Abstract
The effect of the local anesthetic QX222 on the kinetics of miniature endplate currents and acetylcholine induced endplate current fluctuations was studied in voltage clamped cutaneous pectoris muscle of Rana pipiens. Both the endplate current fluctuation spectra and the miniature endplate current decay consisted of two or three components depending upon the holding potential and local anesthetic concentration. The cutoff frequency of each spectral component was equal to the decay rate of its corresponding constituent of the miniature endplate current. Comparison of the relative amplitudes of the spectral and miniature endplate components indicated that QX222 did not act by creating two kinetically distinct populations of acetylcholine receptors. QX222 action could be explained by alteration of the acetylcholine receptors such that they sequentially change conformation form one open state to another. A specific case in which QX222 binds to the open state of the acetyl-choline receptor creating a blocked state, was found to account for the observed relationship between the relative amplitudes of the miniature endplate current and spectral components, as well as the previously observed voltage and concentration sensitivity of the decay rates of endplate current components.
Full text
PDF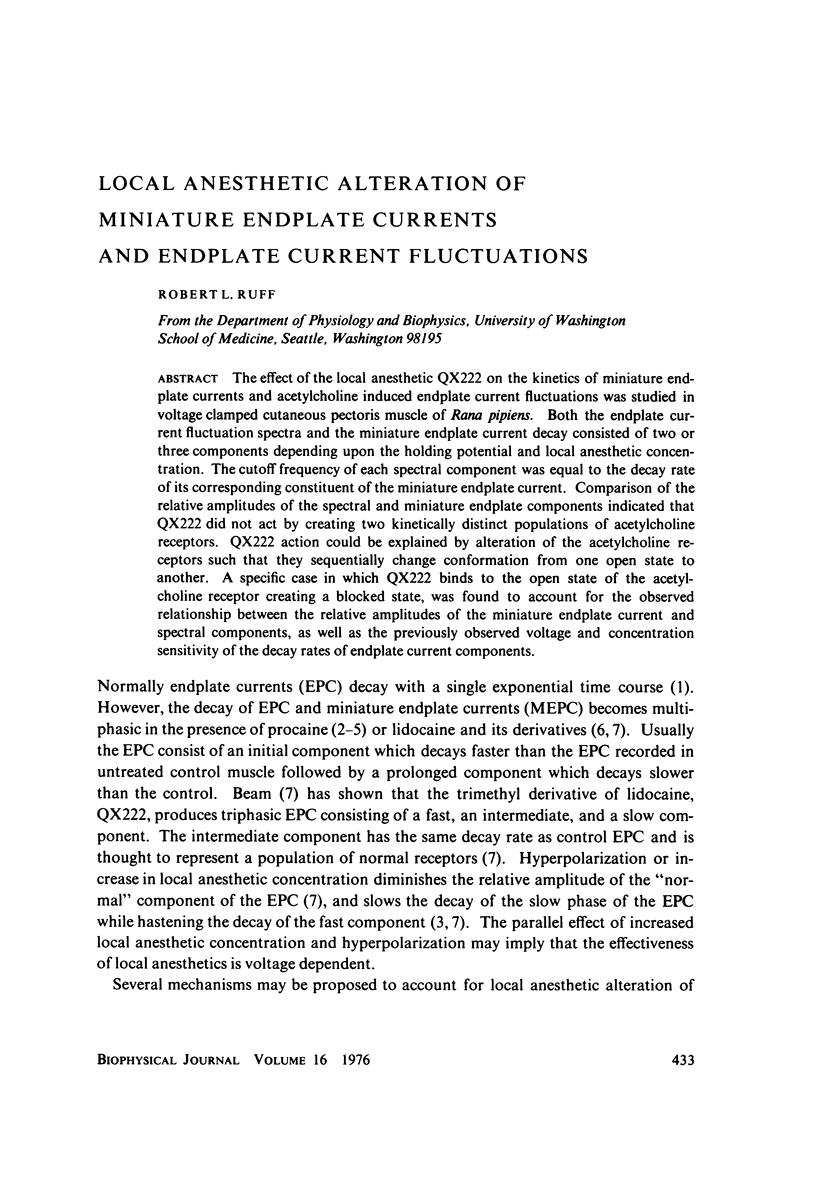
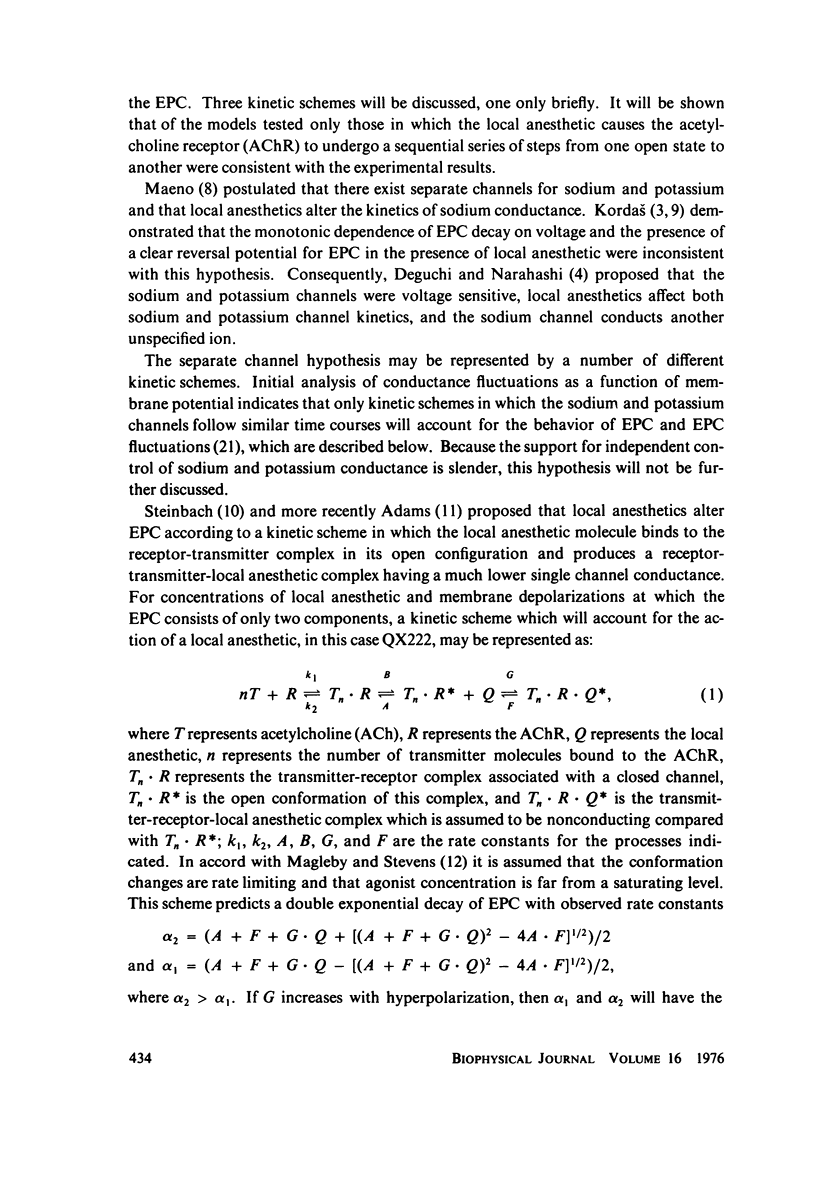
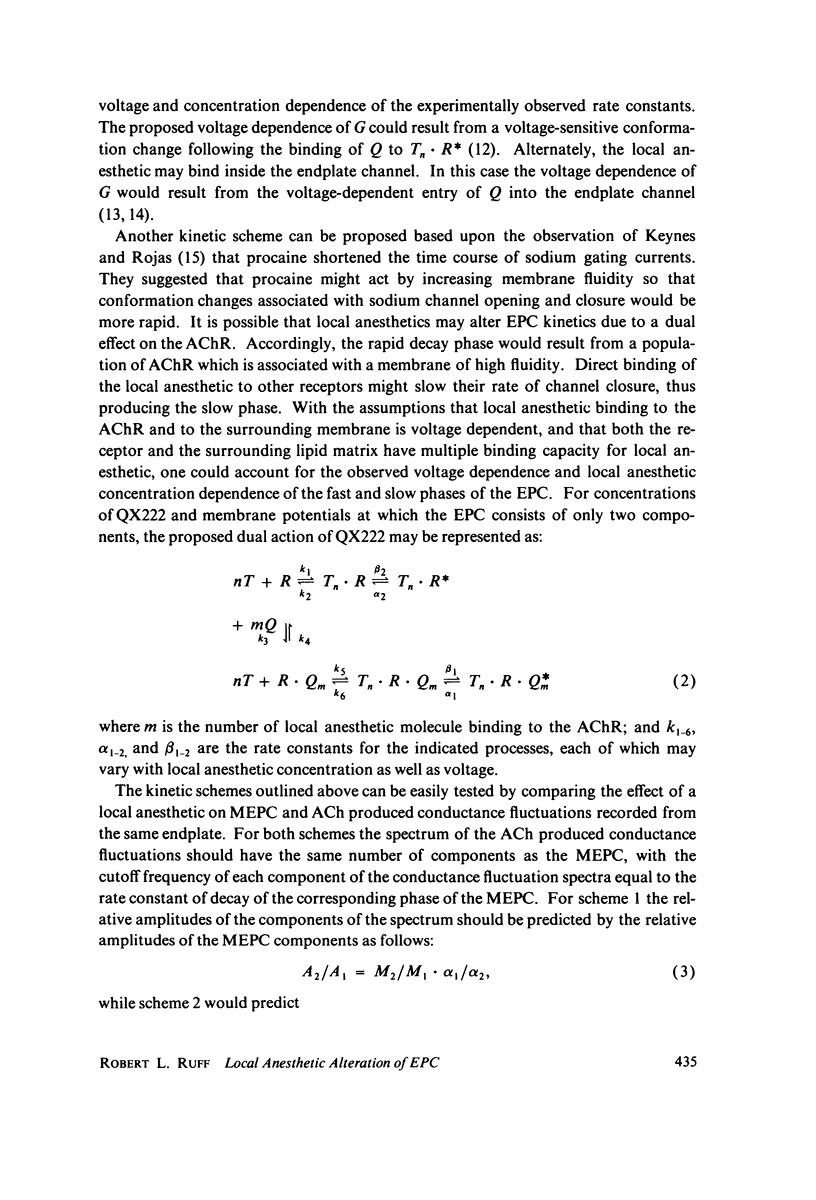
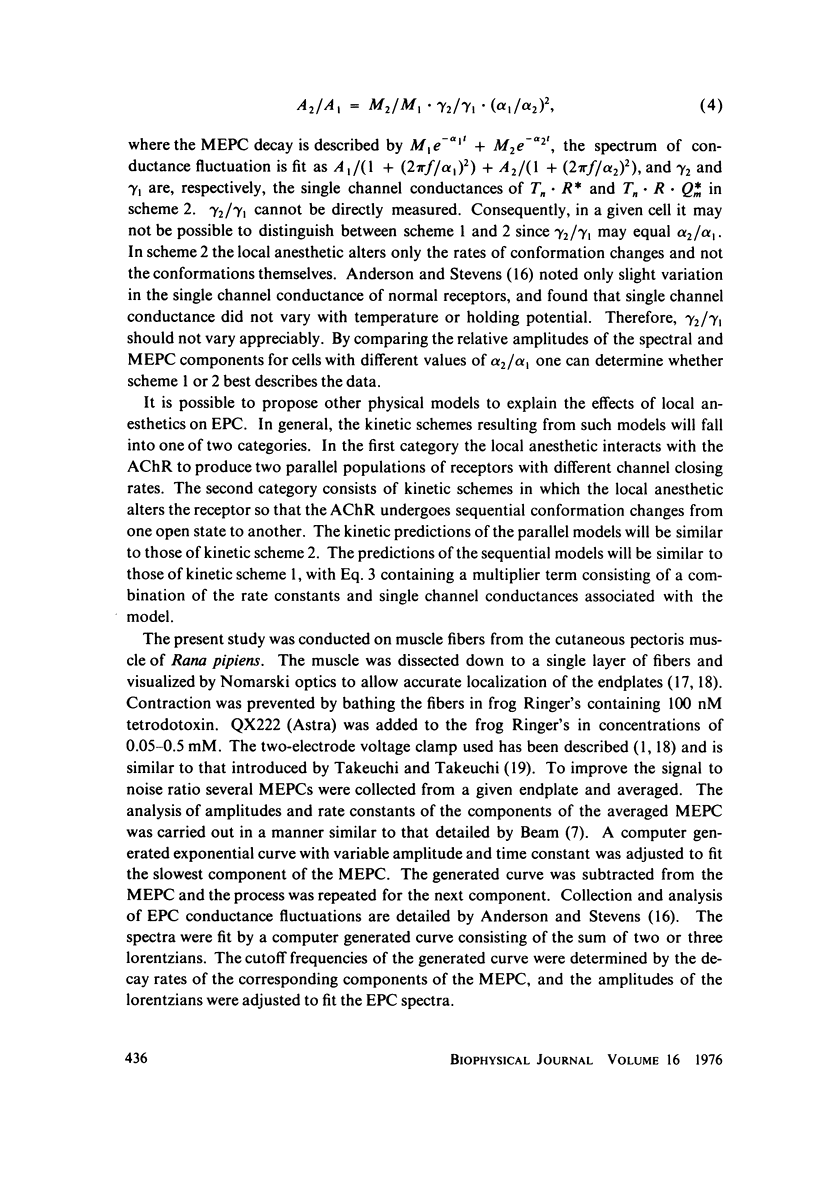
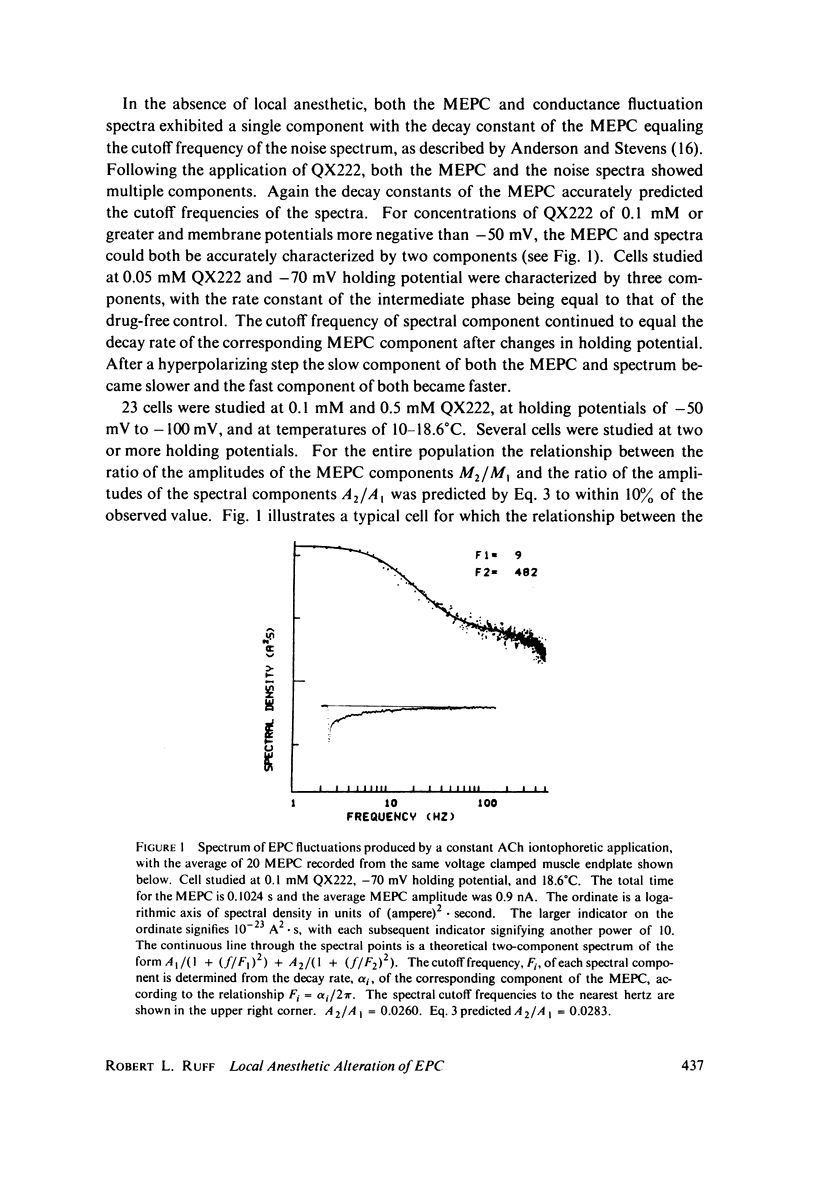
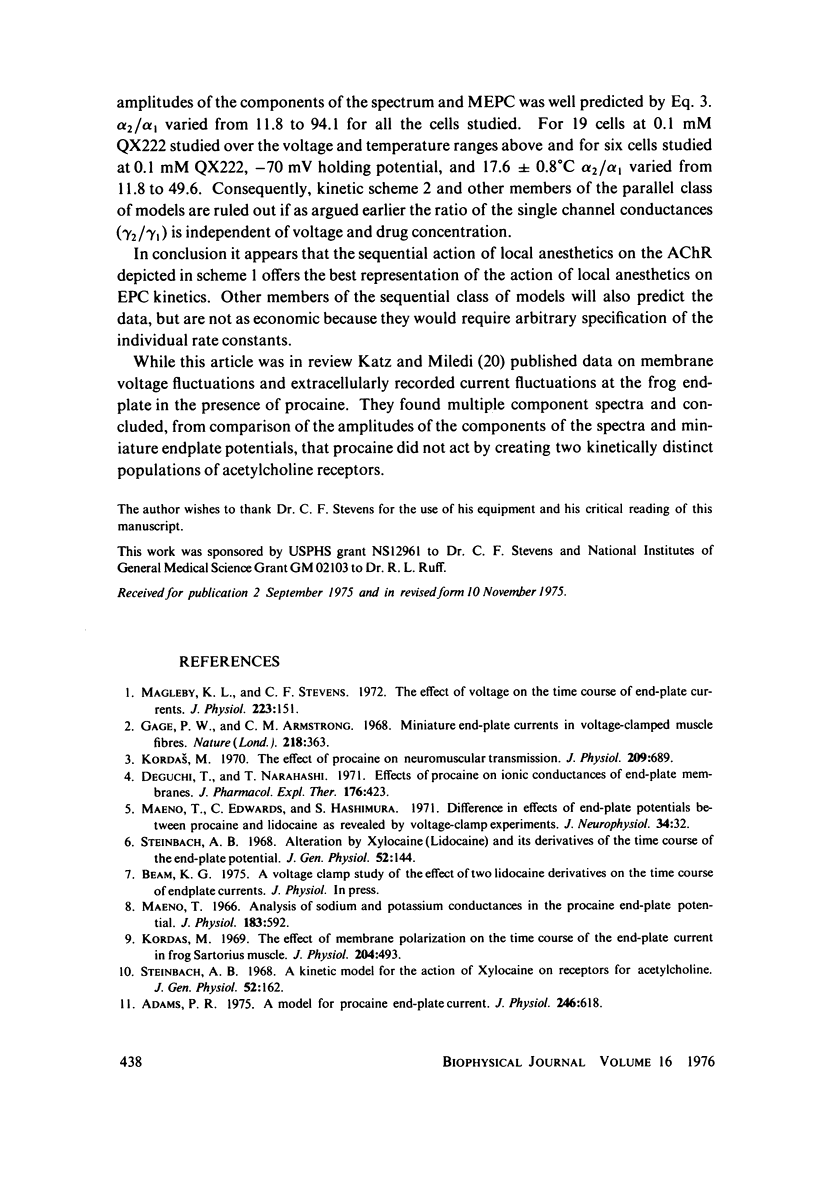
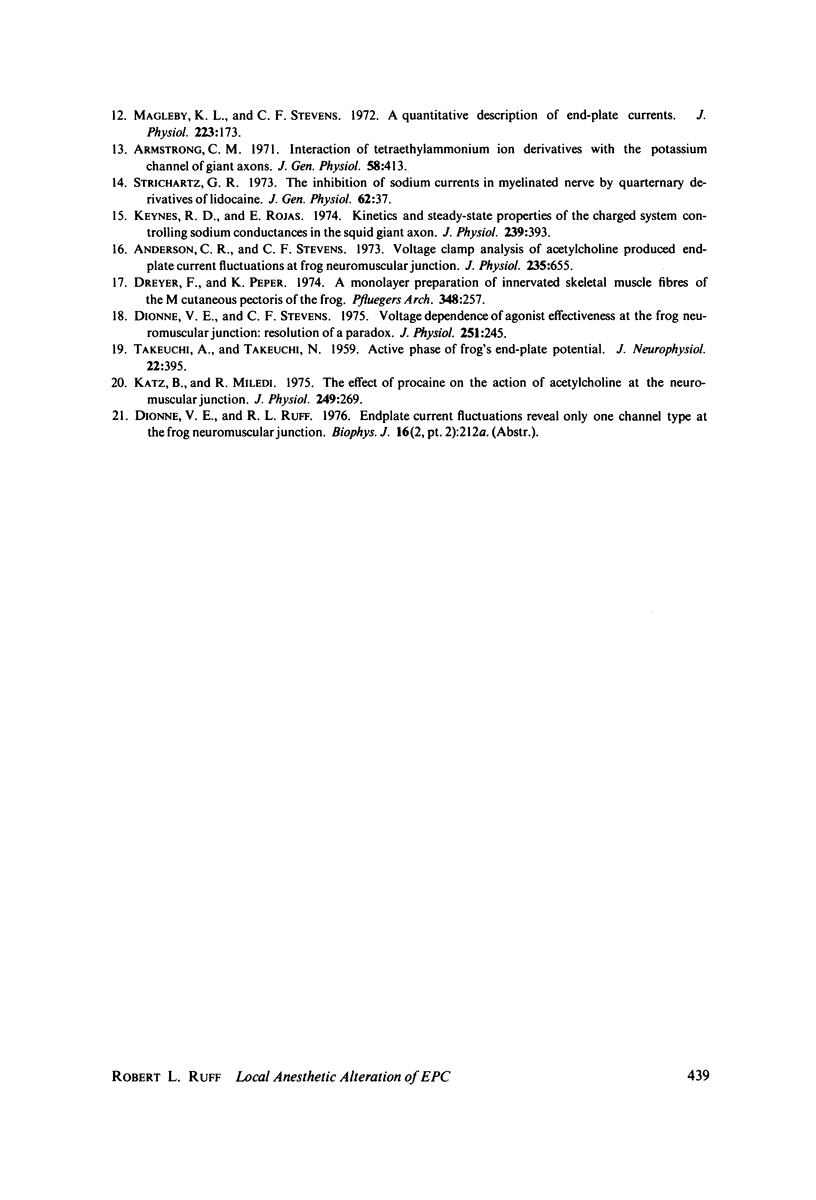
Selected References
These references are in PubMed. This may not be the complete list of references from this article.
- Anderson C. R., Stevens C. F. Voltage clamp analysis of acetylcholine produced end-plate current fluctuations at frog neuromuscular junction. J Physiol. 1973 Dec;235(3):655–691. doi: 10.1113/jphysiol.1973.sp010410. [DOI] [PMC free article] [PubMed] [Google Scholar]
- Armstrong C. M. Interaction of tetraethylammonium ion derivatives with the potassium channels of giant axons. J Gen Physiol. 1971 Oct;58(4):413–437. doi: 10.1085/jgp.58.4.413. [DOI] [PMC free article] [PubMed] [Google Scholar]
- Deguchi T., Narahashi T. Effects of procaine on ionic conductances of end-plate membranes. J Pharmacol Exp Ther. 1971 Feb;176(2):423–433. [PubMed] [Google Scholar]
- Dionne V. E., Stevens C. F. Voltage dependence of agonist effectiveness at the frog neuromuscular junction: resolution of a paradox. J Physiol. 1975 Oct;251(2):245–270. doi: 10.1113/jphysiol.1975.sp011090. [DOI] [PMC free article] [PubMed] [Google Scholar]
- Dreyer F., Peper K. A monolayer preparation of innervated skeletal muscle fibres of the m. cutaneus pectoris of the frog. Pflugers Arch. 1974 Apr 22;348(3):257–262. doi: 10.1007/BF00587416. [DOI] [PubMed] [Google Scholar]
- Gage P. W., Armstrong C. M. Miniature end-plate currents in voltage-clamped muscle fibre. Nature. 1968 Apr 27;218(5139):363–365. doi: 10.1038/218363b0. [DOI] [PubMed] [Google Scholar]
- Katz B., Miledi R. The effect of procaine on the action of acetylcholine at the neuromuscular junction. J Physiol. 1975 Jul;249(2):269–284. doi: 10.1113/jphysiol.1975.sp011015. [DOI] [PMC free article] [PubMed] [Google Scholar]
- Keynes R. D., Rojas E. Kinetics and steady-state properties of the charged system controlling sodium conductance in the squid giant axon. J Physiol. 1974 Jun;239(2):393–434. doi: 10.1113/jphysiol.1974.sp010575. [DOI] [PMC free article] [PubMed] [Google Scholar]
- Kordas M. The effect of membrane polarization on the time course of the end-plate current in frog sartorius muscle. J Physiol. 1969 Oct;204(2):493–502. doi: 10.1113/jphysiol.1969.sp008926. [DOI] [PMC free article] [PubMed] [Google Scholar]
- Kordas M. The effect of procaine on neuromuscular transmission. J Physiol. 1970 Aug;209(3):689–699. doi: 10.1113/jphysiol.1970.sp009186. [DOI] [PMC free article] [PubMed] [Google Scholar]
- Maeno T. Analysis of sodium and potassium conductances in the procaine end-plate potential. J Physiol. 1966 Apr;183(3):592–606. doi: 10.1113/jphysiol.1966.sp007886. [DOI] [PMC free article] [PubMed] [Google Scholar]
- Maeno T., Edwards C., Hashimura S. Difference in effects of end-plate potentials between procaine and lidocaine as revealed by voltage-clamp experiments. J Neurophysiol. 1971 Jan;34(1):32–46. doi: 10.1152/jn.1971.34.1.32. [DOI] [PubMed] [Google Scholar]
- Magleby K. L., Stevens C. F. A quantitative description of end-plate currents. J Physiol. 1972 May;223(1):173–197. doi: 10.1113/jphysiol.1972.sp009840. [DOI] [PMC free article] [PubMed] [Google Scholar]
- Magleby K. L., Stevens C. F. The effect of voltage on the time course of end-plate currents. J Physiol. 1972 May;223(1):151–171. doi: 10.1113/jphysiol.1972.sp009839. [DOI] [PMC free article] [PubMed] [Google Scholar]
- Steinbach A. B. A kinetic model for the action of xylocaine on receptors for acetylcholine. J Gen Physiol. 1968 Jul;52(1):162–180. doi: 10.1085/jgp.52.1.162. [DOI] [PMC free article] [PubMed] [Google Scholar]
- Steinbach A. B. Alteration by xylocaine (lidocaine) and its derivatives of the time course of the end plate potential. J Gen Physiol. 1968 Jul;52(1):144–161. doi: 10.1085/jgp.52.1.144. [DOI] [PMC free article] [PubMed] [Google Scholar]
- Strichartz G. R. The inhibition of sodium currents in myelinated nerve by quaternary derivatives of lidocaine. J Gen Physiol. 1973 Jul;62(1):37–57. doi: 10.1085/jgp.62.1.37. [DOI] [PMC free article] [PubMed] [Google Scholar]
- TAKEUCHI A., TAKEUCHI N. Active phase of frog's end-plate potential. J Neurophysiol. 1959 Jul;22(4):395–411. doi: 10.1152/jn.1959.22.4.395. [DOI] [PubMed] [Google Scholar]