Abstract
Computations show that cathodal rheobase increases with temperature from 0 degrees C to 30 degrees C. Anodal rheobase (stimulation at the end of an indefinitely long anodal pulse) also increases with temperature, but goes to infinity at a critical temperature 17.13 degrees C, above which such excitation is impossible. For a stimulus consisting of any step change of current from I0 to I1, a threshold curve of I1 is plotted against I0. As the temperature increases, this curve rises. Its intersection with the horizontal axis, which determines the anodal rheobase, goes to infinity at the critical temperature. This phenomenon is caused by the saturation of the variables m, h, n for strongly hyperpolarized potentials, combined with the relative speeding up of the inhibitory process with increasing temperature. The threshold charge Q in an instantaneous anodal current pulse (of zero duration) goes to infinity at the same temperature, with a similar explanation in terms of threshold curves in the I1 vs. Q plane. The fact that the critical temperature for both cases is the same is generalized by the conjecture that for any anodal current waveform whatever, as its amplitude approaches infinity, the trajectory in the phase space following its cessation approaches the same limiting trajectory. This limiting trajectory changes from suprathreshold to subthreshold at the critical temperature.
Full text
PDF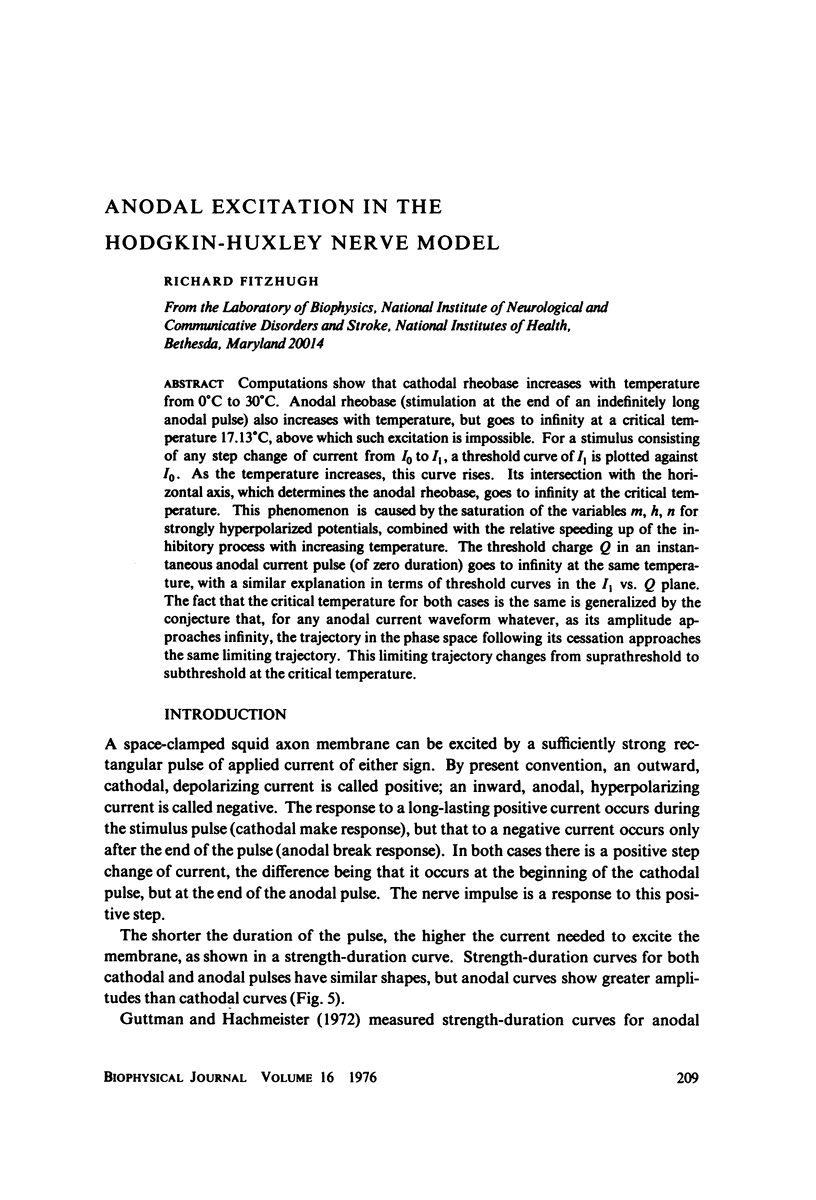
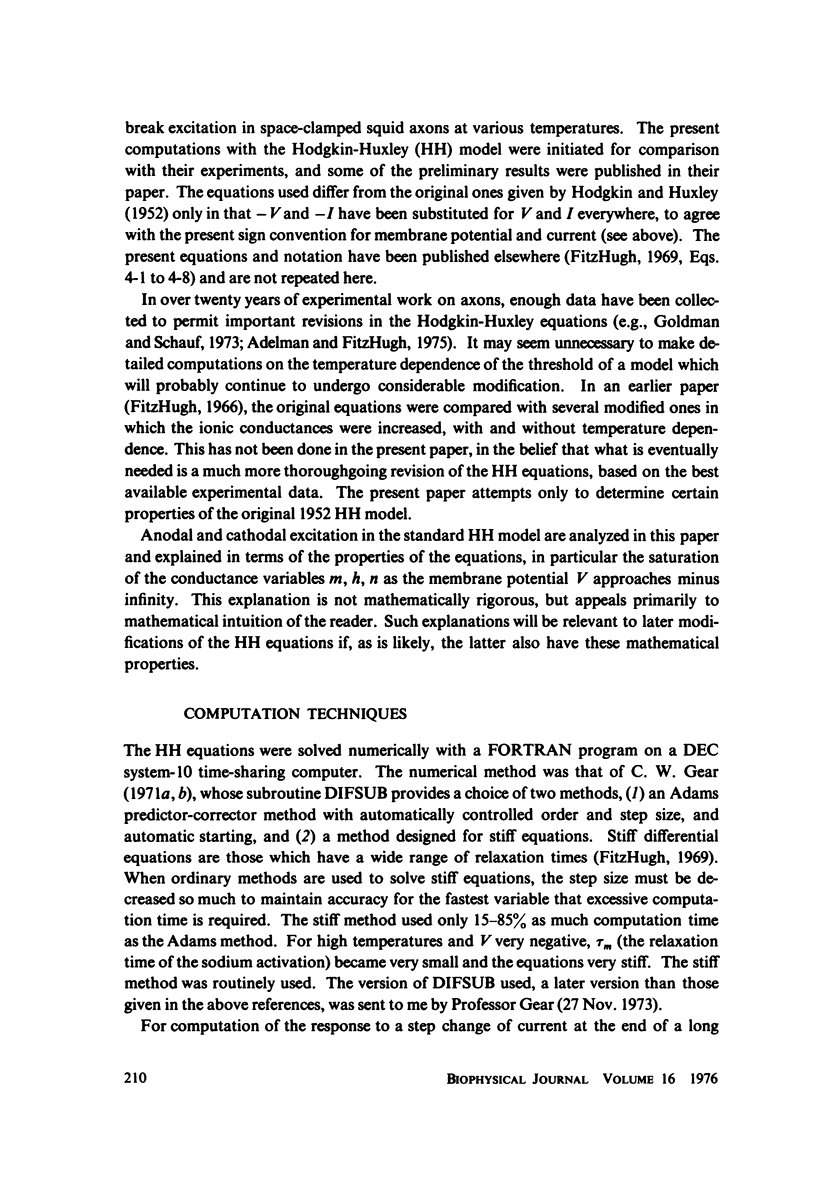
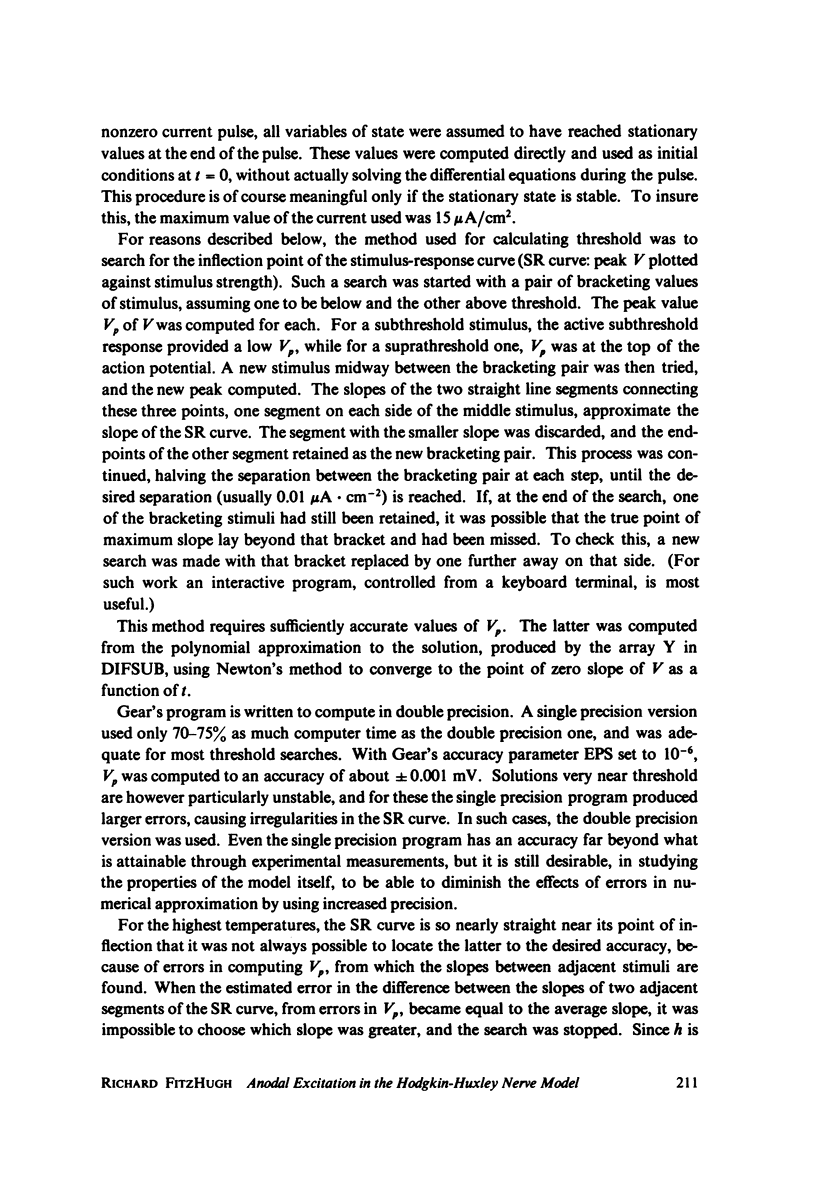
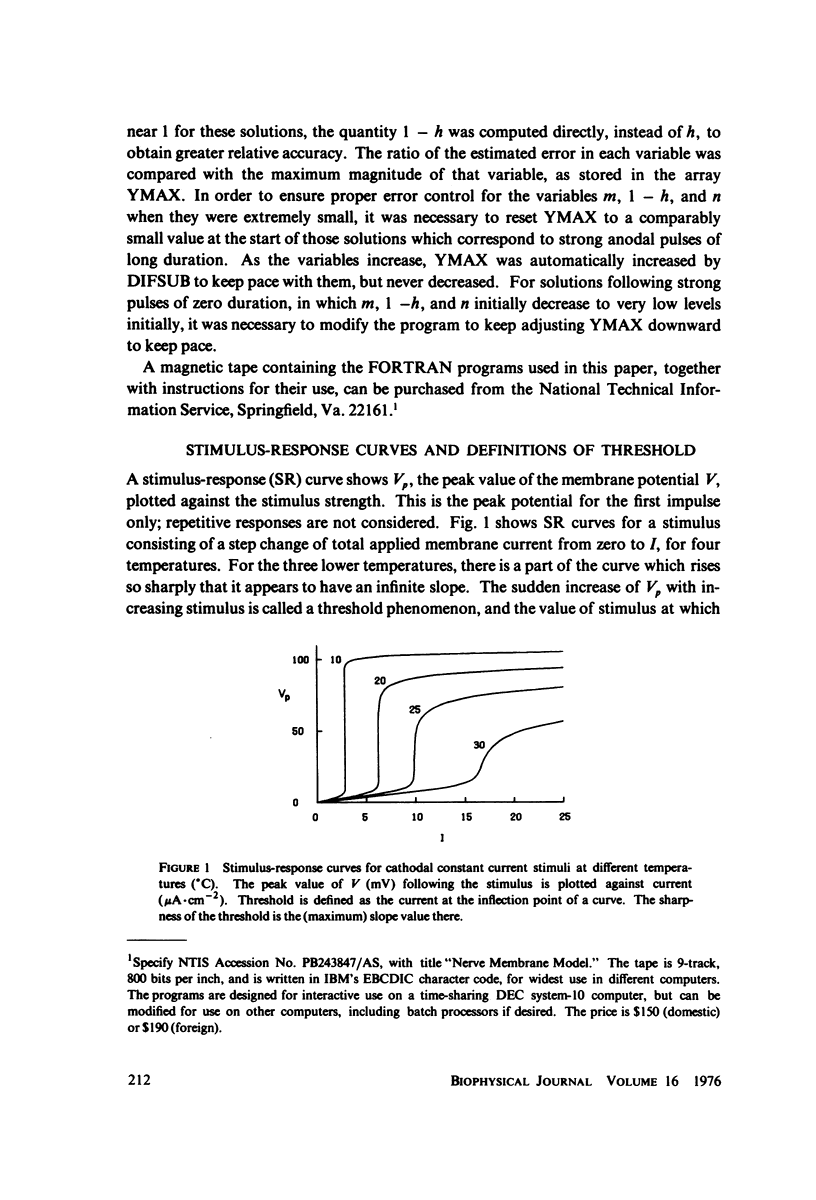
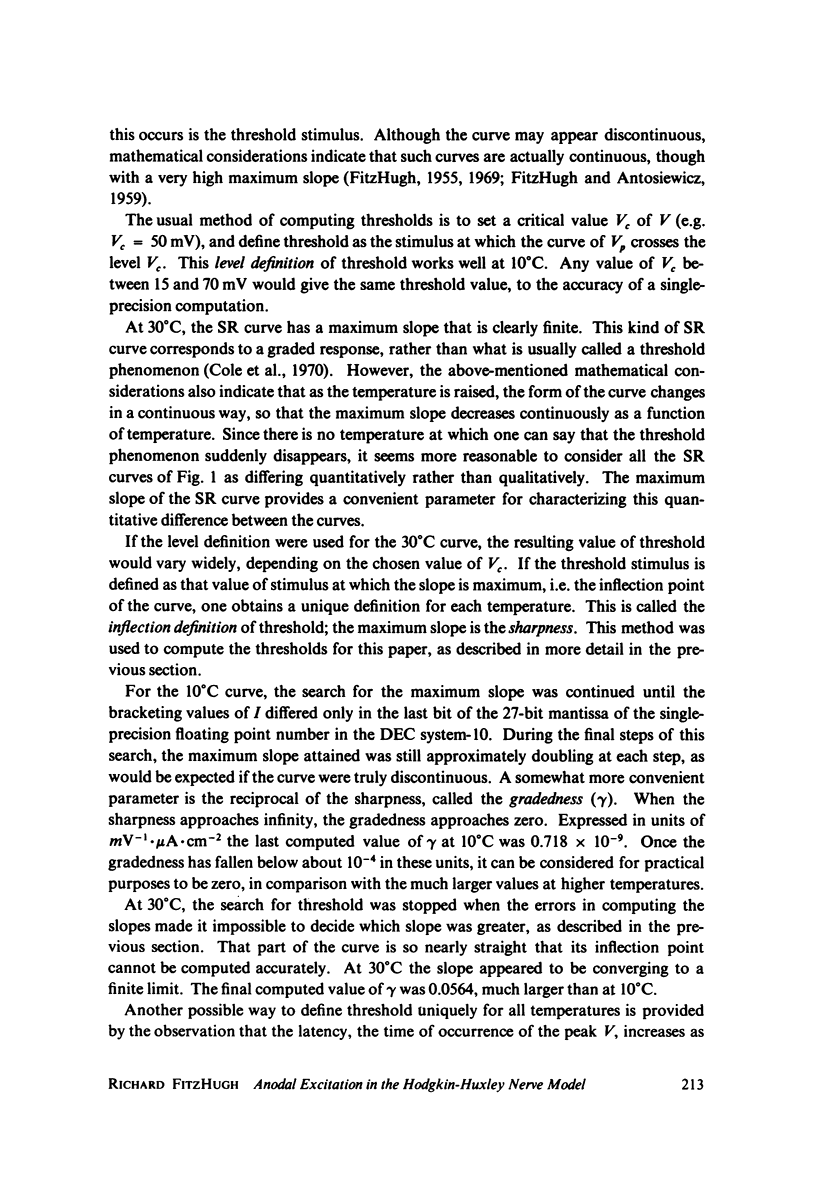
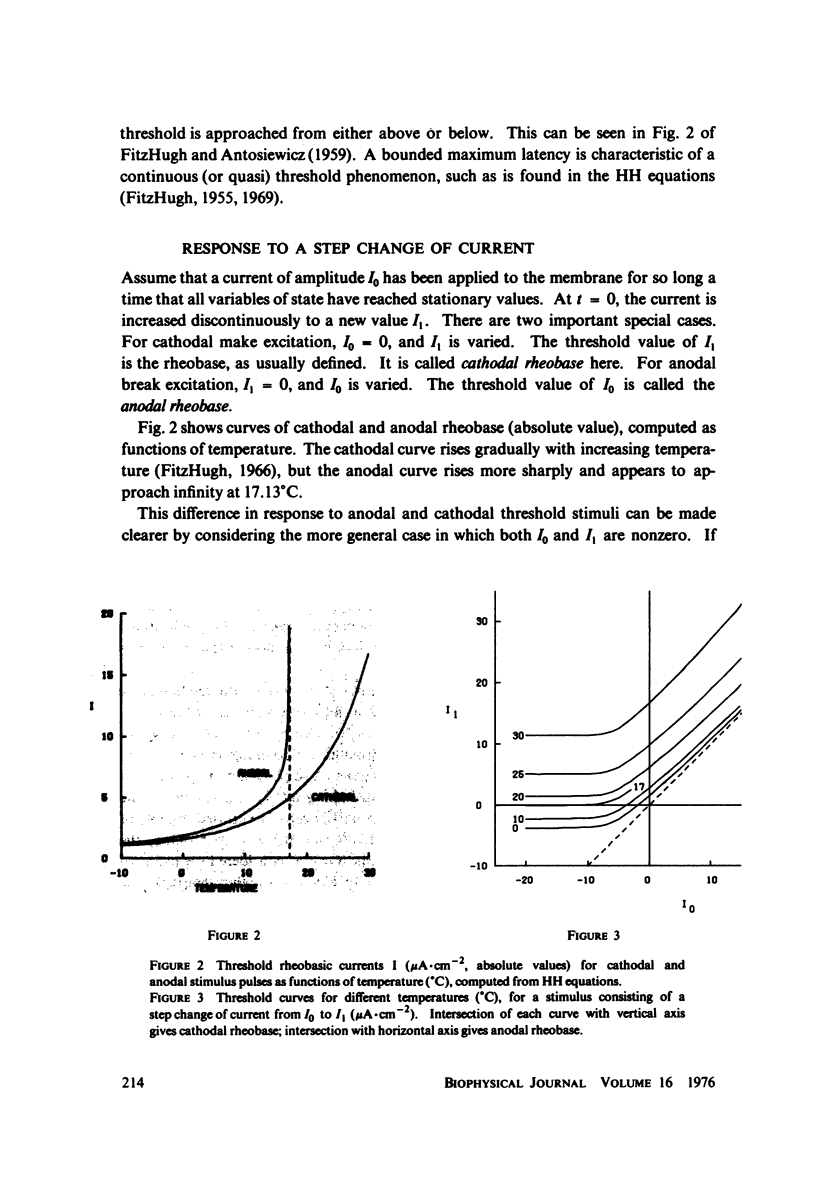
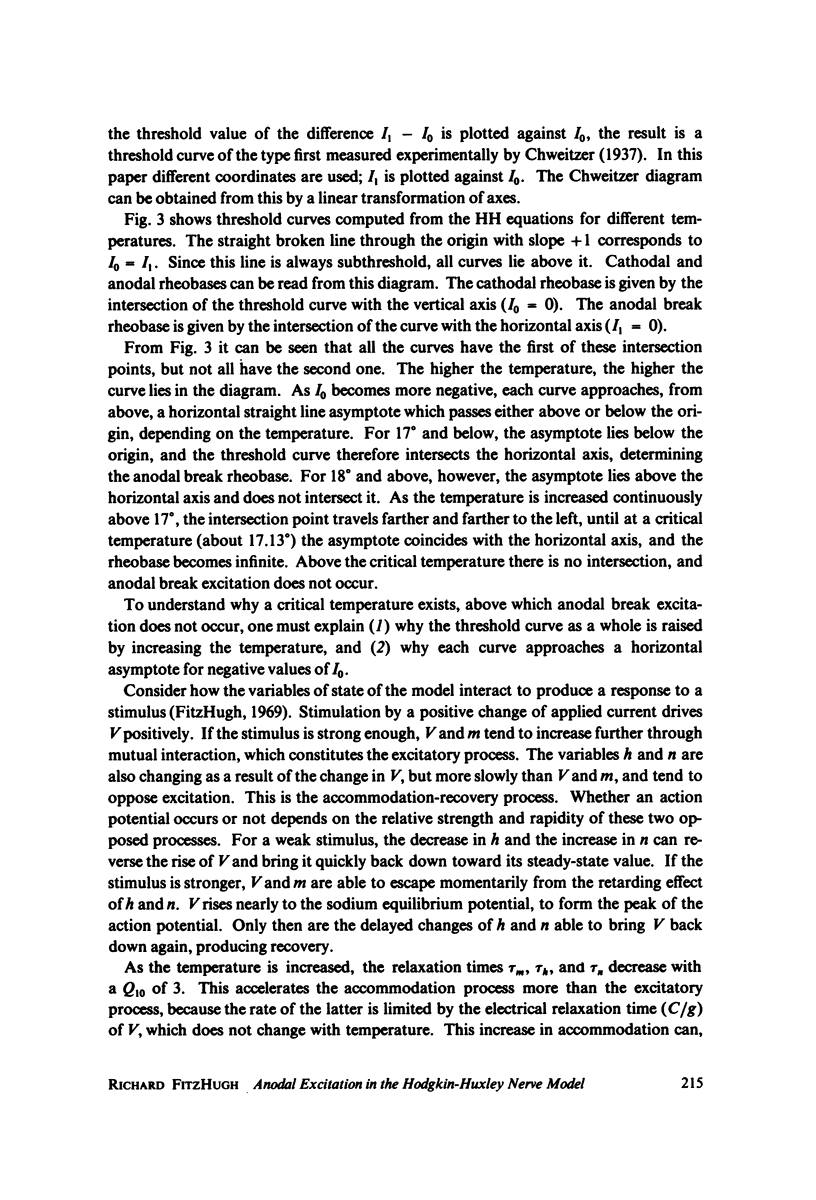
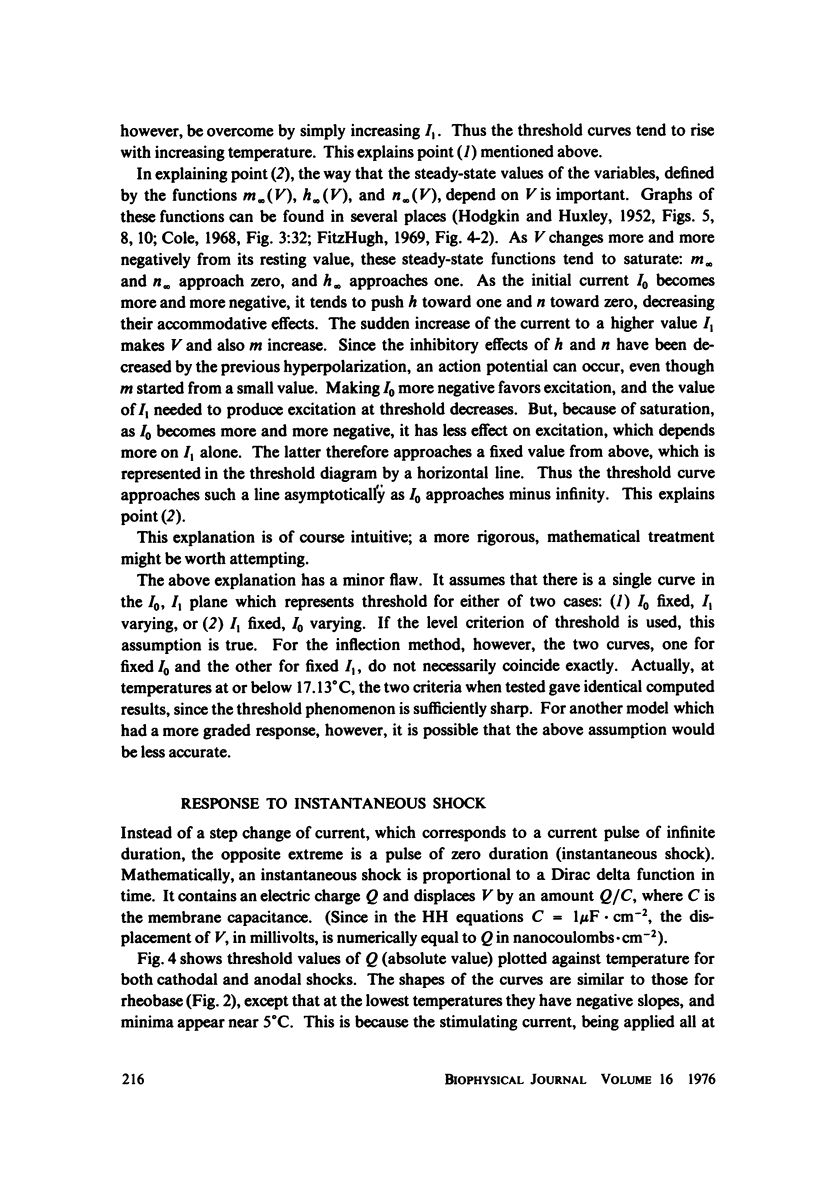
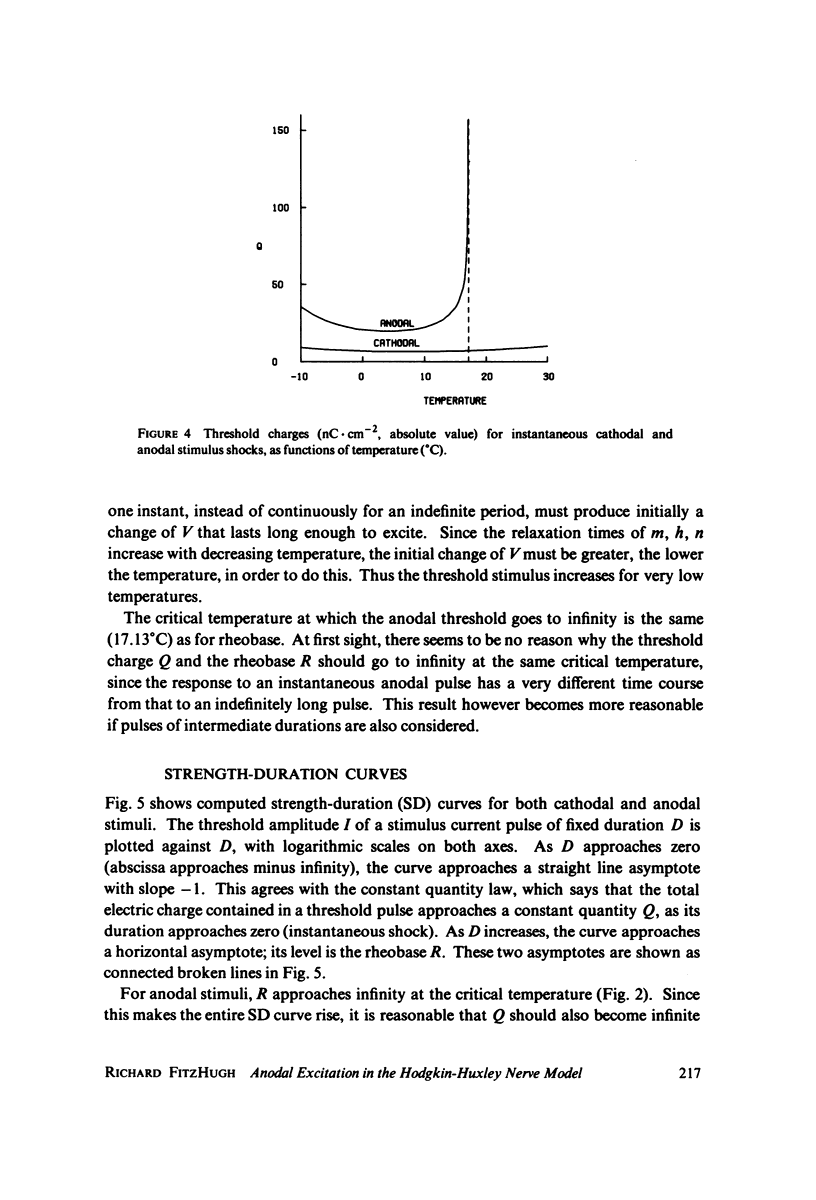
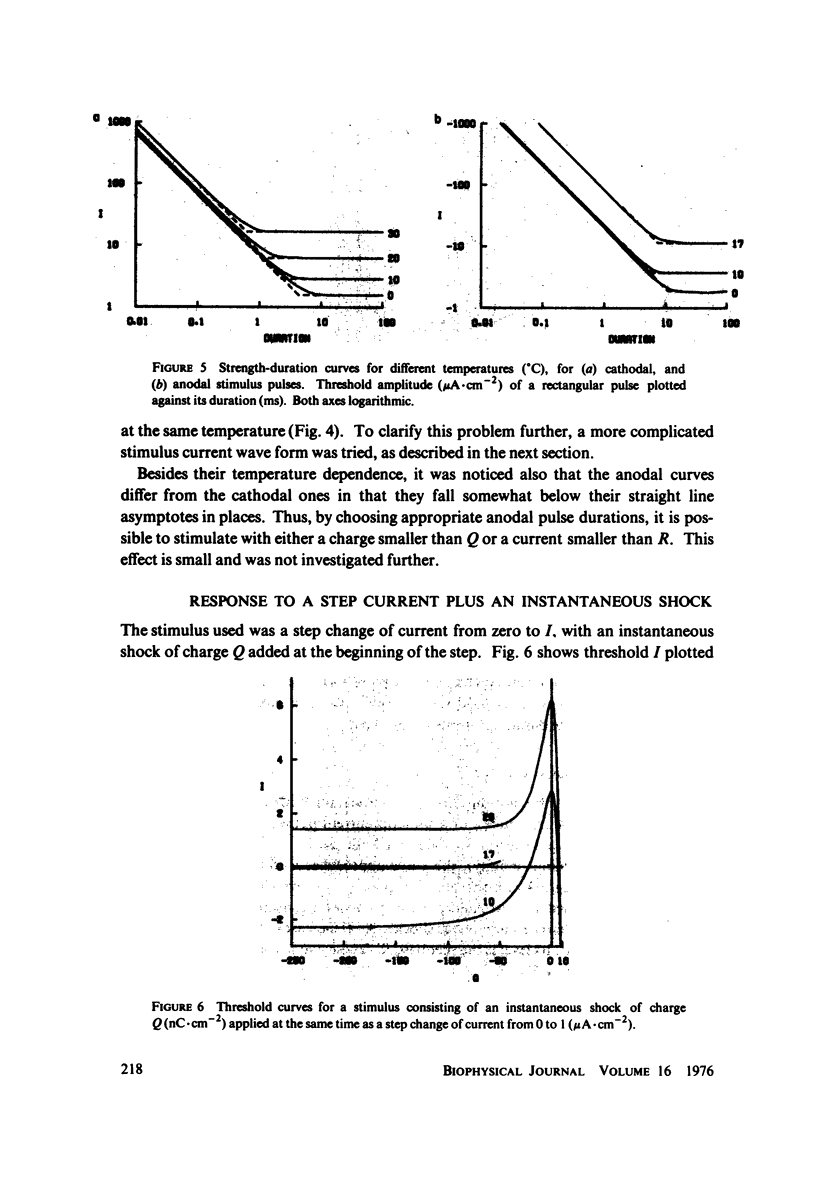
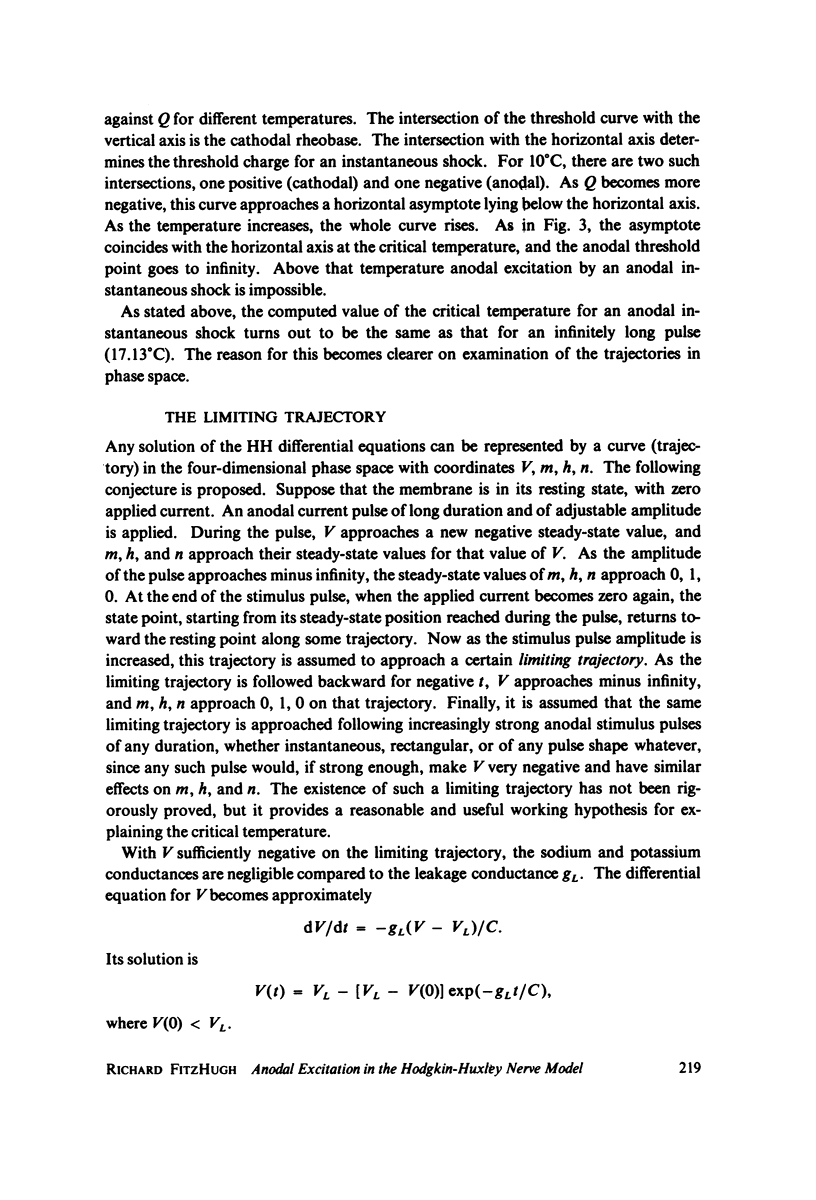
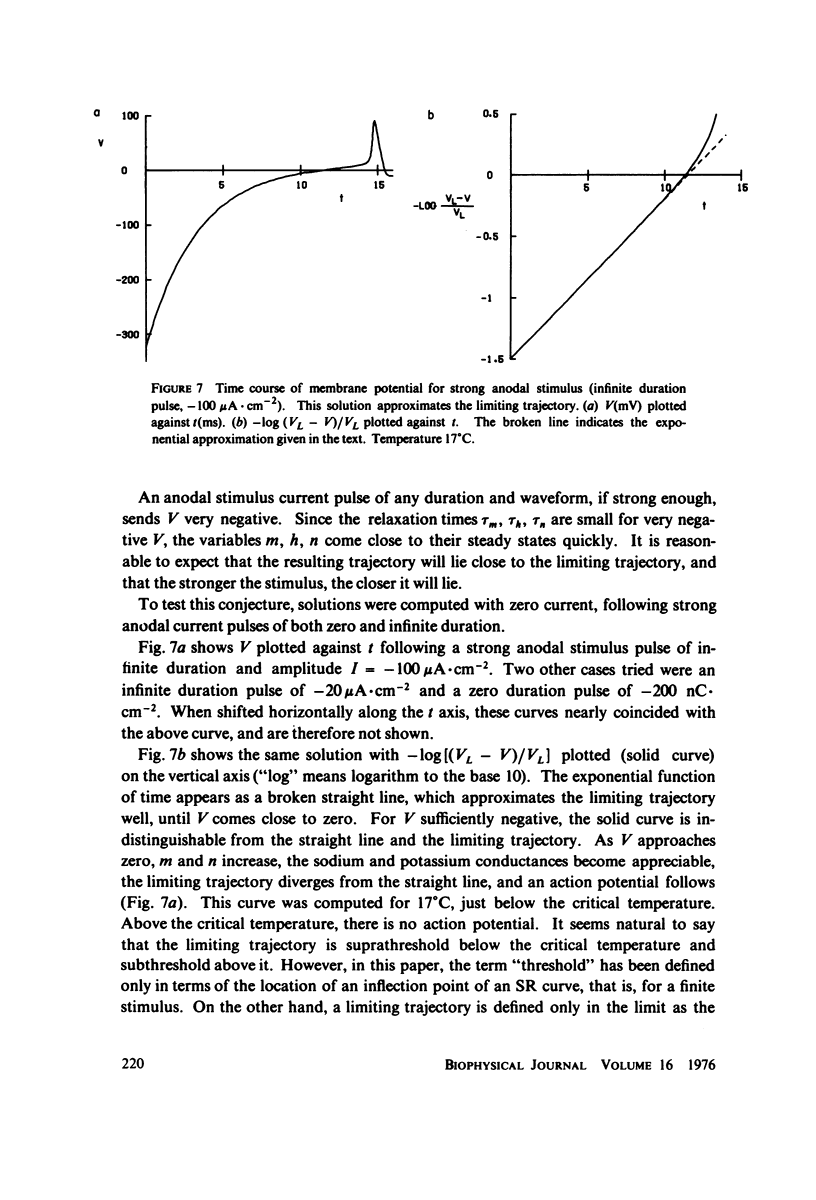
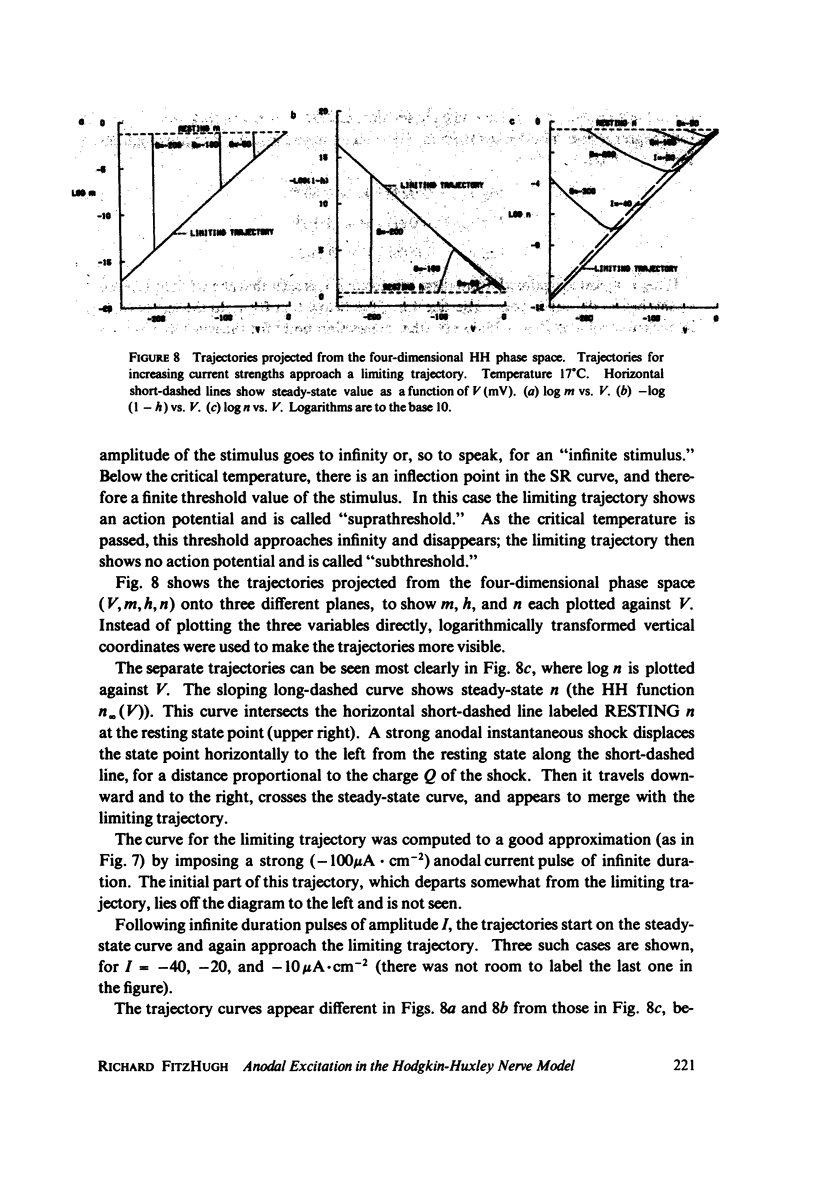
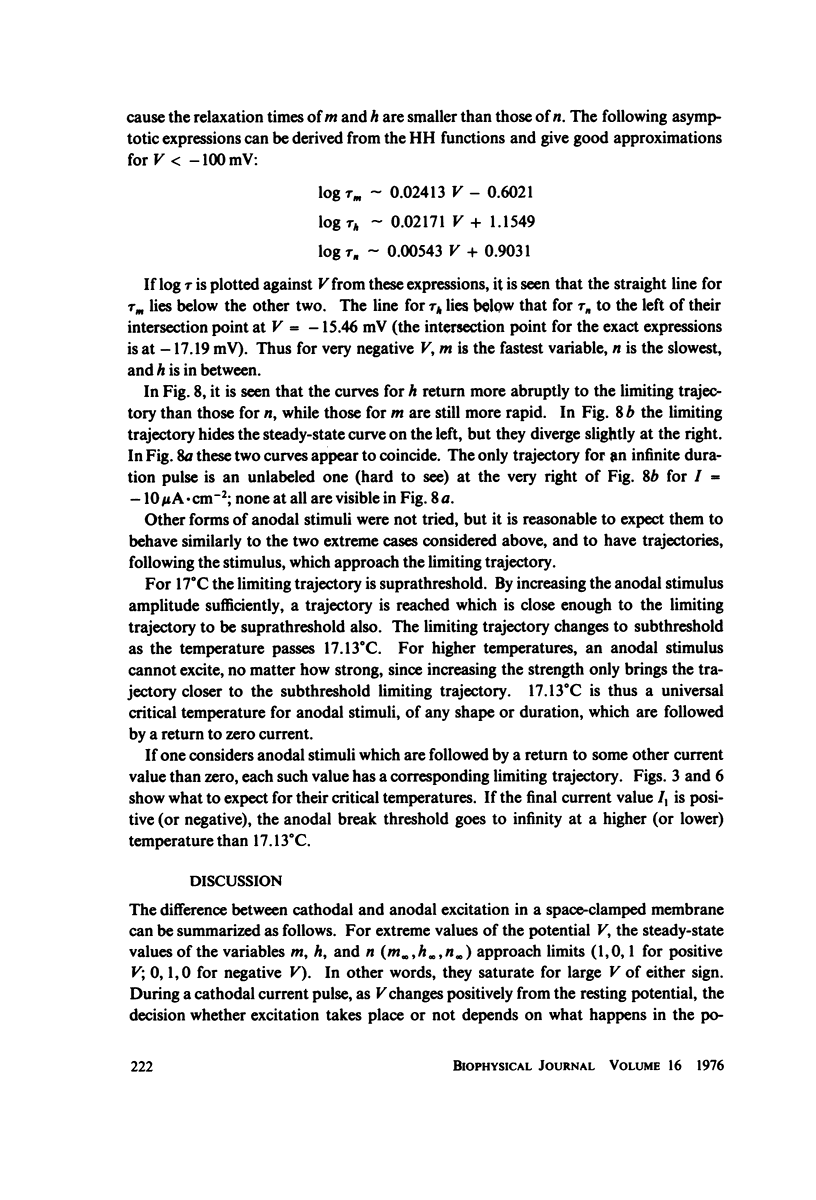
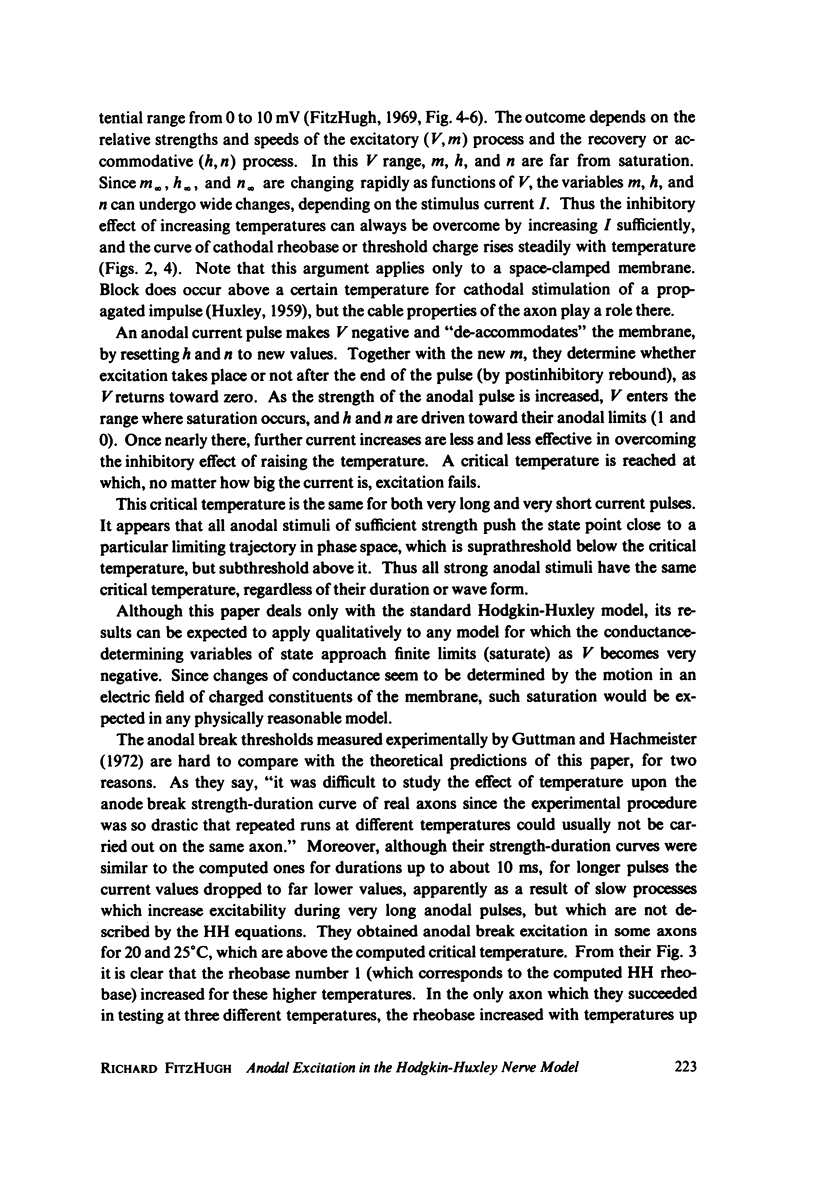
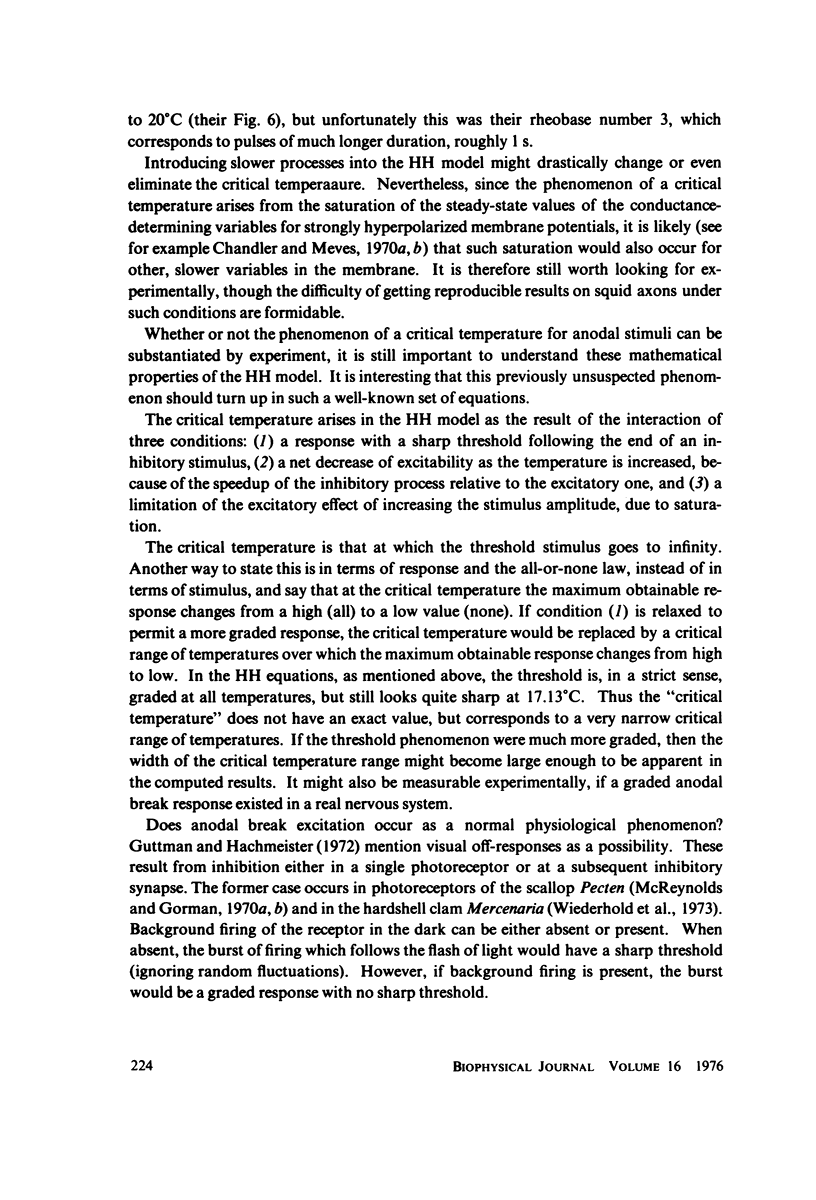
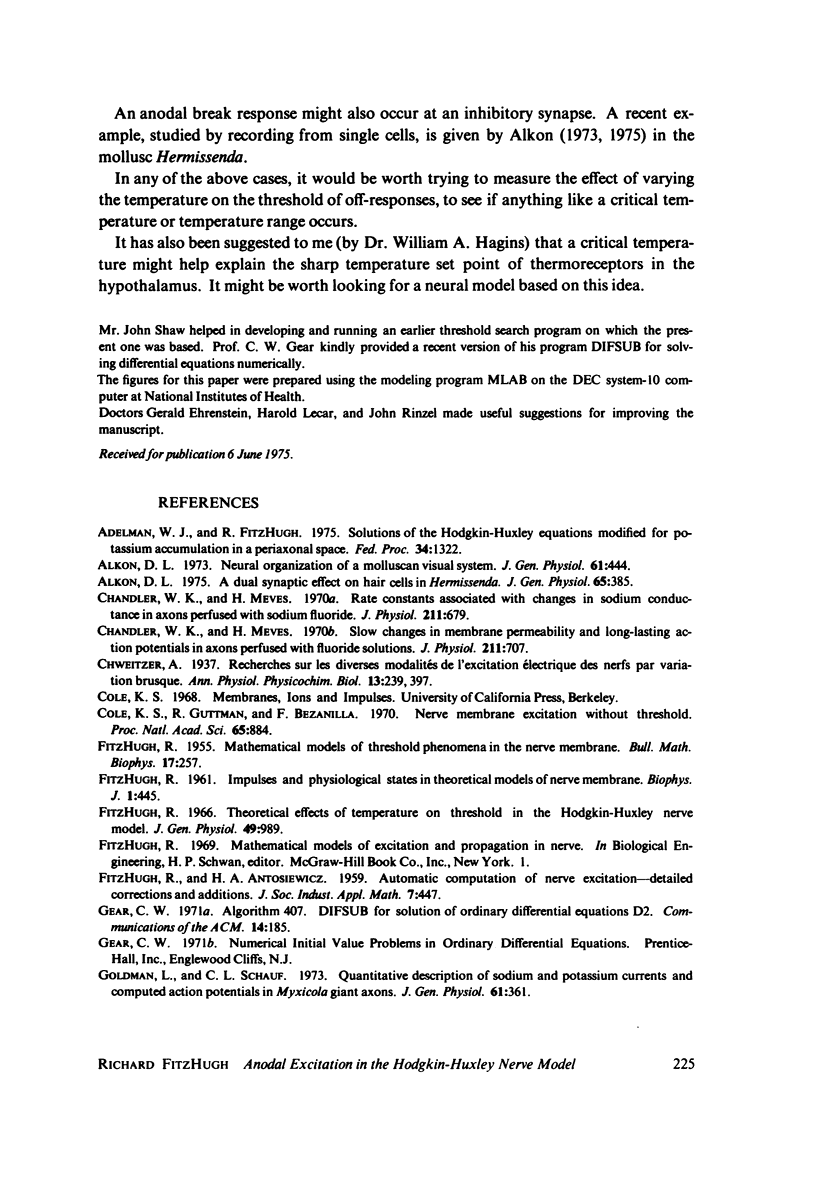
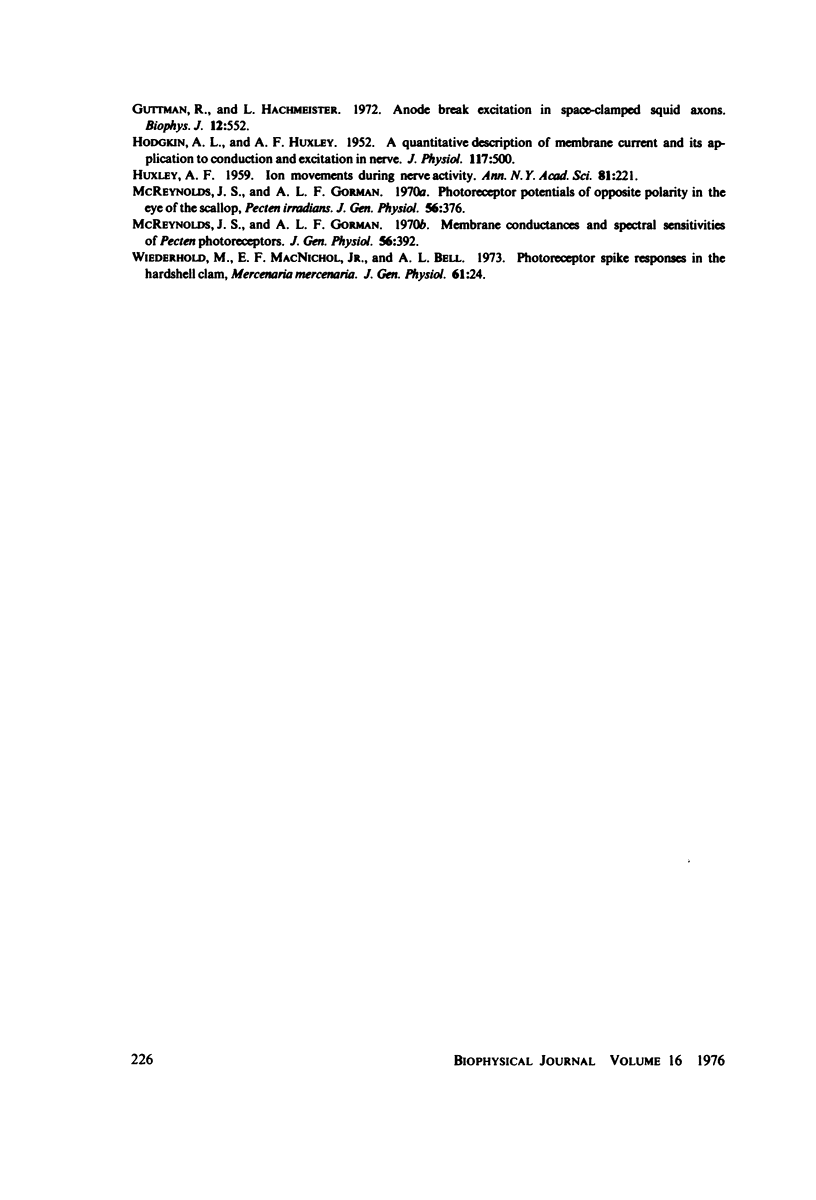
Selected References
These references are in PubMed. This may not be the complete list of references from this article.
- Adelman W. J., Jr, Fitzhugh R. Solutions of the Hodgkin-Huxley equations modified for potassium accumulation in a periaxonal space. Fed Proc. 1975 Apr;34(5):1322–1329. [PubMed] [Google Scholar]
- Alkon D. L. A dual synaptic effect on hair cells in Hermissenda. J Gen Physiol. 1975 Mar;65(3):385–397. doi: 10.1085/jgp.65.3.385. [DOI] [PMC free article] [PubMed] [Google Scholar]
- Alkon D. L. Neural organization of a molluscan visual system. J Gen Physiol. 1973 Apr;61(4):444–461. doi: 10.1085/jgp.61.4.444. [DOI] [PMC free article] [PubMed] [Google Scholar]
- Chandler W. K., Meves H. Rate constants associated with changes in sodium conductance in axons perfused with sodium fluoride. J Physiol. 1970 Dec;211(3):679–705. doi: 10.1113/jphysiol.1970.sp009299. [DOI] [PMC free article] [PubMed] [Google Scholar]
- Chandler W. K., Meves H. Slow changes in membrane permeability and long-lasting action potentials in axons perfused with fluoride solutions. J Physiol. 1970 Dec;211(3):707–728. doi: 10.1113/jphysiol.1970.sp009300. [DOI] [PMC free article] [PubMed] [Google Scholar]
- Cole K. S., Guttman R., Bezanilla F. Nerve membrane excitation without threshold. Proc Natl Acad Sci U S A. 1970 Apr;65(4):884–891. doi: 10.1073/pnas.65.4.884. [DOI] [PMC free article] [PubMed] [Google Scholar]
- Fitzhugh R. Impulses and Physiological States in Theoretical Models of Nerve Membrane. Biophys J. 1961 Jul;1(6):445–466. doi: 10.1016/s0006-3495(61)86902-6. [DOI] [PMC free article] [PubMed] [Google Scholar]
- Fitzhugh R. Theoretical effect of temperature on threshold in the Hodgkin-Huxley nerve model. J Gen Physiol. 1966 May;49(5):989–1005. doi: 10.1085/jgp.49.5.989. [DOI] [PMC free article] [PubMed] [Google Scholar]
- Goldman L., Schauf C. L. Quantitative description of sodium and potassium currents and computed action potentials in Myxicola giant axons. J Gen Physiol. 1973 Mar;61(3):361–384. doi: 10.1085/jgp.61.3.361. [DOI] [PMC free article] [PubMed] [Google Scholar]
- Guttman R., Hachmeister L. Anode break excitation in space-clamped squid axons. Biophys J. 1972 May;12(5):552–563. doi: 10.1016/S0006-3495(72)86103-4. [DOI] [PMC free article] [PubMed] [Google Scholar]
- HODGKIN A. L., HUXLEY A. F. A quantitative description of membrane current and its application to conduction and excitation in nerve. J Physiol. 1952 Aug;117(4):500–544. doi: 10.1113/jphysiol.1952.sp004764. [DOI] [PMC free article] [PubMed] [Google Scholar]
- HUXLEY A. F. Ion movements during nerve activity. Ann N Y Acad Sci. 1959 Aug 28;81:221–246. doi: 10.1111/j.1749-6632.1959.tb49311.x. [DOI] [PubMed] [Google Scholar]
- McReynolds J. S., Gorman A. L. Membrane conductances and spectral sensitivities of Pecten photoreceptors. J Gen Physiol. 1970 Sep;56(3):392–406. doi: 10.1085/jgp.56.3.392. [DOI] [PMC free article] [PubMed] [Google Scholar]
- McReynolds J. S., Gorman A. L. Photoreceptor potentials of opposite polarity in the eye of the scallop, Pecten irradians. J Gen Physiol. 1970 Sep;56(3):376–391. doi: 10.1085/jgp.56.3.376. [DOI] [PMC free article] [PubMed] [Google Scholar]
- Wiederhold M. L., MacNichol E. F., Jr, Bell A. L. Photoreceptor spike responses in the hardshell clam, Mercenaria mercenaria. J Gen Physiol. 1973 Jan;61(1):24–55. doi: 10.1085/jgp.61.1.24. [DOI] [PMC free article] [PubMed] [Google Scholar]