Abstract
1. Rohon-Beard cells in the spinal cord of Xenopus tadpoles have been studied in animals from early neural tube to free-swimming larval stages. The onset and further development of electrical excitability of these neurones has been investigated in different ionic environments, to determine the ionic species carrying the inward current of the action potential.
2. The cells appear inexcitable at early stages (Nieuwkoop & Faber stages 18-20) and do not give action potentials to depolarizing current pulses.
3. The action potential is first recorded at stage 20. (A) The inward current is carried by Ca2+ at stages 20-25, since it is blocked by mm quantitites of La3+, Co2+ or Mn2+ and is unaffected by removal of Na+ or the addition of tetrodotoxin (TTX). (B) The action potential is an elevated plateau of long duration (mean 190 msec at stages 20-22). The duration decreases exponentially with repetitive stimulation. (C) The specific Ca2+ conductance (gCa) at the onset of the plateau of the action potential is 2·6 × 10-4 mho/cm2. Calculations show that a single action potential raises [Ca2+]1 by more than 100-fold.
4. At later times (stages 25-40), the inward current of the action potential is carried by both Na+ and Ca2+: the action potential has two components, an initial spike which is blocked by removal of Na+ or addition of TTX, followed by a plateau which is blocked by La3+, Co2+ or Mn2+.
5. Finally (stages 40-51), the inward current is primarily carried by Na+, since the action potential is blocked only by removal of Na+ or addition of TTX, and the overshoot agrees with the prediction of the Nernst equation for a Na-selective membrane. When the outward current channel is blocked and cells exposed to Na-free solutions, 67% of cells at the latest stages studied were incapable of producing action potentials in which the inward current is carried by divalent cations.
6. The duration of the action potential decreases from a maximum of about 1000 msec to about 1 msec during development. The maximum input resistance (Rin) decreases from ca. 1000 to 100 MΩ.
7. The calcium action potential may play a role in the development of excitability and the growth of the neurones.
Full text
PDF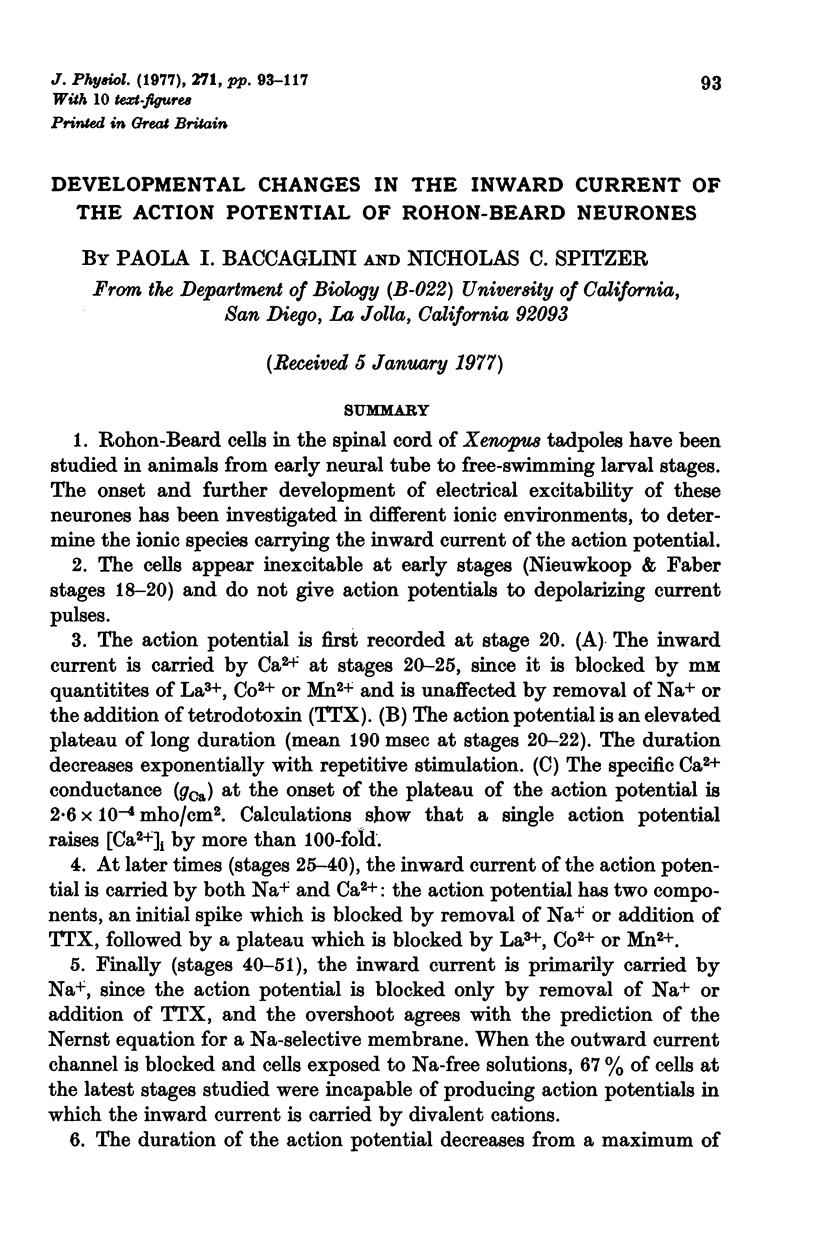
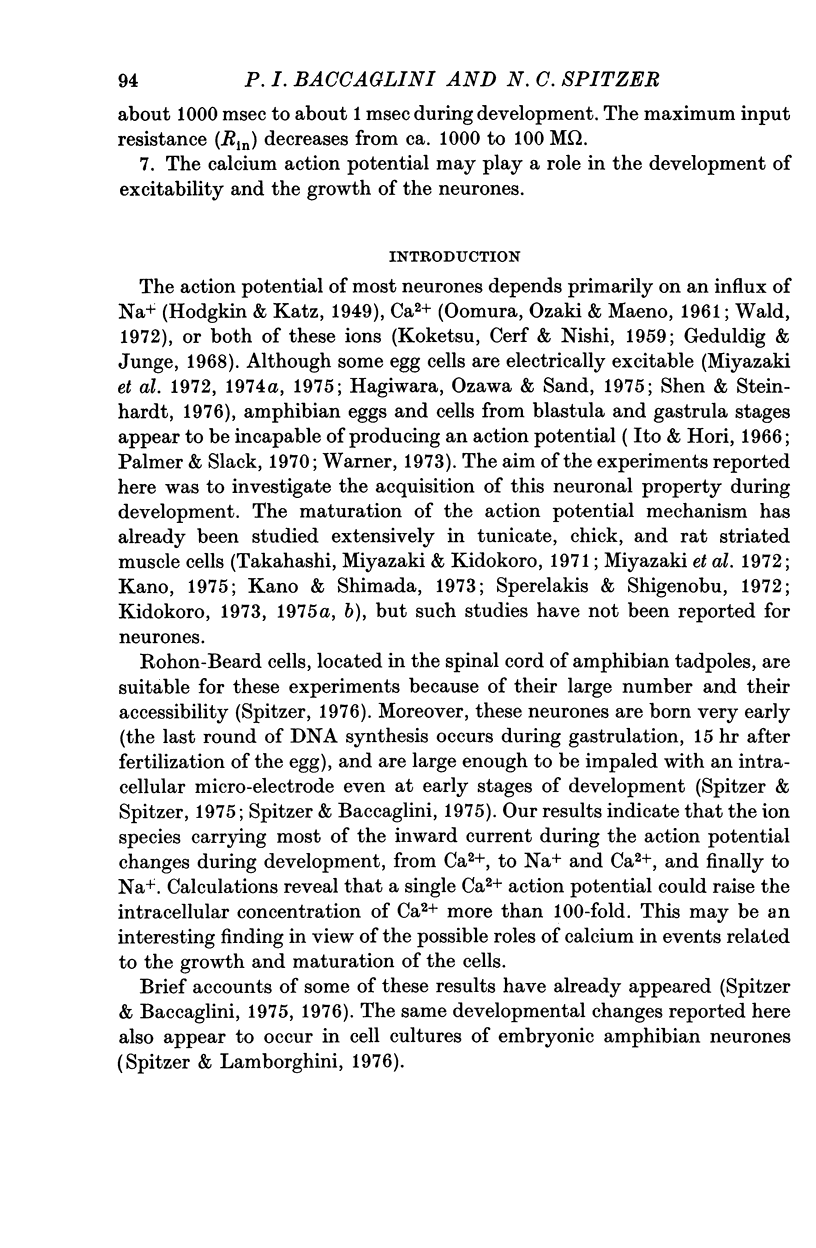
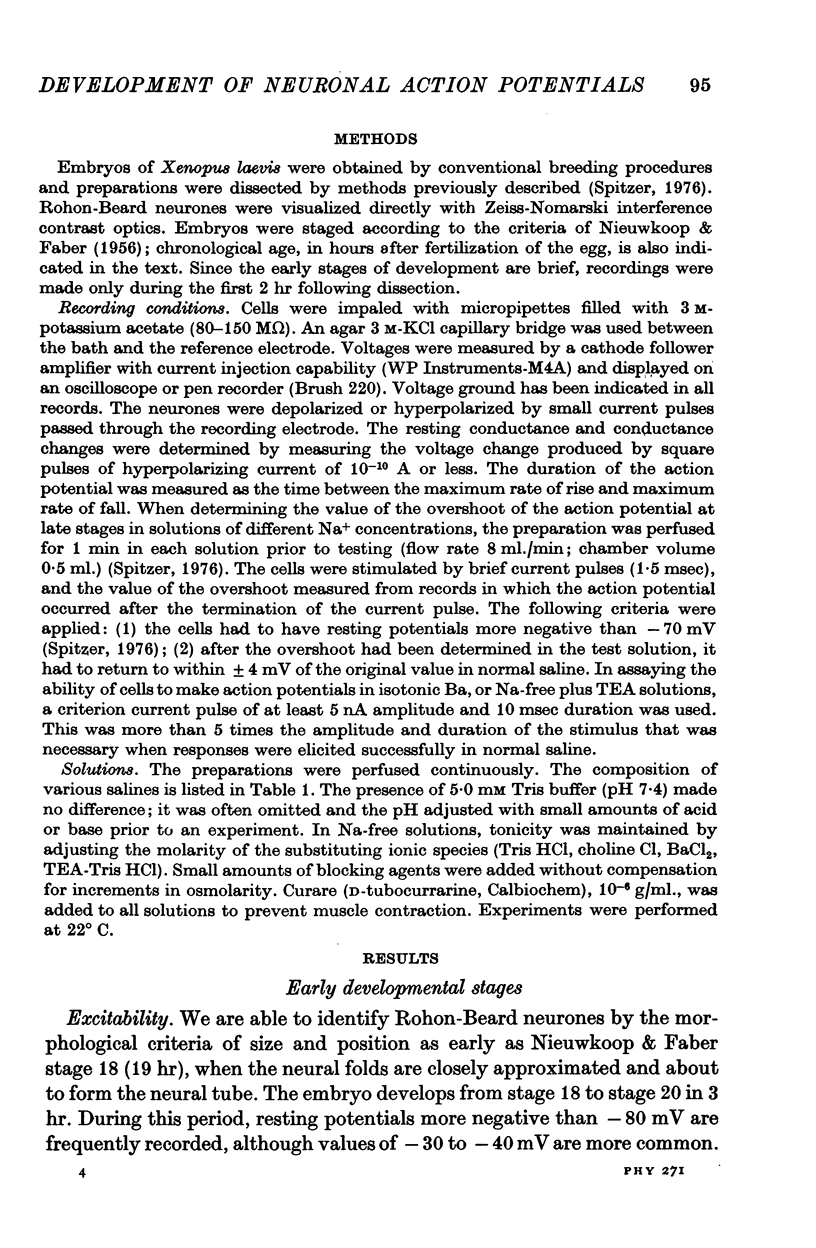
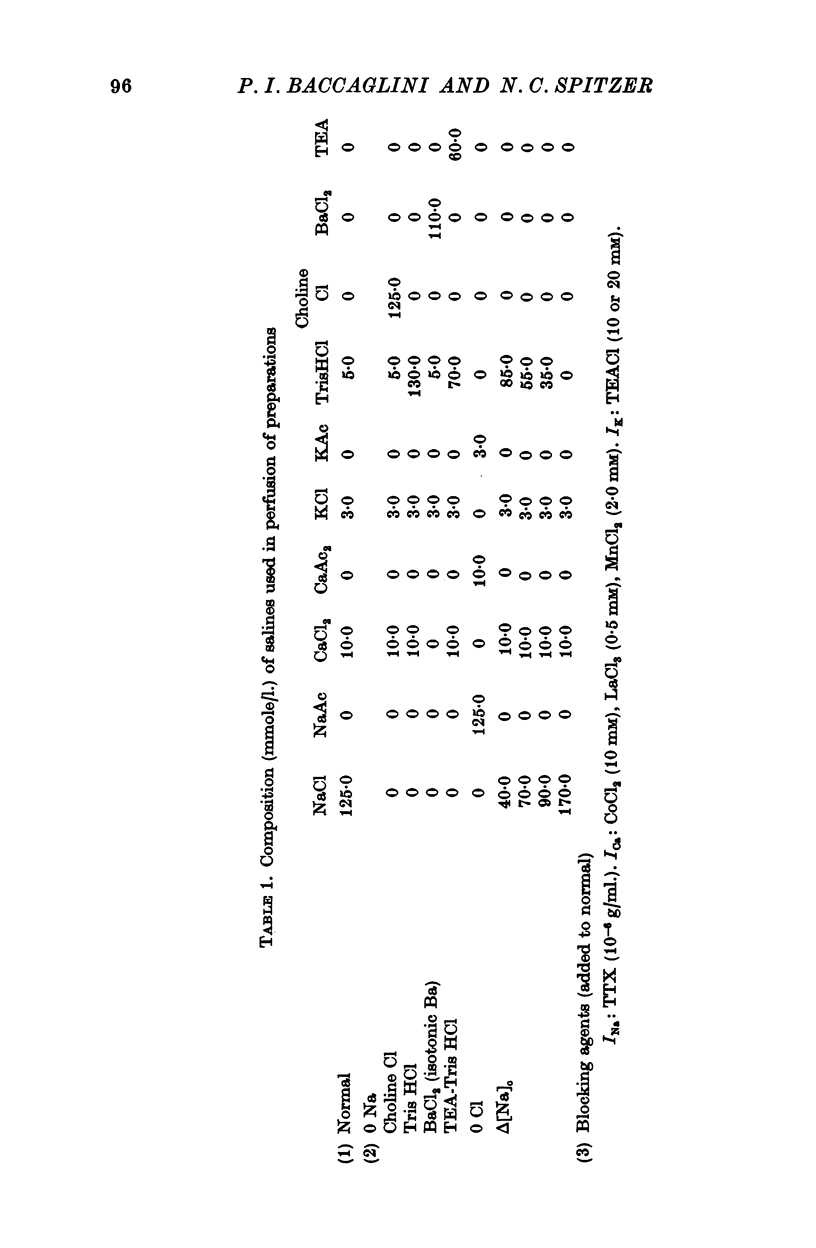
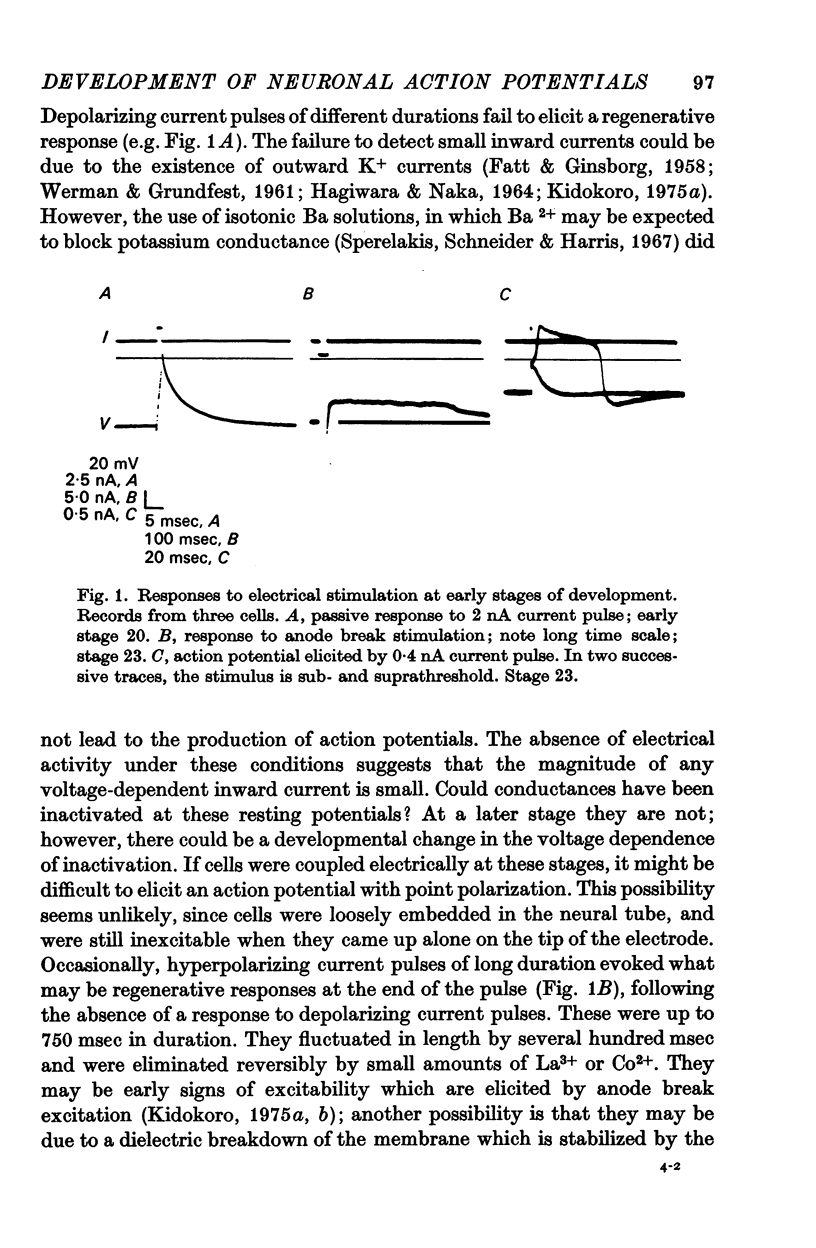
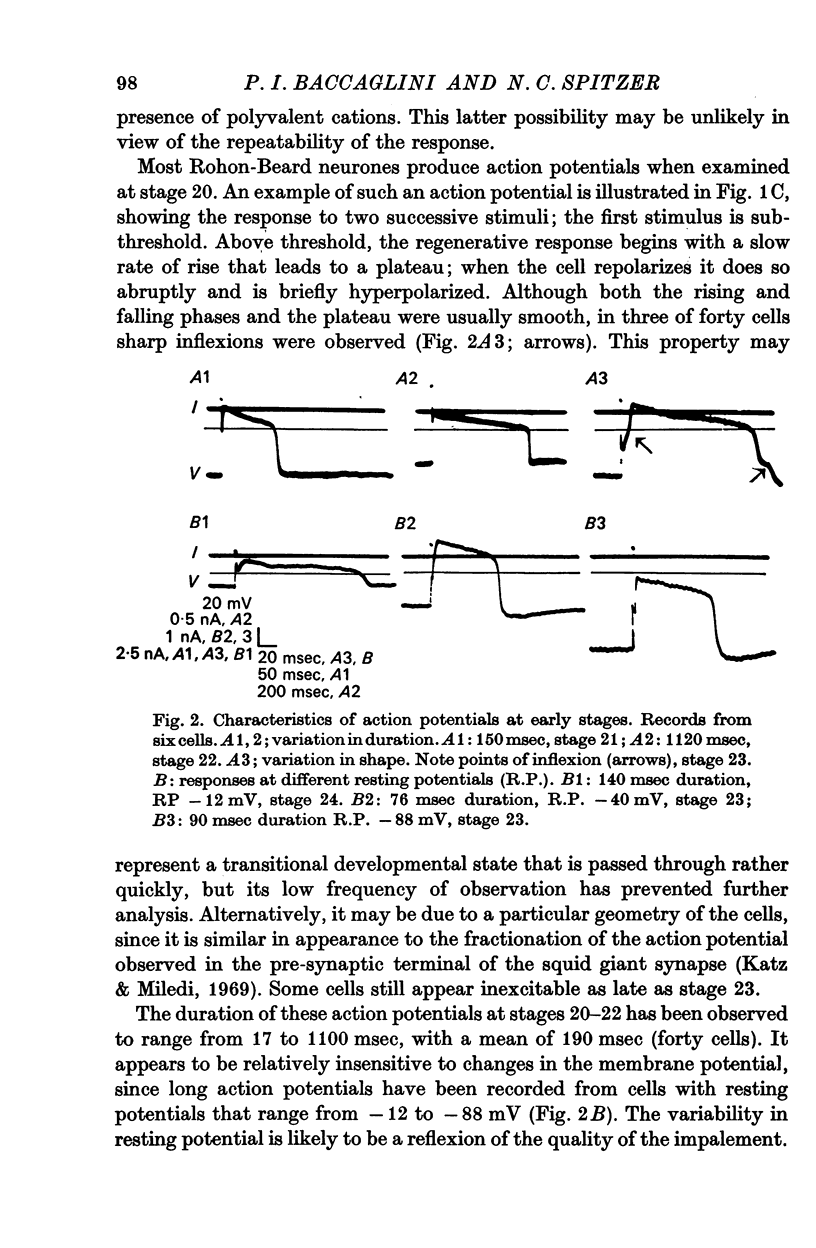
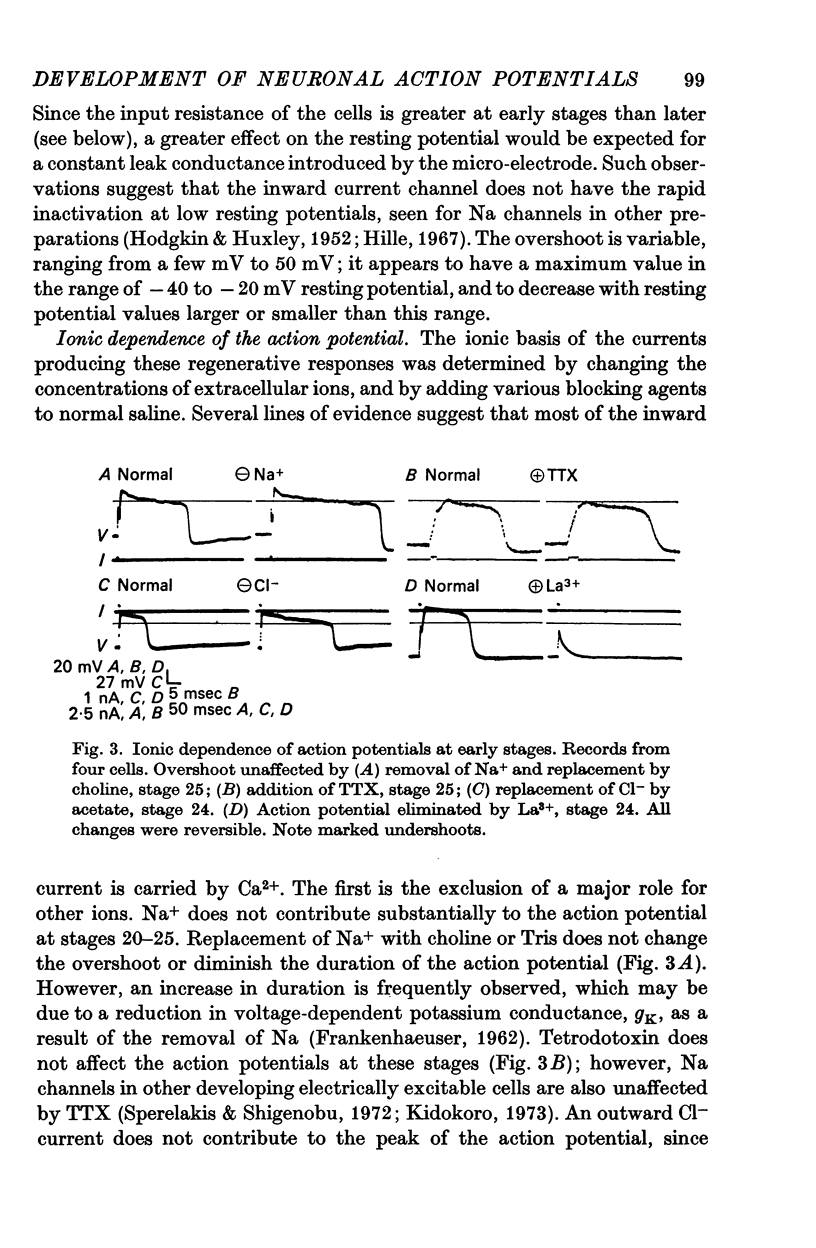
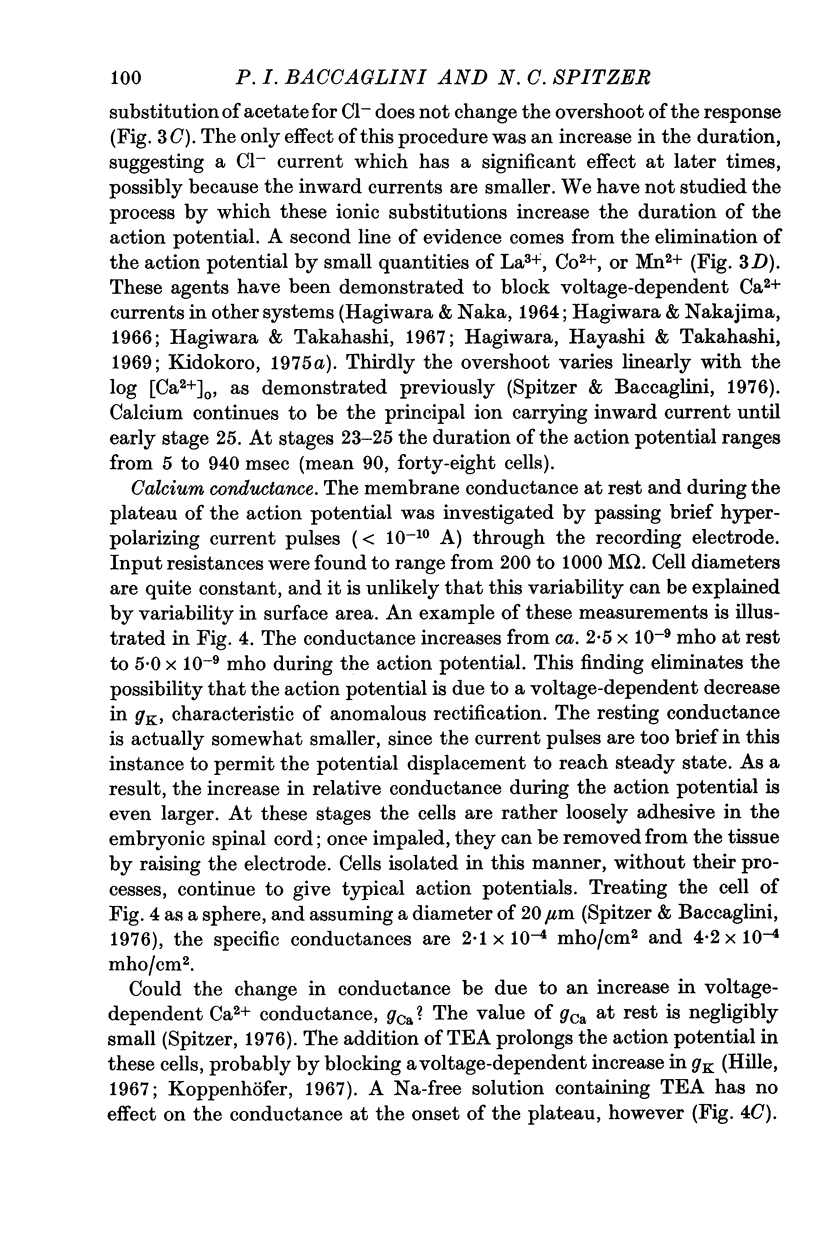
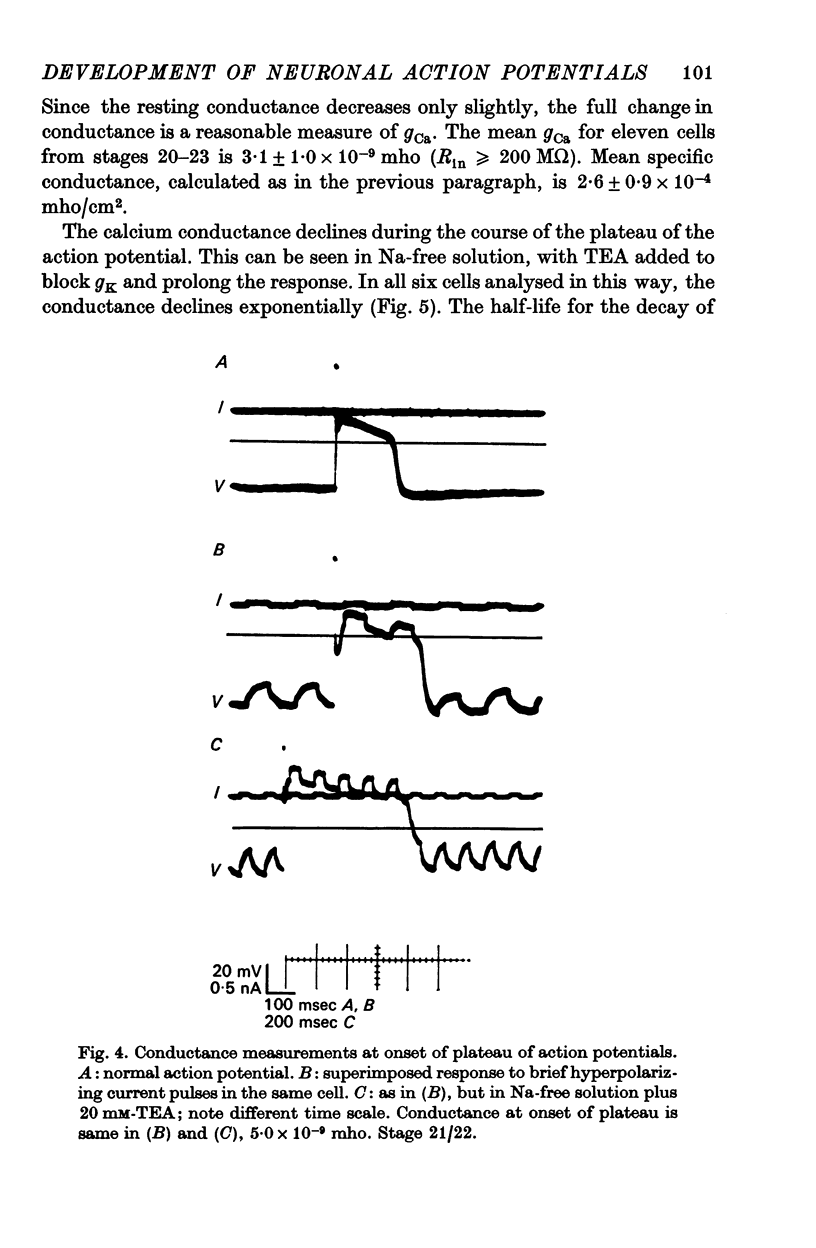
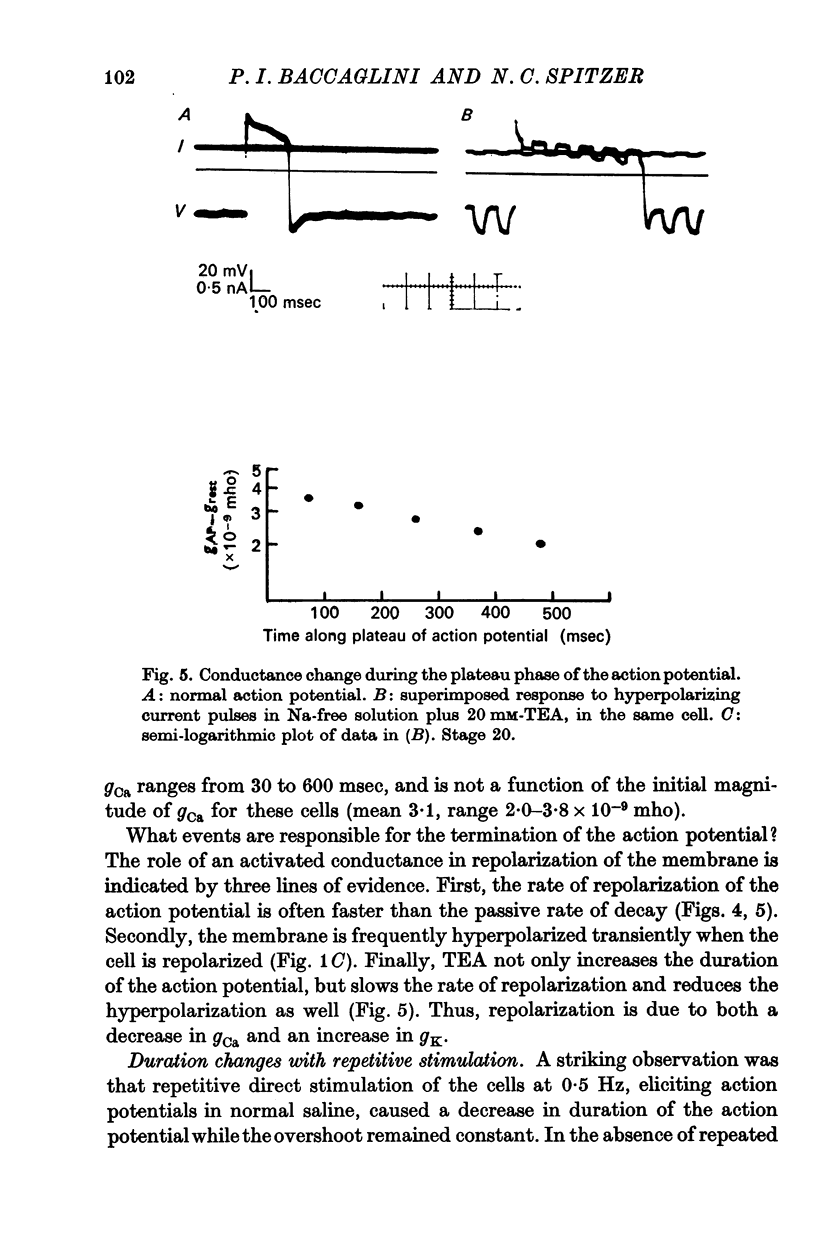
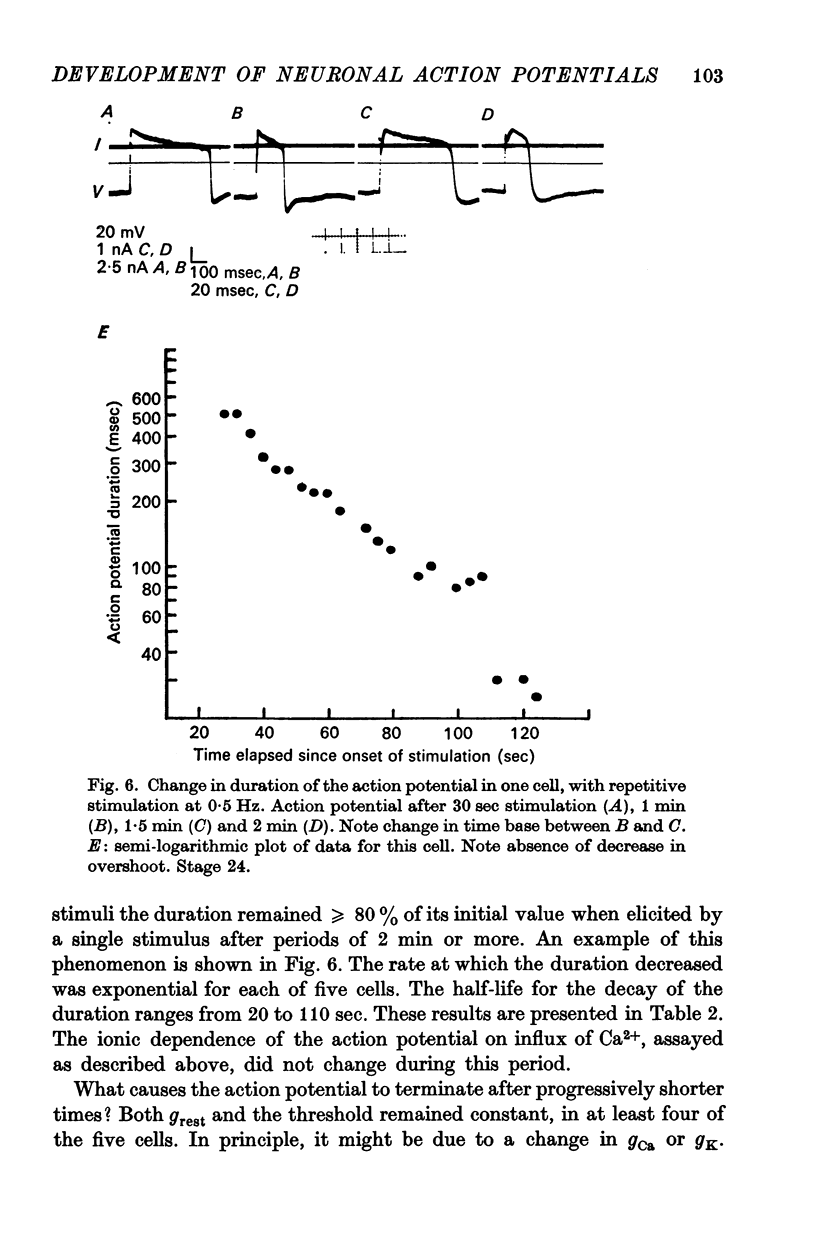
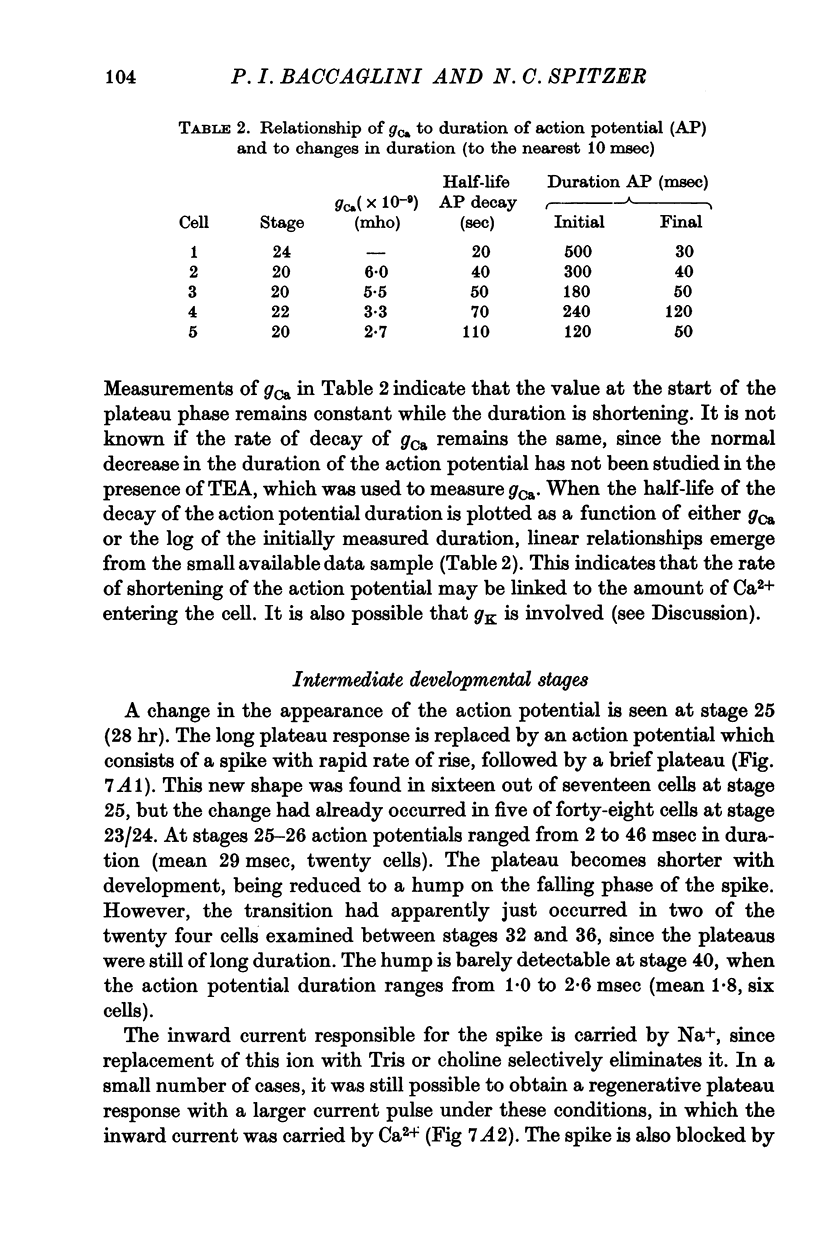
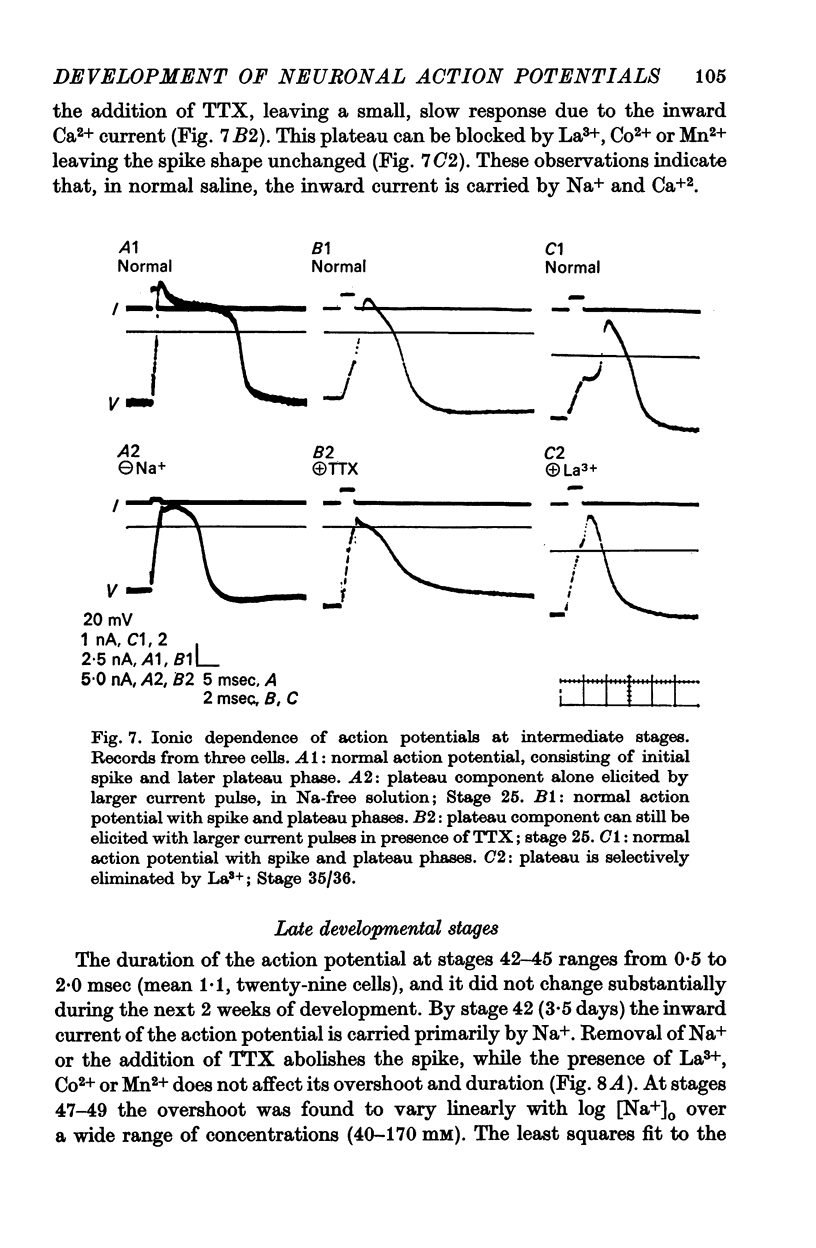
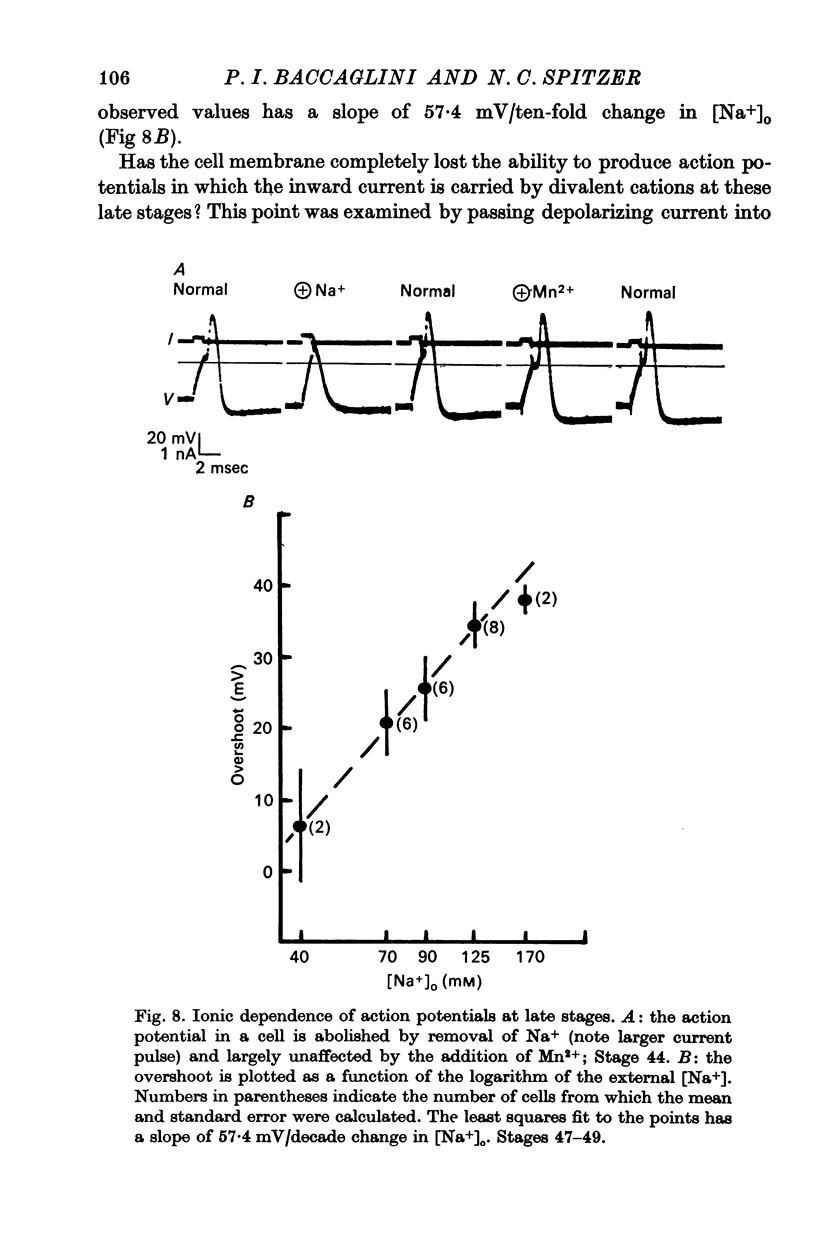
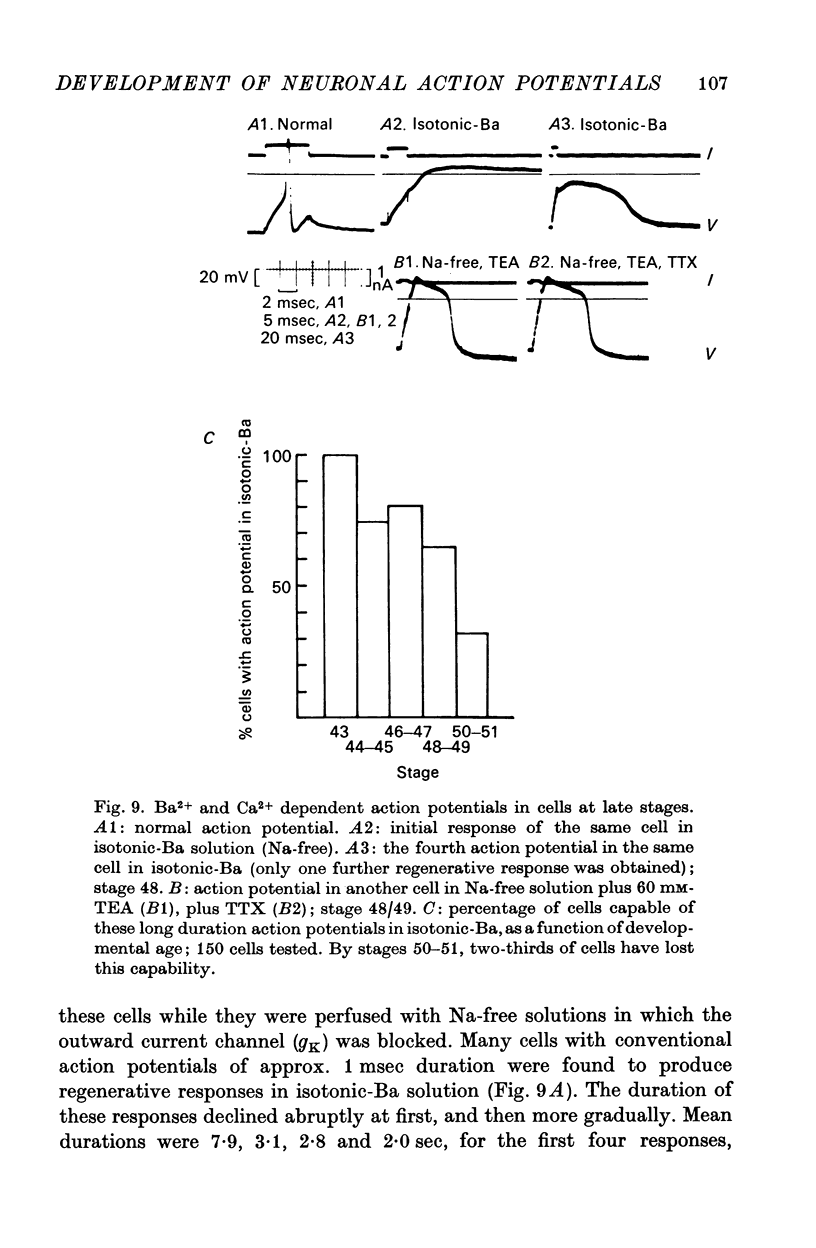
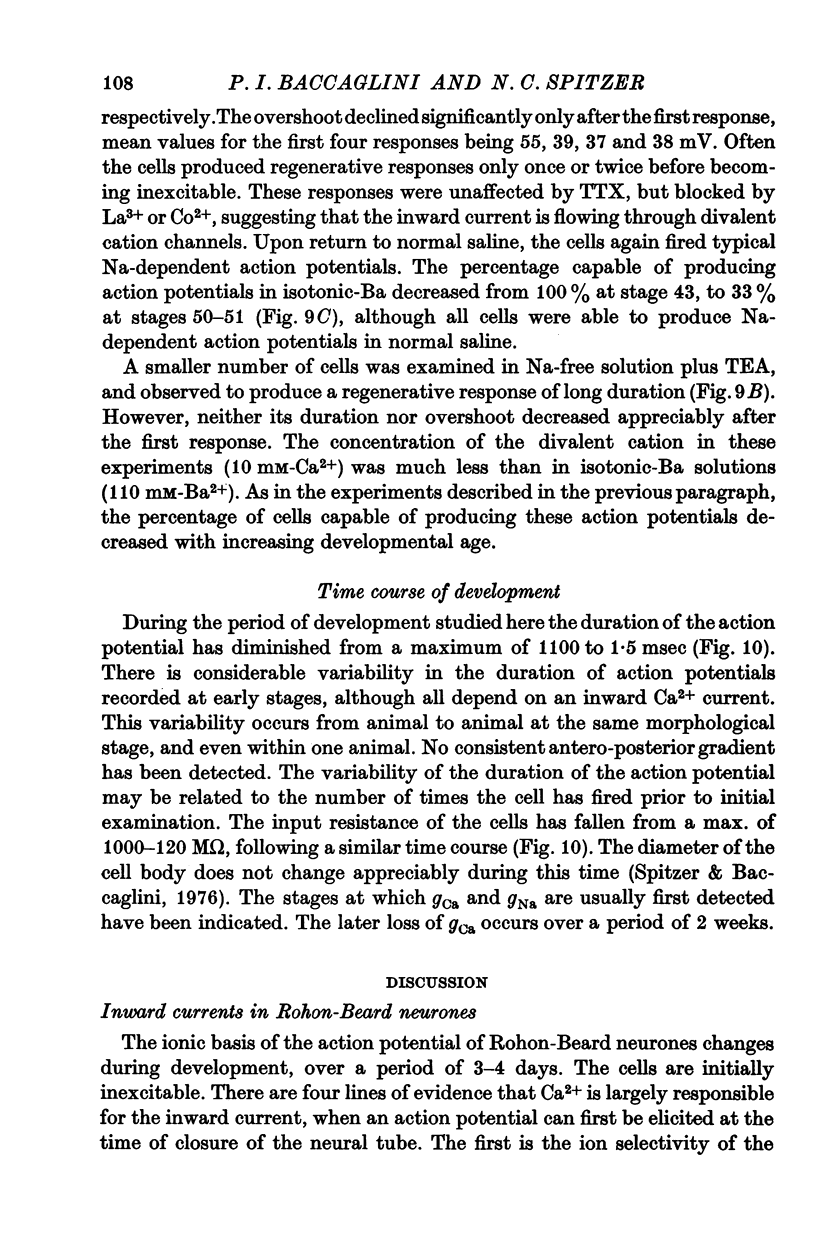
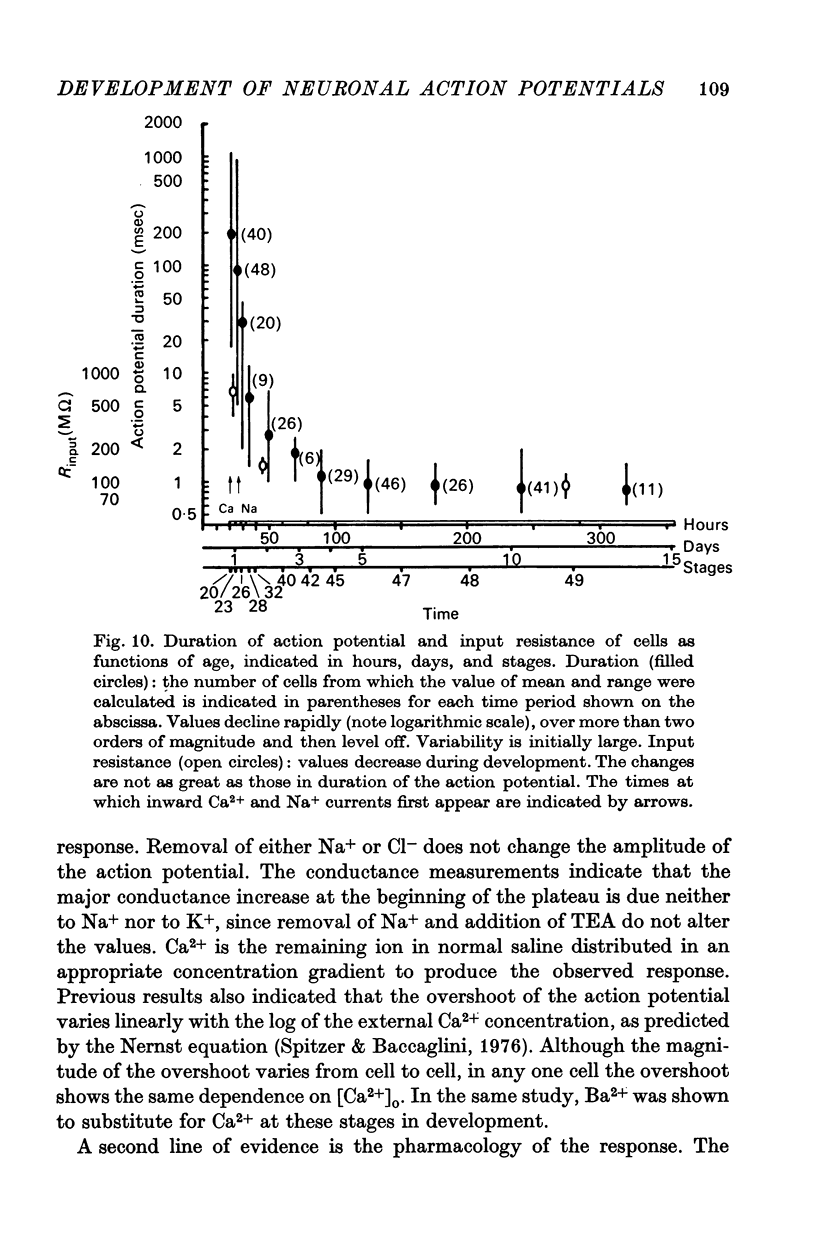
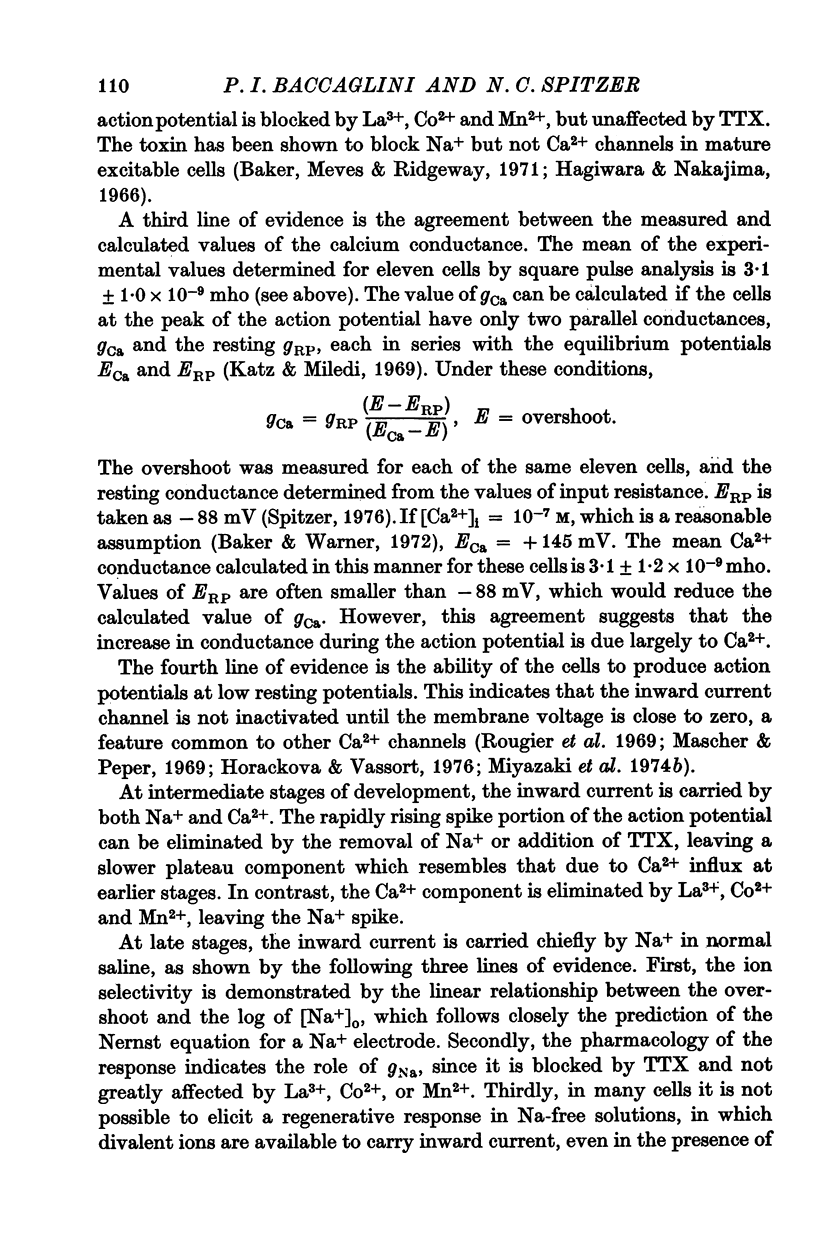
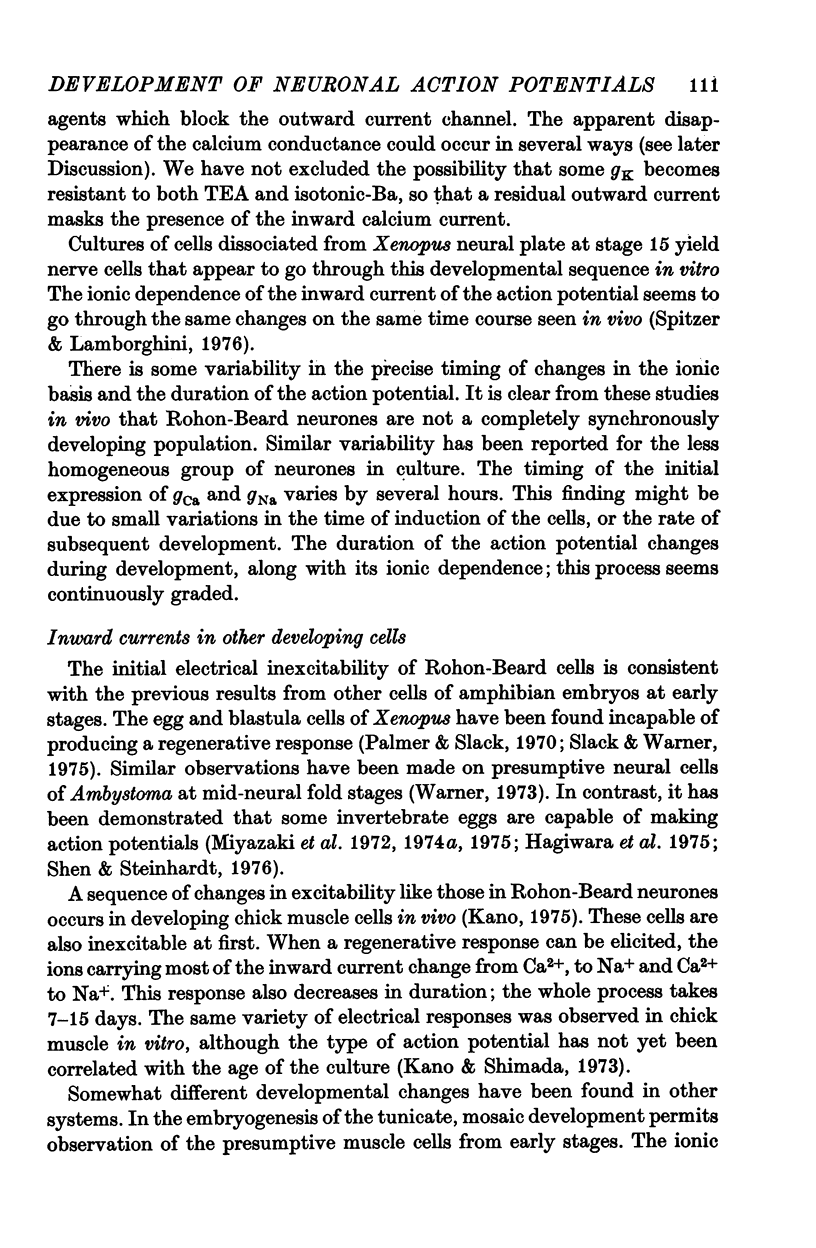
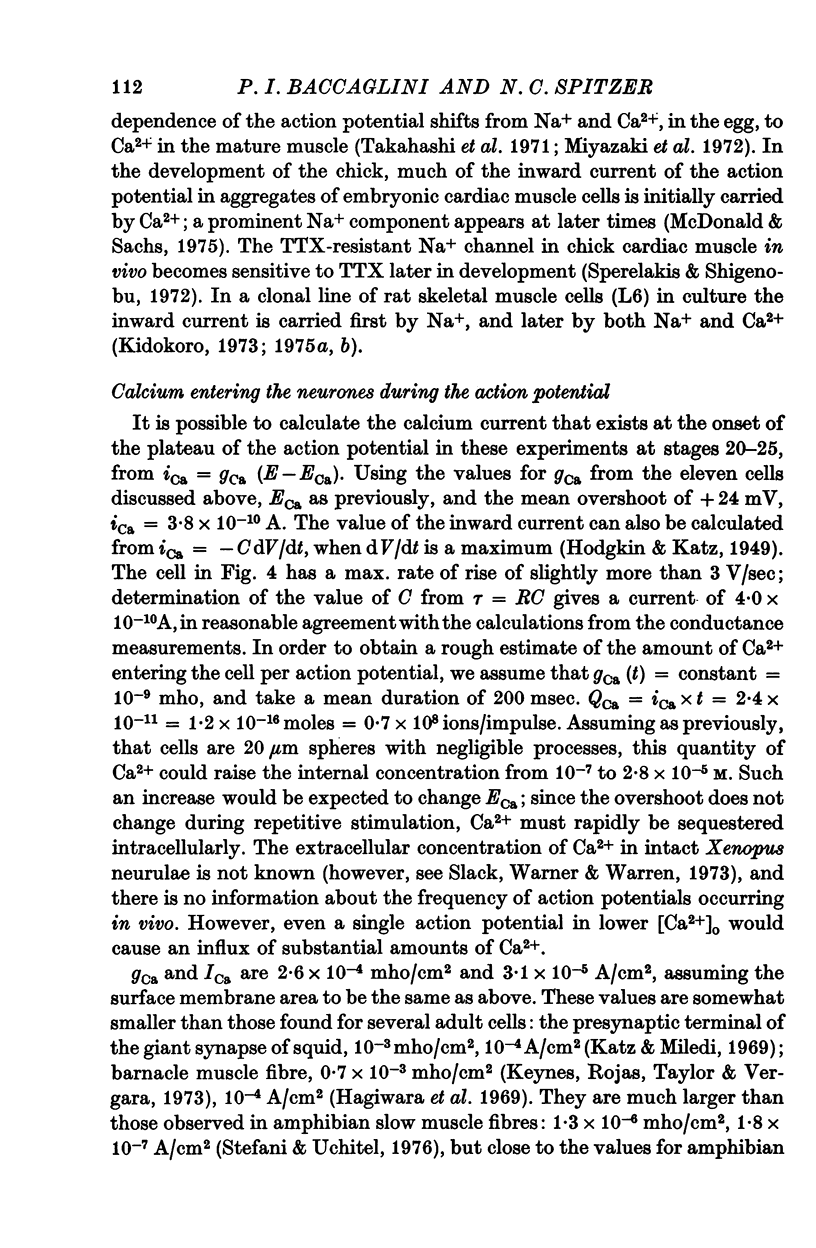
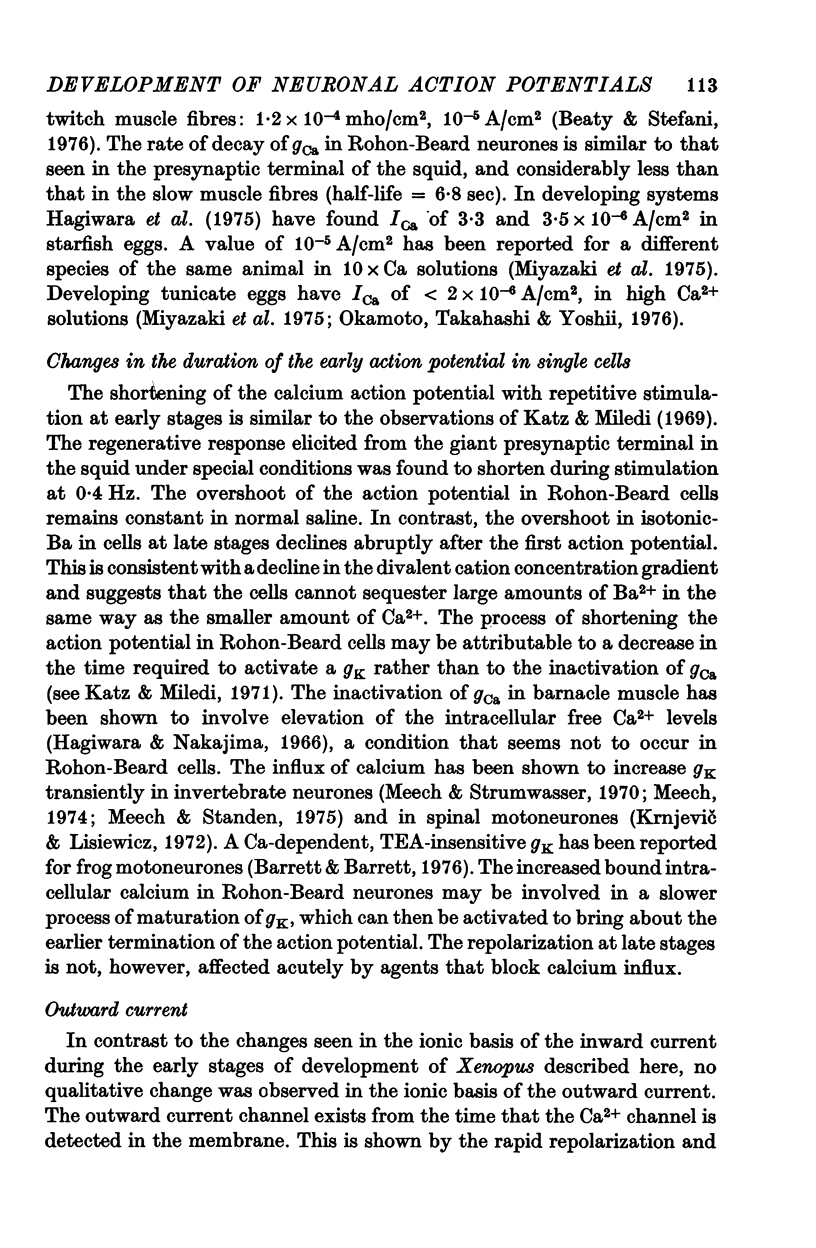
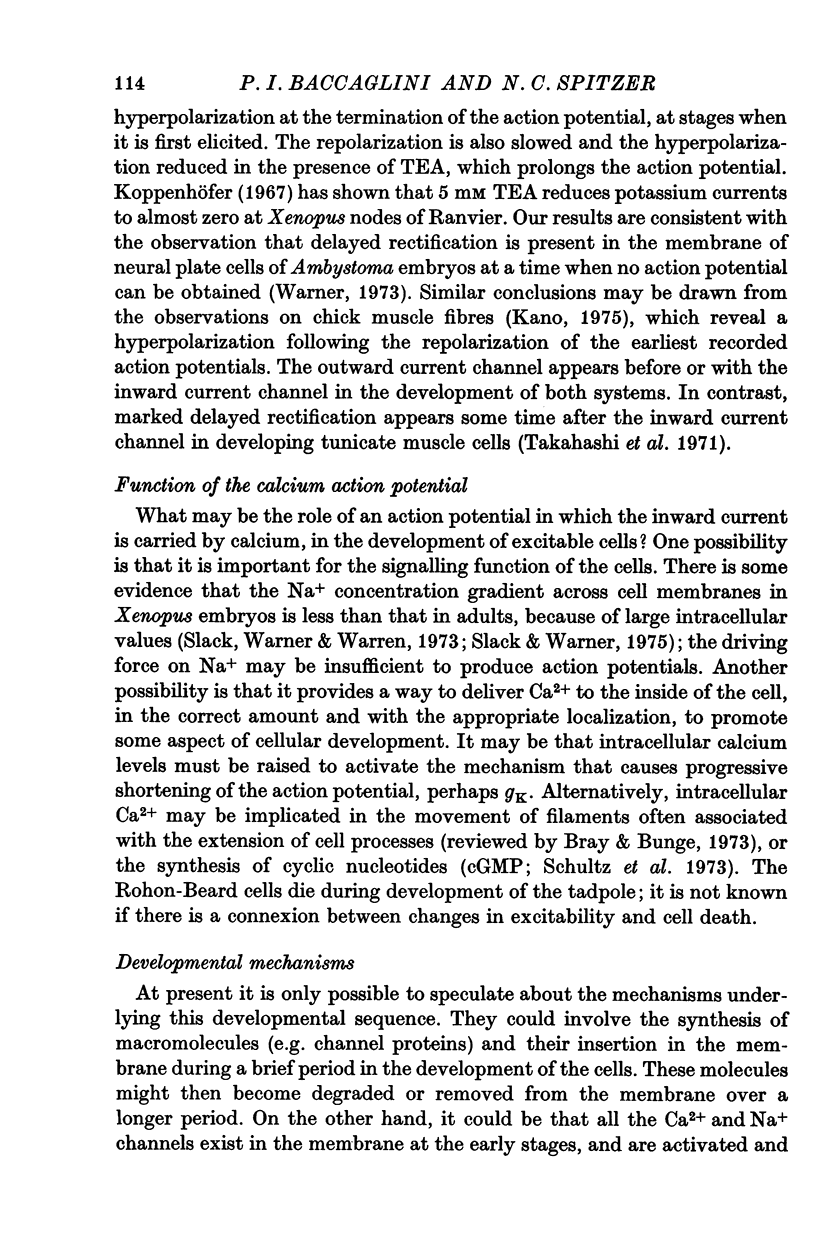
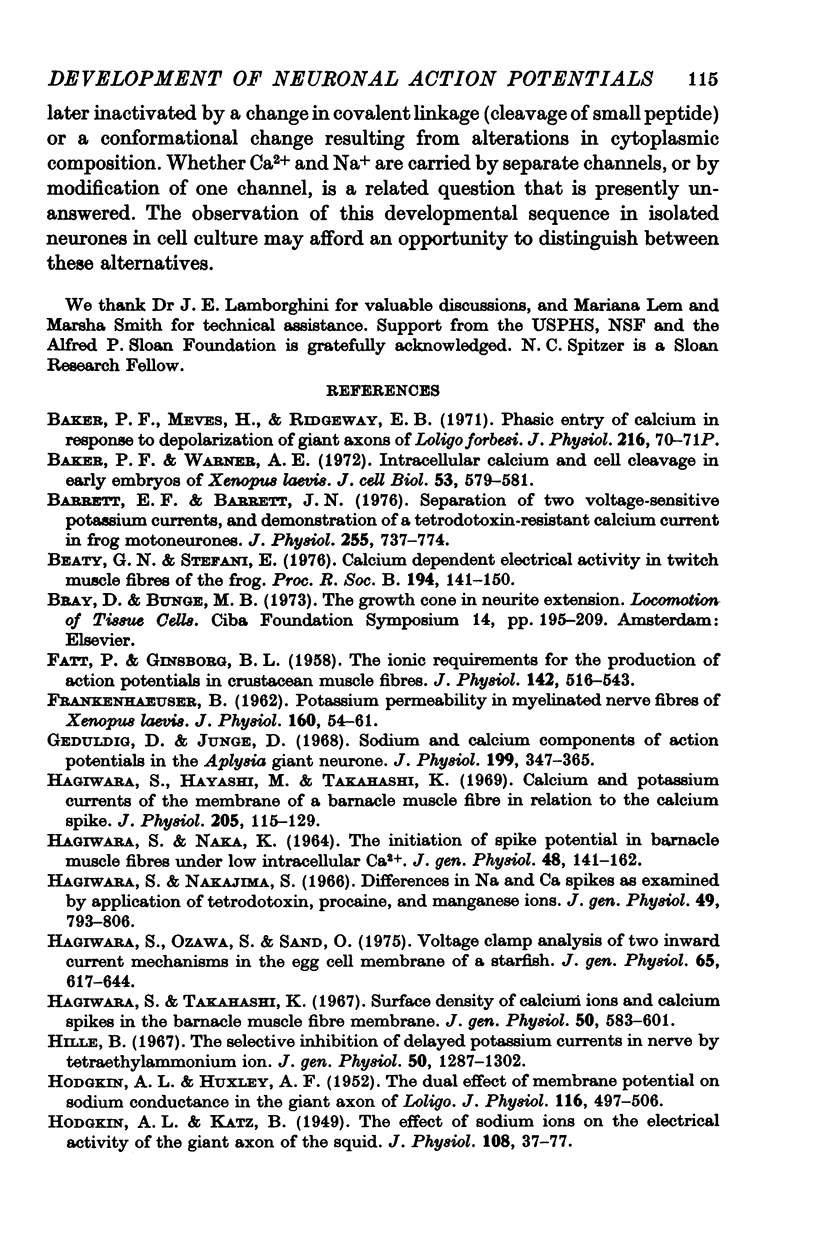
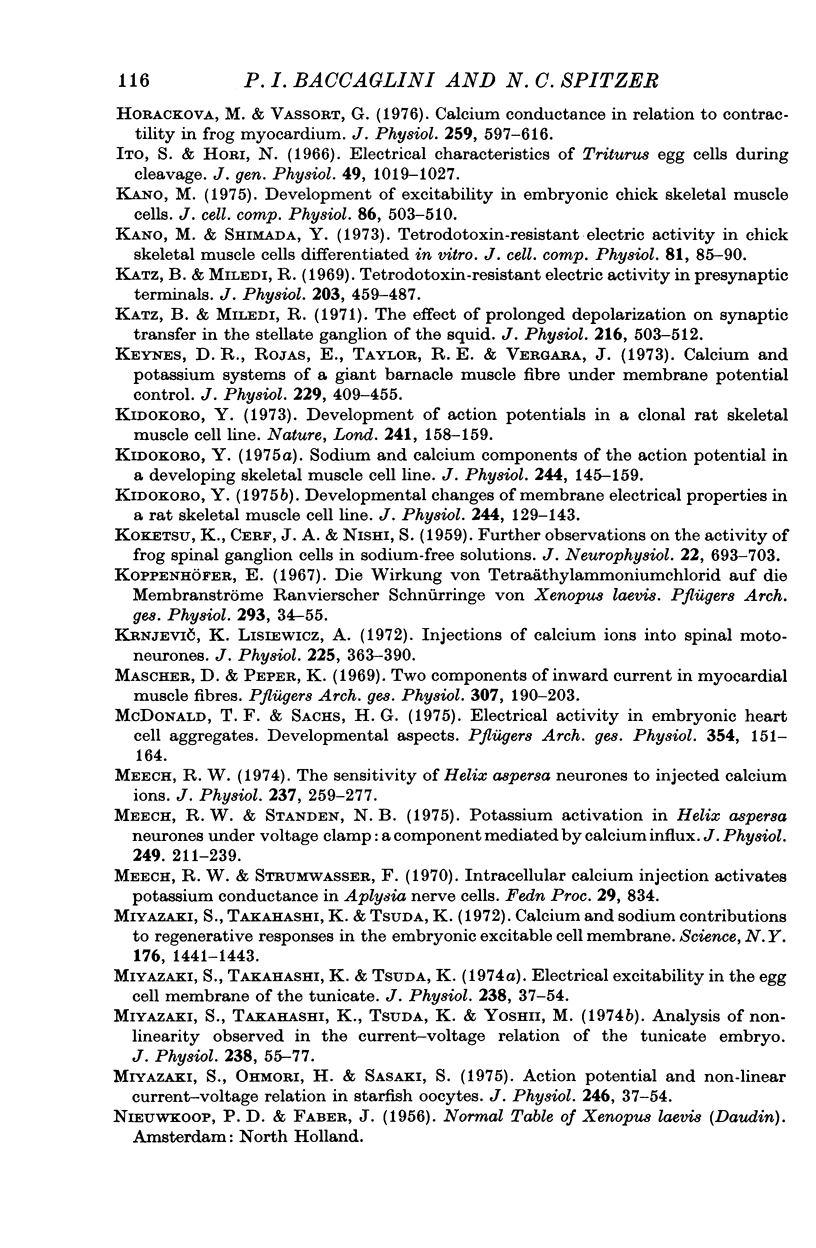
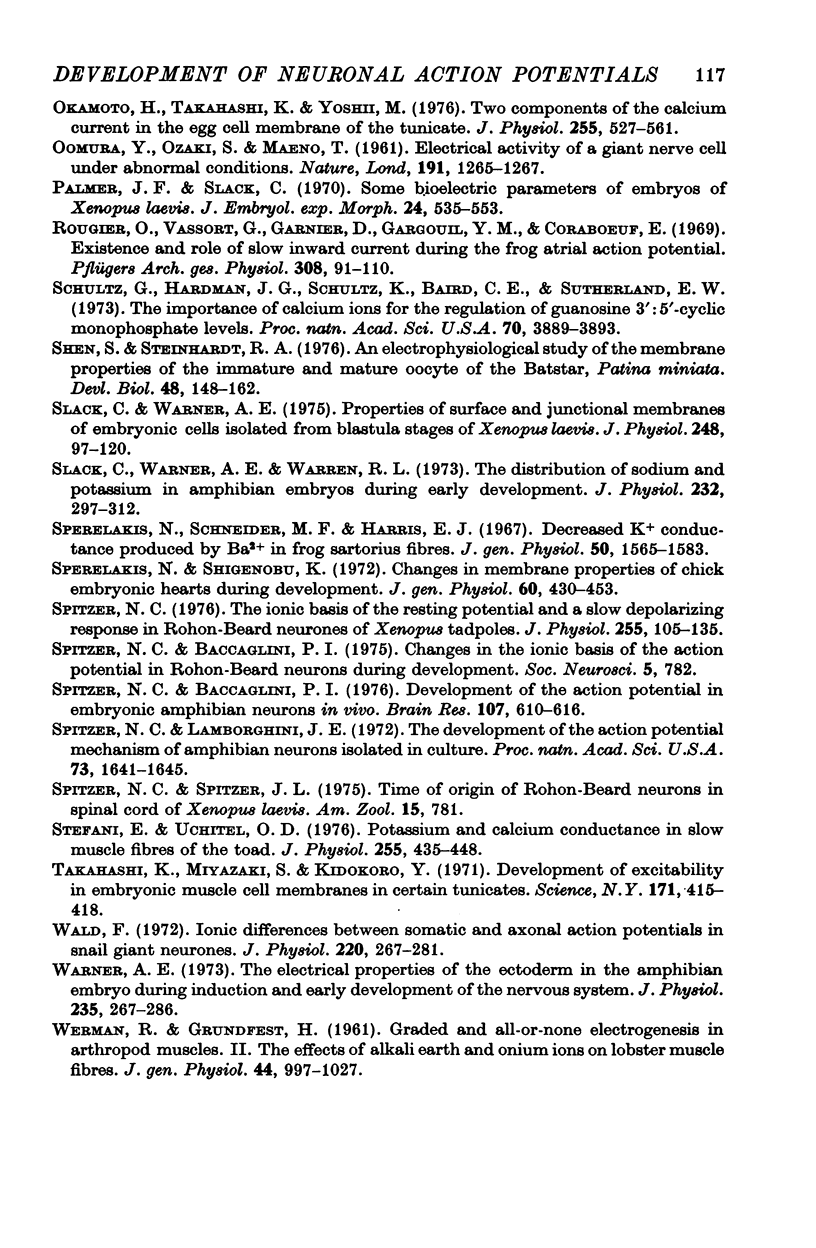
Selected References
These references are in PubMed. This may not be the complete list of references from this article.
- Baker P. F., Meves H., Ridgway E. B. Phasic entry of calcium in response to depolarization of giant axons of Loligo forbesi. J Physiol. 1971 Jul;216(2):70P–71P. [PubMed] [Google Scholar]
- Beaty G. N., Stefani E. Calcium dependent electrical activity in twitch muscle fibres of the frog. Proc R Soc Lond B Biol Sci. 1976 Aug 27;194(1114):141–150. doi: 10.1098/rspb.1976.0070. [DOI] [PubMed] [Google Scholar]
- Bray D., Bunge M. B. The growth cone in neurite extension. Ciba Found Symp. 1973;14:195–209. doi: 10.1002/9780470719978.ch9. [DOI] [PubMed] [Google Scholar]
- FRANKENHAEUSER B. Potassium permeability in myelinated nerve fibres of Xenopus laevis. J Physiol. 1962 Jan;160:54–61. doi: 10.1113/jphysiol.1962.sp006834. [DOI] [PMC free article] [PubMed] [Google Scholar]
- Geduldig D., Junge D. Sodium and calcium components of action potentials in the Aplysia giant neurone. J Physiol. 1968 Dec;199(2):347–365. doi: 10.1113/jphysiol.1968.sp008657. [DOI] [PMC free article] [PubMed] [Google Scholar]
- HAGIWARA S., NAKA K. I. THE INITIATION OF SPIKE POTENTIAL IN BARNACLE MUSCLE FIBERS UNDER LOW INTRACELLULAR CA++. J Gen Physiol. 1964 Sep;48:141–162. doi: 10.1085/jgp.48.1.141. [DOI] [PMC free article] [PubMed] [Google Scholar]
- HODGKIN A. L., HUXLEY A. F. The dual effect of membrane potential on sodium conductance in the giant axon of Loligo. J Physiol. 1952 Apr;116(4):497–506. doi: 10.1113/jphysiol.1952.sp004719. [DOI] [PMC free article] [PubMed] [Google Scholar]
- HODGKIN A. L., KATZ B. The effect of sodium ions on the electrical activity of giant axon of the squid. J Physiol. 1949 Mar 1;108(1):37–77. doi: 10.1113/jphysiol.1949.sp004310. [DOI] [PMC free article] [PubMed] [Google Scholar]
- Hagiwara S., Hayashi H., Takahashi K. Calcium and potassium currents of the membrane of a barnacle muscle fibre in relation to the calcium spike. J Physiol. 1969 Nov;205(1):115–129. doi: 10.1113/jphysiol.1969.sp008955. [DOI] [PMC free article] [PubMed] [Google Scholar]
- Hagiwara S., Nakajima S. Differences in Na and Ca spikes as examined by application of tetrodotoxin, procaine, and manganese ions. J Gen Physiol. 1966 Mar;49(4):793–806. doi: 10.1085/jgp.49.4.793. [DOI] [PMC free article] [PubMed] [Google Scholar]
- Hagiwara S., Ozawa S., Sand O. Voltage clamp analysis of two inward current mechanisms in the egg cell membrane of a starfish. J Gen Physiol. 1975 May;65(5):617–644. doi: 10.1085/jgp.65.5.617. [DOI] [PMC free article] [PubMed] [Google Scholar]
- Hagiwara S., Takahashi K. Surface density of calcium ions and calcium spikes in the barnacle muscle fiber membrane. J Gen Physiol. 1967 Jan;50(3):583–601. doi: 10.1085/jgp.50.3.583. [DOI] [PMC free article] [PubMed] [Google Scholar]
- Hille B. The selective inhibition of delayed potassium currents in nerve by tetraethylammonium ion. J Gen Physiol. 1967 May;50(5):1287–1302. doi: 10.1085/jgp.50.5.1287. [DOI] [PMC free article] [PubMed] [Google Scholar]
- Horackova M., Vassort G. Calcium conductance in relation to contractility in frog myocardium. J Physiol. 1976 Aug;259(3):597–616. doi: 10.1113/jphysiol.1976.sp011485. [DOI] [PMC free article] [PubMed] [Google Scholar]
- Ito S., Hori N. Electrical characteristics of Triturus egg cells during cleavage. J Gen Physiol. 1966 May;49(5):1019–1027. doi: 10.1085/jgp.49.5.1019. [DOI] [PMC free article] [PubMed] [Google Scholar]
- KOKETSU K., CERF J. A., NISHI S. Further observations on electrical activity of frog spinal ganglion cells in sodium-free solutions. J Neurophysiol. 1959 Nov;22:693–703. doi: 10.1152/jn.1959.22.6.693. [DOI] [PubMed] [Google Scholar]
- Kano M. Development of excitability in embryonic chick skeletal muscle cells. J Cell Physiol. 1975 Dec;86(3 Pt 1):503–510. doi: 10.1002/jcp.1040860307. [DOI] [PubMed] [Google Scholar]
- Kano M., Shimada Y. Tetrodotoxin-resistant electric activity in chick skeletal muscle cells differentiated in vitro. J Cell Physiol. 1973 Feb;81(1):85–89. doi: 10.1002/jcp.1040810110. [DOI] [PubMed] [Google Scholar]
- Katz B., Miledi R. Tetrodotoxin-resistant electric activity in presynaptic terminals. J Physiol. 1969 Aug;203(2):459–487. doi: 10.1113/jphysiol.1969.sp008875. [DOI] [PMC free article] [PubMed] [Google Scholar]
- Katz B., Miledi R. The effect of prolonged depolarization on synaptic transfer in the stellate ganglion of the squid. J Physiol. 1971 Jul;216(2):503–512. doi: 10.1113/jphysiol.1971.sp009537. [DOI] [PMC free article] [PubMed] [Google Scholar]
- Keynes R. D., Rojas E., Taylor R. E., Vergara J. Calcium and potassium systems of a giant barnacle muscle fibre under membrane potential control. J Physiol. 1973 Mar;229(2):409–455. doi: 10.1113/jphysiol.1973.sp010146. [DOI] [PMC free article] [PubMed] [Google Scholar]
- Kidokoro Y. Development of action potentials in a clonal rat skeletal muscle cell line. Nat New Biol. 1973 Jan 31;241(109):158–159. doi: 10.1038/newbio241158a0. [DOI] [PubMed] [Google Scholar]
- Kidokoro Y. Developmental changes of membrane electrical properties in a rat skeletal muscle cell line. J Physiol. 1975 Jan;244(1):129–143. doi: 10.1113/jphysiol.1975.sp010787. [DOI] [PMC free article] [PubMed] [Google Scholar]
- Kidokoro Y. Sodium and calcium components of the action potential in a developing skeletal muscle cell line. J Physiol. 1975 Jan;244(1):145–159. doi: 10.1113/jphysiol.1975.sp010788. [DOI] [PMC free article] [PubMed] [Google Scholar]
- Mascher D., Peper K. Two components of inward current in myocardial muscle fibers. Pflugers Arch. 1969;307(3):190–203. doi: 10.1007/BF00592084. [DOI] [PubMed] [Google Scholar]
- McDonald T. F., Sachs H. G. Electrical activity in embryonic heart cell aggregates. Developmental aspects. Pflugers Arch. 1975;354(2):151–164. doi: 10.1007/BF00579945. [DOI] [PubMed] [Google Scholar]
- Meech R. W., Standen N. B. Potassium activation in Helix aspersa neurones under voltage clamp: a component mediated by calcium influx. J Physiol. 1975 Jul;249(2):211–239. doi: 10.1113/jphysiol.1975.sp011012. [DOI] [PMC free article] [PubMed] [Google Scholar]
- Meech R. W. The sensitivity of Helix aspersa neurones to injected calcium ions. J Physiol. 1974 Mar;237(2):259–277. doi: 10.1113/jphysiol.1974.sp010481. [DOI] [PMC free article] [PubMed] [Google Scholar]
- Miyazaki S. I., Ohmori H., Sasaki S. Action potential and non-linear current-voltage relation in starfish oocytes. J Physiol. 1975 Mar;246(1):37–54. doi: 10.1113/jphysiol.1975.sp010879. [DOI] [PMC free article] [PubMed] [Google Scholar]
- Miyazaki S. I., Takahashi K., Tsuda K. Electrical excitability in the egg cell membrane of the tunicate. J Physiol. 1974 Apr;238(1):37–54. doi: 10.1113/jphysiol.1974.sp010509. [DOI] [PMC free article] [PubMed] [Google Scholar]
- Miyazaki S. I., Takahashi K., Tsuda K., Yoshii M. Analysis of non-linearity observed in the current-voltage relation of the tunicate embryo. J Physiol. 1974 Apr;238(1):55–77. doi: 10.1113/jphysiol.1974.sp010510. [DOI] [PMC free article] [PubMed] [Google Scholar]
- Miyazaki S., Takahashi K., Tsuda K. Calcium and sodium contributions to regenerative responses in the embryonic excitable cell membrane. Science. 1972 Jun 30;176(4042):1441–1443. doi: 10.1126/science.176.4042.1441. [DOI] [PubMed] [Google Scholar]
- OOMURA Y., OZAKI S., MAENO T. Electrical activity of a giant nerve cell under abnormal conditions. Nature. 1961 Sep 23;191:1265–1267. doi: 10.1038/1911265a0. [DOI] [PubMed] [Google Scholar]
- Okamoto H., Takahashi K., Yoshii M. Two components of the calcium current in the egg cell membrane of the tunicate. J Physiol. 1976 Feb;255(2):527–561. doi: 10.1113/jphysiol.1976.sp011294. [DOI] [PMC free article] [PubMed] [Google Scholar]
- Palmer J. F., Slack C. Some bio-electric parameters of early Xenopus embryos. J Embryol Exp Morphol. 1970 Nov;24(3):535–553. [PubMed] [Google Scholar]
- Rougier O., Vassort G., Garnier D., Gargouil Y. M., Coraboeuf E. Existence and role of a slow inward current during the frog atrial action potential. Pflugers Arch. 1969;308(2):91–110. doi: 10.1007/BF00587018. [DOI] [PubMed] [Google Scholar]
- Schultz G., Hardman J. G., Schultz K., Baird C. E., Sutherland E. W. The importance of calcium ions for the regulation of guanosine 3':5'-cyclic monophosphage levels. Proc Natl Acad Sci U S A. 1973 Dec;70(12):3889–3893. doi: 10.1073/pnas.70.12.3889. [DOI] [PMC free article] [PubMed] [Google Scholar]
- Shen S., Steinhardt R. A. An electrophysiological study of the membrane properties of the immature and mature oocyte of the batstar, Patiria miniata. Dev Biol. 1976 Jan;48(1):148–162. doi: 10.1016/0012-1606(76)90053-1. [DOI] [PubMed] [Google Scholar]
- Slack C., Warner A. E. Properties of surface and junctional membranes of embryonic cells isolated from blastula stages of Xenopus laevis. J Physiol. 1975 Jun;248(1):97–120. doi: 10.1113/jphysiol.1975.sp010964. [DOI] [PMC free article] [PubMed] [Google Scholar]
- Slack C., Warner A. E., Warren R. L. The distribution of sodium and potassium in amphibian embryos during early development. J Physiol. 1973 Jul;232(2):297–312. doi: 10.1113/jphysiol.1973.sp010271. [DOI] [PMC free article] [PubMed] [Google Scholar]
- Sperelakis N., Schneider M. F., Harris E. J. Decreased K+ conductance produced by Ba++ in frog sartorius fibers. J Gen Physiol. 1967 Jul;50(6):1565–1583. doi: 10.1085/jgp.50.6.1565. [DOI] [PMC free article] [PubMed] [Google Scholar]
- Sperelakis N., Shigenobu K. Changes in membrane properties of chick embryonic hearts during development. J Gen Physiol. 1972 Oct;60(4):430–453. doi: 10.1085/jgp.60.4.430. [DOI] [PMC free article] [PubMed] [Google Scholar]
- Spitzer N. C., Baccaglini P. I. Development of the action potential in embryo amphibian neurons in vivo. Brain Res. 1976 May 14;107(3):610–616. doi: 10.1016/0006-8993(76)90148-7. [DOI] [PubMed] [Google Scholar]
- Spitzer N. C., Lamborghini J. E. The development of the action potential mechanism of amphibian neurons isolated in culture. Proc Natl Acad Sci U S A. 1976 May;73(5):1641–1645. doi: 10.1073/pnas.73.5.1641. [DOI] [PMC free article] [PubMed] [Google Scholar]
- Spitzer N. C. The ionic basis of the resting potential and a slow depolarizing response in Rohon-Beard neurones of Xenopus tadpoles. J Physiol. 1976 Feb;255(1):105–135. doi: 10.1113/jphysiol.1976.sp011272. [DOI] [PMC free article] [PubMed] [Google Scholar]
- Stefani E., Uchitel O. D. Potassium and calcium conductance in slow muscle fibres of the toad. J Physiol. 1976 Feb;255(2):435–448. doi: 10.1113/jphysiol.1976.sp011288. [DOI] [PMC free article] [PubMed] [Google Scholar]
- Takahashi K., Miyazaki S. I., Kidokoro Y. Development of excitability in embryonic muscle cell membranes in certain tunicates. Science. 1971 Jan 29;171(3969):415–418. doi: 10.1126/science.171.3969.415. [DOI] [PubMed] [Google Scholar]
- WERMAN R., GRUNDFEST H. Graded and all-or-none electrogenesis in arthropod muscle. II. The effects of alkali-earth and onium ions on lobster muscle fibers. J Gen Physiol. 1961 May;44:997–1027. doi: 10.1085/jgp.44.5.997. [DOI] [PMC free article] [PubMed] [Google Scholar]
- Wald F. Ionic differences between somatic and axonal action potentials in snail giant neurones. J Physiol. 1972 Jan;220(2):267–281. doi: 10.1113/jphysiol.1972.sp009706. [DOI] [PMC free article] [PubMed] [Google Scholar]
- Warner A. E. The electrical properties of the ectoderm in the amphibian embryo during induction and early development of the nervous system. J Physiol. 1973 Nov;235(1):267–286. doi: 10.1113/jphysiol.1973.sp010387. [DOI] [PMC free article] [PubMed] [Google Scholar]