Abstract
1. Glycerol-extracted flight muscle from the giant water-bug, Lethocerus cordofanus, undergoes contraction when deprived of ATP. This rigor contraction occurs in the presence of the calcium chelating agent EGTA, under conditions in which the predicted free calcium ion concentration is less than 5 × 10-9 M. A mechanically similar contraction has been observed by depriving the muscle of Mg2+, in the presence of 5 mM-ATP.
2. In the rigor contraction tensions of up to 120 mN/mm2 under isometric conditions, and shortenings of between 2½ and 6% under isotonic conditions, have been observed at 20° C.
3. Muscles in rigor can be relaxed by the addition of ATP. The minimum concentration of ATP required to give full relaxation depends upon other ionic constituents of the solutions, and upon temperature, but is between 0·3 and 1 mM at 20° C. Lower concentrations result in partial reduction of tension and stiffness.
4. Pyrophosphate (PP) causes a reduction in the tension of muscle which has developed rigor under isometric conditions, but the stiffness, when measured at frequencies between 1 and 100 Hz, remains indistinguishable from that of rigor muscle. The stiffness when measured by applying slow length changes is comparable to that of ATP-relaxed muscle. It is suggested that in PP-relaxed muscle the cross-bridges remain in close proximity to, but are not rigidly attached to, the I filaments, resulting in a high viscous interaction between the two sets of filaments.
5. The addition of low concentrations of ADP (less than about 0·7 mM) to rigor muscle in the absence of ATP causes an increase in tension. The effect is sigmoid, and is probably due to the formation of low concentrations of ATP throughout the body of the fibre by myokinase activity. Larger concentrations of ADP (about 5 mM) added to rigor muscle cause relaxation, probably due to the formation of higher ATP concentrations.
6. AMP and inorganic orthophosphate have little effect upon the mechanical properties of rigor muscle.
7. There is a delay of about 1 min before the onset of rigor contraction when fibres are transferred from an ATP-solution to one containing no ATP, due to the transfer of ATP in the body of the fibres. Both ATP hydrolysis by the fibres and diffusion of ATP into the bathing solution contribute significantly to the rate of depletion of ATP.
8. Rabbit psoas muscle shows a similar rigor contraction in the presence of EGTA, and has mechanical properties similar to those described for Lethocerus flight muscle in the presence of pyrophosphate.
Full text
PDF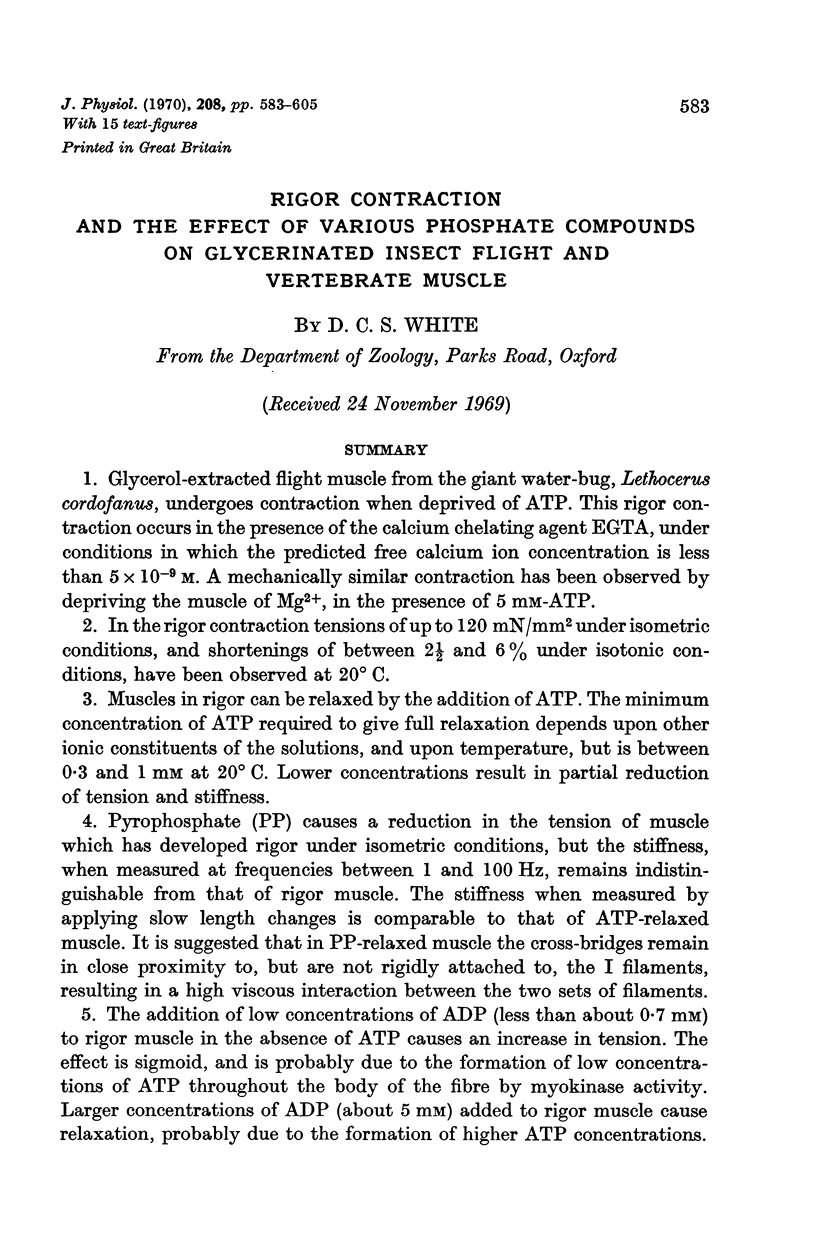
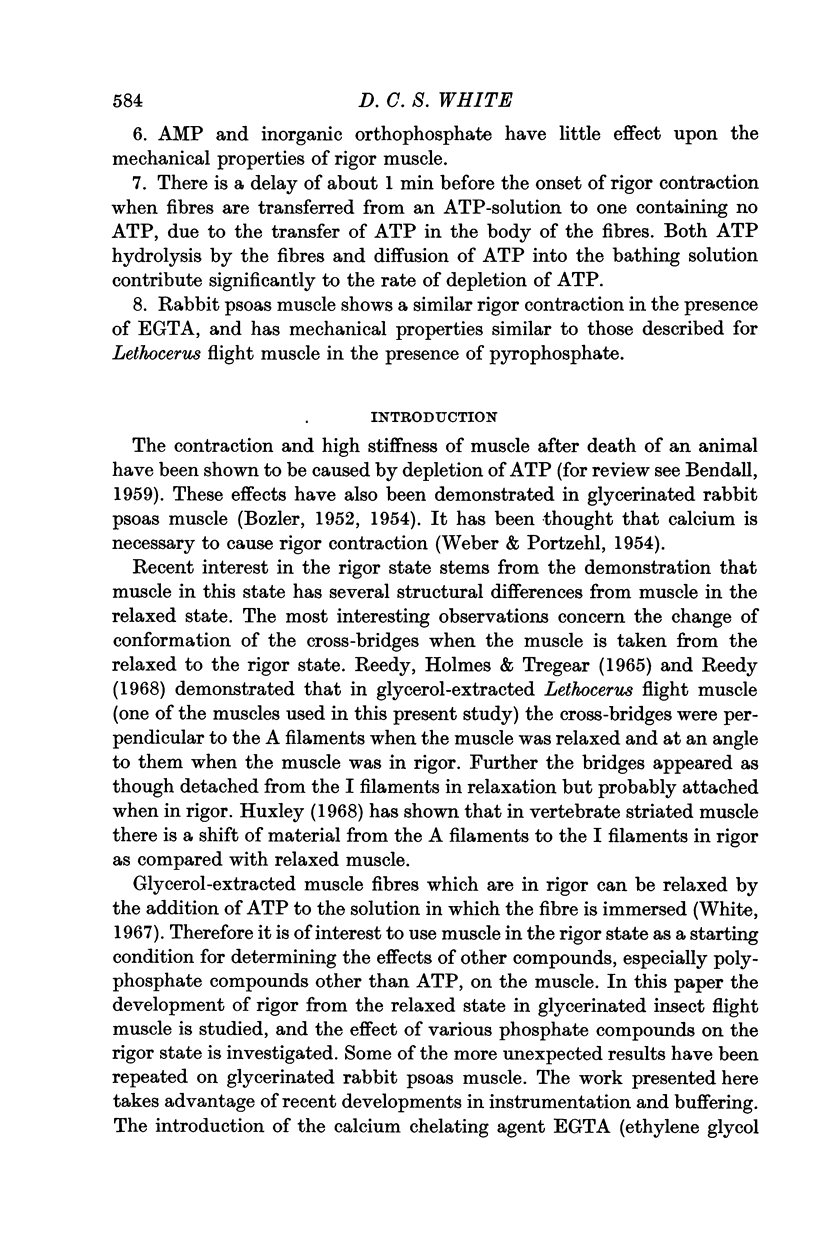
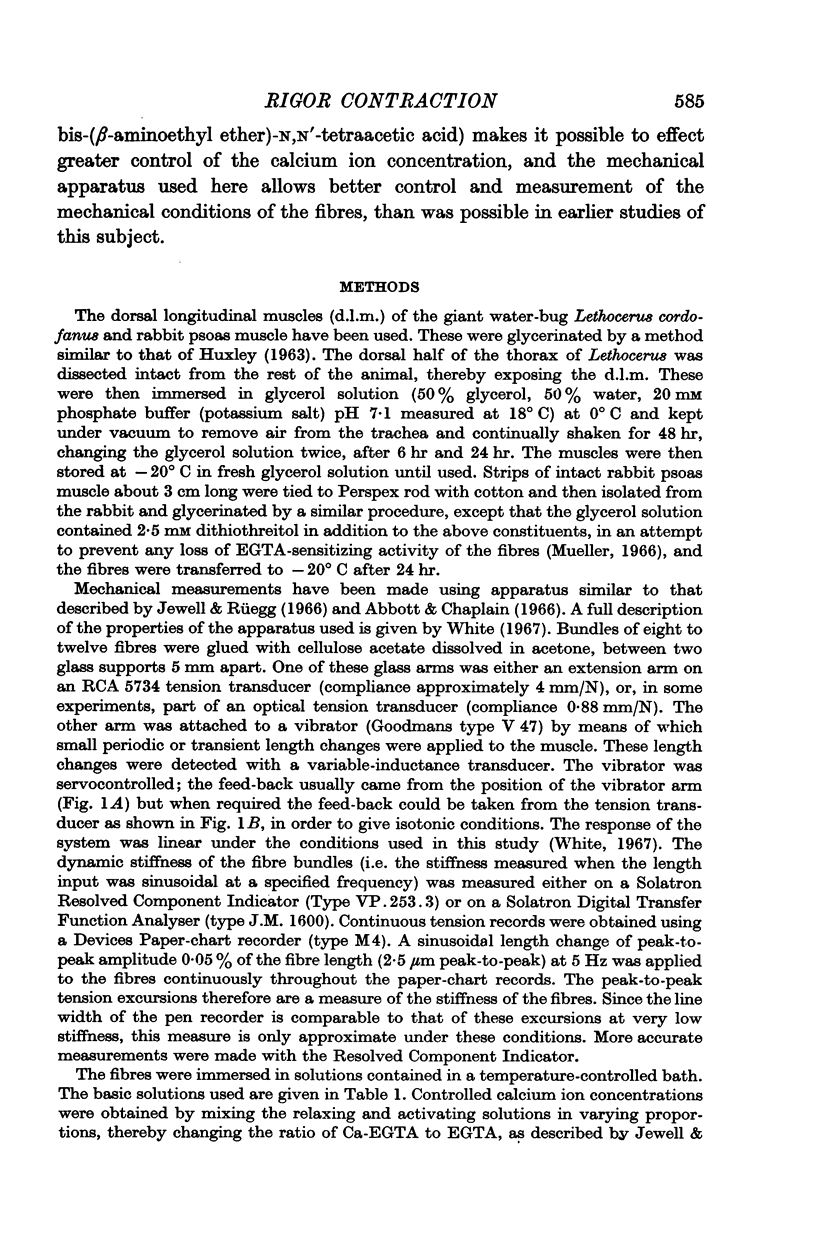
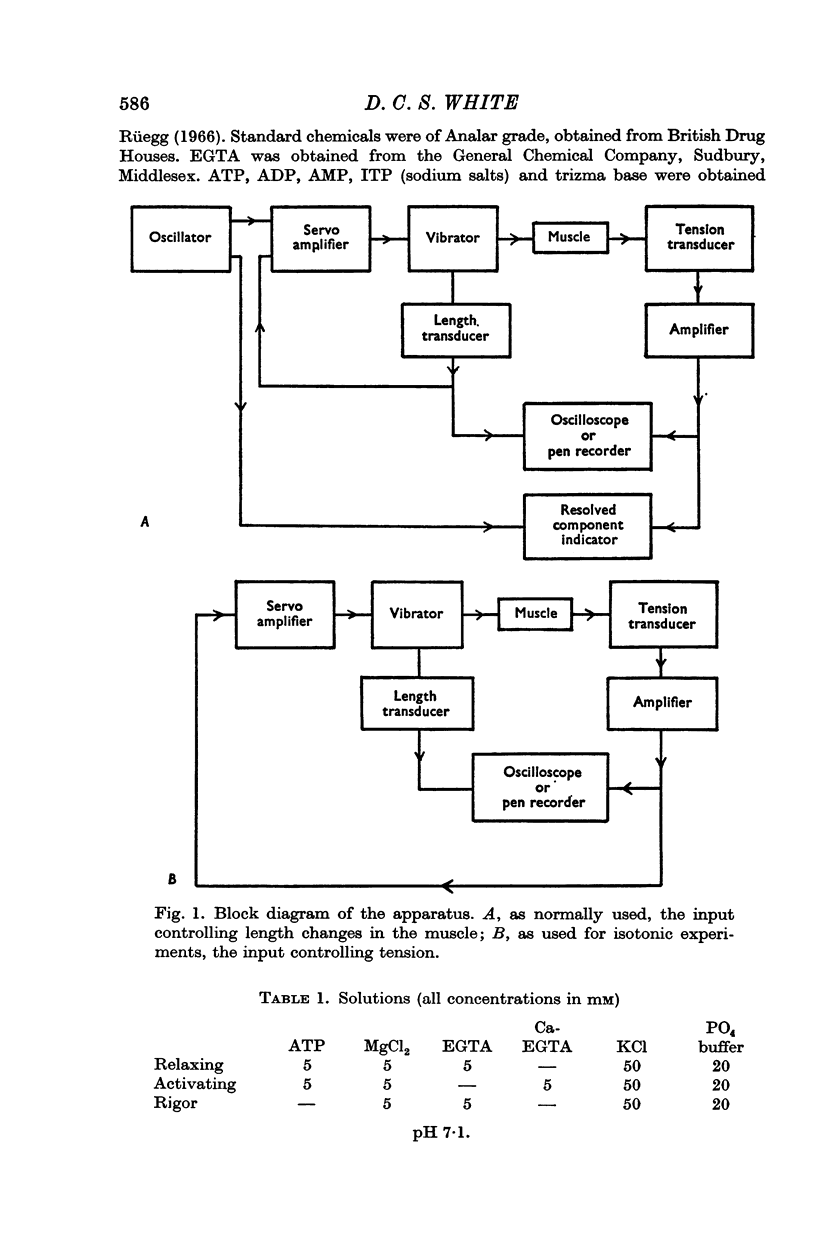
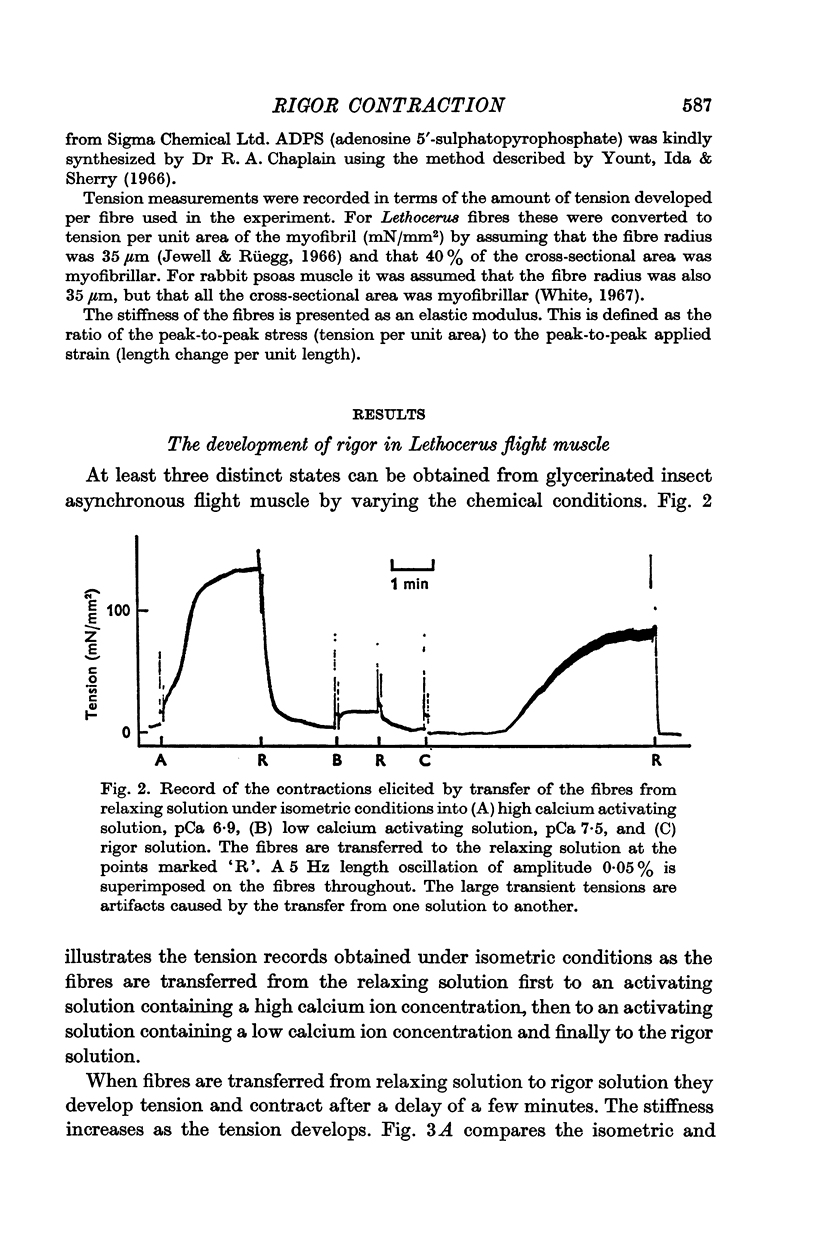
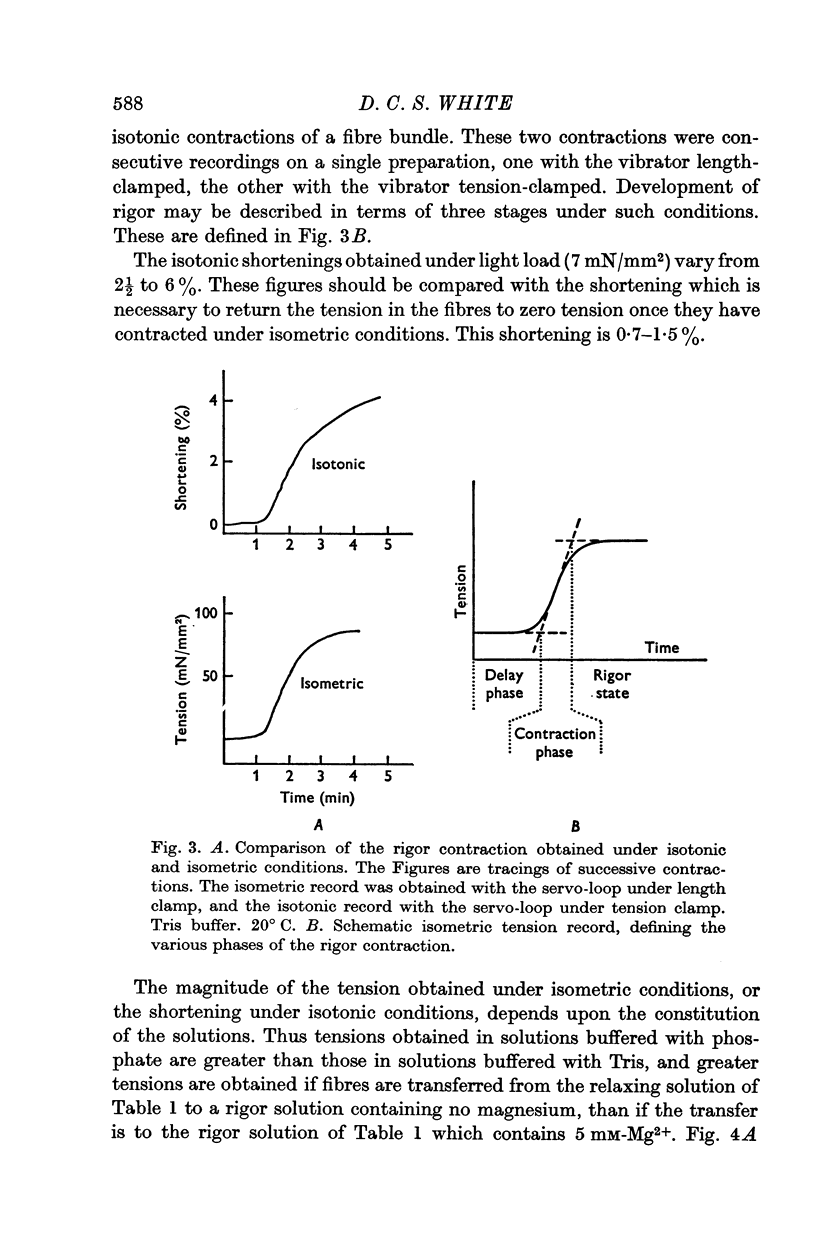
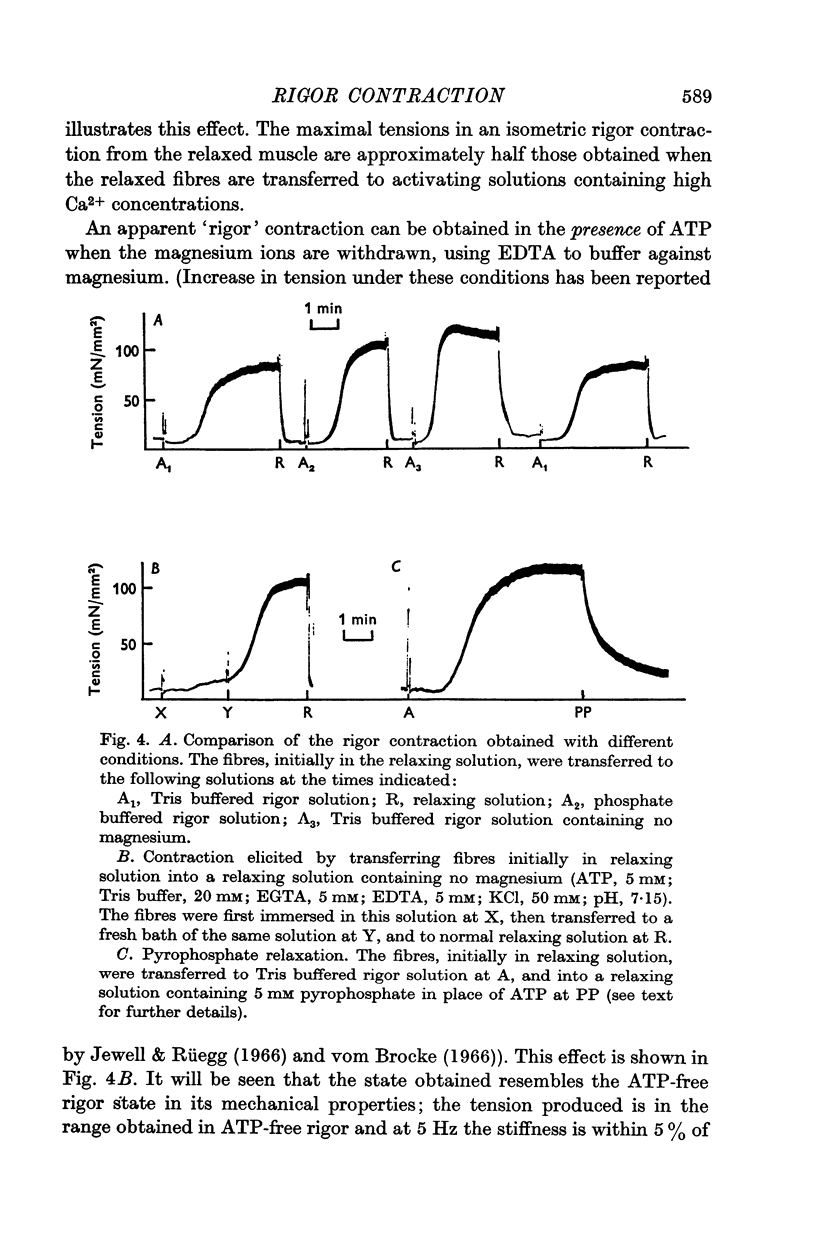
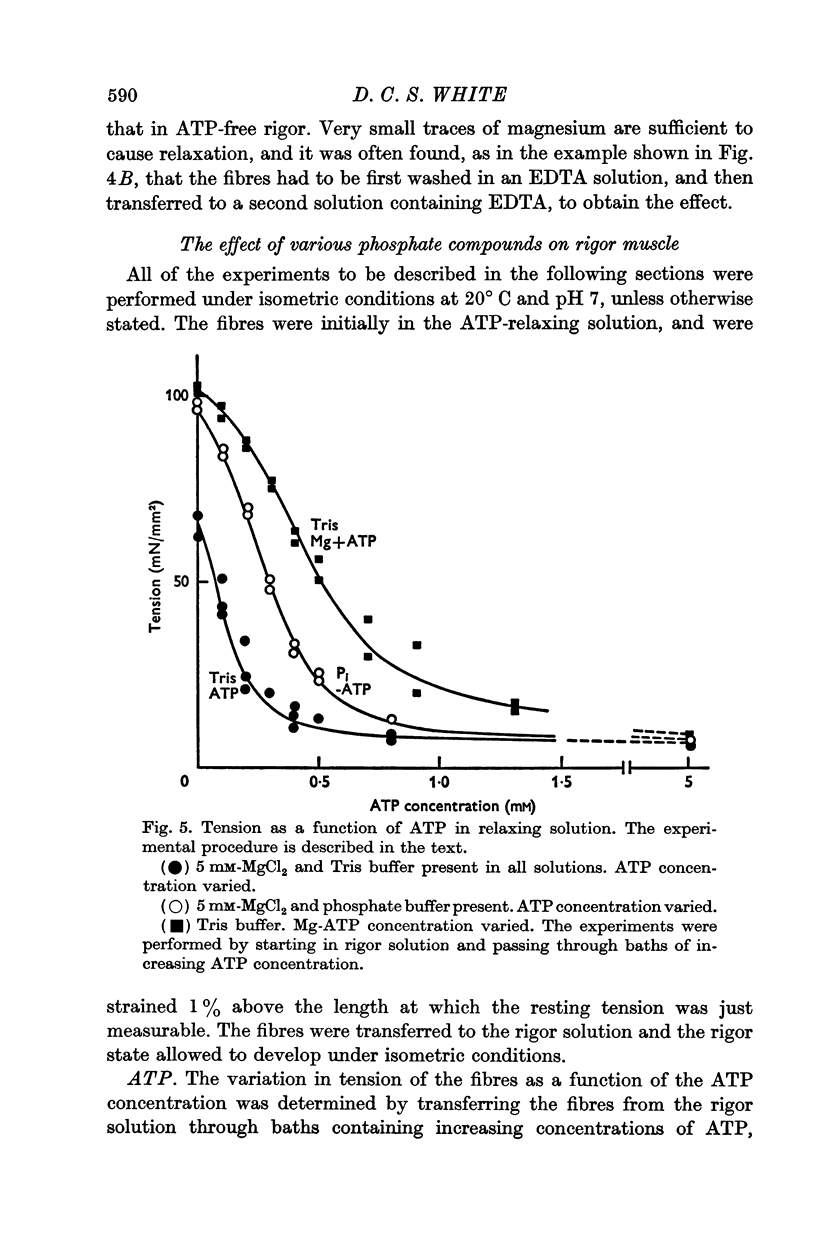
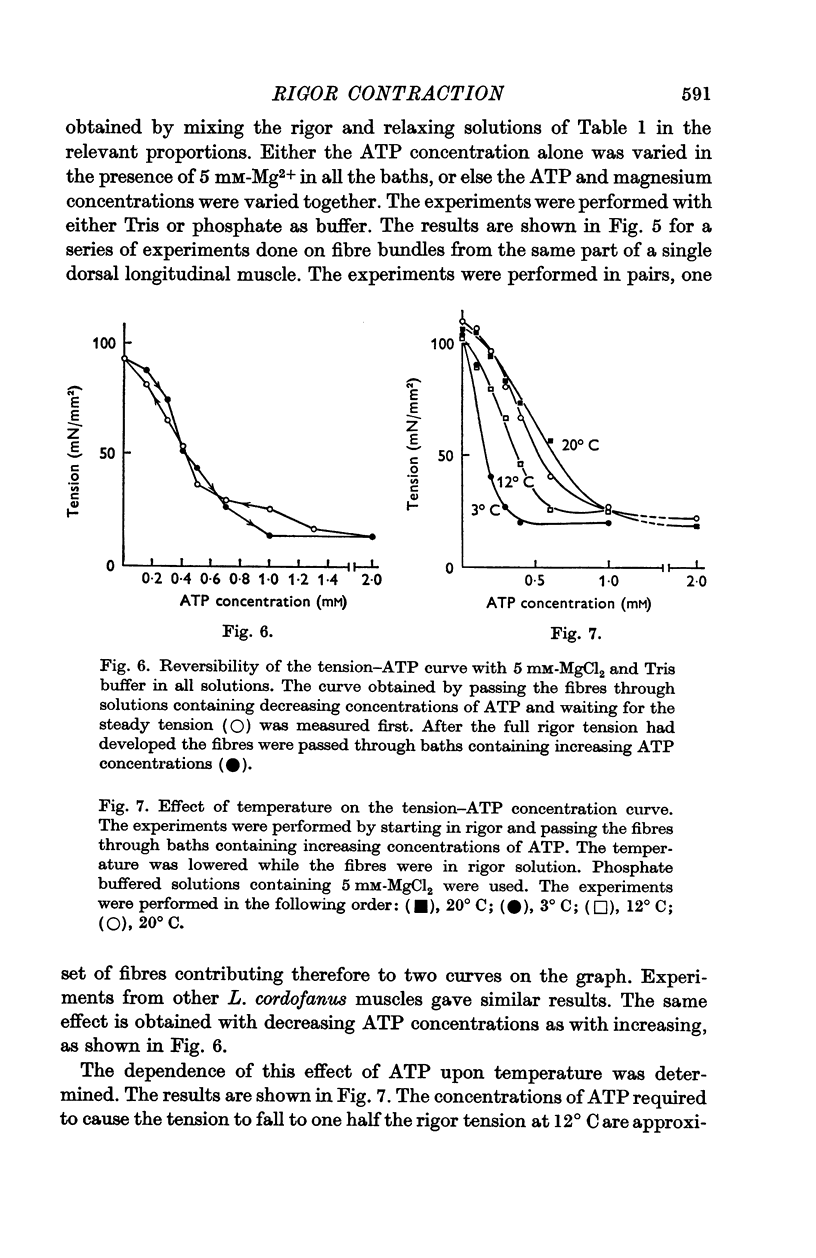
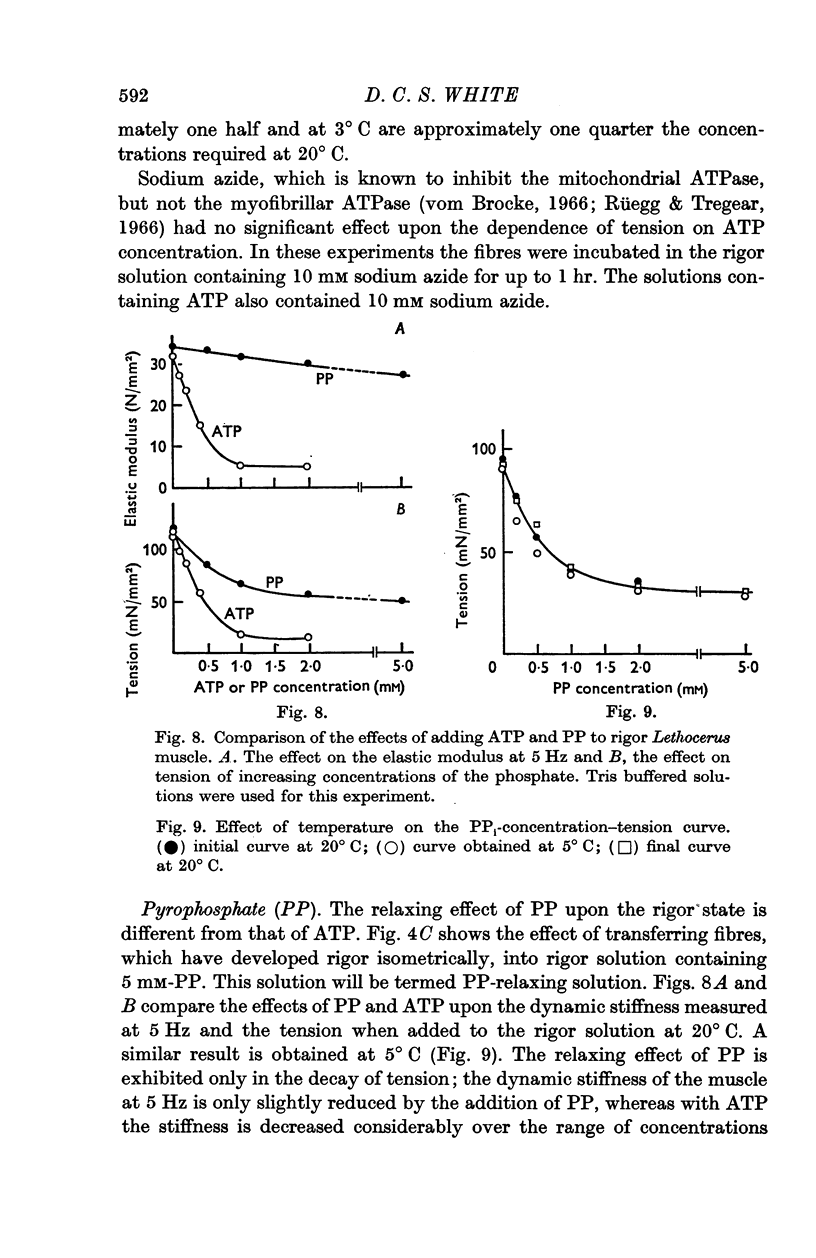
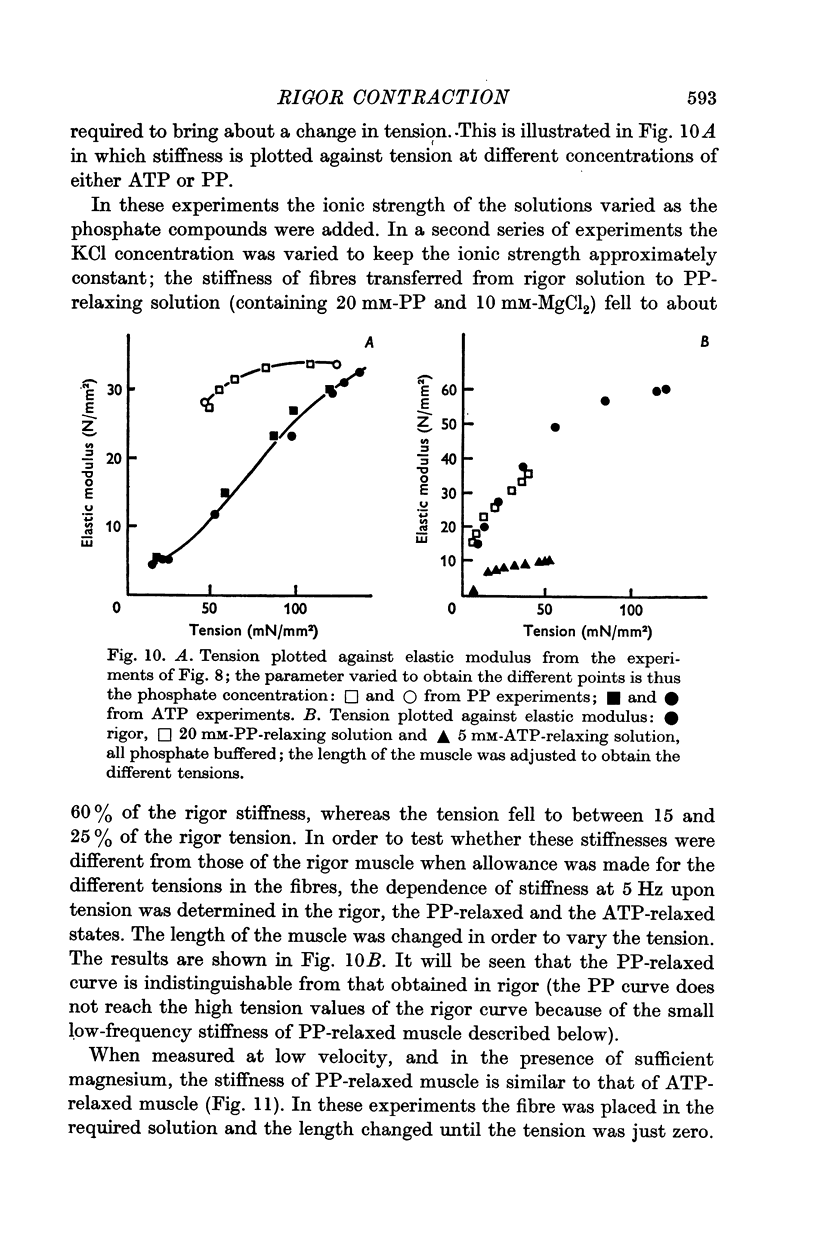
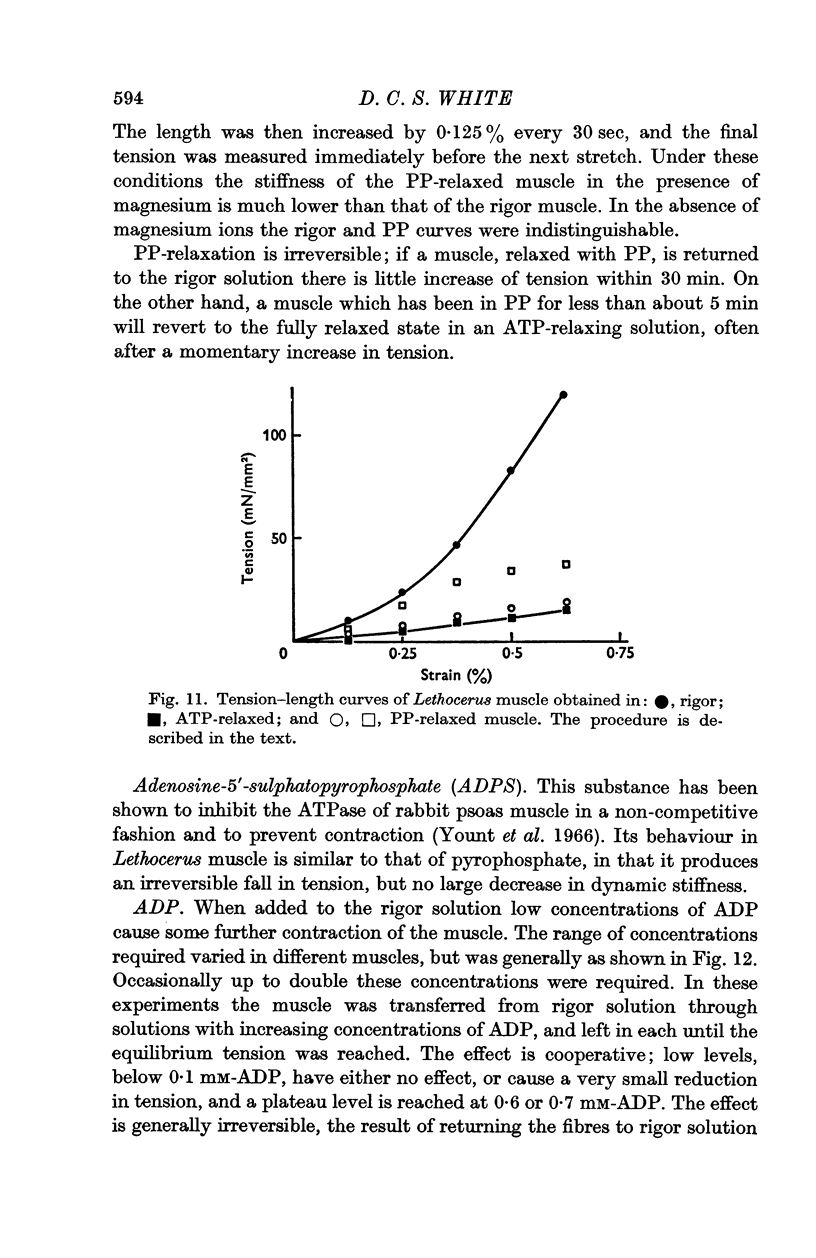
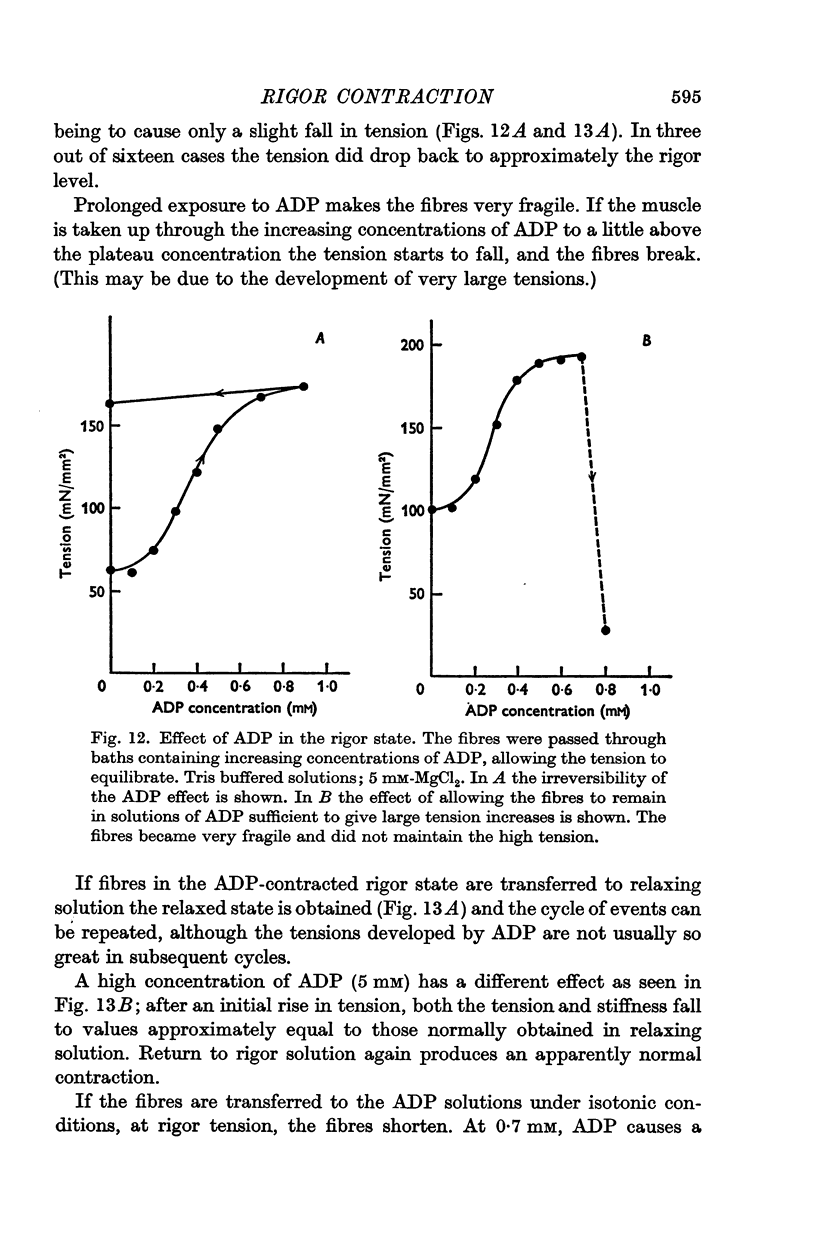
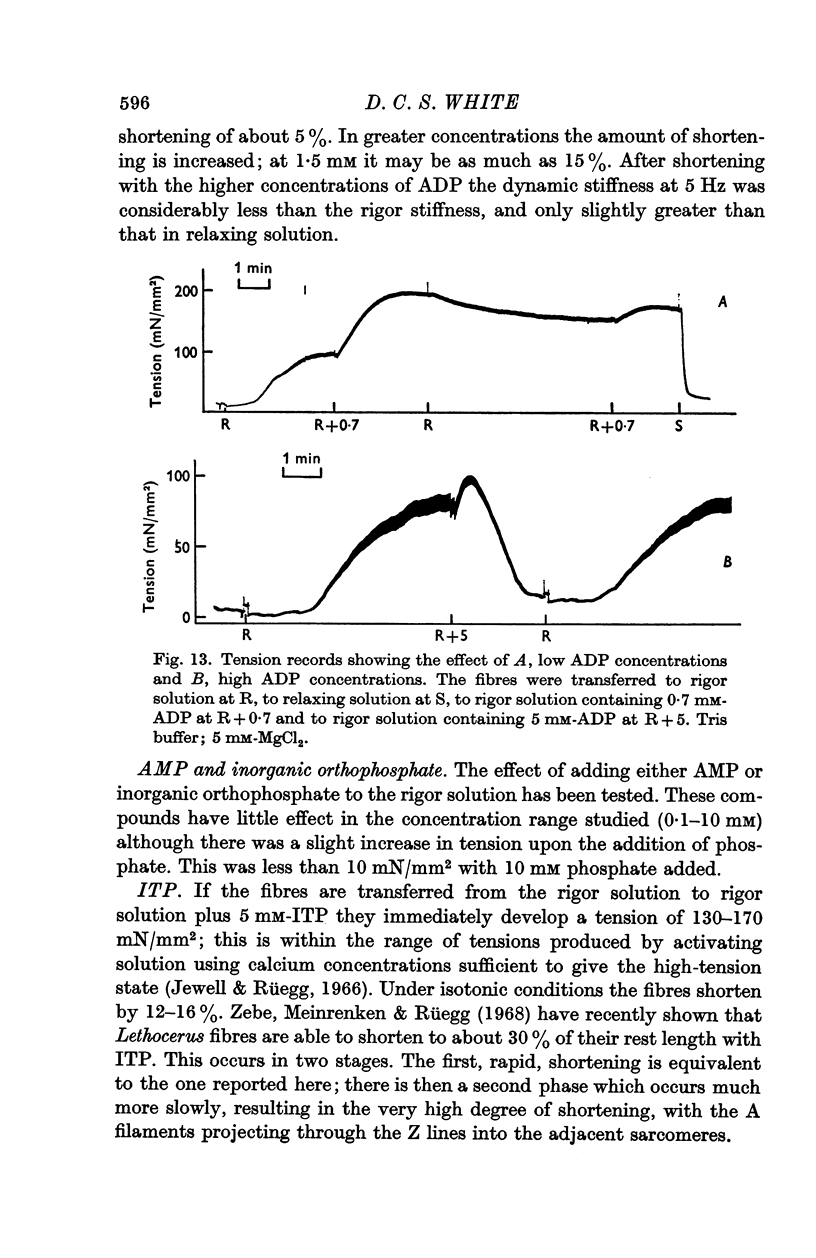
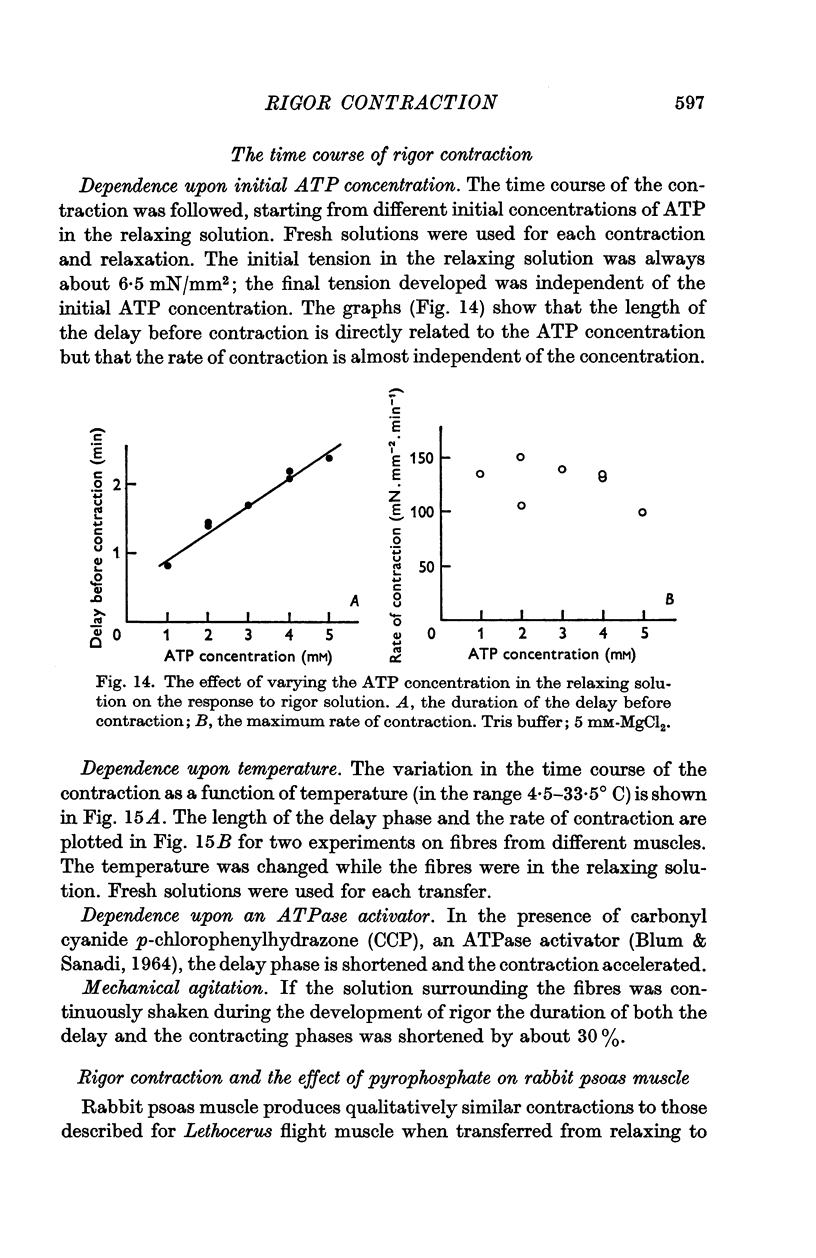
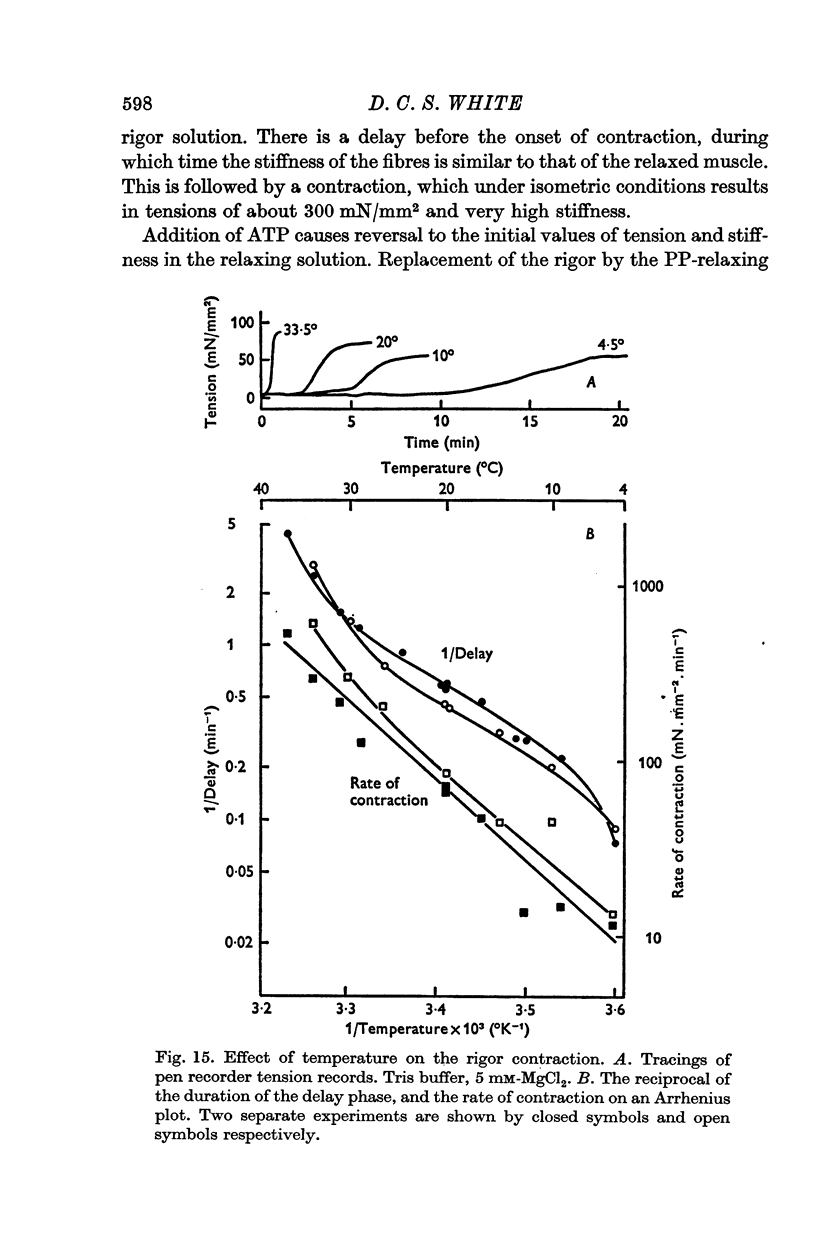
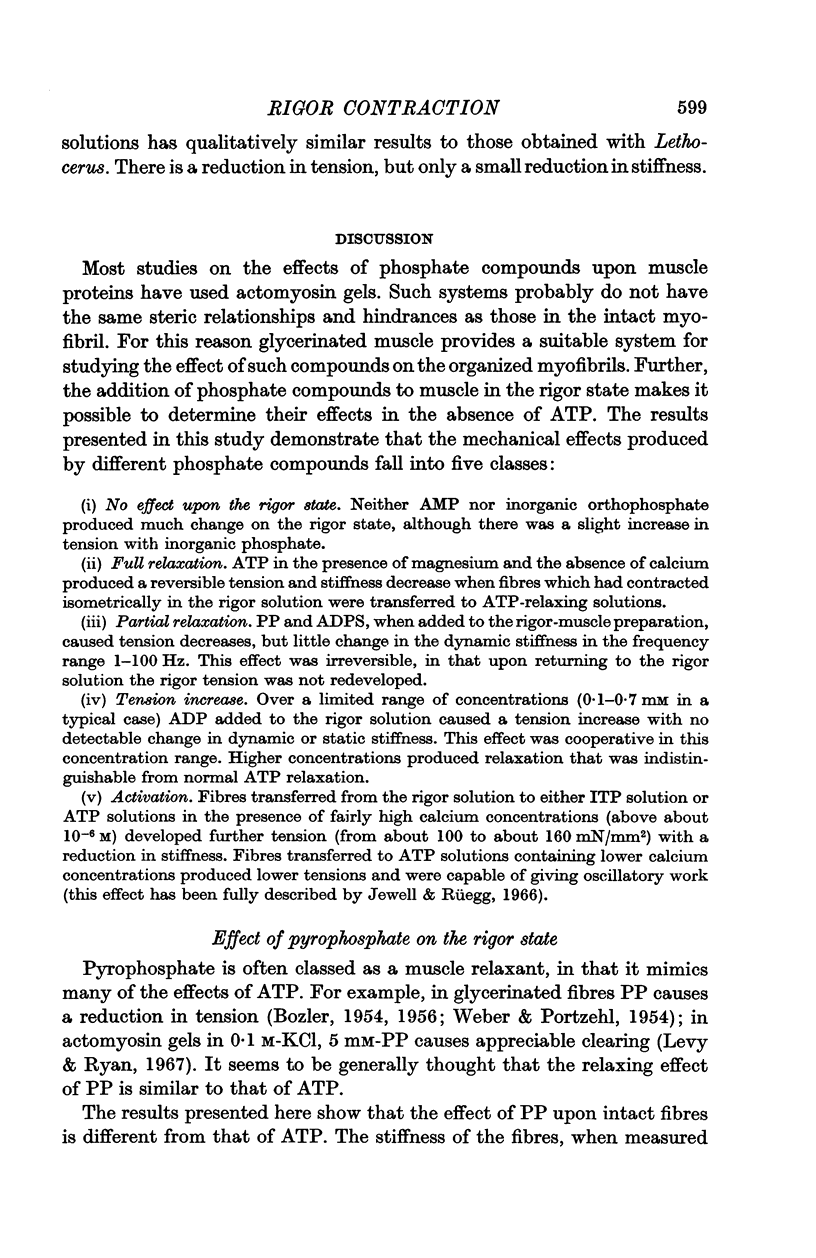
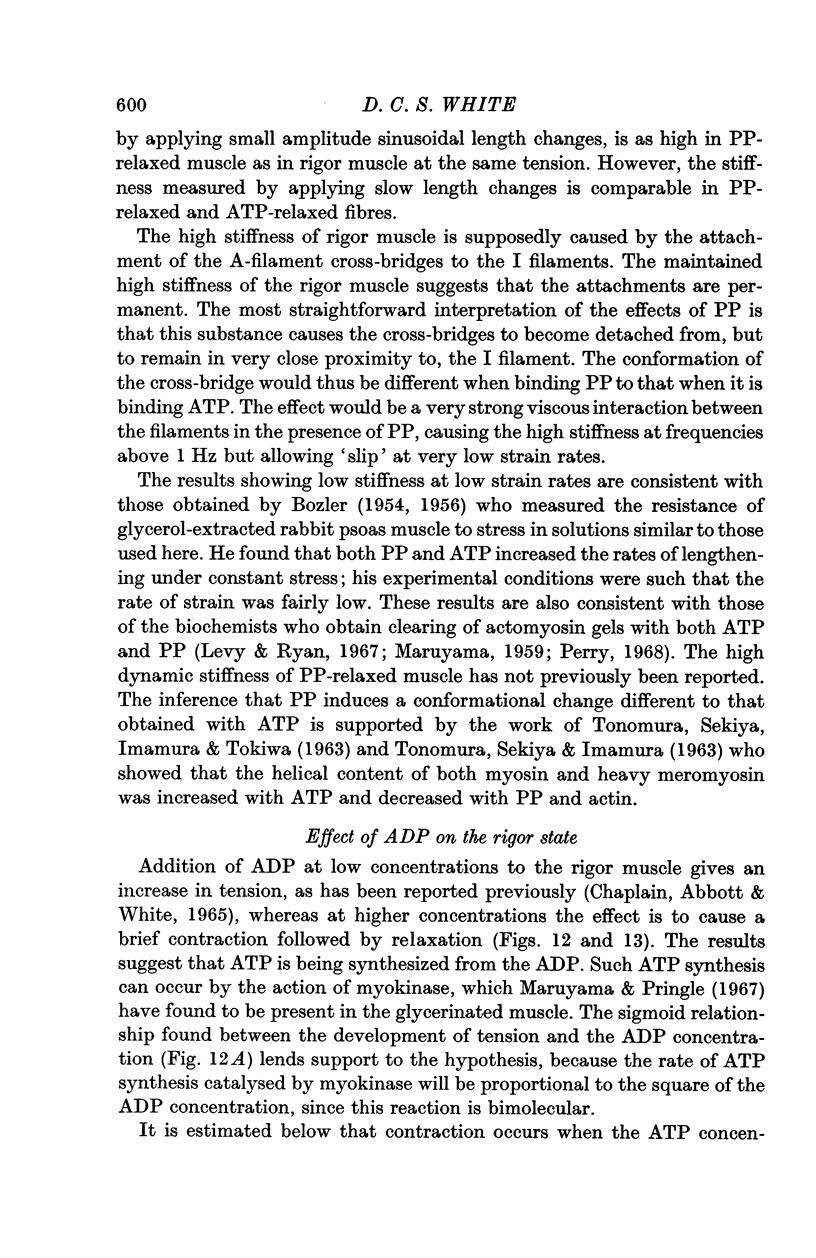
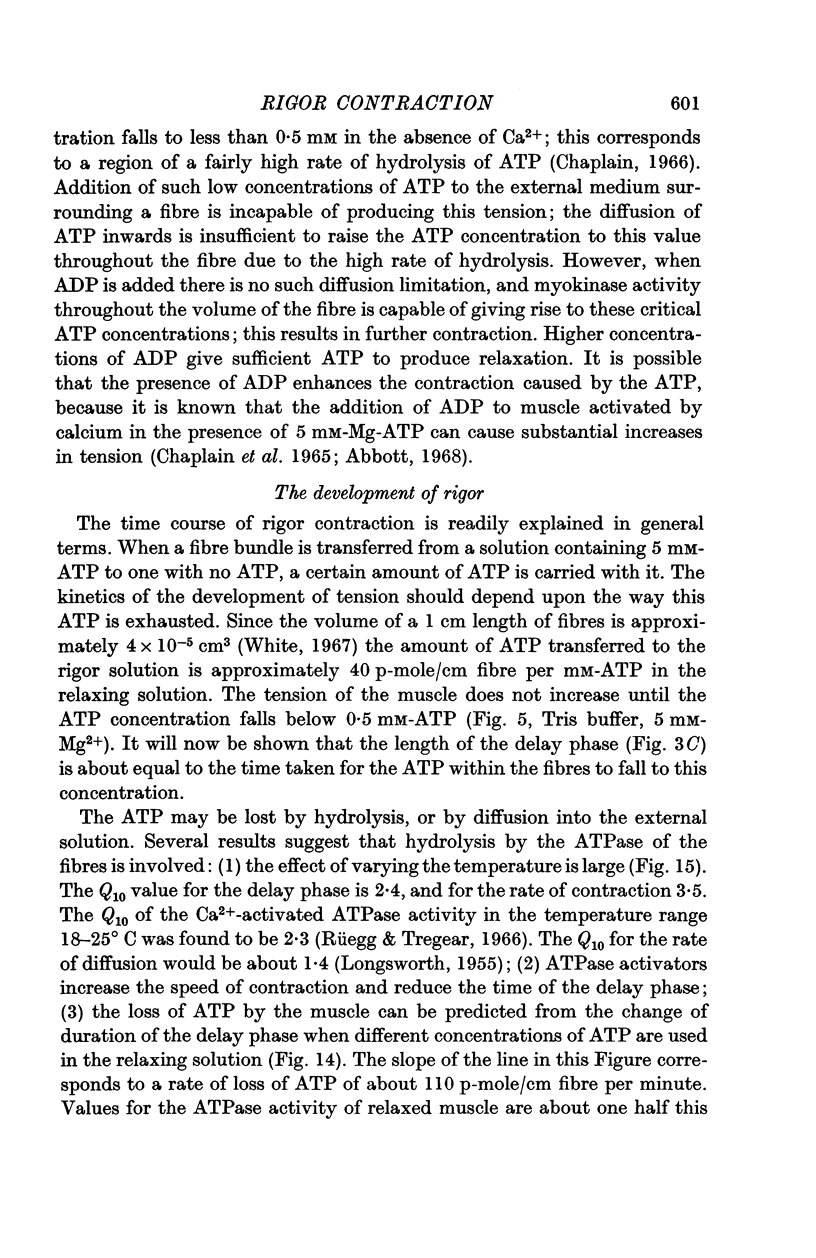
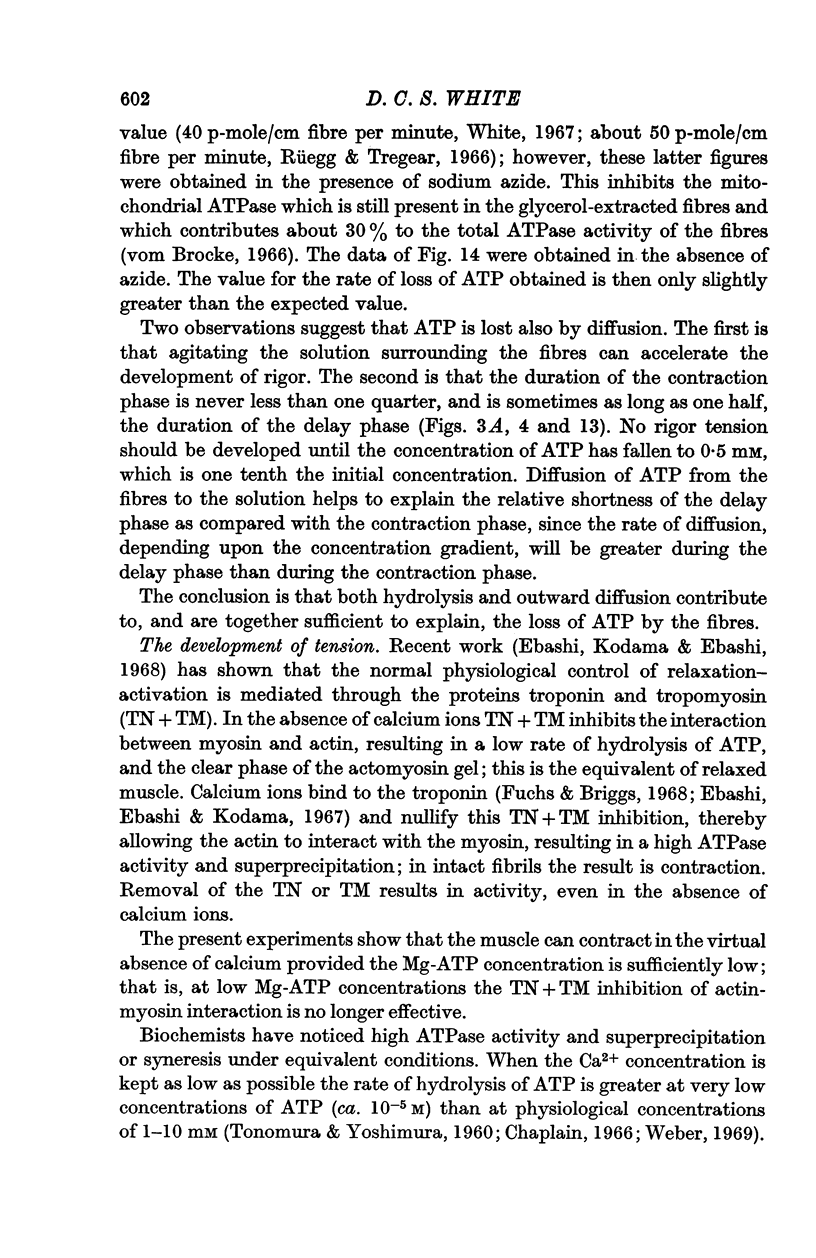
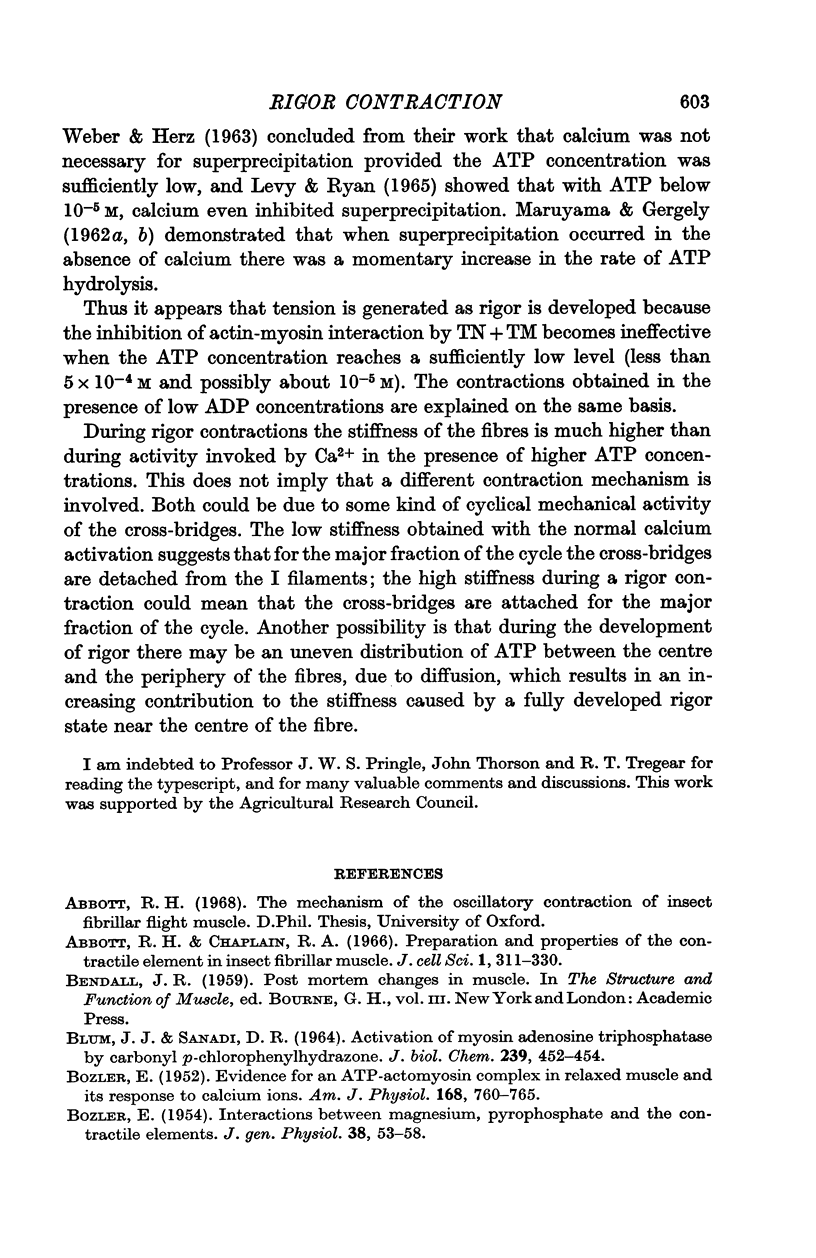
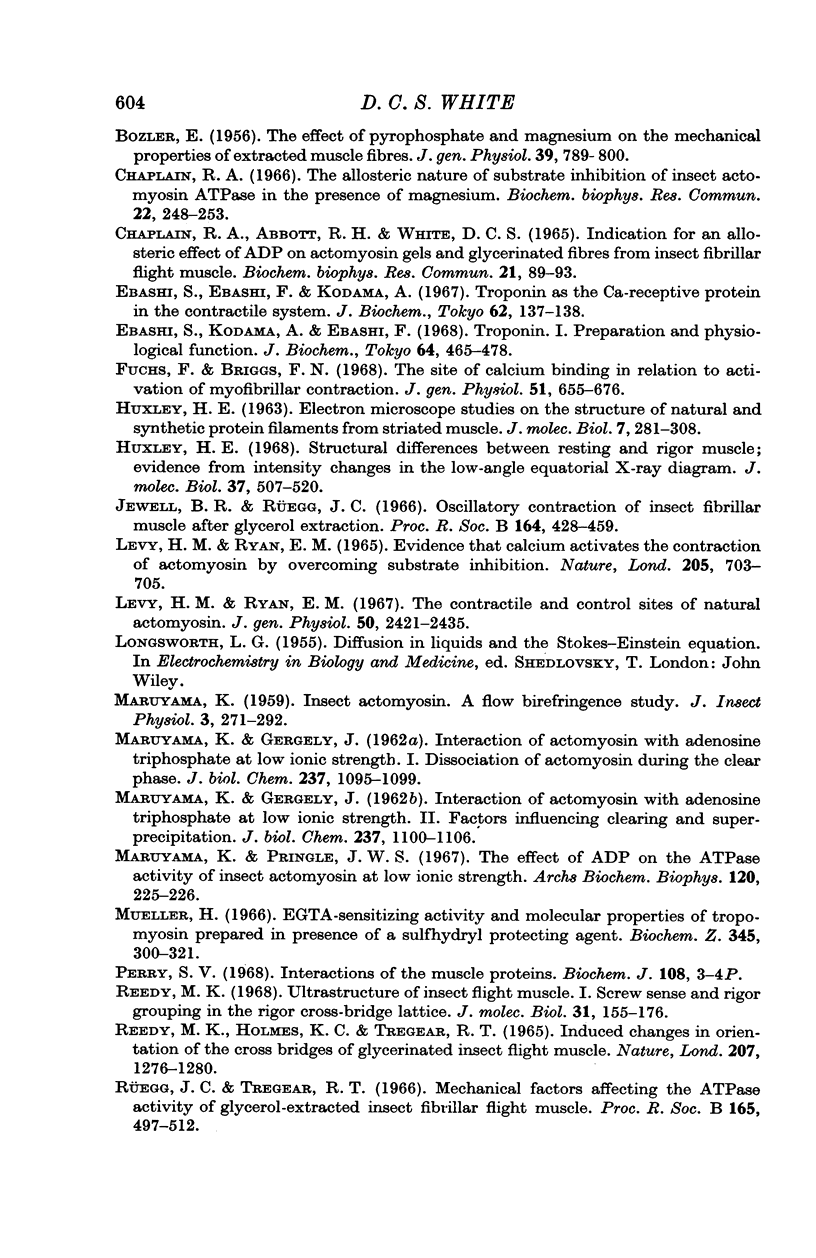
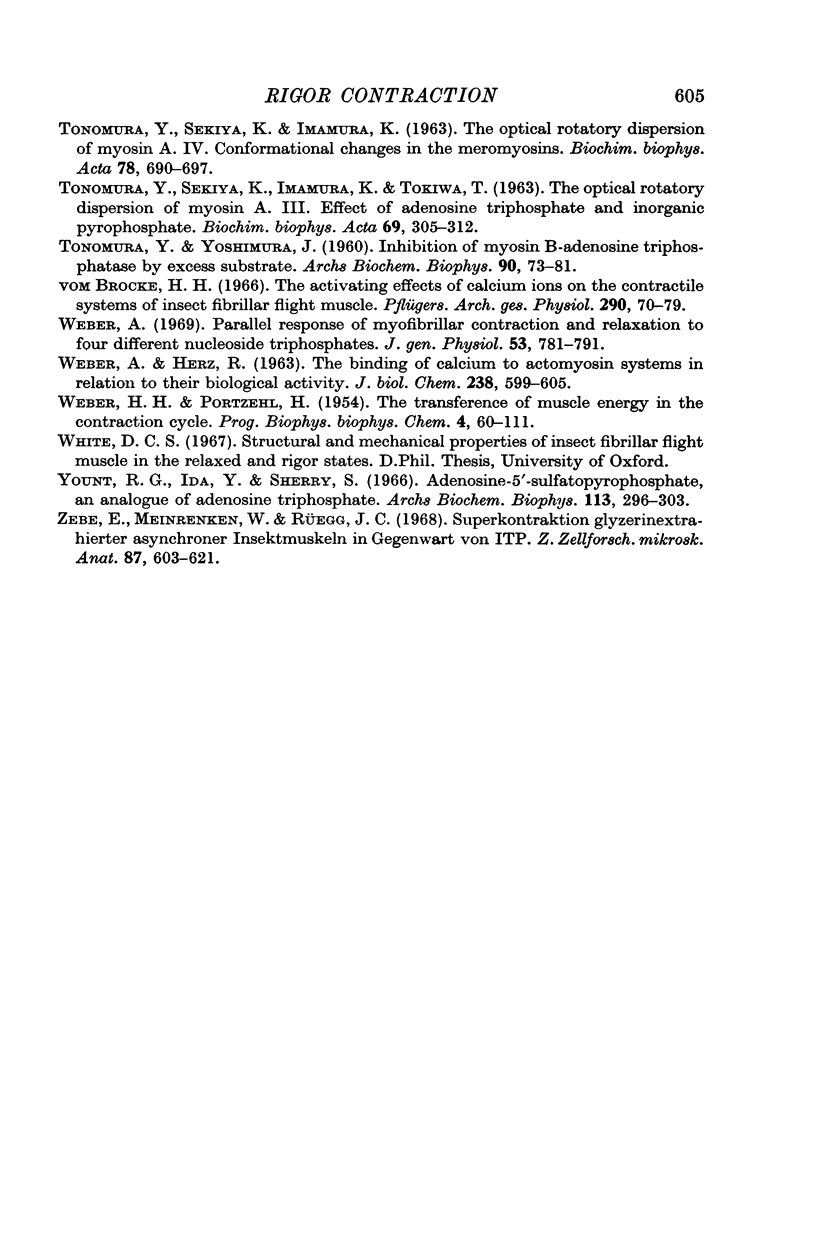
Selected References
These references are in PubMed. This may not be the complete list of references from this article.
- Abbott R. H., Chaplain R. A. Preparation and properties of the contractile element of insect fibrillar muscle. J Cell Sci. 1966 Sep;1(3):311–330. doi: 10.1242/jcs.1.3.311. [DOI] [PubMed] [Google Scholar]
- BLUM J. J., SANADI D. R. ACTIVATION OF MYOSIN ADENOSINE TRIPHOSPHATASE BY CARBONYL CYANIDE P-CHLOROPHENYLHYDRAZONE. J Biol Chem. 1964 Feb;239:452–454. [PubMed] [Google Scholar]
- BOZLER E. Evidence of an ATP-actomyosin complex in relaxed muscle and its response to calcium ions. Am J Physiol. 1952 Mar;168(3):760–765. doi: 10.1152/ajplegacy.1952.168.3.760. [DOI] [PubMed] [Google Scholar]
- BOZLER E. Interactions between magnesium, pyrophosphate, and the contractile elements. J Gen Physiol. 1954 Sep 20;38(1):53–58. doi: 10.1085/jgp.38.1.53. [DOI] [PMC free article] [PubMed] [Google Scholar]
- BOZLER E. The effect of polyphosphates and magnesium on the mechanical properties of extracted muscle fibers. J Gen Physiol. 1956 May 20;39(5):789–800. doi: 10.1085/jgp.39.5.789. [DOI] [PMC free article] [PubMed] [Google Scholar]
- Brocke HH vom The activating effects of calcium ions on the contractile systems of insect fibrillar flight muscle. Pflugers Arch Gesamte Physiol Menschen Tiere. 1966;290(1):70–79. doi: 10.1007/BF00362620. [DOI] [PubMed] [Google Scholar]
- Chaplain R. A., Abbott R. H., White D. C. Indication for an allosteric effect of ADP on actomyosin gels and glycerinated fibres from insect fibrillar flight muscle. Biochem Biophys Res Commun. 1965 Oct 26;21(2):89–93. doi: 10.1016/0006-291x(65)90091-4. [DOI] [PubMed] [Google Scholar]
- Ebashi S., Ebashi F., Kodama A. Troponin as the Ca++-receptive protein in the contractile system. J Biochem. 1967 Jul;62(1):137–138. doi: 10.1093/oxfordjournals.jbchem.a128628. [DOI] [PubMed] [Google Scholar]
- Ebashi S., Kodama A., Ebashi F. Troponin. I. Preparation and physiological function. J Biochem. 1968 Oct;64(4):465–477. doi: 10.1093/oxfordjournals.jbchem.a128918. [DOI] [PubMed] [Google Scholar]
- Fuchs F., Briggs F. N. The site of calcium binding in relation to the activation of myofibrillar contraction. J Gen Physiol. 1968 May;51(5):655–676. doi: 10.1085/jgp.51.5.655. [DOI] [PMC free article] [PubMed] [Google Scholar]
- Huxley H. E. Structural difference between resting and rigor muscle; evidence from intensity changes in the lowangle equatorial x-ray diagram. J Mol Biol. 1968 Nov 14;37(3):507–520. doi: 10.1016/0022-2836(68)90118-6. [DOI] [PubMed] [Google Scholar]
- LEVY H. M., RYAN E. M. EVIDENCE THAT CALCIUM ACTIVATES THE CONTRACTION OF ACTOMYOSIN BY OVERCOMING SUBSTRATE INHIBITION. Nature. 1965 Feb 13;205:703–705. doi: 10.1038/205703b0. [DOI] [PubMed] [Google Scholar]
- Levy H. M., Ryan E. M. The contractile and control sites of natural actomyosin. J Gen Physiol. 1967 Nov;50(10):2421–2435. doi: 10.1085/jgp.50.10.2421. [DOI] [PMC free article] [PubMed] [Google Scholar]
- MARUYAMA K., GERGELY J. Interaction of actomyosin with adenosine triphosphate at low ionic strength. I. Dis-sociation of actomyosin during the clear phase. J Biol Chem. 1962 Apr;237:1095–1099. [PubMed] [Google Scholar]
- MARUYAMA K., GERGELY J. Interaction of actomyosin with adenosine triphosphate at low ionic strength. II. Factors influencing clearing and superprecipitation: adenosine triphosphatase and birefringence of flow studies. J Biol Chem. 1962 Apr;237:1100–1106. [PubMed] [Google Scholar]
- Maruyama K., Pringle J. W. The effect of ADP on the ATPase activity of insect actomyosin at low ionic strength. Arch Biochem Biophys. 1967 Apr;120(1):225–227. doi: 10.1016/0003-9861(67)90619-4. [DOI] [PubMed] [Google Scholar]
- Reedy M. K., Holmes K. C., Tregear R. T. Induced changes in orientation of the cross-bridges of glycerinated insect flight muscle. Nature. 1965 Sep 18;207(5003):1276–1280. doi: 10.1038/2071276a0. [DOI] [PubMed] [Google Scholar]
- Reedy M. K. Ultrastructure of insect flight muscle. I. Screw sense and structural grouping in the rigor cross-bridge lattice. J Mol Biol. 1968 Jan 28;31(2):155–176. doi: 10.1016/0022-2836(68)90437-3. [DOI] [PubMed] [Google Scholar]
- Rüegg J. C., Tregear R. T. Mechanical factors affecting the ATPase activity of glycerol-extracted insect fibrillar flight muscle. Proc R Soc Lond B Biol Sci. 1966 Oct 11;165(1001):497–512. doi: 10.1098/rspb.1966.0080. [DOI] [PubMed] [Google Scholar]
- TONOMURA Y., SEKIYA K., IMAMURA K. THE OPTICAL ROTATORY DISPERSION OF MYOSIN A. IV. CONFORMATIONAL CHANGES IN MEROMYOSINS. Biochim Biophys Acta. 1963 Dec 13;78:690–697. doi: 10.1016/0006-3002(63)91034-5. [DOI] [PubMed] [Google Scholar]
- TONOMURA Y., SEKIYA K., IMAMURA K., TOKIWA T. The optical-rotatory dispersion of myosin A. III. Effect of adenosine triphosphate and inorganic pyrophosphate. Biochim Biophys Acta. 1963 Feb 5;69:305–312. doi: 10.1016/0006-3002(63)91263-0. [DOI] [PubMed] [Google Scholar]
- TONOMURA Y., YOSHIMURA J. Inhibition of myosin B-adenosinetriphosphatase by excess substrate. Arch Biochem Biophys. 1960 Sep;90:73–81. doi: 10.1016/0003-9861(60)90614-7. [DOI] [PubMed] [Google Scholar]
- WEBER A., HERZ R. The binding of calcium to actomyosin systems in relation to their biological activity. J Biol Chem. 1963 Feb;238:599–605. [PubMed] [Google Scholar]
- Weber A. Parallel response of myofibrillar contraction and relaxation to four different nucleoside triphophates. J Gen Physiol. 1969 Jun;53(6):781–791. doi: 10.1085/jgp.53.6.781. [DOI] [PMC free article] [PubMed] [Google Scholar]
- Zebe E., Meinrenken W., Rüegg J. C. Superkontraktion glycerinextrahierter asynchroner Insektenmuskeln in Gegenwart von ITP. Z Zellforsch Mikrosk Anat. 1968 Apr 25;87(4):603–621. [PubMed] [Google Scholar]