Abstract
1. The influence of previous mechanical events on myocardial contractility has been investigated in the cat papillary muscle preparation.
2. When a muscle that had been producing a stable response under isometric conditions was allowed to shorten isotonically, its ability to do so increased in successive beats until it reached a steady level, which represented a potentiated state compared with that seen in the first isotonic beat and in the preceding stable isometric contractions.
3. The increase in tension development in the first isometric beat after a period of isotonic beating was used as an index of the degree of potentiation. It was found to be well correlated with the changes in other parameters that could have been used for this purpose.
4. The main determinants of the degree of potentiation produced by a period of isotonic beating were:
(a) the amount by which the muscle shortened. This was inversely related to the force opposing shortening (i.e. the isotonic load);
(b) the number of isotonic beats. There was some potentiation (about 10%) after a single isotonic beat, but the number of beats required for maximal potentiation (up to 25%) depended on the frequency of stimulation; about 8 beats were required at 24 min-1.
5. An isotonic release during the rise of tension in an isometric response was even more effective in potentiating the next isometric beat than an afterloaded contraction against the same load. Isotonic releases at later times had a diminishing influence on tension development in the next isometric beat.
6. In the absence of stimulation, the potentiated state produced by a period of isotonic beating decayed with a half-time of about 50 sec. When the muscle was stimulated it disappeared sooner, and its rate of decay depended on the frequency of stimulation; at 24 min-1 about 8 beats were required to restore contractility to its previous steady level.
7. The characteristics of the decay of the potentiated state were closely similar to those of the potentiated states that can be produced by various electrical interventions, and the possibility that all of these might have the same underlying mechanism is discussed.
8. Attention is drawn to the practical implications of this phenomenon in the design of experiments in which the muscle contracts under changing mechanical conditions.
Full text
PDF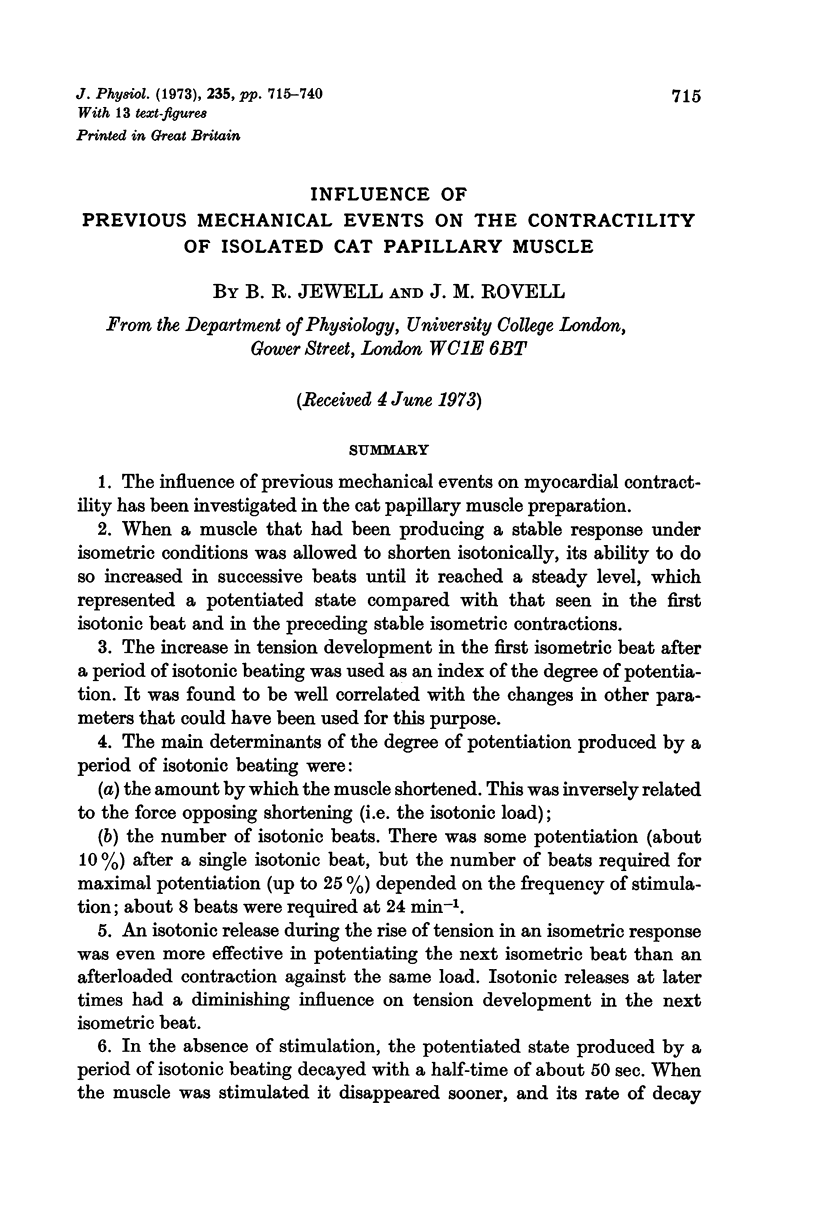
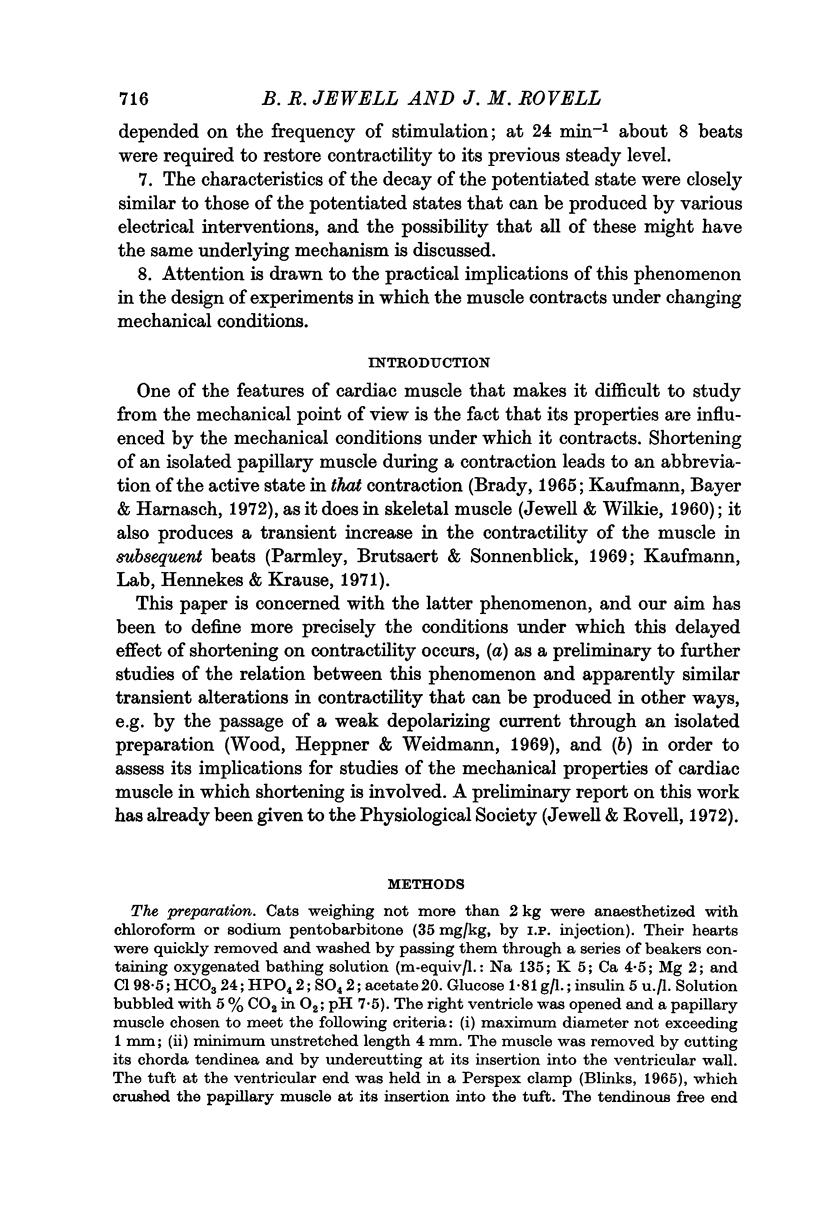
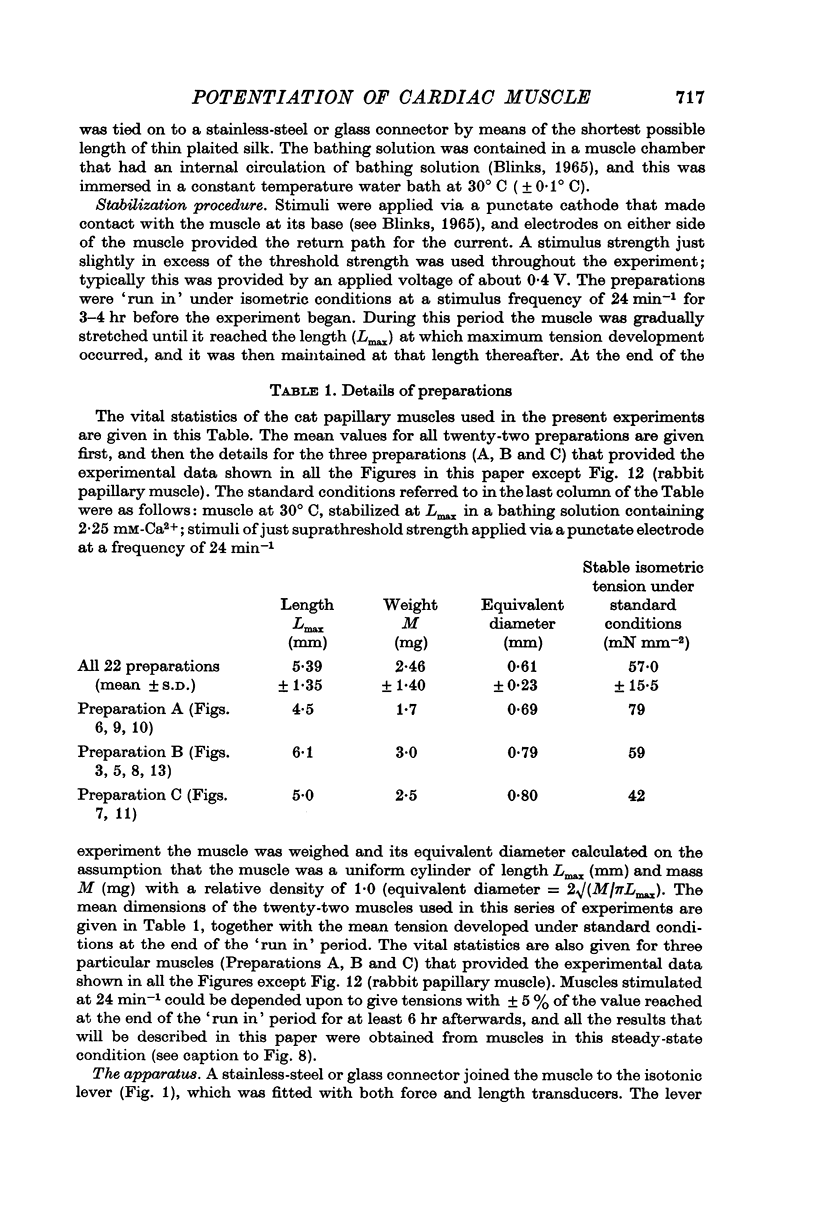
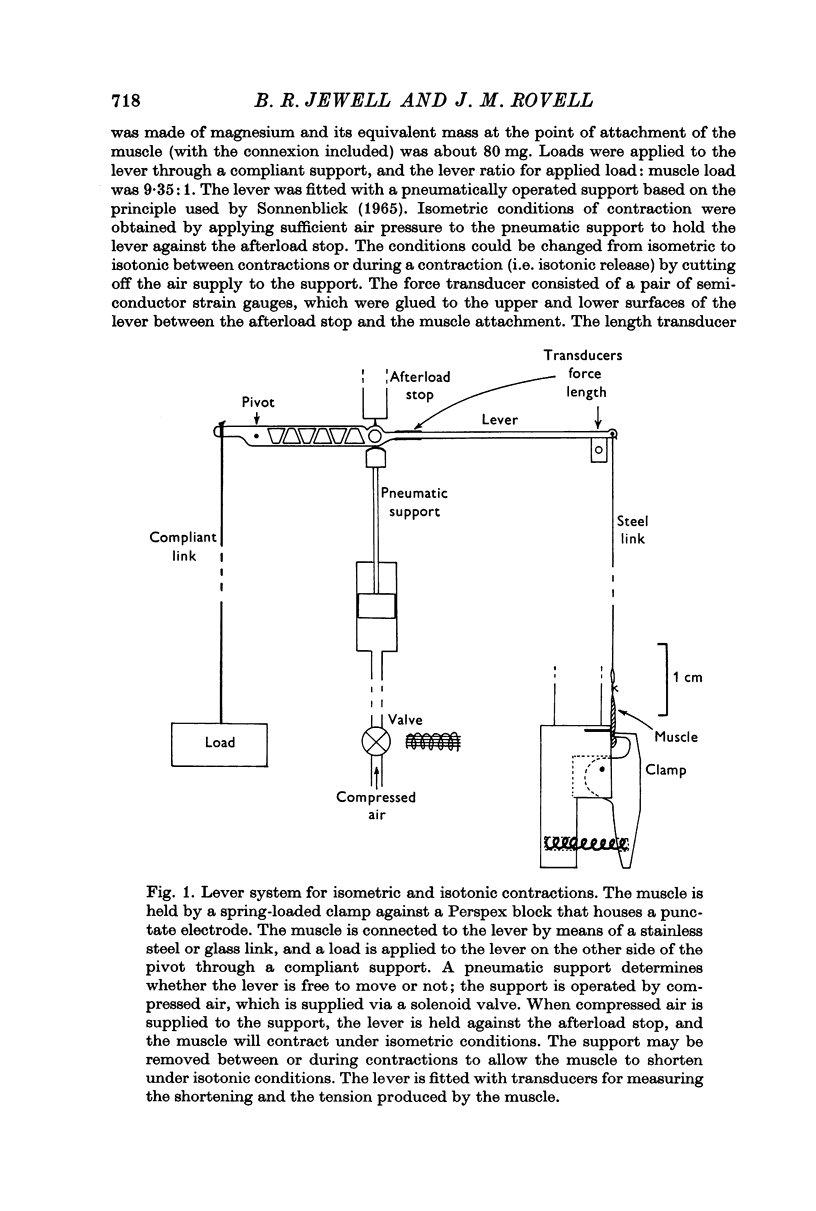
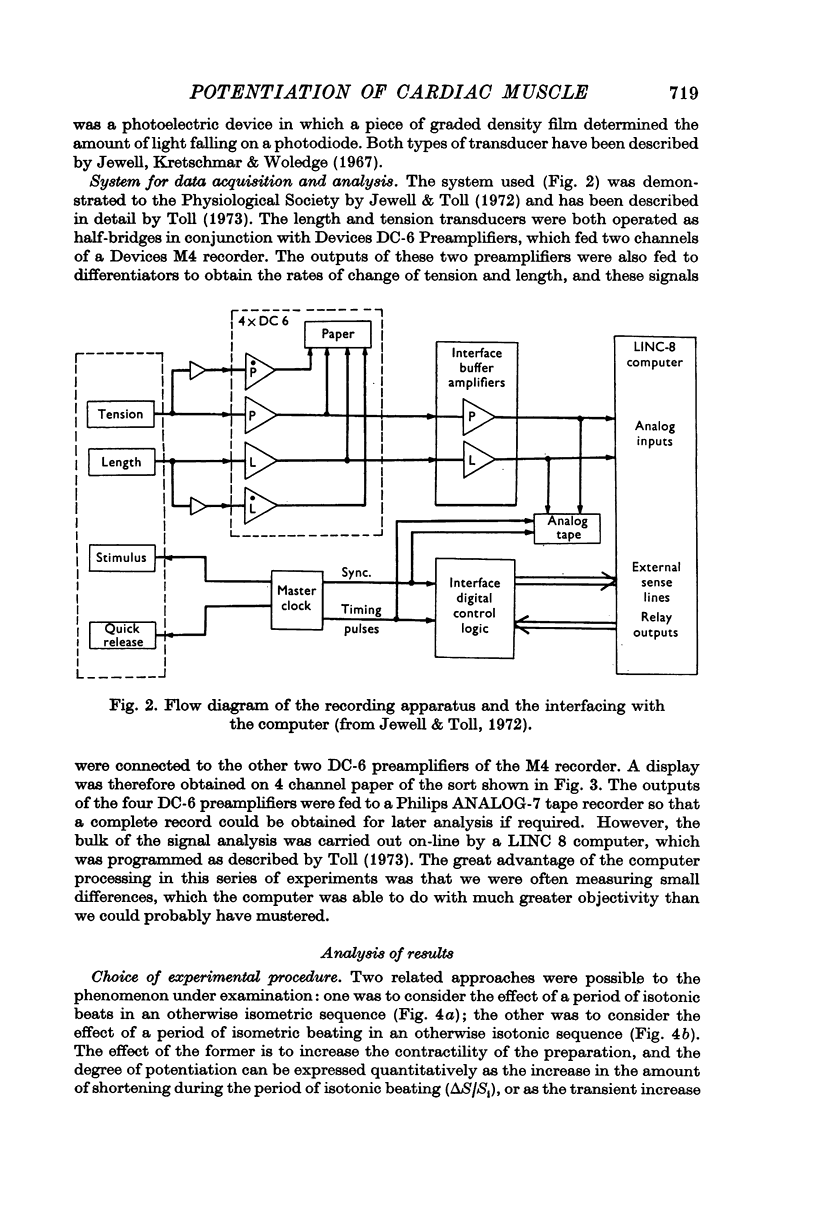

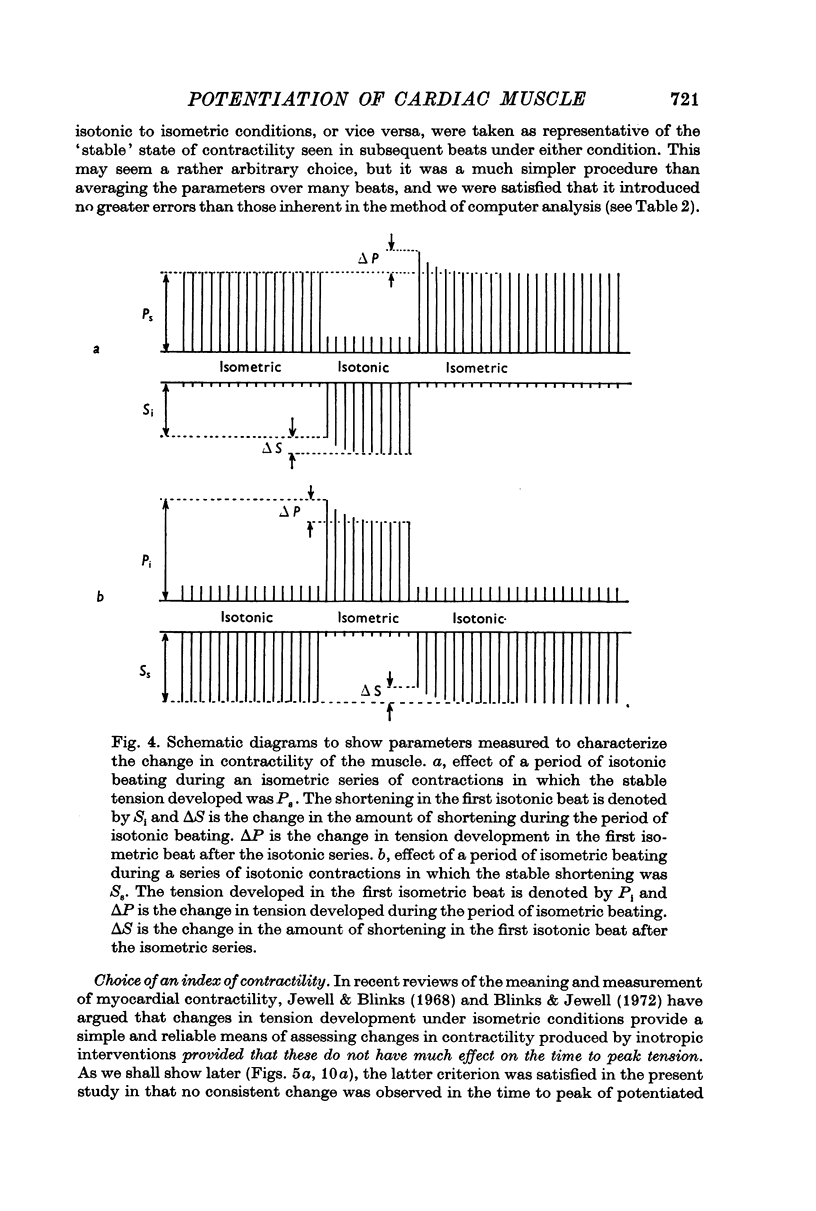
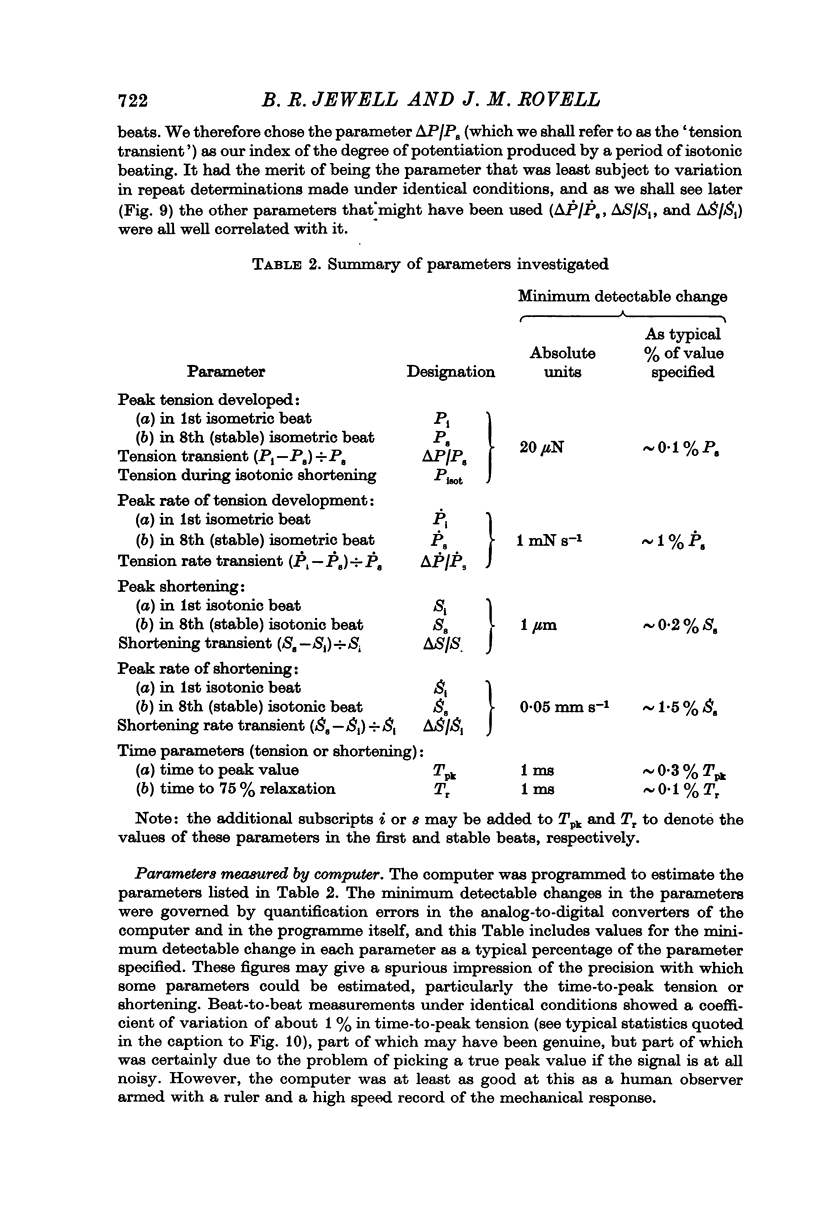
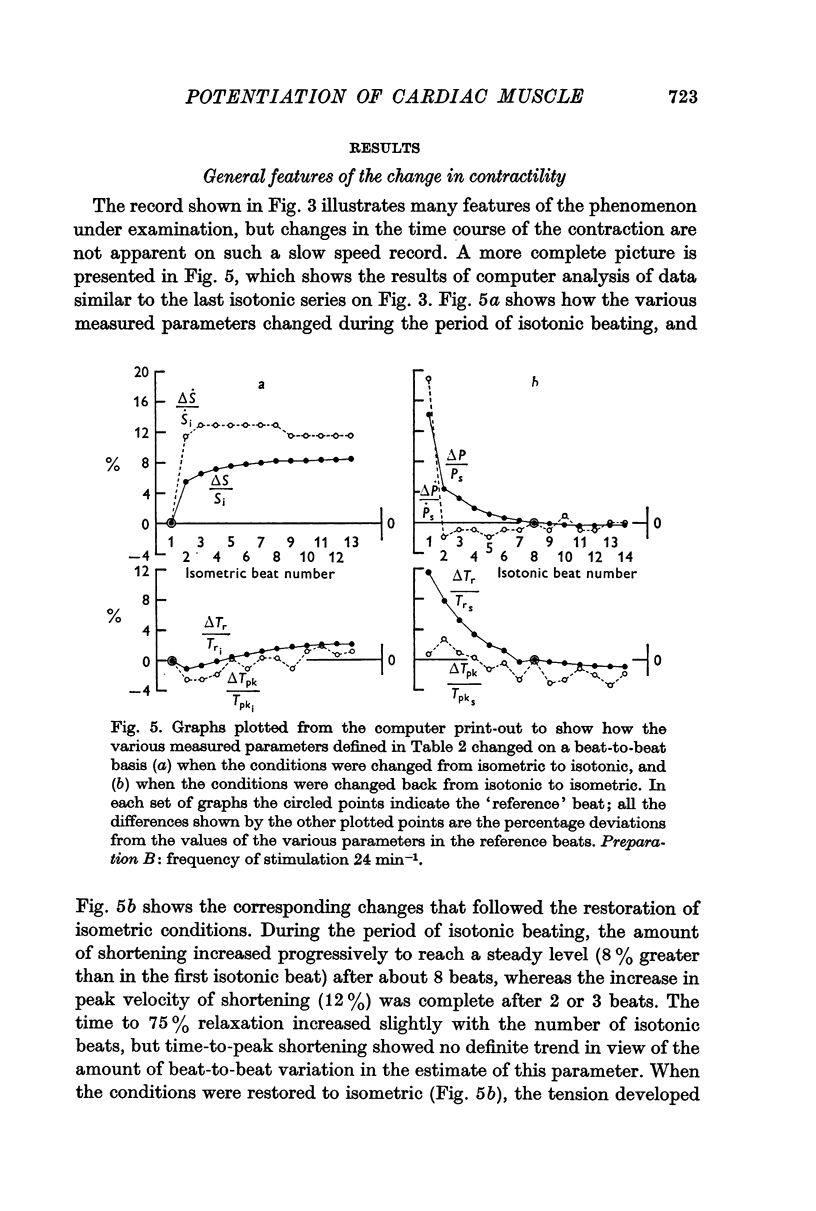
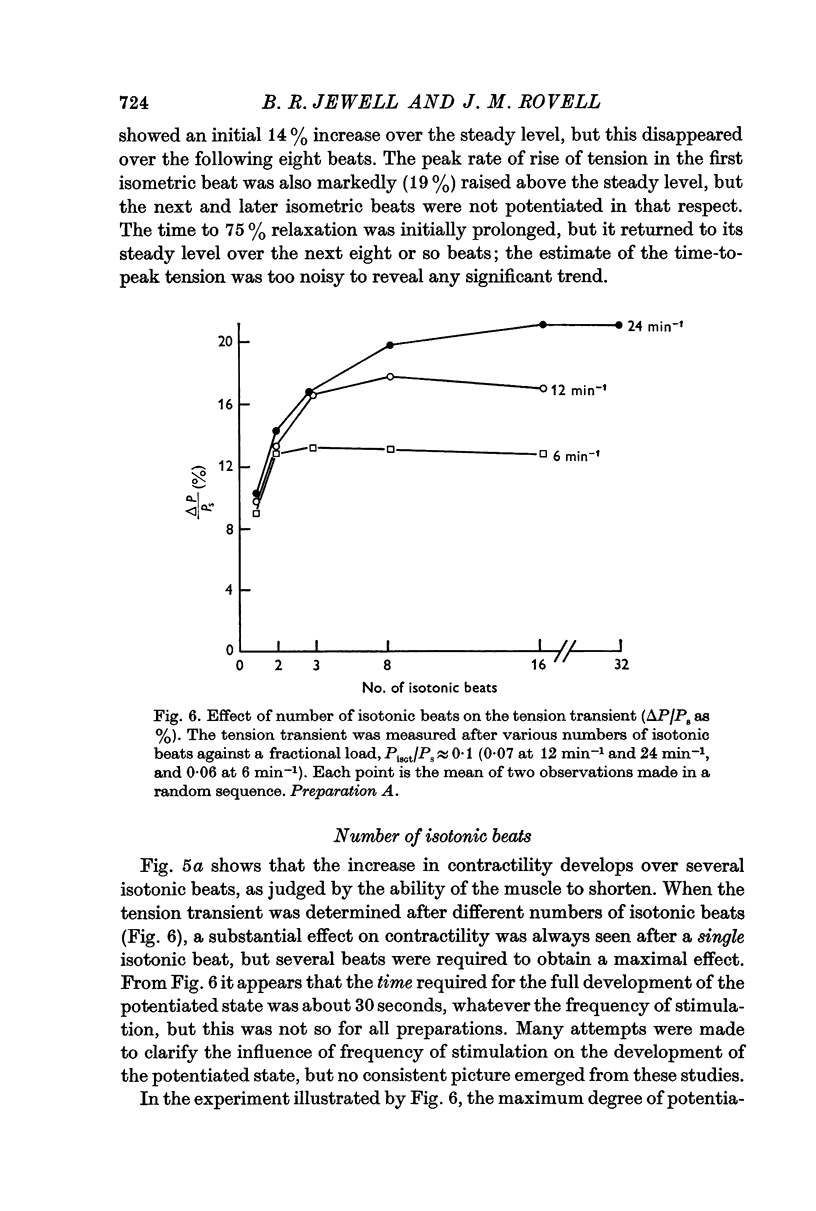
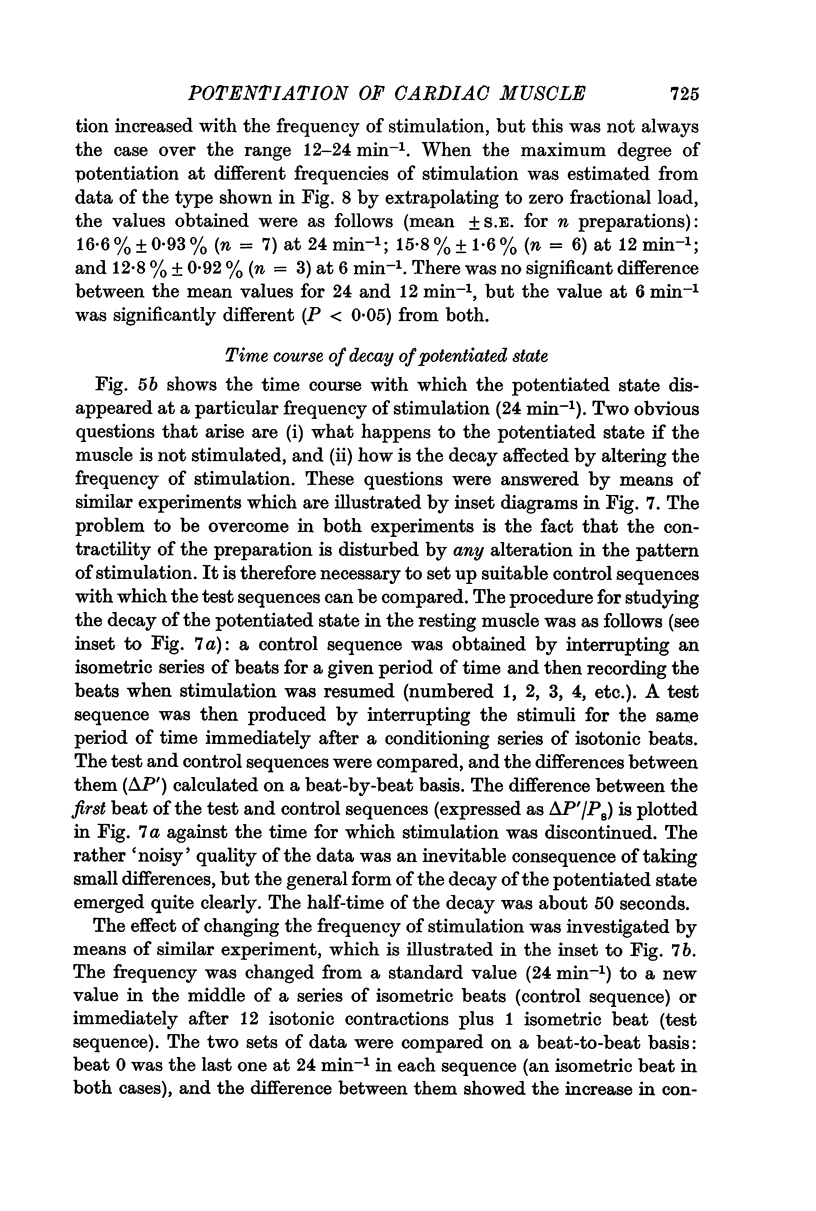
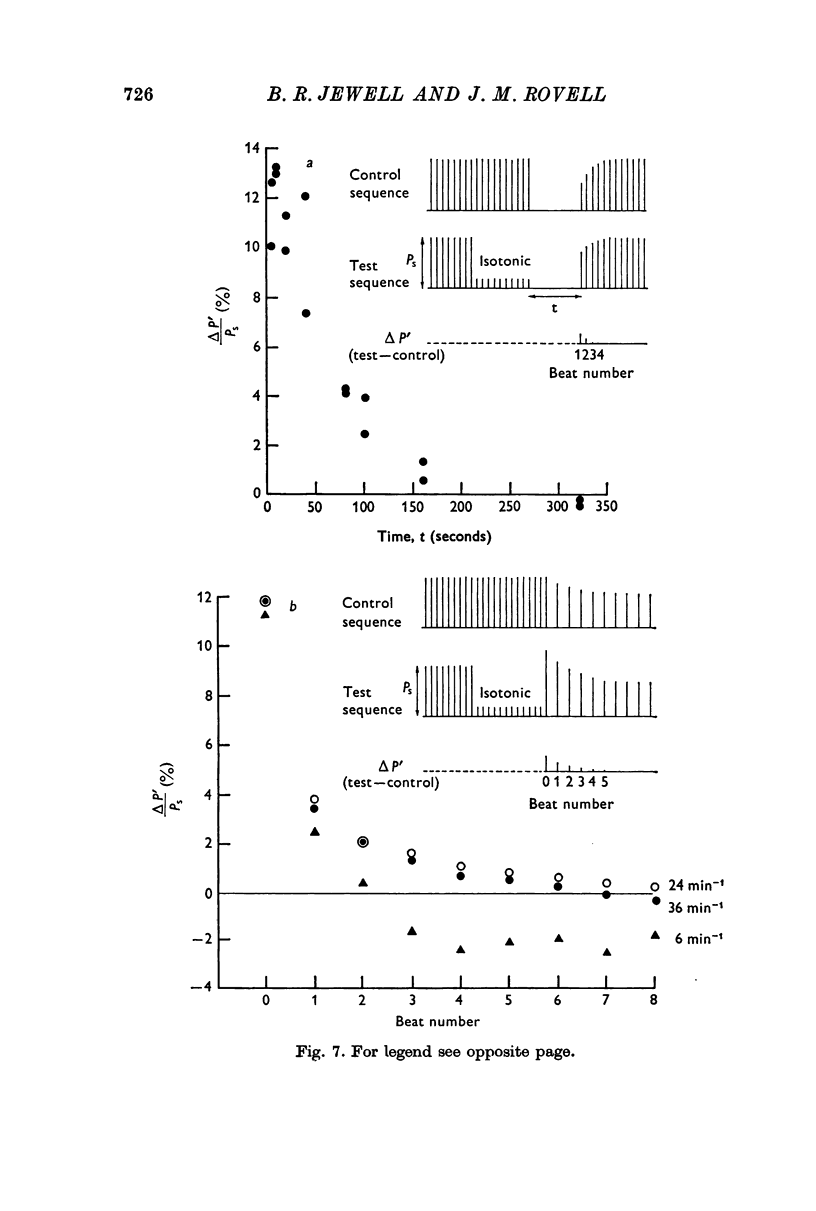
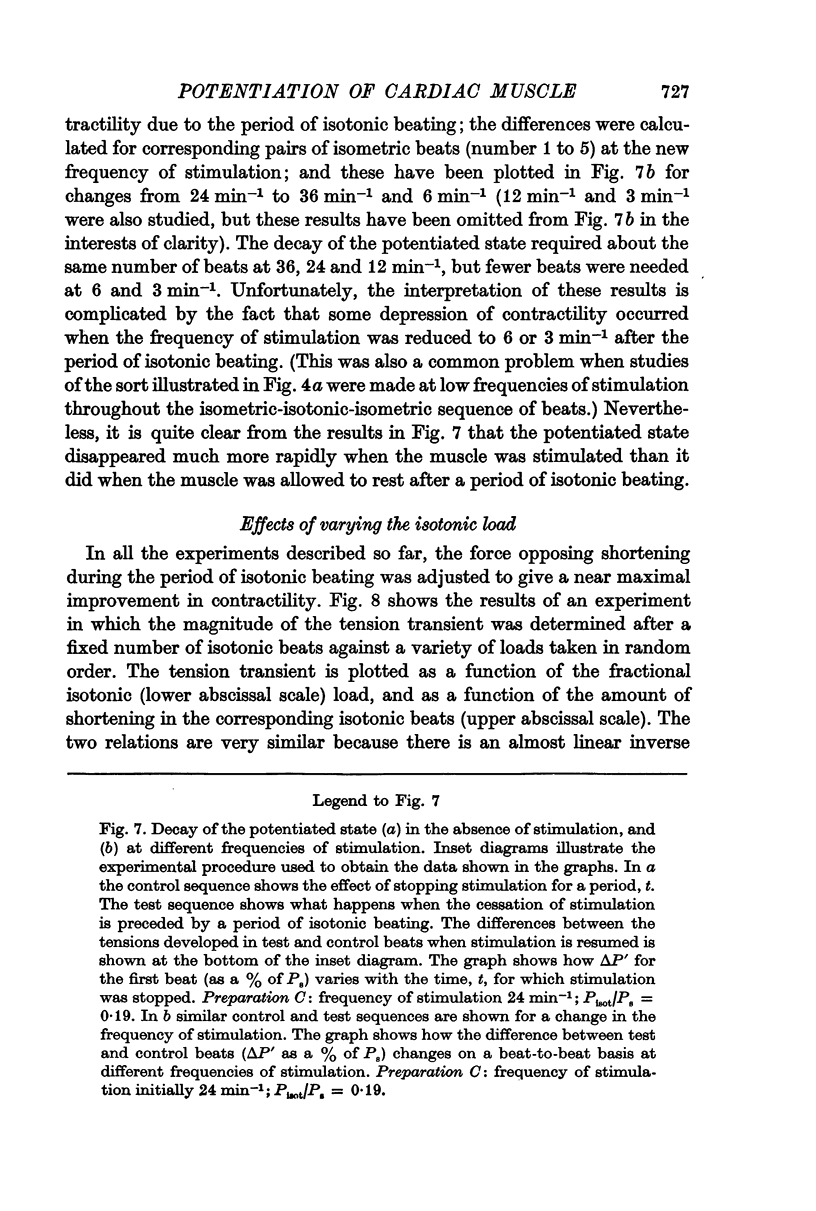
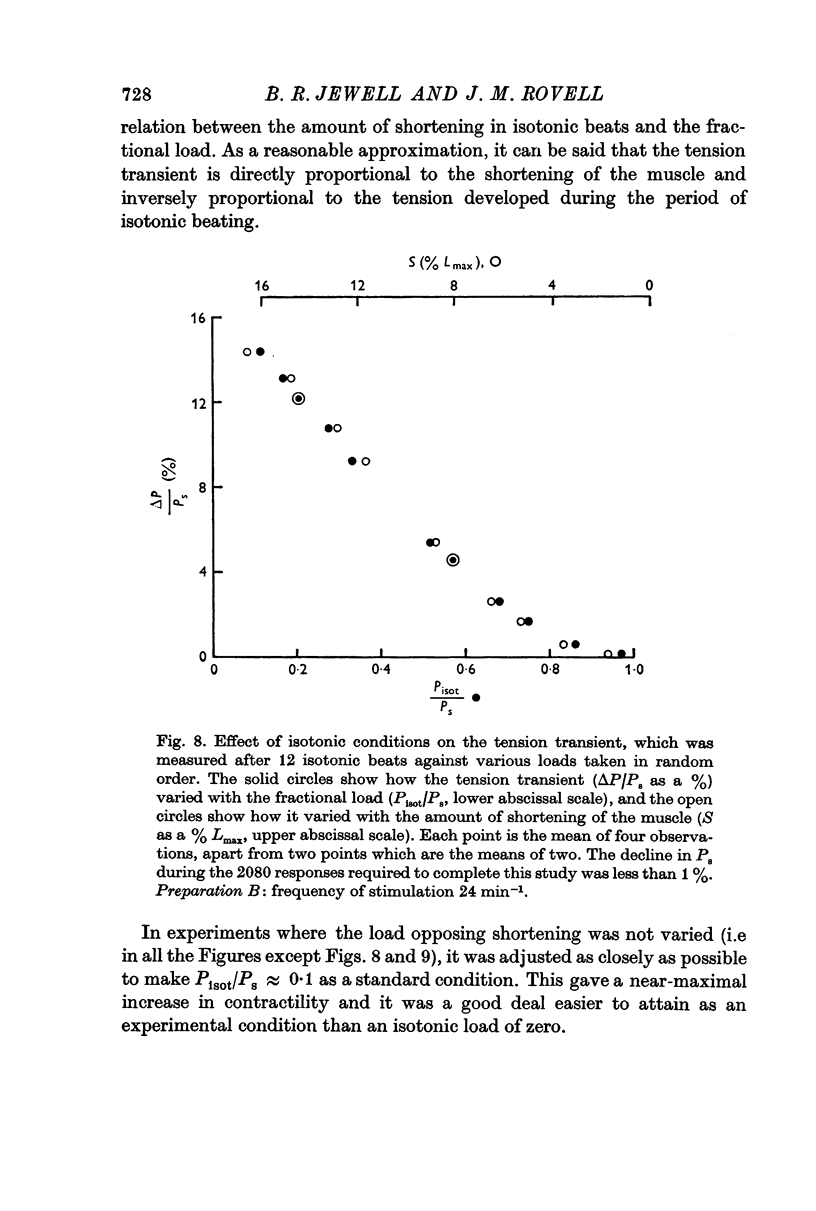
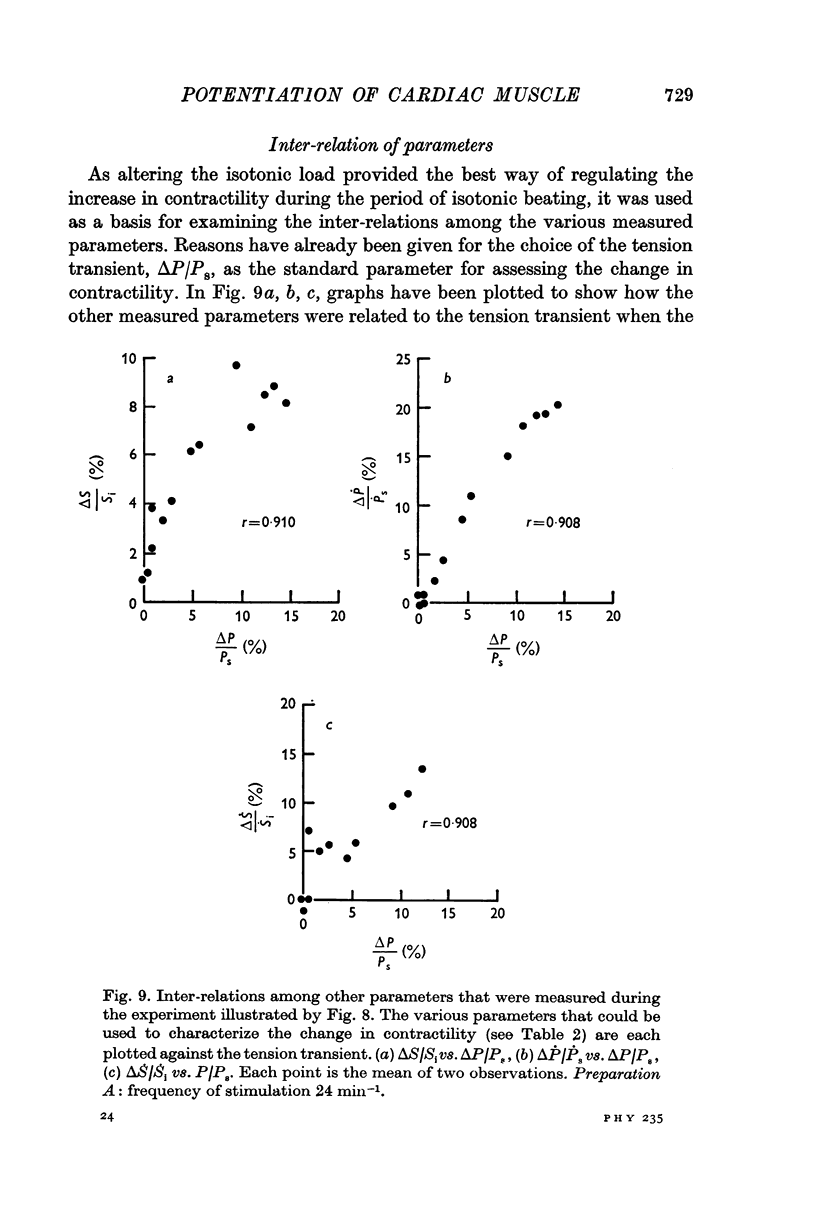
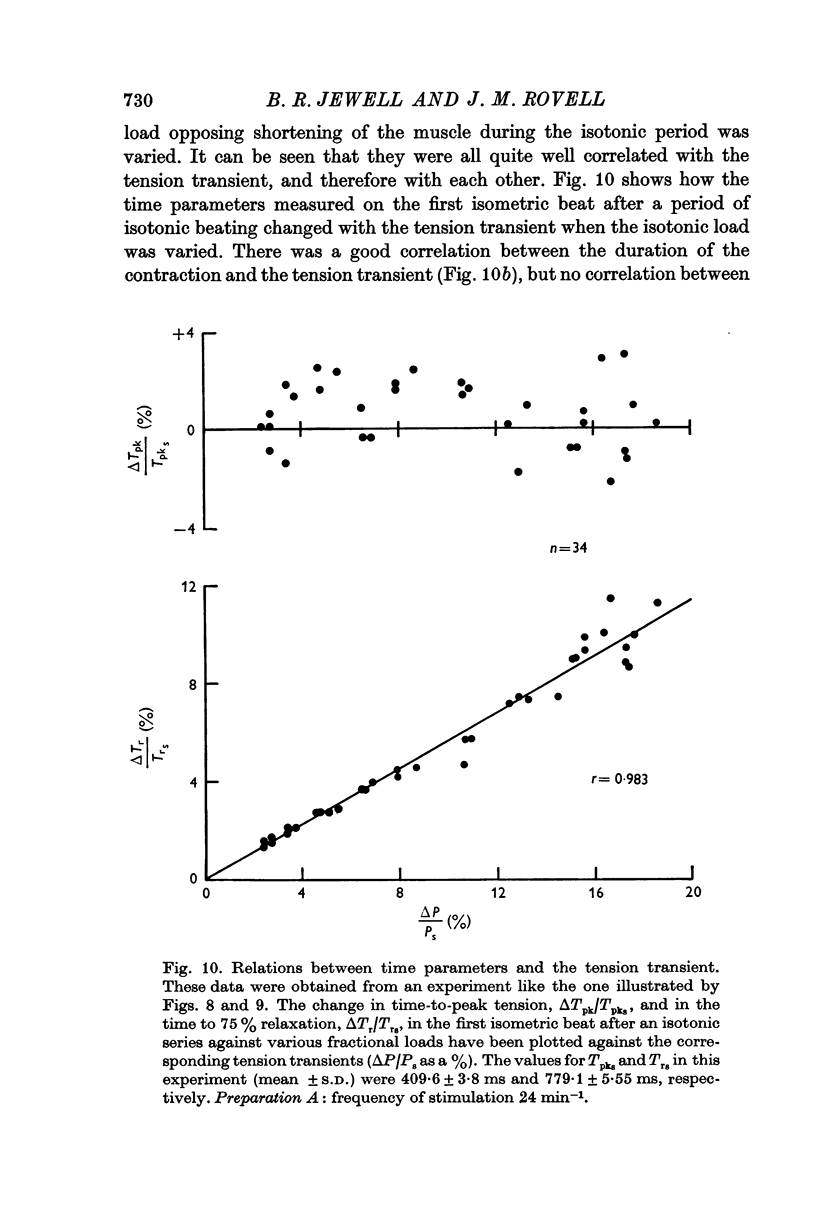
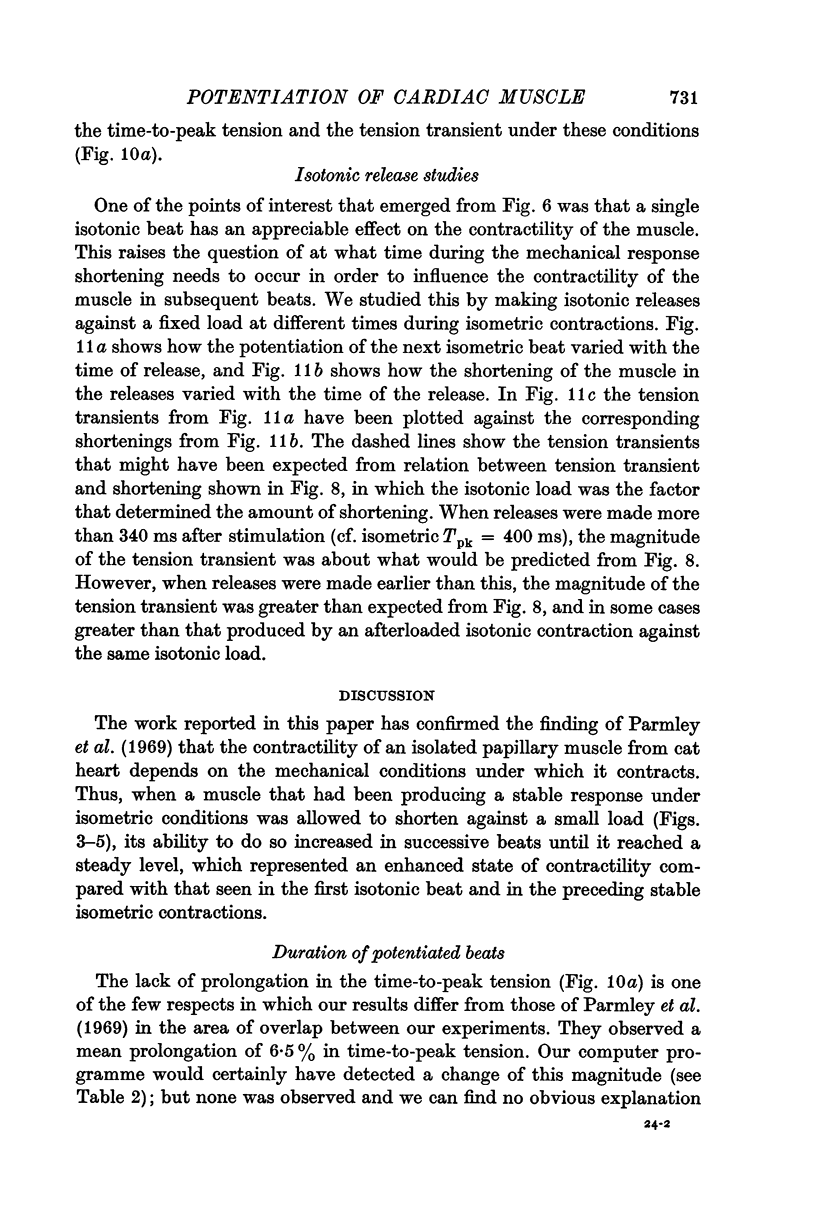
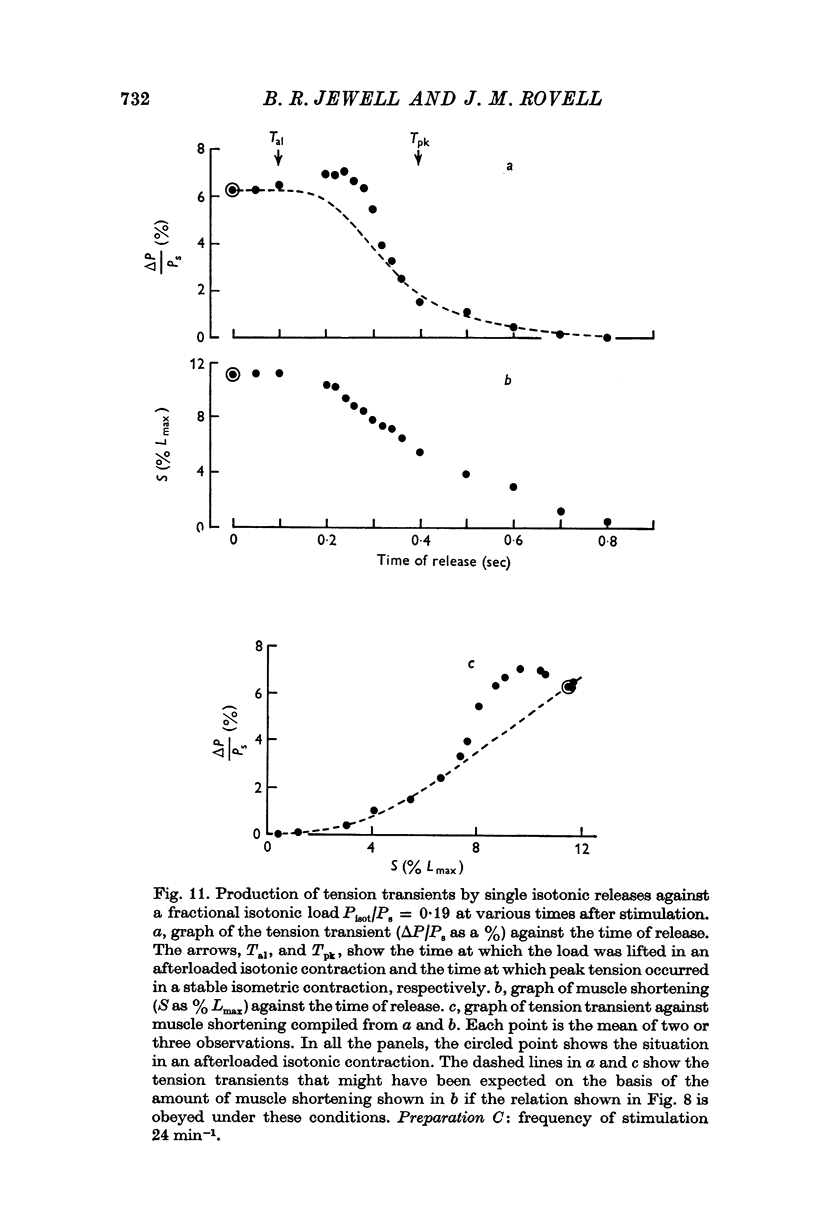
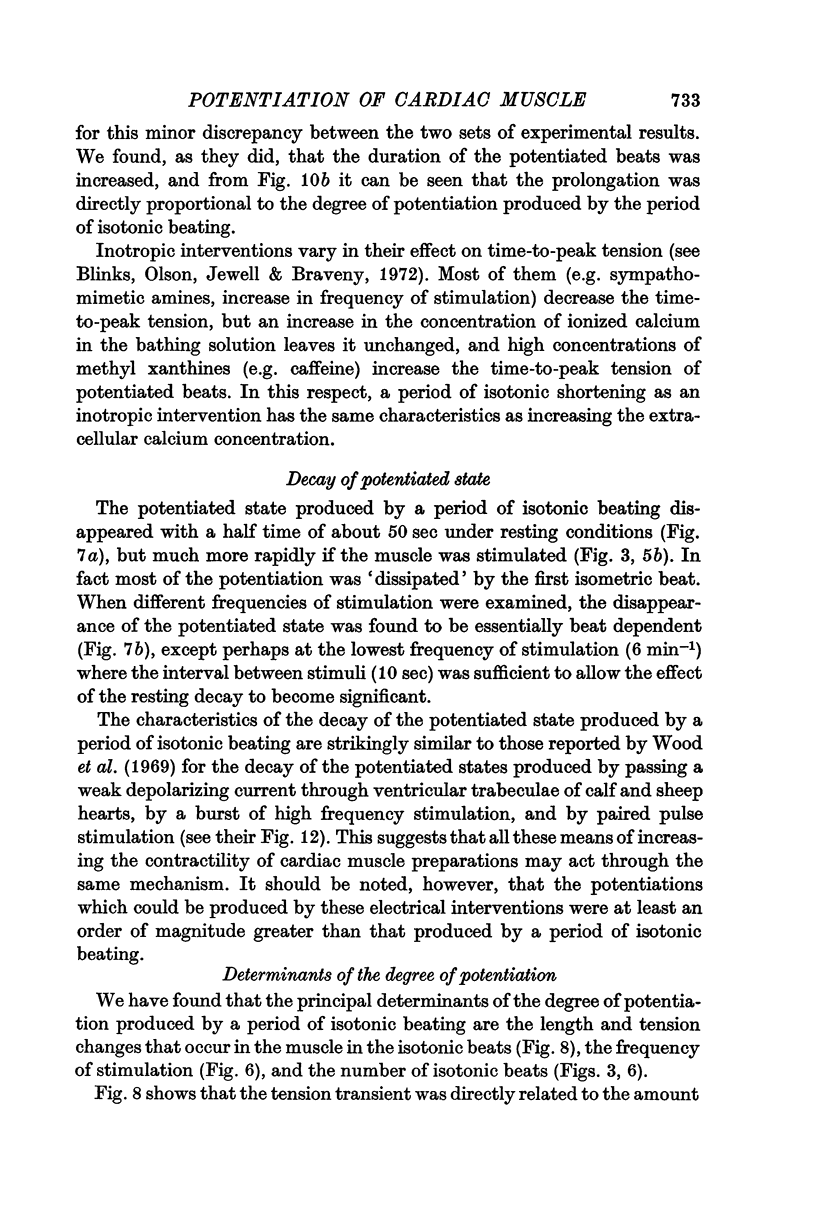
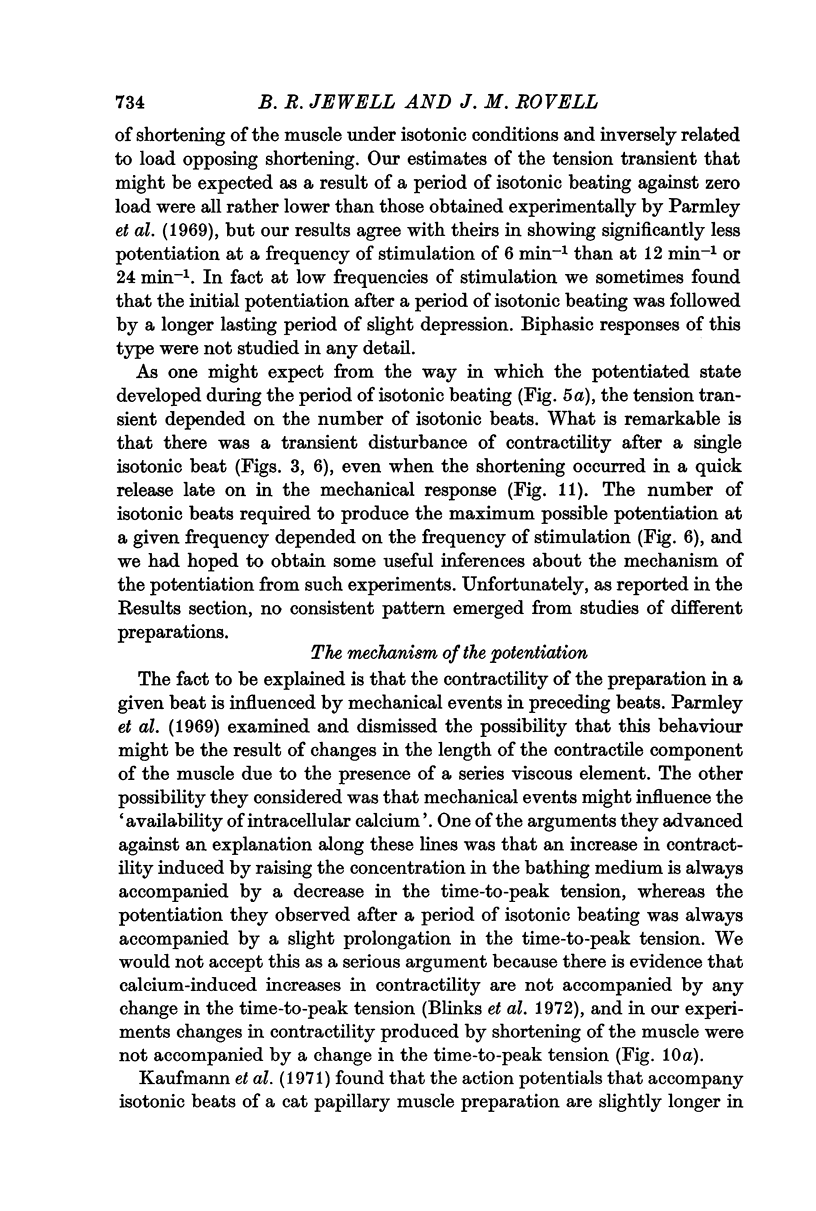
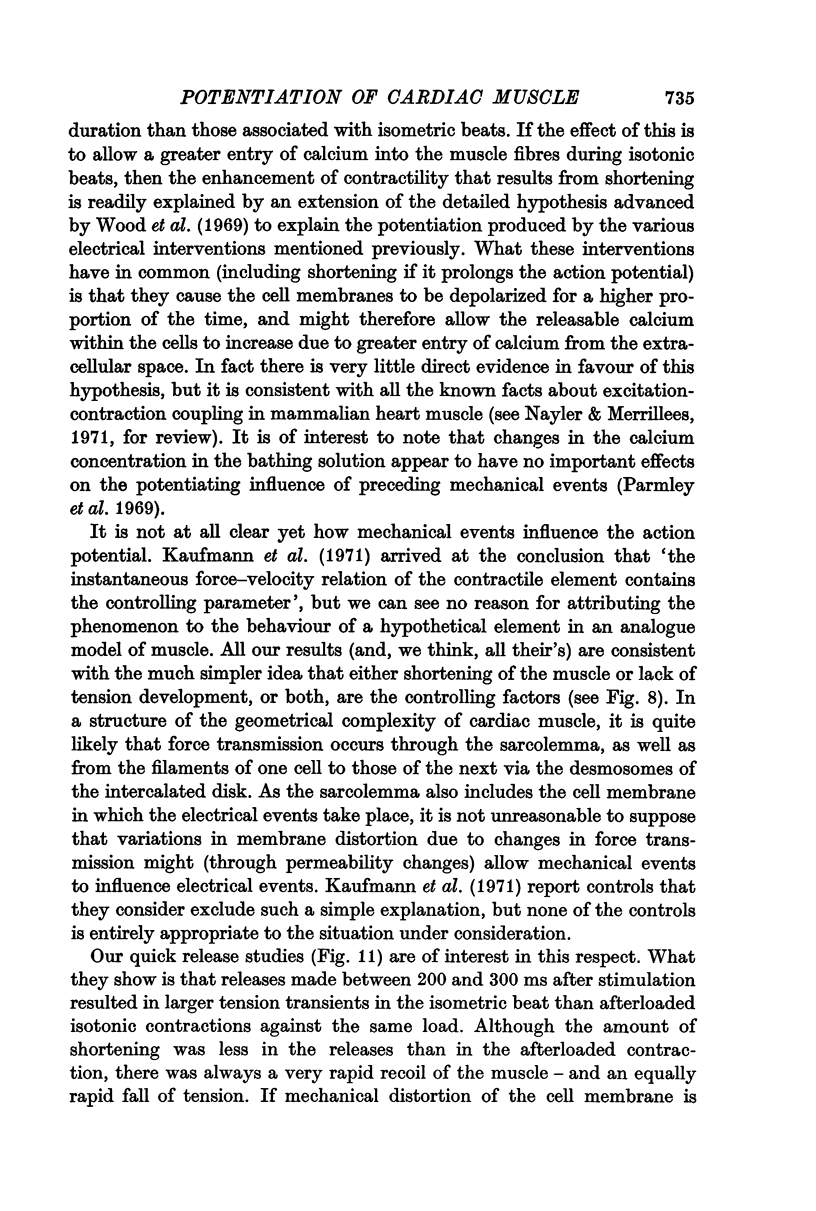
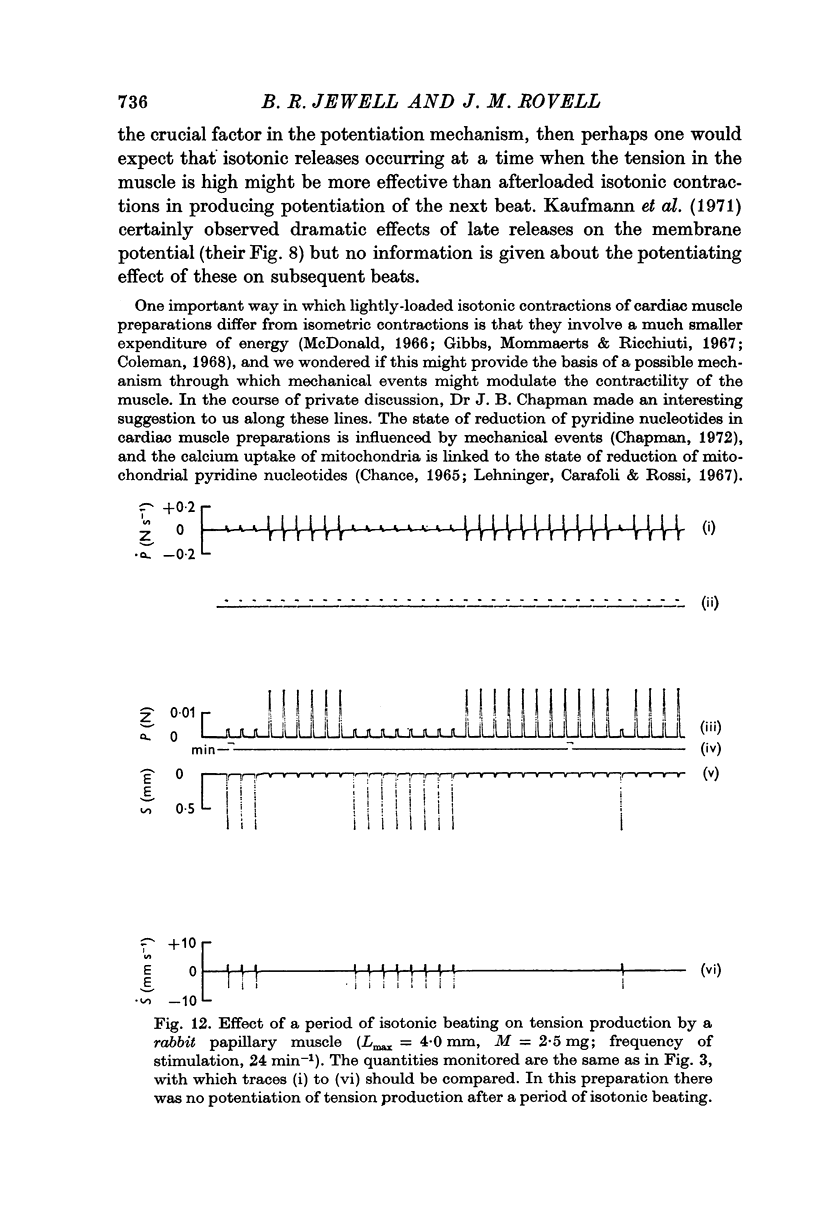
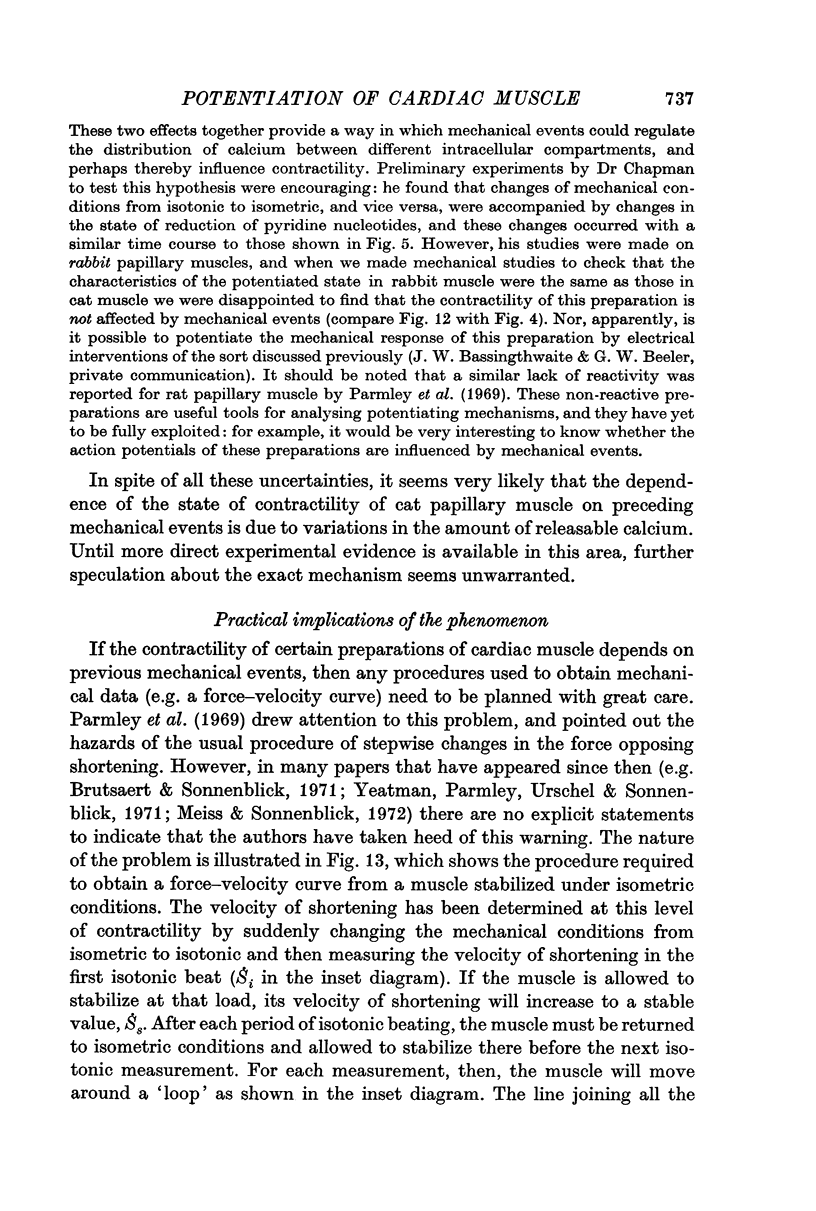
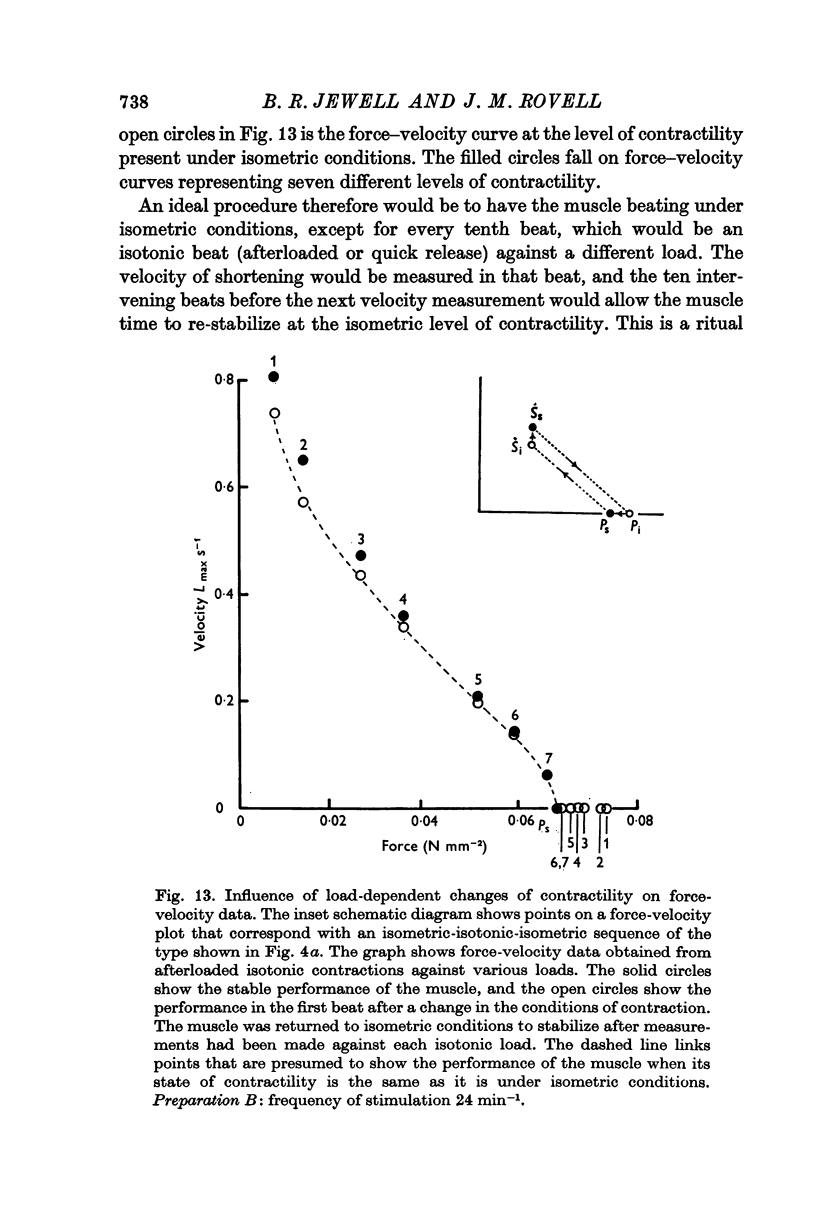
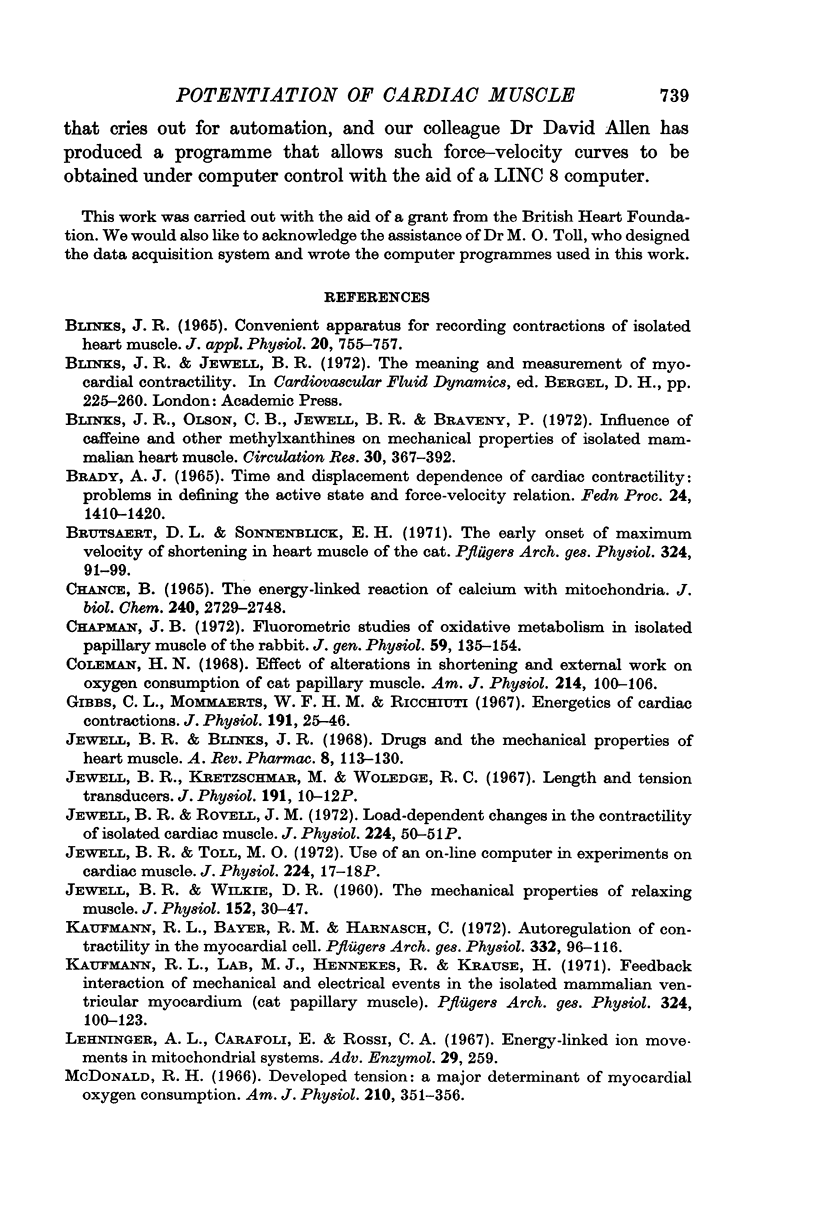
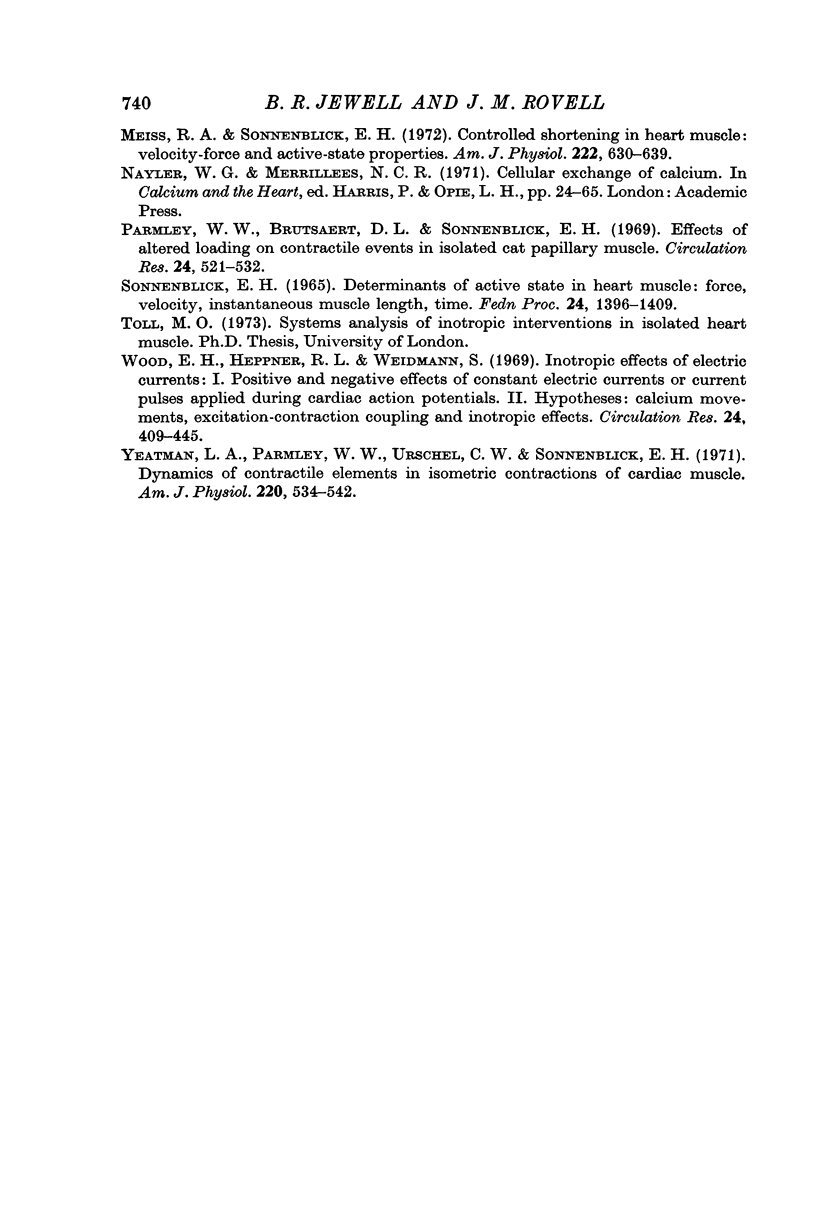
Selected References
These references are in PubMed. This may not be the complete list of references from this article.
- Blinks J. R., Olson C. B., Jewell B. R., Bravený P. Influence of caffeine and other methylxanthines on mechanical properties of isolated mammalian heart muscle. Evidence for a dual mechanism of action. Circ Res. 1972 Apr;30(4):367–392. doi: 10.1161/01.res.30.4.367. [DOI] [PubMed] [Google Scholar]
- Brady A. J. Time and displacement dependence of cardiac contractility: problems in defining the active state and force-velocity relations. Fed Proc. 1965 Nov-Dec;24(6):1410–1420. [PubMed] [Google Scholar]
- Brutsaert D. L., Sonnenblick E. H. The early onset of maximum velocity of shortening in heart muscle of the cat. Pflugers Arch. 1971;324(2):91–99. doi: 10.1007/BF00592655. [DOI] [PubMed] [Google Scholar]
- CHANCE B. THE ENERGY-LINKED REACTION OF CALCIUM WITH MITOCHONDRIA. J Biol Chem. 1965 Jun;240:2729–2748. [PubMed] [Google Scholar]
- Chapman J. B. Fluorometric studies of oxidative metabolism in isolated papillary muscle of the rabbit. J Gen Physiol. 1972 Feb;59(2):135–154. doi: 10.1085/jgp.59.2.135. [DOI] [PMC free article] [PubMed] [Google Scholar]
- Coleman H. N. Effect of alterations in shortening and external work on oxygen consumption of cat papillary muscle. Am J Physiol. 1968 Jan;214(1):100–106. doi: 10.1152/ajplegacy.1968.214.1.100. [DOI] [PubMed] [Google Scholar]
- Gibbs C. L., Mommaerts W. F., Ricchiuti N. V. Energetics of cardiac contractions. J Physiol. 1967 Jul;191(1):25–46. doi: 10.1113/jphysiol.1967.sp008235. [DOI] [PMC free article] [PubMed] [Google Scholar]
- JEWELL B. R., WILKIE D. R. The mechanical properties of relaxing muscle. J Physiol. 1960 Jun;152:30–47. doi: 10.1113/jphysiol.1960.sp006467. [DOI] [PMC free article] [PubMed] [Google Scholar]
- Jewell B. R., Blinks J. R. Drugs and the mechanical properties of heart muscle. Annu Rev Pharmacol. 1968;8:113–130. doi: 10.1146/annurev.pa.08.040168.000553. [DOI] [PubMed] [Google Scholar]
- Jewell B. R., Kretzschmar M., Woledge R. C. Length and tension transducers. J Physiol. 1967 Jul;191(1):10P–12P. [PubMed] [Google Scholar]
- Jewell B. R., Rovell J. M. Load-dependent changes in the contractility of isolated cardiac muscle. J Physiol. 1972 Jul;224(1):50P–51P. [PubMed] [Google Scholar]
- Jewell B. R., Toll M. O. Use of an on-line computer in experiments on cardiac muscle. J Physiol. 1972 Jul;224(1):17P–18P. [PubMed] [Google Scholar]
- Kaufmann R. L., Bayer R. M., Harnasch C. Autoregulation of contractility in the myocardial cell. Displacement as a controlling parameter. Pflugers Arch. 1972;332(2):96–116. [PubMed] [Google Scholar]
- Kaufmann R. L., Lab M. J., Hennekes R., Krause H. Feedback interaction of mechanical and electrical events in the isolated mammalian ventricular myocardium (cat papillary muscle). Pflugers Arch. 1971;324(2):100–123. doi: 10.1007/BF00592656. [DOI] [PubMed] [Google Scholar]
- Lehninger A. L., Carafoli E., Rossi C. S. Energy-linked ion movements in mitochondrial systems. Adv Enzymol Relat Areas Mol Biol. 1967;29:259–320. doi: 10.1002/9780470122747.ch6. [DOI] [PubMed] [Google Scholar]
- McDonald R. H., Jr Developed tension: a major determinant of myocardial oxygen consumption. Am J Physiol. 1966 Feb;210(2):351–356. doi: 10.1152/ajplegacy.1966.210.2.351. [DOI] [PubMed] [Google Scholar]
- Meiss R. A., Sonnenblick E. H. Controlled shortening in heart muscle: velocity-force and active-state properties. Am J Physiol. 1972 Mar;222(3):630–639. doi: 10.1152/ajplegacy.1972.222.3.630. [DOI] [PubMed] [Google Scholar]
- Parmley W. W., Brutsaert D. L., Sonnenblick E. H. Effects of altered loading on contractile events in isolated cat papillary muscle. Circ Res. 1969 Apr;24(4):521–532. doi: 10.1161/01.res.24.4.521. [DOI] [PubMed] [Google Scholar]
- Sonnenblick E. H. Determinants of active state in heart muscle: force, velocity, instantaneous muscle length, time. Fed Proc. 1965 Nov-Dec;24(6):1396–1409. [PubMed] [Google Scholar]
- Wood E. H., Heppner R. L., Weidmann S. Inotropic effects of electric currents. I. Positive and negative effects of constant electric currents or current pulses applied during cardiac action potentials. II. Hypotheses: calcium movements, excitation-contraction coupling and inotropic effects. Circ Res. 1969 Mar;24(3):409–445. doi: 10.1161/01.res.24.3.409. [DOI] [PubMed] [Google Scholar]
- Yeatman L. A., Jr, Parmley W. W., Urschel C. W., Sonnenblick E. H. Dynamics of contractile elements in isometric contractions of cardiac muscle. Am J Physiol. 1971 Feb;220(2):534–542. doi: 10.1152/ajplegacy.1971.220.2.534. [DOI] [PubMed] [Google Scholar]