Abstract
The apparent cytoplasmic resistivity of two different giant cells has been measured using an extension of a previously developed single microelectrode technique. Each cell is penetrated by a metal microelectrode whose complex impedance is measured as a function of frequency between 500 kHz and 5.7 MHz. By plotting the measured impedance data on the complex Z plane and extrapolating the data to infinite frequency, the substantial effects of electrode polarization can be overcome. For Aplysia giant neurons and muscle fibers of the giant barnacle, the extrapolated cytoplasmic specific resistivities are 40 and 74 omega-cm, respectively, at infinite frequency. The barnacle data are in excellent agreement with sarcoplasmic resistivity values derived from the measured cable properties of other marine organisms, and from high frequency conductivity cell measurements in intact barnacle muscle tissue. In the Aplysia neurons, the frequency-dependent part of the electrode impedance is larger when the electrode is in a cell than when it is in an electrolyte solution with the same specific resistivity as the aqueous cytoplasm; however, the phase angle of the frequency-dependent component of the electrode impedance is the same in both cases. This suggests that the high apparent values of cytoplasmic resistivity found using the single microelectrode technique at lower frequencies probably reflect an artifact caused by reduction of the effective surface area of the electrode by intracellular membranes, with a corresponding increase in its polarization impedance.
Full text
PDF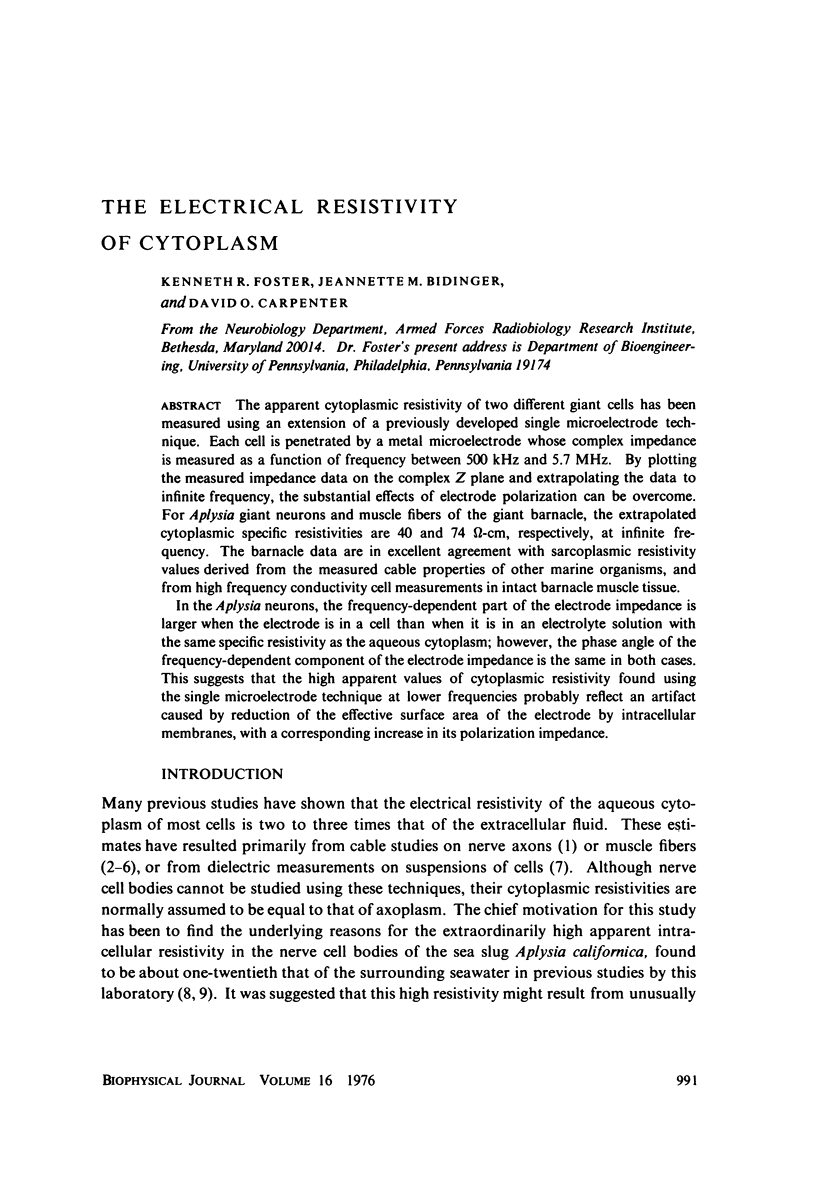
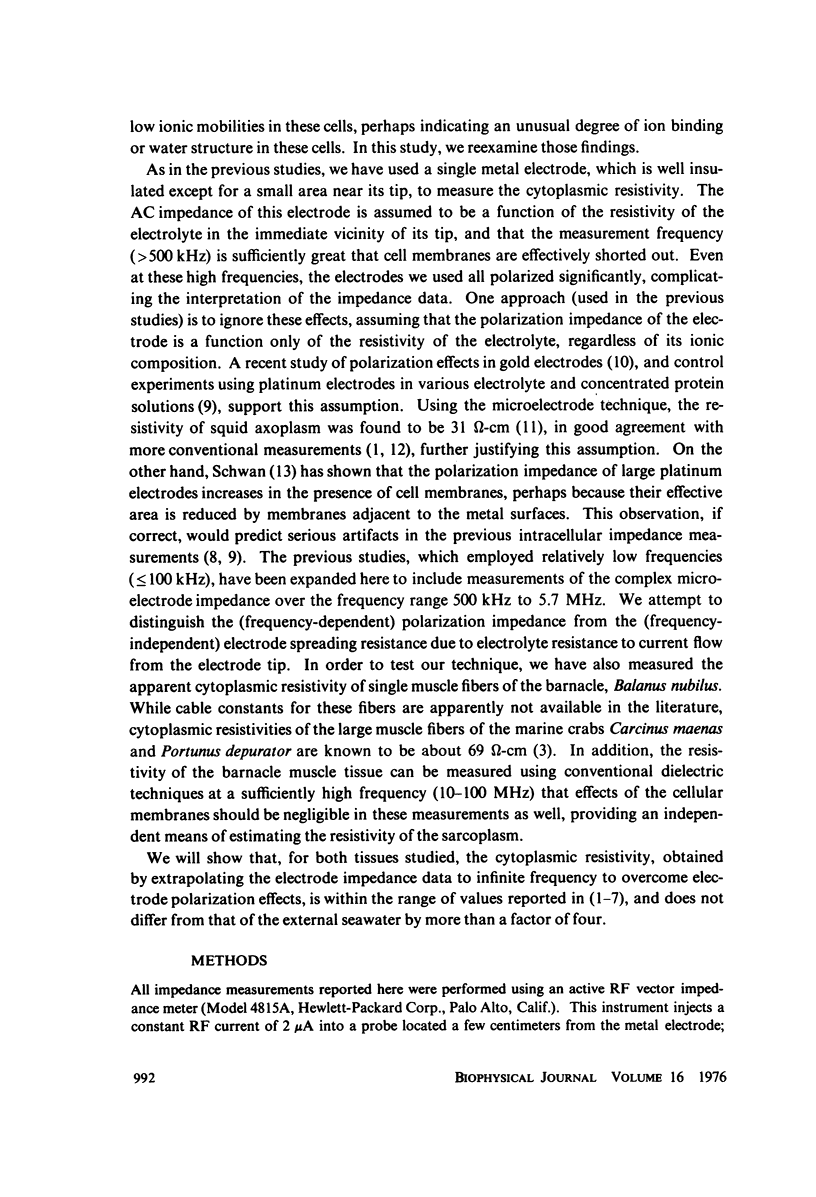
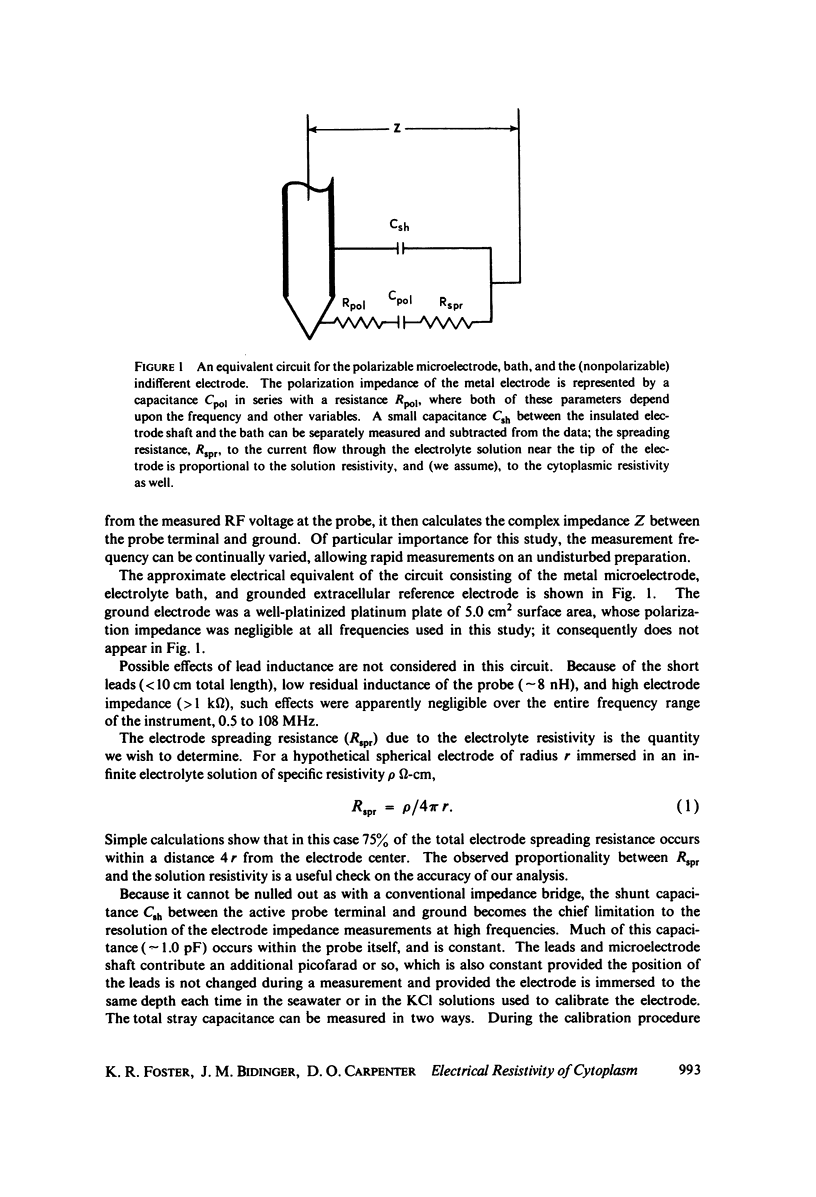
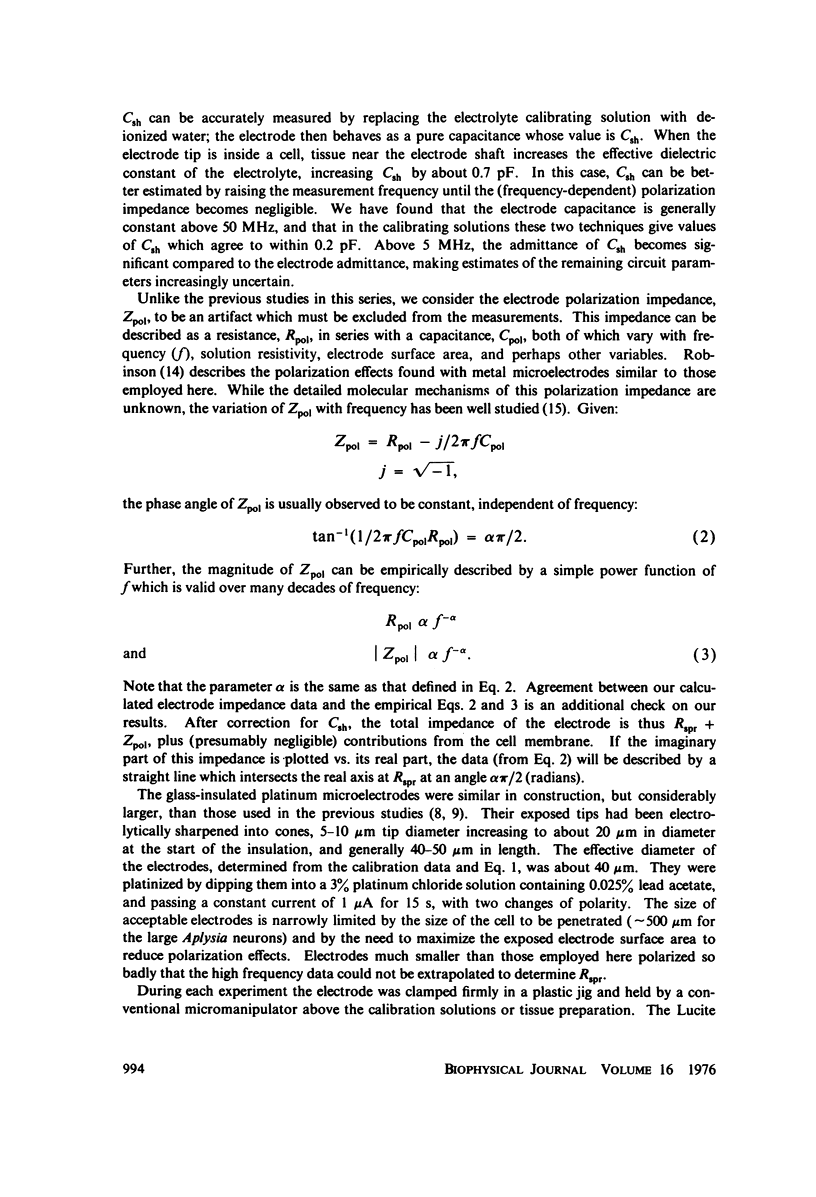
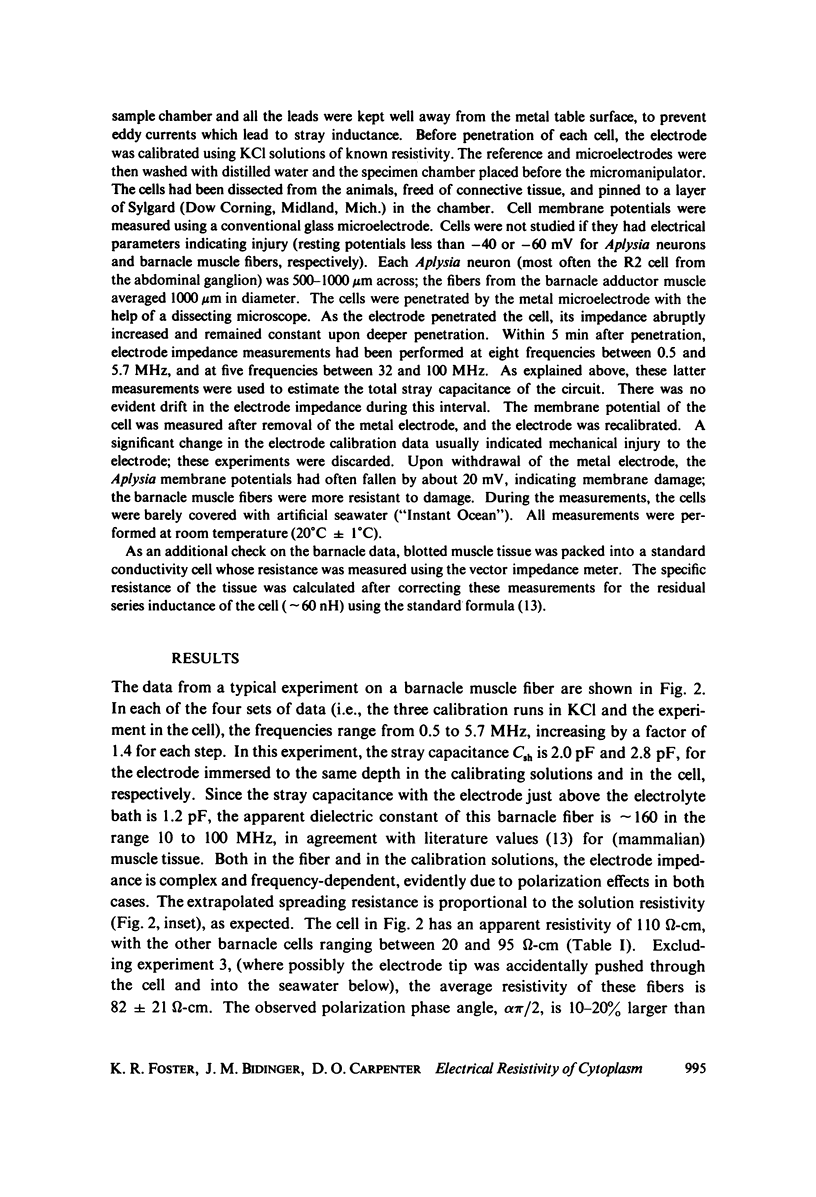
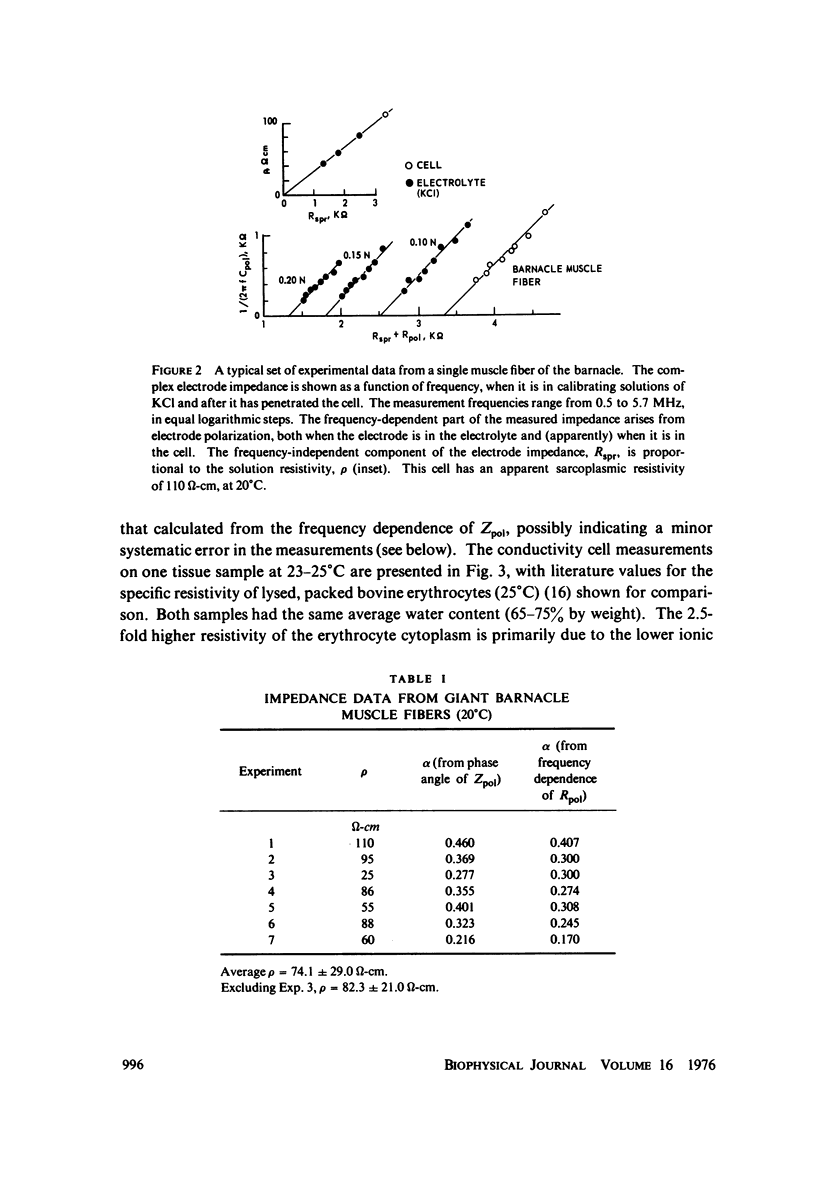
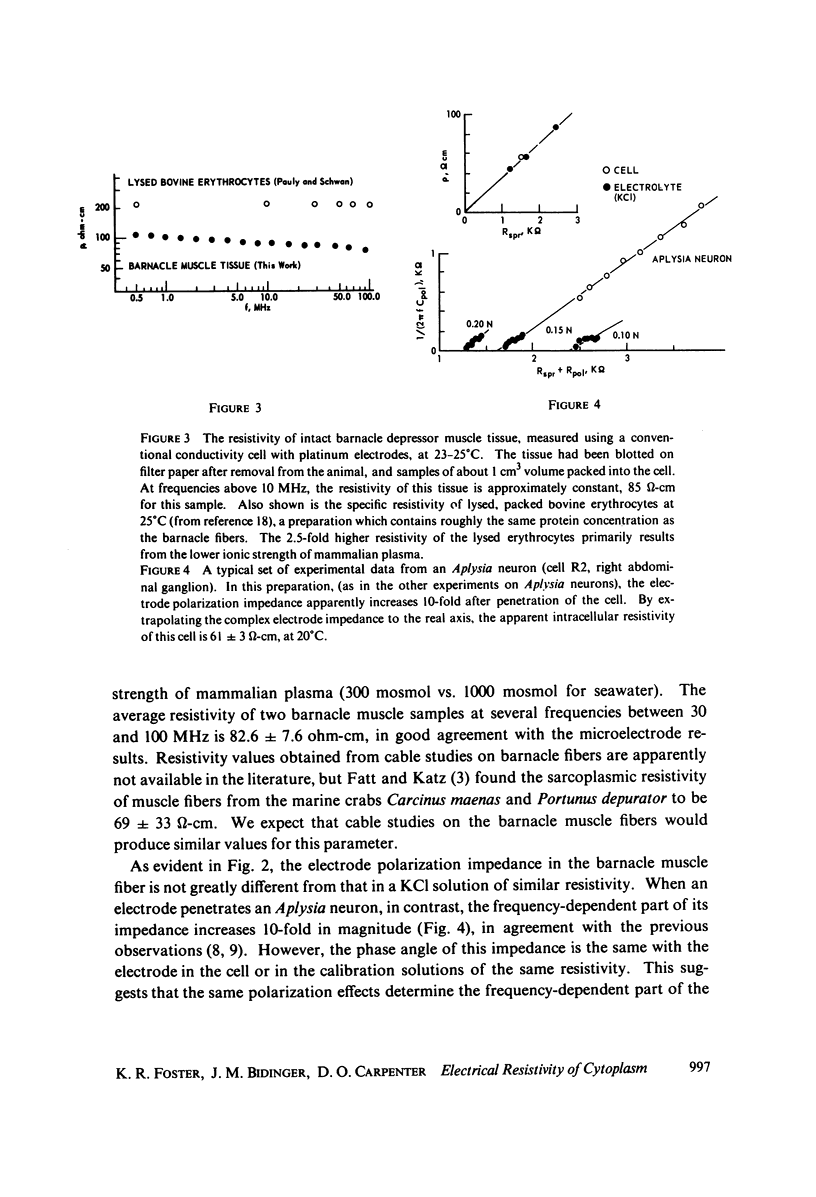
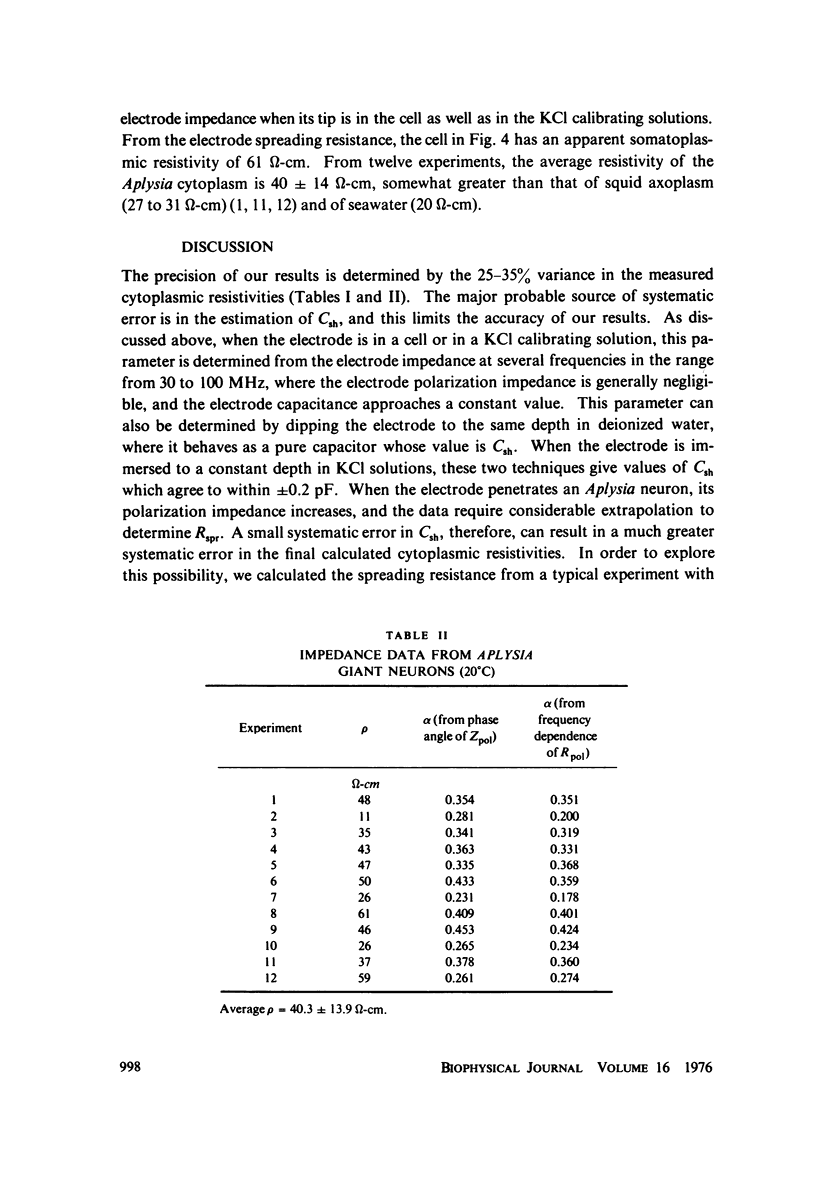
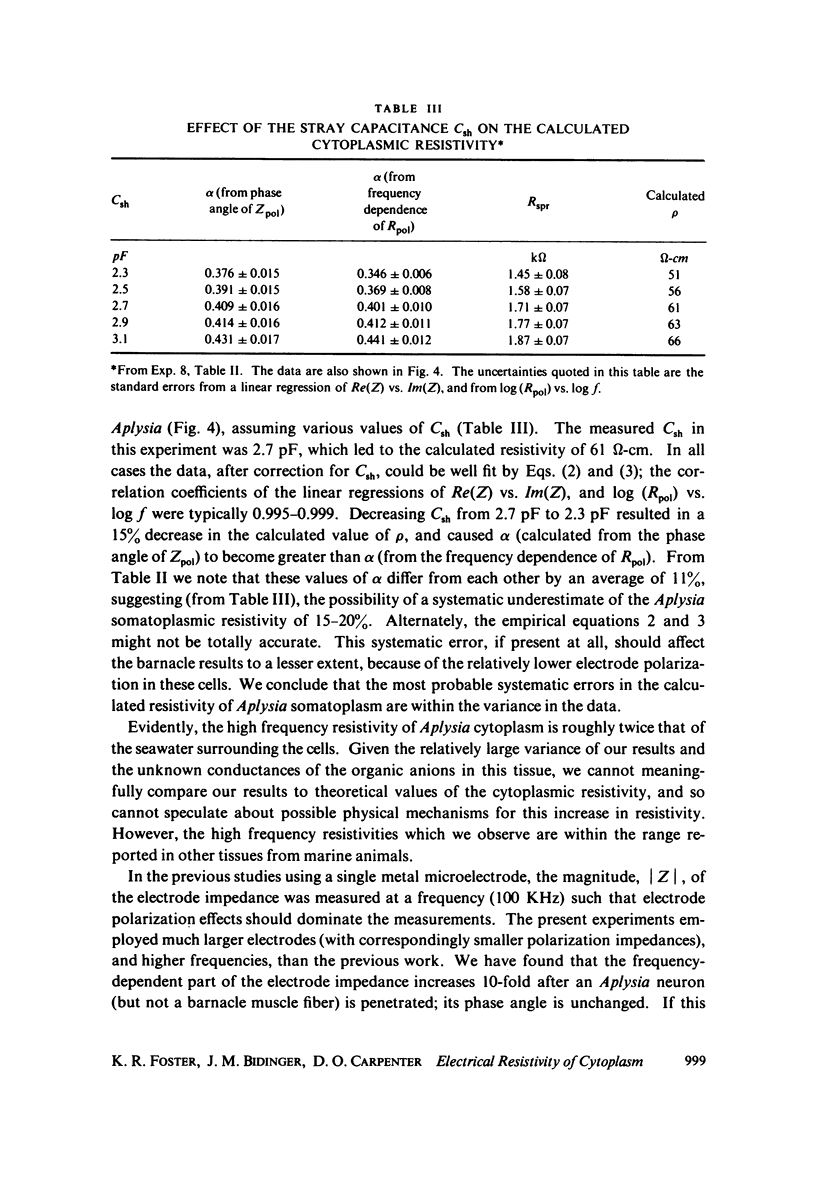
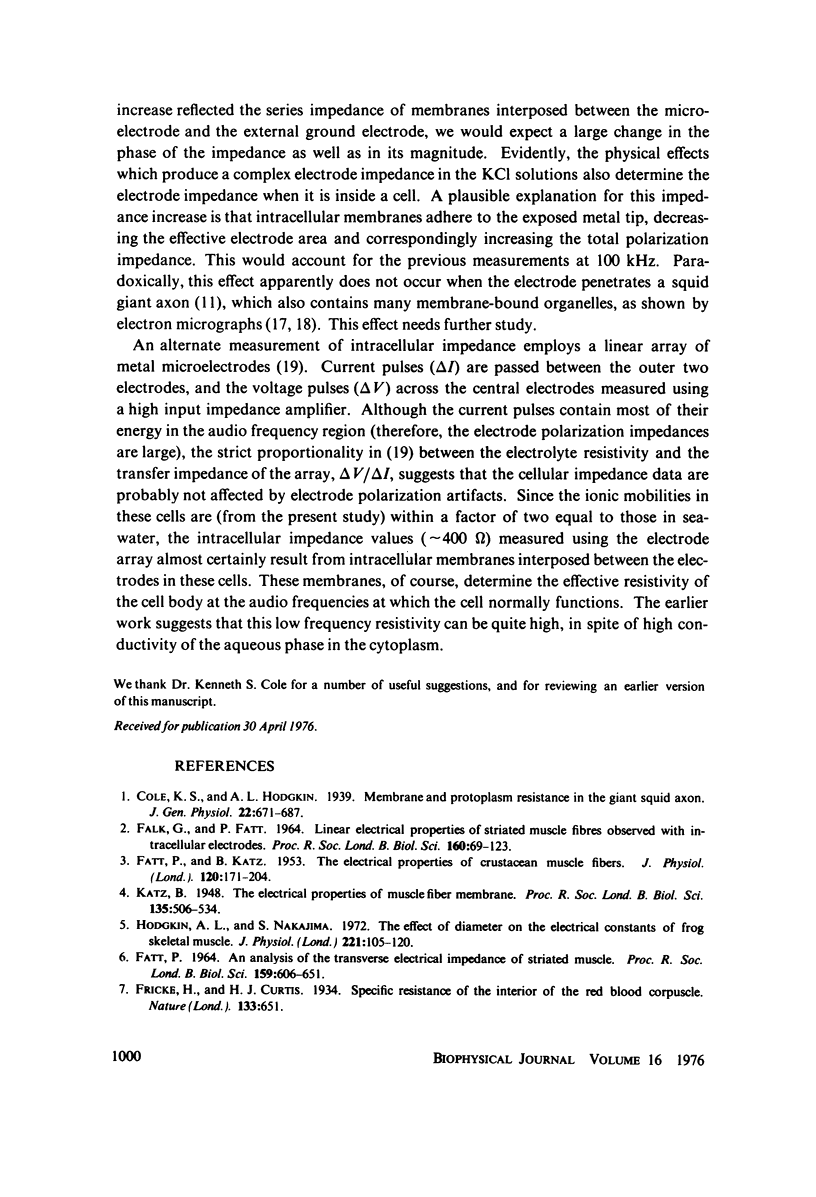
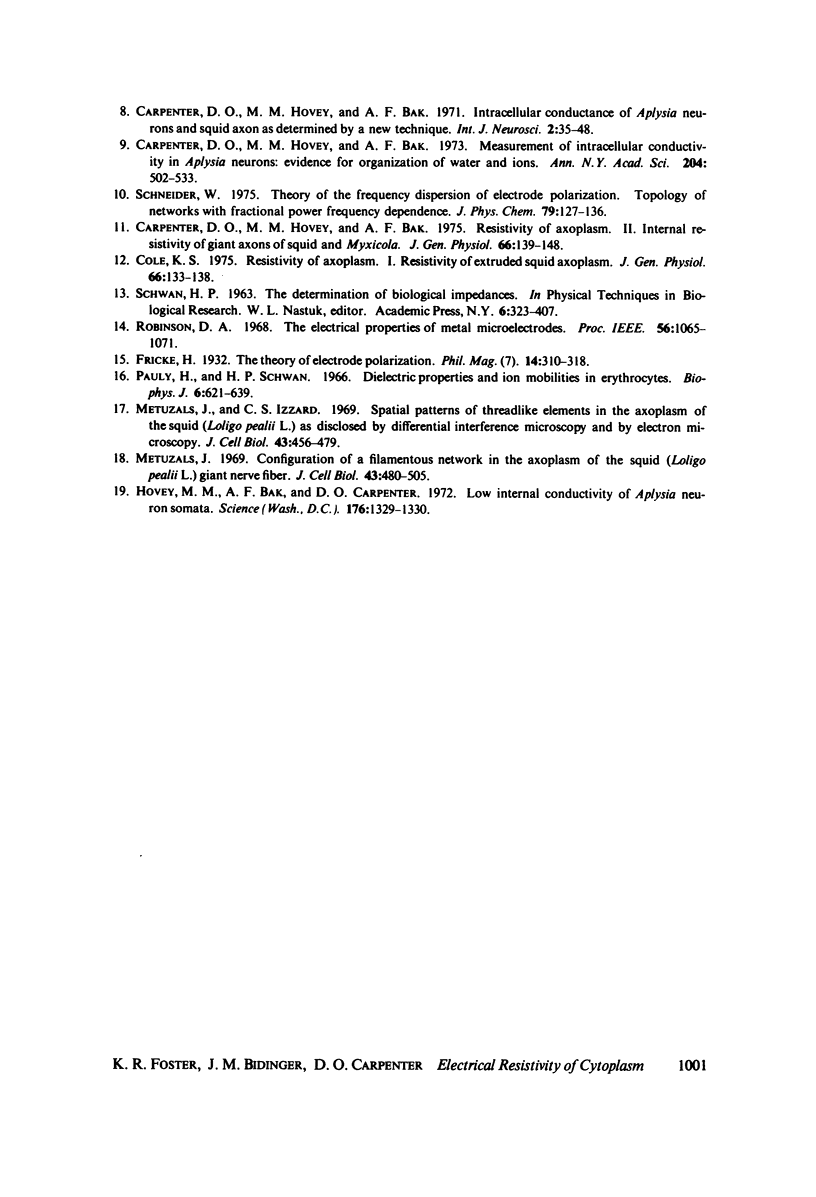
Selected References
These references are in PubMed. This may not be the complete list of references from this article.
- Carpenter D. O., Hovey M. M., Bak A. F. Intracellular conductance of Aplysia neurons and squid axon as determined by a new technique. Int J Neurosci. 1971 Jul;2(1):35–48. doi: 10.3109/00207457109146991. [DOI] [PubMed] [Google Scholar]
- Carpenter D. O., Hovey M. M., Bak A. F. Measurements of intracellular conductivity in Aplysia neurons: evidence for organization of water and ions. Ann N Y Acad Sci. 1973 Mar 30;204:502–533. doi: 10.1111/j.1749-6632.1973.tb30801.x. [DOI] [PubMed] [Google Scholar]
- Carpenter D. O., Hovey M. M., Bak A. F. Resistivity of axoplasm. II. Internal resistivity of giant axons of squid and Myxicola. J Gen Physiol. 1975 Aug;66(2):139–148. doi: 10.1085/jgp.66.2.139. [DOI] [PMC free article] [PubMed] [Google Scholar]
- Cole K. S., Hodgkin A. L. MEMBRANE AND PROTOPLASM RESISTANCE IN THE SQUID GIANT AXON. J Gen Physiol. 1939 May 20;22(5):671–687. doi: 10.1085/jgp.22.5.671. [DOI] [PMC free article] [PubMed] [Google Scholar]
- Cole K. S. Resistivity of axoplasm. I. Resistivity of extruded squid axoplasm. J Gen Physiol. 1975 Aug;66(2):133–138. doi: 10.1085/jgp.66.2.133. [DOI] [PMC free article] [PubMed] [Google Scholar]
- FALK G., FATT P. LINEAR ELECTRICAL PROPERTIES OF STRIATED MUSCLE FIBRES OBSERVED WITH INTRACELLULAR ELECTRODES. Proc R Soc Lond B Biol Sci. 1964 Apr 14;160:69–123. doi: 10.1098/rspb.1964.0030. [DOI] [PubMed] [Google Scholar]
- FATT P., KATZ B. The electrical properties of crustacean muscle fibres. J Physiol. 1953 Apr 28;120(1-2):171–204. doi: 10.1113/jphysiol.1953.sp004884. [DOI] [PMC free article] [PubMed] [Google Scholar]
- Hodgkin A. L., Nakajima S. The effect of diameter on the electrical constants of frog skeletal muscle fibres. J Physiol. 1972 Feb;221(1):105–120. doi: 10.1113/jphysiol.1972.sp009742. [DOI] [PMC free article] [PubMed] [Google Scholar]
- Hovey M. M., Bak A. F., Carpenter D. O. Low internal conductivity of Aplysia neuron somata. Science. 1972 Jun 23;176(4041):1329–1331. doi: 10.1126/science.176.4041.1329. [DOI] [PubMed] [Google Scholar]
- Metuzals J. Configuration of a filamentous network in the axoplasm of the squid (Loligo pealii L.) giant nerve fiber. J Cell Biol. 1969 Dec;43(3):480–505. doi: 10.1083/jcb.43.3.480. [DOI] [PMC free article] [PubMed] [Google Scholar]
- Metuzals J., Izzard C. S. Spatial patterns of threadlike elements in the axoplasm of the giant nerve fiber of the squid (Loligo pealii L.) as disclosed by differential interference microscopy and by electron microscopy. J Cell Biol. 1969 Dec;43(3):456–479. doi: 10.1083/jcb.43.3.456. [DOI] [PMC free article] [PubMed] [Google Scholar]
- Pauly H., Schwan H. P. Dielectric properties and ion mobility in erythrocytes. Biophys J. 1966 Sep;6(5):621–639. doi: 10.1016/S0006-3495(66)86682-1. [DOI] [PMC free article] [PubMed] [Google Scholar]