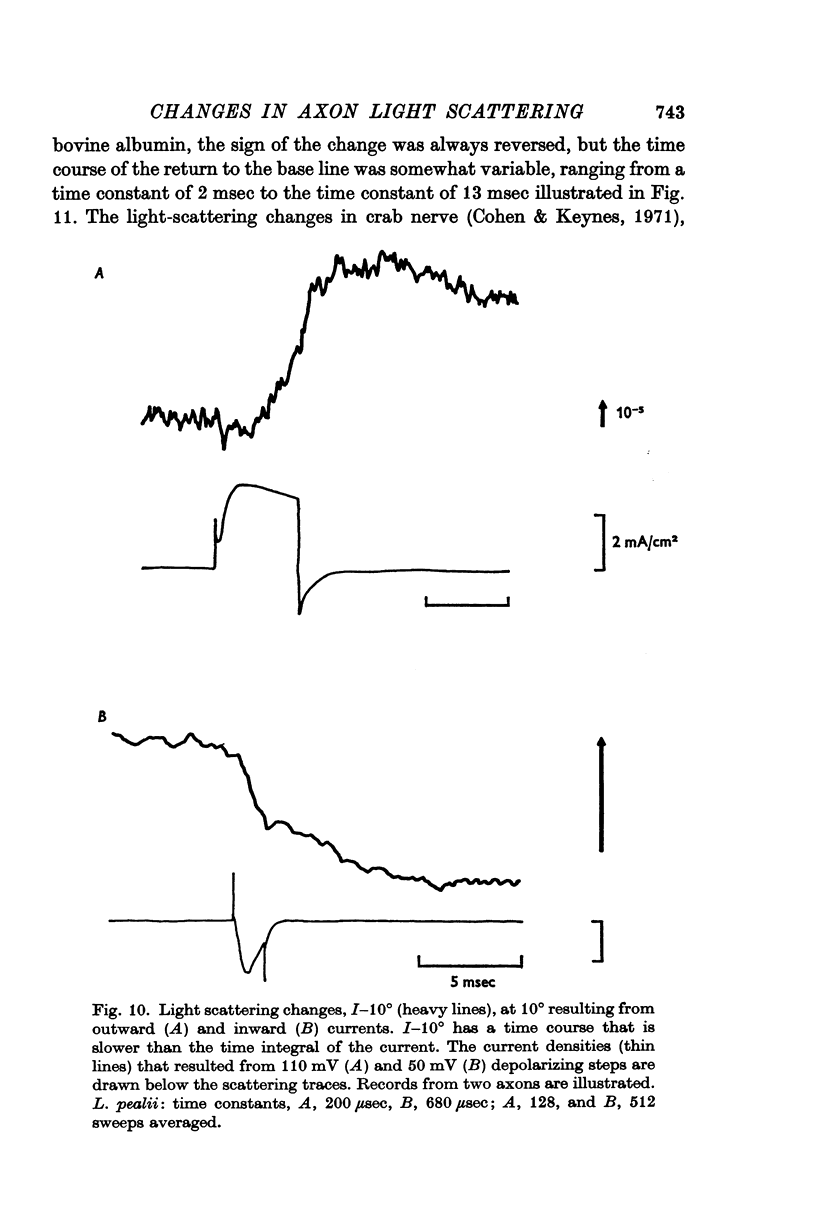
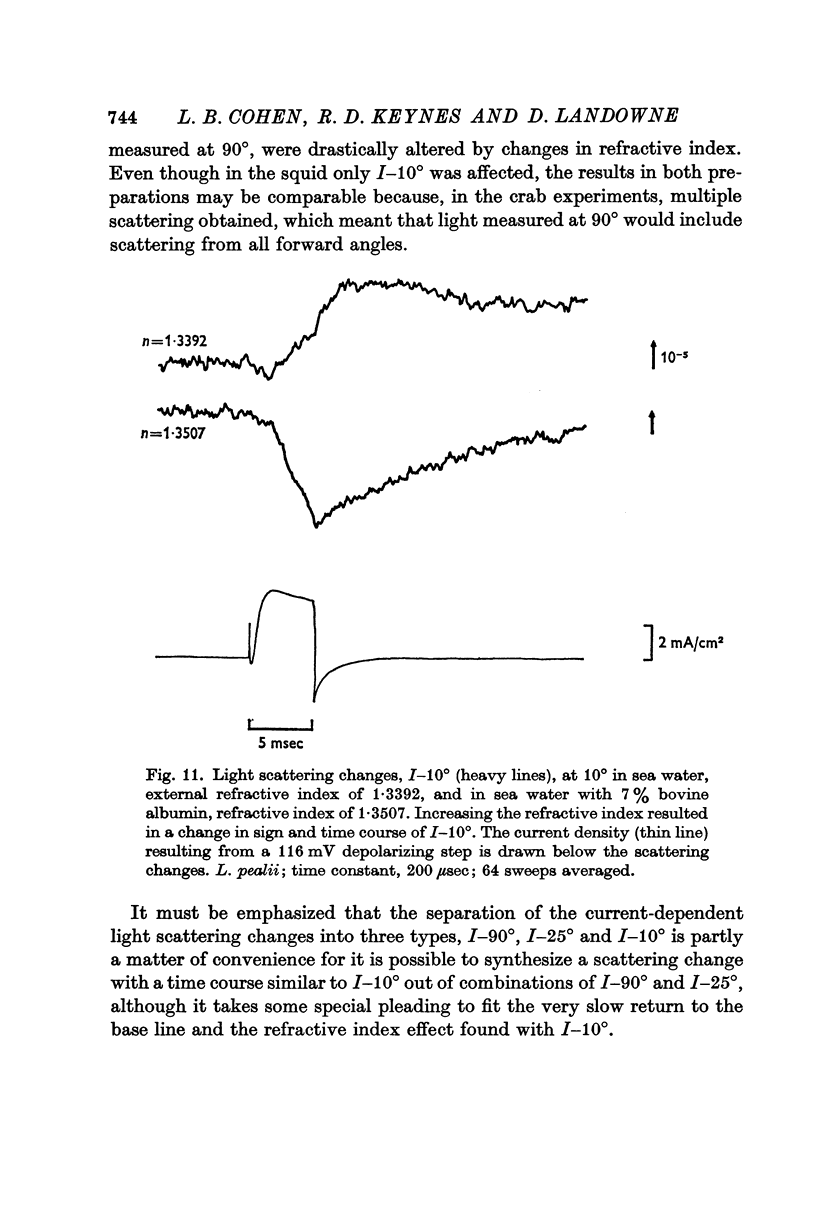
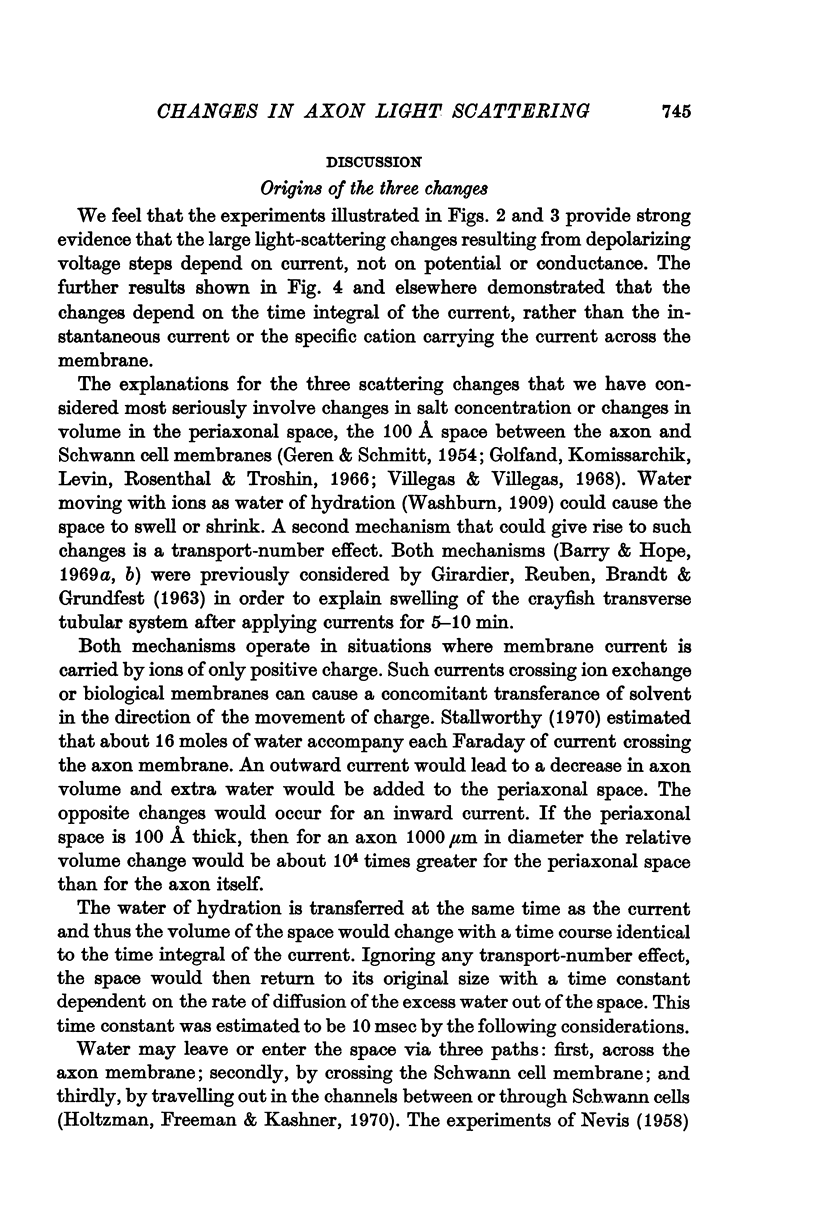
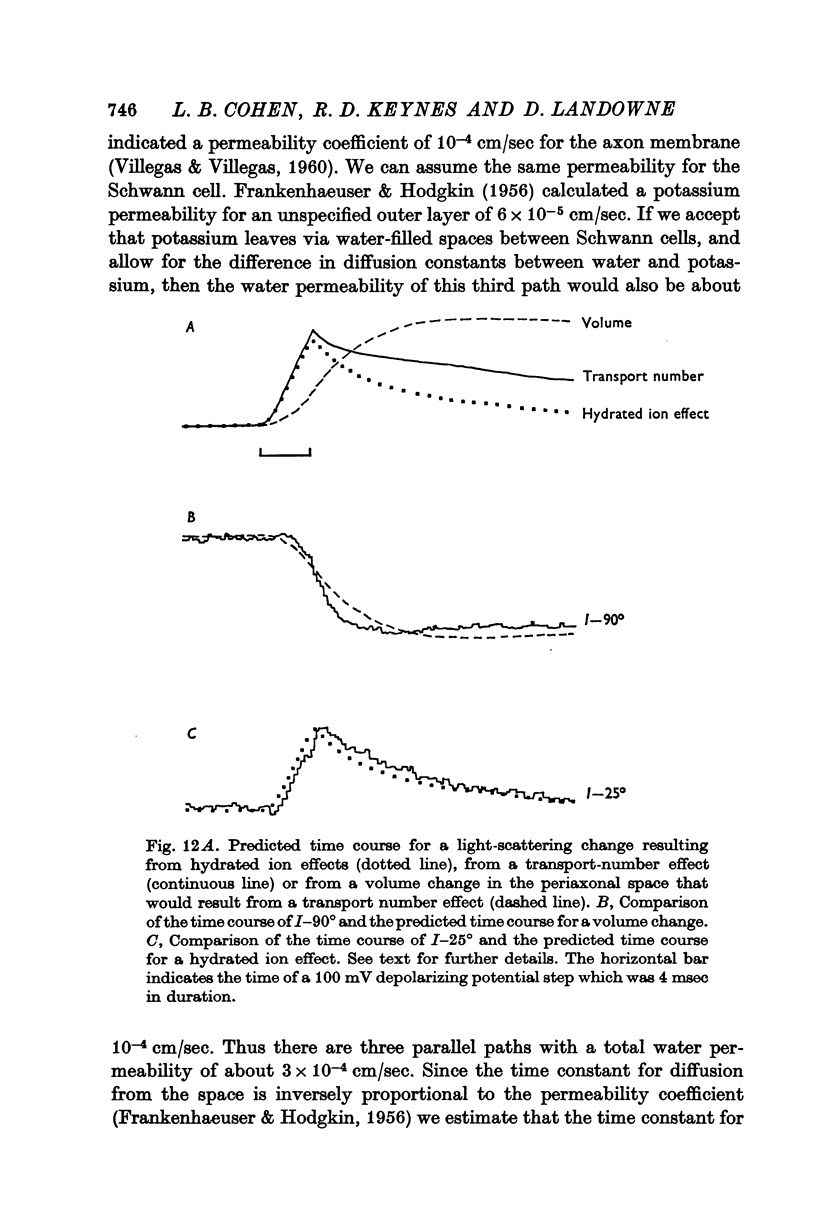
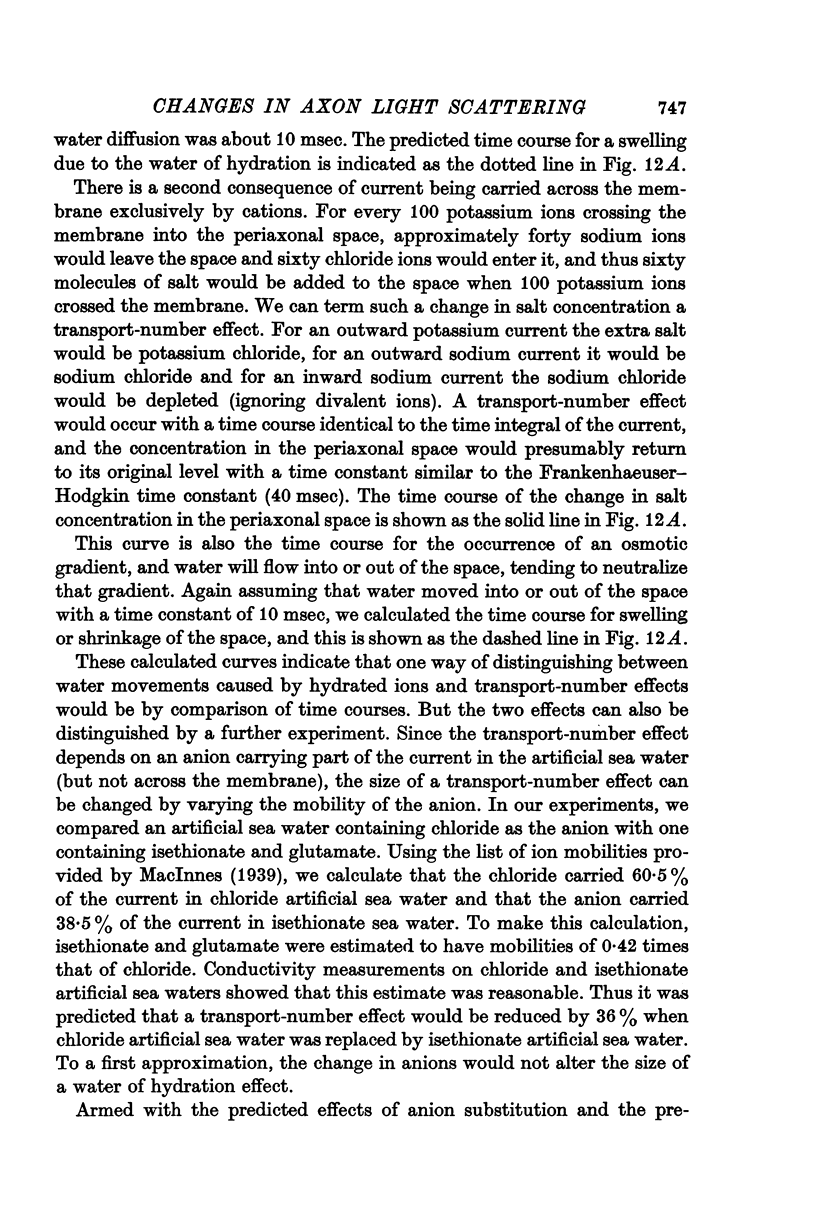
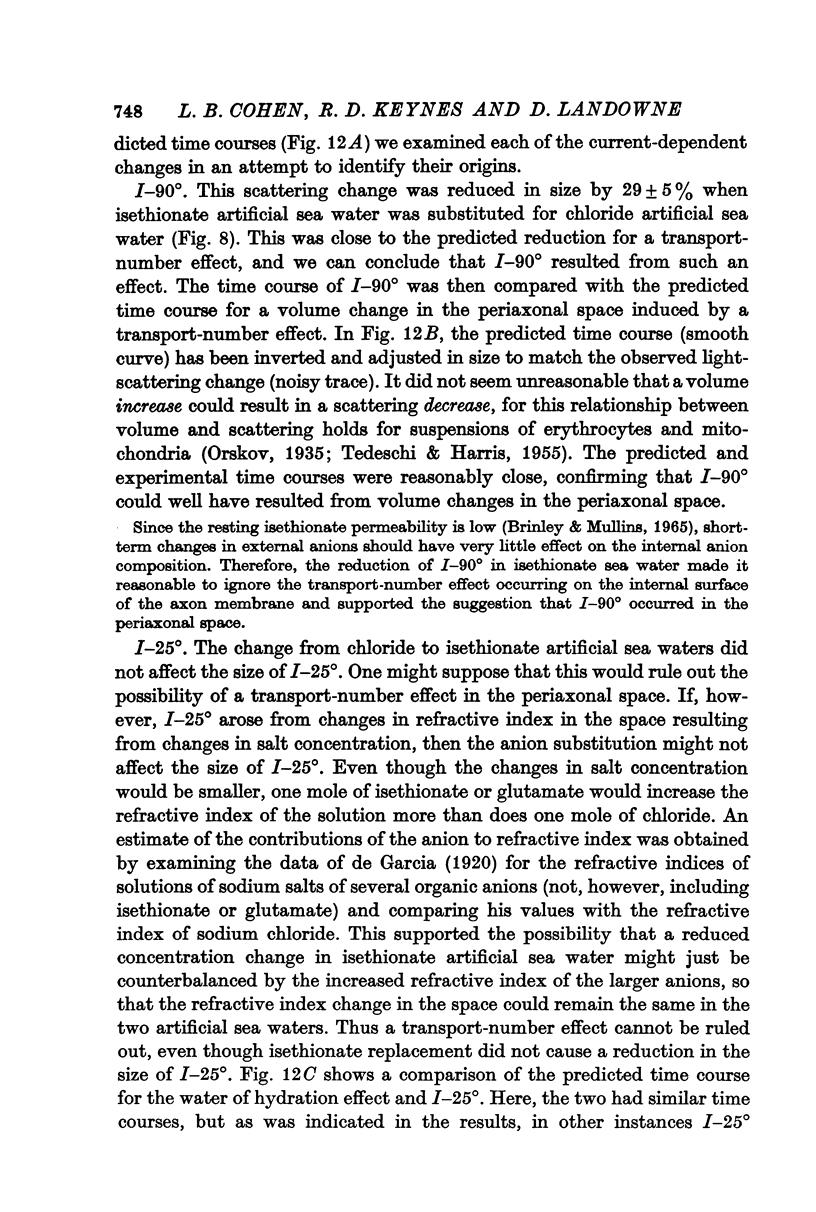
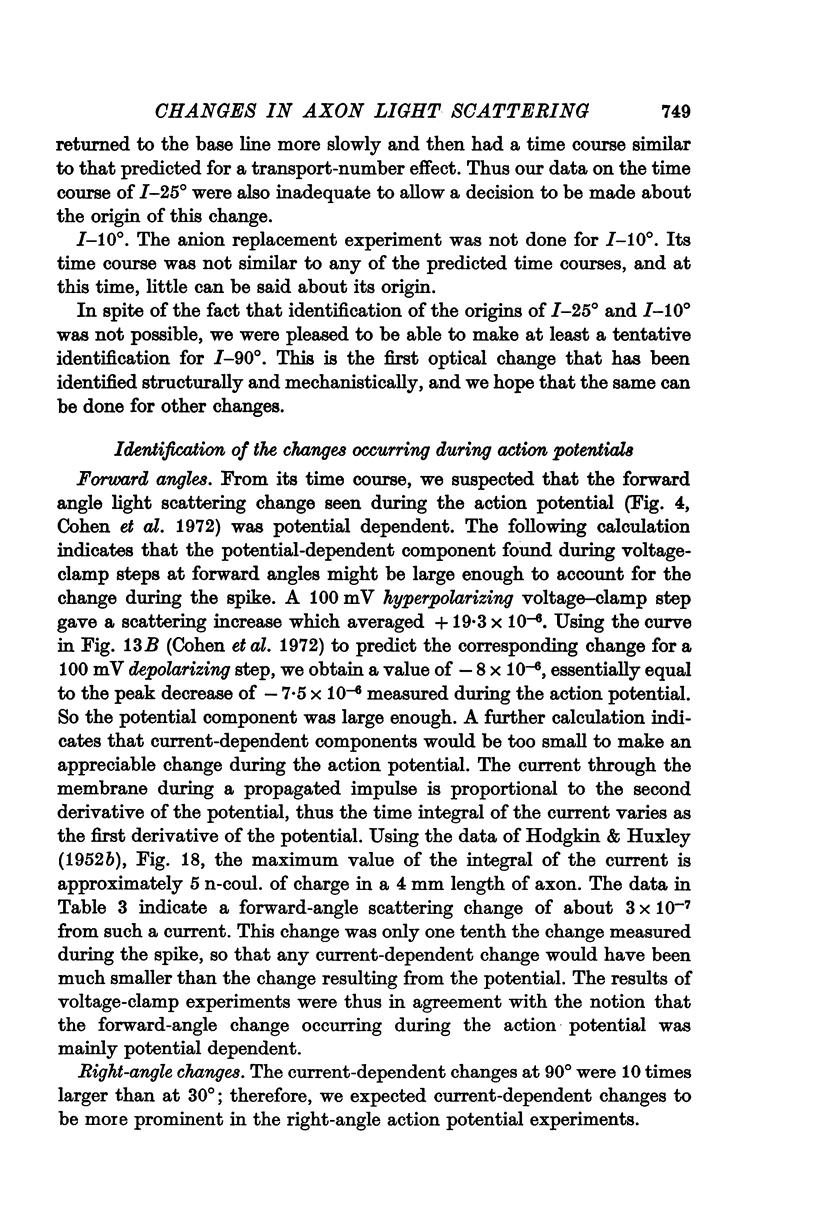
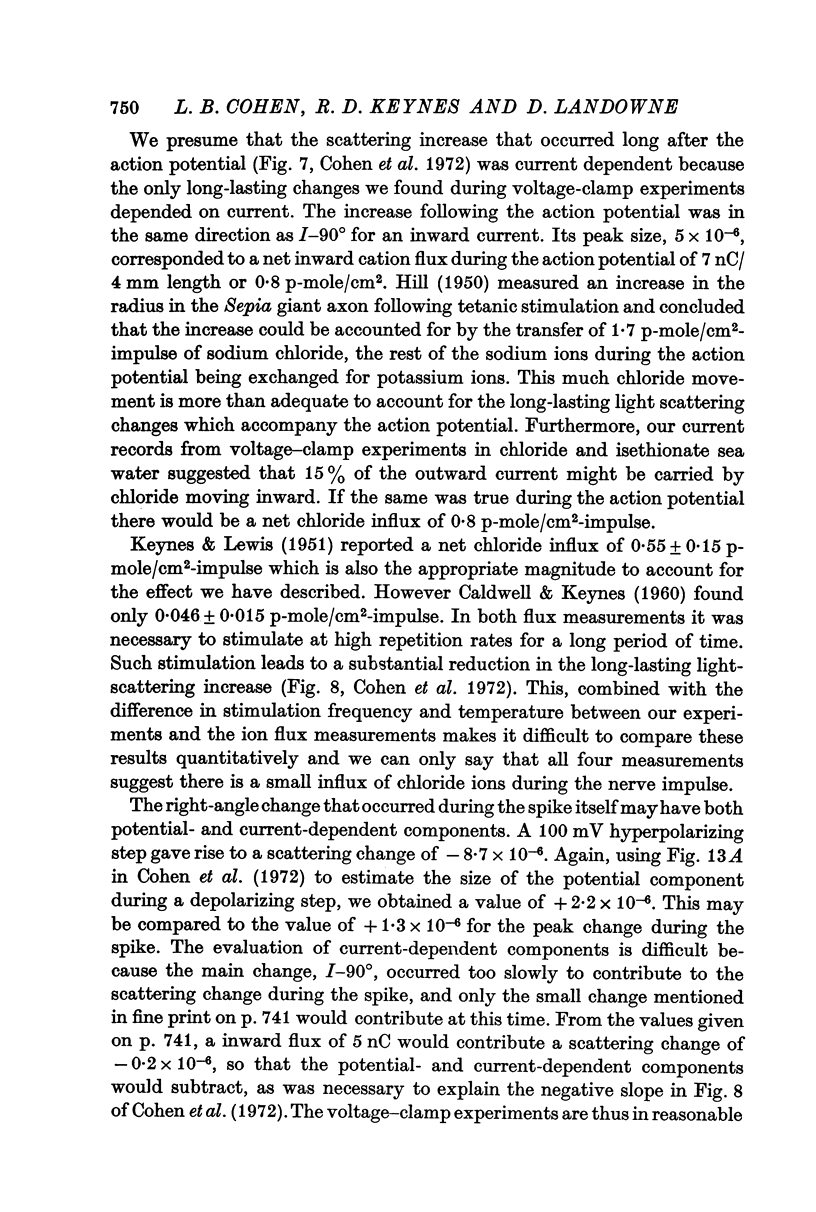
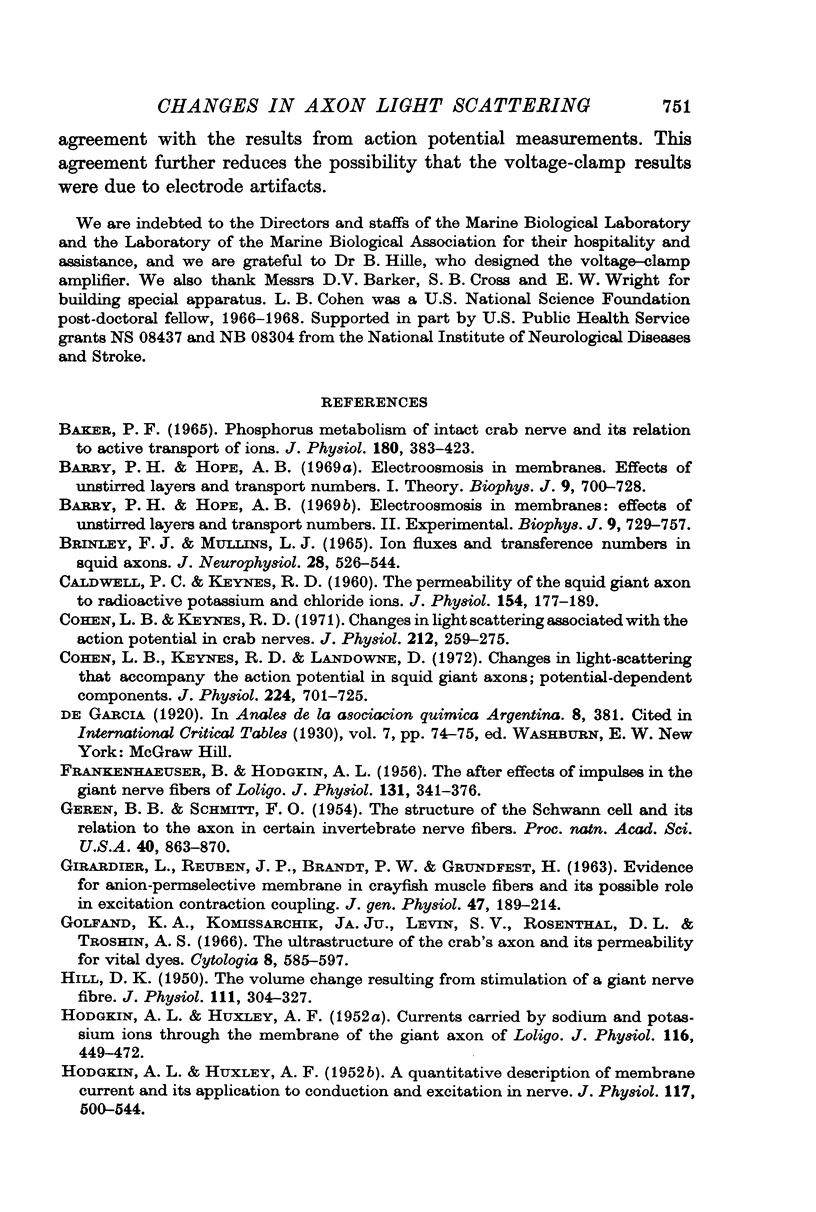
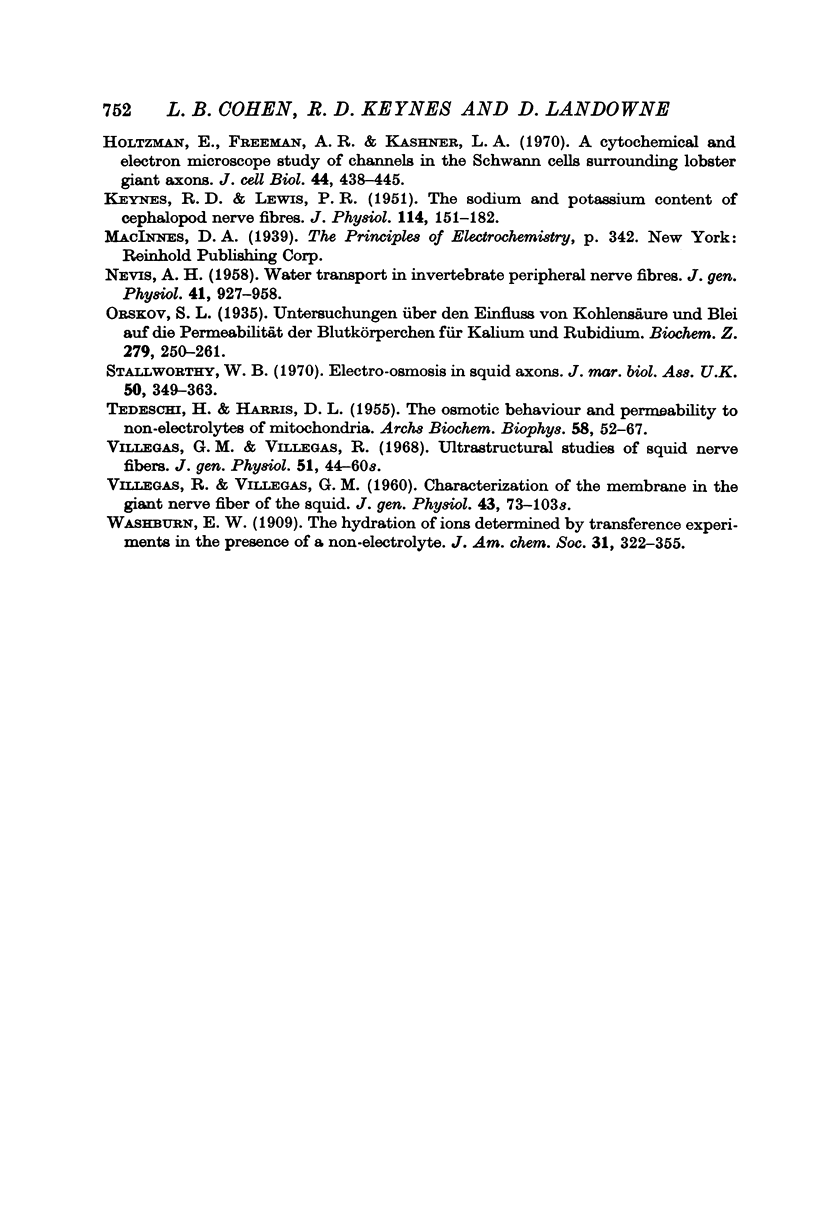
Selected References
These references are in PubMed. This may not be the complete list of references from this article.
- BRINLEY F. J., Jr, MULLINS L. J. ION FLUXES AND TRANSFERENCE NUMBER IN SQUID AXONS. J Neurophysiol. 1965 May;28:526–544. doi: 10.1152/jn.1965.28.3.526. [DOI] [PubMed] [Google Scholar]
- Baker P. F. Phosphorus metabolism of intact crab nerve and its relation to the active transport of ions. J Physiol. 1965 Sep;180(2):383–423. doi: 10.1113/jphysiol.1965.sp007709. [DOI] [PMC free article] [PubMed] [Google Scholar]
- Barry P. H., Hope A. B. Electroosmosis in membranes: effects of unstirred layers and transport numbers. I. Theory. Biophys J. 1969 May;9(5):700–728. doi: 10.1016/S0006-3495(69)86413-1. [DOI] [PMC free article] [PubMed] [Google Scholar]
- Barry P. H., Hope A. B. Electroosmosis in membranes: effects of unstirred layers and transport numbers. II. Experimental. Biophys J. 1969 May;9(5):729–757. doi: 10.1016/S0006-3495(69)86414-3. [DOI] [PMC free article] [PubMed] [Google Scholar]
- CALDWELL P. C., KEYNES R. D. The permeability of the squid giant axon to radioactive potassium and chloride ions. J Physiol. 1960 Nov;154:177–189. doi: 10.1113/jphysiol.1960.sp006572. [DOI] [PMC free article] [PubMed] [Google Scholar]
- Cohen L. B., Keynes R. D. Changes in light scattering associated with the action potential in crab nerves. J Physiol. 1971 Jan;212(1):259–275. doi: 10.1113/jphysiol.1971.sp009321. [DOI] [PMC free article] [PubMed] [Google Scholar]
- Cohen L. B., Keynes R. D., Landowne D. Changes in light scattering that accompany the action potential in squid giant axons: potential-dependent components. J Physiol. 1972 Aug;224(3):701–725. doi: 10.1113/jphysiol.1972.sp009919. [DOI] [PMC free article] [PubMed] [Google Scholar]
- FRANKENHAEUSER B., HODGKIN A. L. The after-effects of impulses in the giant nerve fibres of Loligo. J Physiol. 1956 Feb 28;131(2):341–376. doi: 10.1113/jphysiol.1956.sp005467. [DOI] [PMC free article] [PubMed] [Google Scholar]
- GIRARDIER L., REUBEN J. P., BRANDT P. W., GRUNDFEST H. EVIDENCE FOR ANION-PERMSELECTIVE MEMBRANE IN CRAYFISH MUSCLE FIBERS AND ITS POSSIBLE ROLE IN EXCITATION-CONTRACTION COUPLING. J Gen Physiol. 1963 Sep;47:189–214. doi: 10.1085/jgp.47.1.189. [DOI] [PMC free article] [PubMed] [Google Scholar]
- Geren B. B., Schmitt F. O. THE STRUCTURE OF THE SCHWANN CELL AND ITS RELATION TO THE AXON IN CERTAIN INVERTEBRATE NERVE FIBERS. Proc Natl Acad Sci U S A. 1954 Sep;40(9):863–870. doi: 10.1073/pnas.40.9.863. [DOI] [PMC free article] [PubMed] [Google Scholar]
- Gol'fand K. A., Komissarchik Ia Iu, Levin S. V., Rozental' D. L., Troshin A. S. Ul'trastruktura aksona kraba i ego pronitsaemost' dlia vital'nykh krasitelei. Tsitologiia. 1966 Sep-Oct;8(5):585–597. [PubMed] [Google Scholar]
- HILL D. K. The volume change resulting from stimulation of a giant nerve fibre. J Physiol. 1950 Oct 16;111(3-4):304–327. doi: 10.1113/jphysiol.1950.sp004481. [DOI] [PMC free article] [PubMed] [Google Scholar]
- HODGKIN A. L., HUXLEY A. F. A quantitative description of membrane current and its application to conduction and excitation in nerve. J Physiol. 1952 Aug;117(4):500–544. doi: 10.1113/jphysiol.1952.sp004764. [DOI] [PMC free article] [PubMed] [Google Scholar]
- HODGKIN A. L., HUXLEY A. F. Currents carried by sodium and potassium ions through the membrane of the giant axon of Loligo. J Physiol. 1952 Apr;116(4):449–472. doi: 10.1113/jphysiol.1952.sp004717. [DOI] [PMC free article] [PubMed] [Google Scholar]
- Holtzman E., Freeman A. R., Kashner L. A. A cytochemical and electron microscope study of channels in the Schwann cells surrounding lobster giant axons. J Cell Biol. 1970 Feb;44(2):438–445. doi: 10.1083/jcb.44.2.438. [DOI] [PMC free article] [PubMed] [Google Scholar]
- KEYNES R. D., LEWIS P. R. The sodium and potassium content of cephalopod nerve fibers. J Physiol. 1951 Jun;114(1-2):151–182. doi: 10.1113/jphysiol.1951.sp004609. [DOI] [PMC free article] [PubMed] [Google Scholar]
- NEVIS A. H. Water transport in invertebrate peripheral nerve fibers. J Gen Physiol. 1958 May 20;41(5):927–958. doi: 10.1085/jgp.41.5.927. [DOI] [PMC free article] [PubMed] [Google Scholar]
- TEDESCHI H., HARRIS D. L. The osmotic behavior and permeability to non-electrolytes of mitochondria. Arch Biochem Biophys. 1955 Sep;58(1):52–67. doi: 10.1016/0003-9861(55)90092-8. [DOI] [PubMed] [Google Scholar]
- VILLEGAS R., VILLEGAS G. M. Characterization of the membranes in the giant nerve fiber of the squid. J Gen Physiol. 1960 May;43:73–103. doi: 10.1085/jgp.43.5.73. [DOI] [PMC free article] [PubMed] [Google Scholar]