Abstract
1. Muscles with high intracellular sodium concentrations can extrude sodium into solutions which contain 10 m-equiv/l. of either potassium or rubidium. Potassium or rubidium replaces the extruded intracellular sodium. These cation movements take place equally well when the external anion is chloride or sulphate, though muscles deteriorate if left for long periods in sulphate solutions.
2. Measurements of intracellular potentials during extrusion of sodium into solutions containing potassium show:
(a) an internal potential more negative than the potassium equilibrium potential (EK); at 20° C the difference is nearly 20 mV.
(b) that a difference between the membrane potential and EK is dependent on temperature and is abolished by 10-5 M ouabain.
(c) an internal potential which becomes more negative in the presence of 0·1% cocaine, a concentration of cocaine which substantially increases the membrane resistance to potassium movement.
In the absence of potassium or rubidium no such hyperpolarization occurs.
3. When muscles extrude into solutions which contain rubidium they have internal potentials which are 10-20 mV more negative than when extruding sodium into corresponding solutions containing potassium.
4. Measurements of electrical conductance in the potassium solution suggest that the electrochemical potential difference for potassium ions may be large enough to account for the measured inward potassium movements during sodium extrusion. The reliability of the measurements does not, however, exclude the possibility that some part of the inward potassium movement is chemically linked to outward movement.
5. Measurements of membrane conductance in solutions containing rubidium, and of net movements of rubidium in the presence and absence of ouabain, lead to the conclusion that at least 90% of the inward rubidium movement during sodium extrusion must be chemically linked to the sodium movement.
6. The hyperpolarization during extrusion of sodium could be explained on the basis of a fall of the potassium or rubidium concentration in a region of the extracellular space immediately external to the membrane. It is argued that certain characteristics of the hyperpolarization make it difficult to explain the hyperpolarization on this basis alone, though some part of it may be due to extracellular depletion of either potassium or rubidium.
The main conclusion is that the sodium pump is capable of transferring electric charge across the membrane in which it is operating, but that, in a given time, the net charge transferred is less than the charge on the sodium ions that the pump has transported, by an amount that corresponds to the charge on the potassium or rubidium ions chemically transported by the pump.
Full text
PDF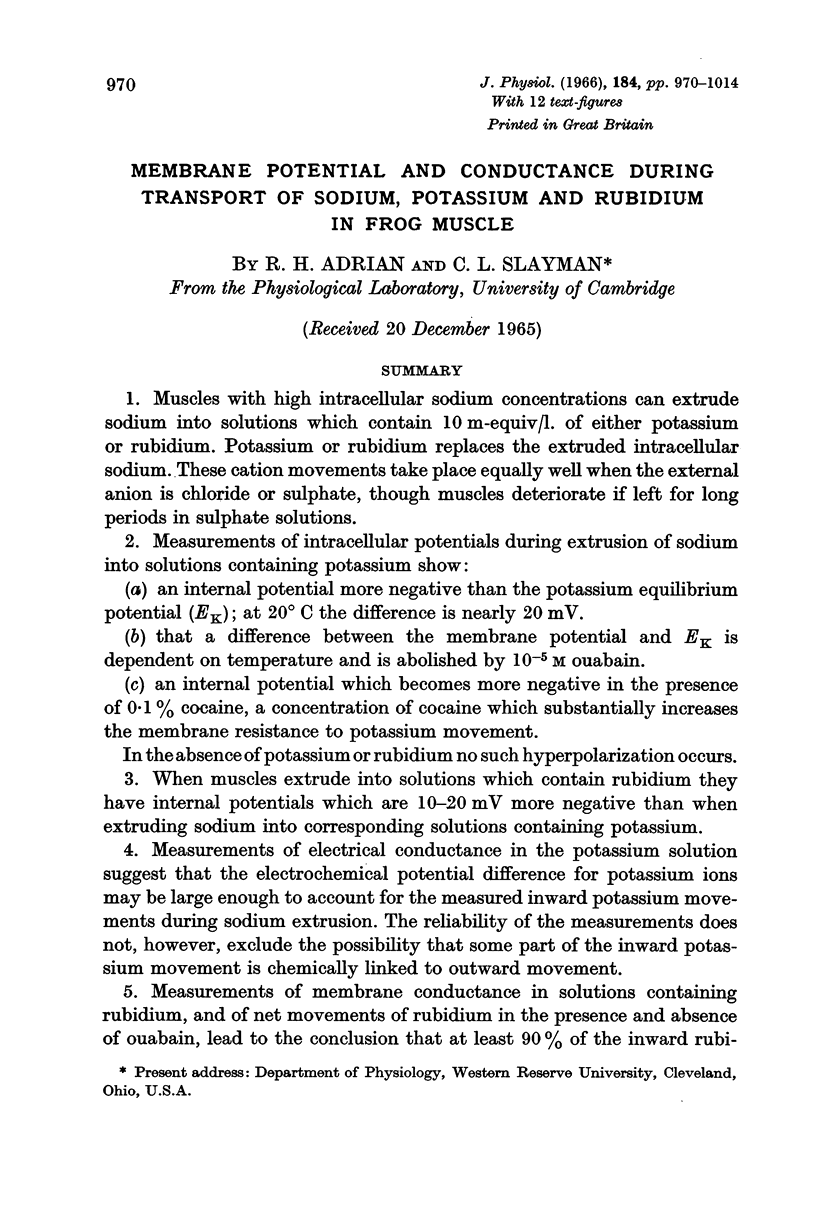
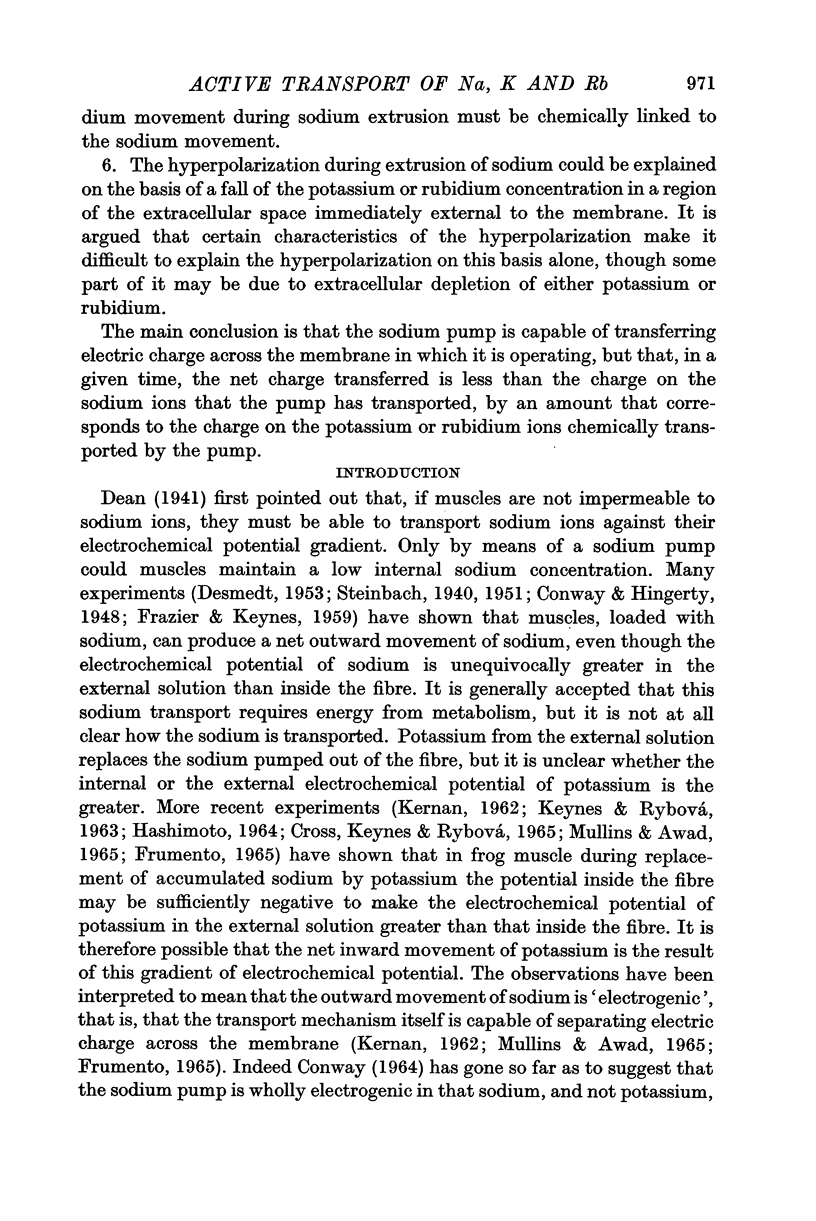
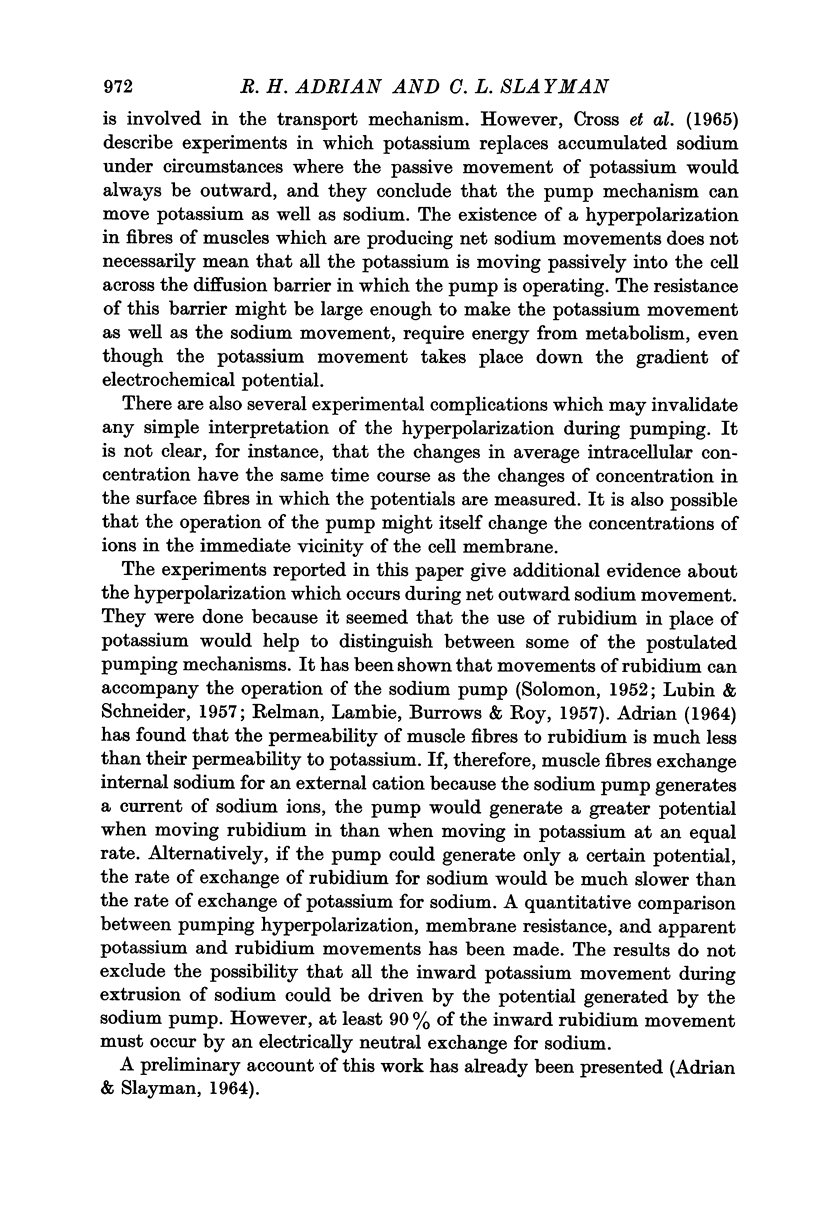
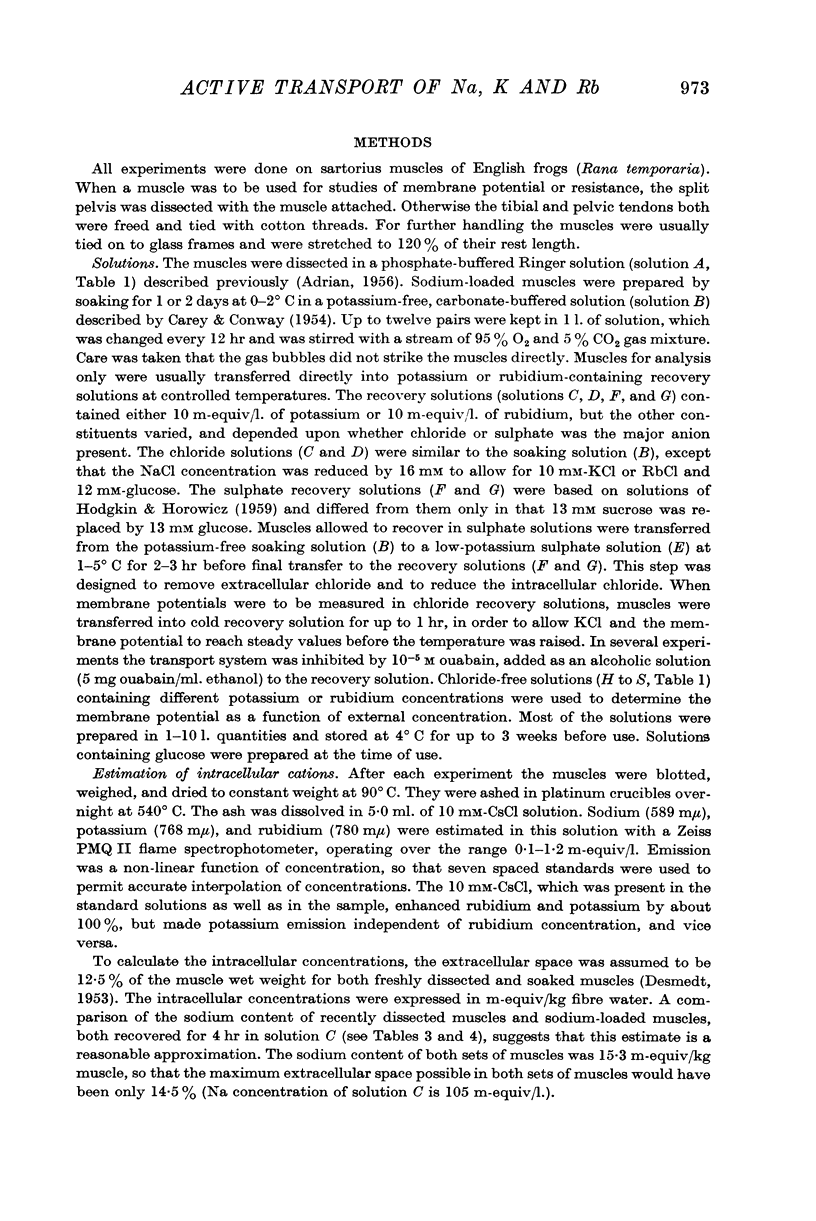
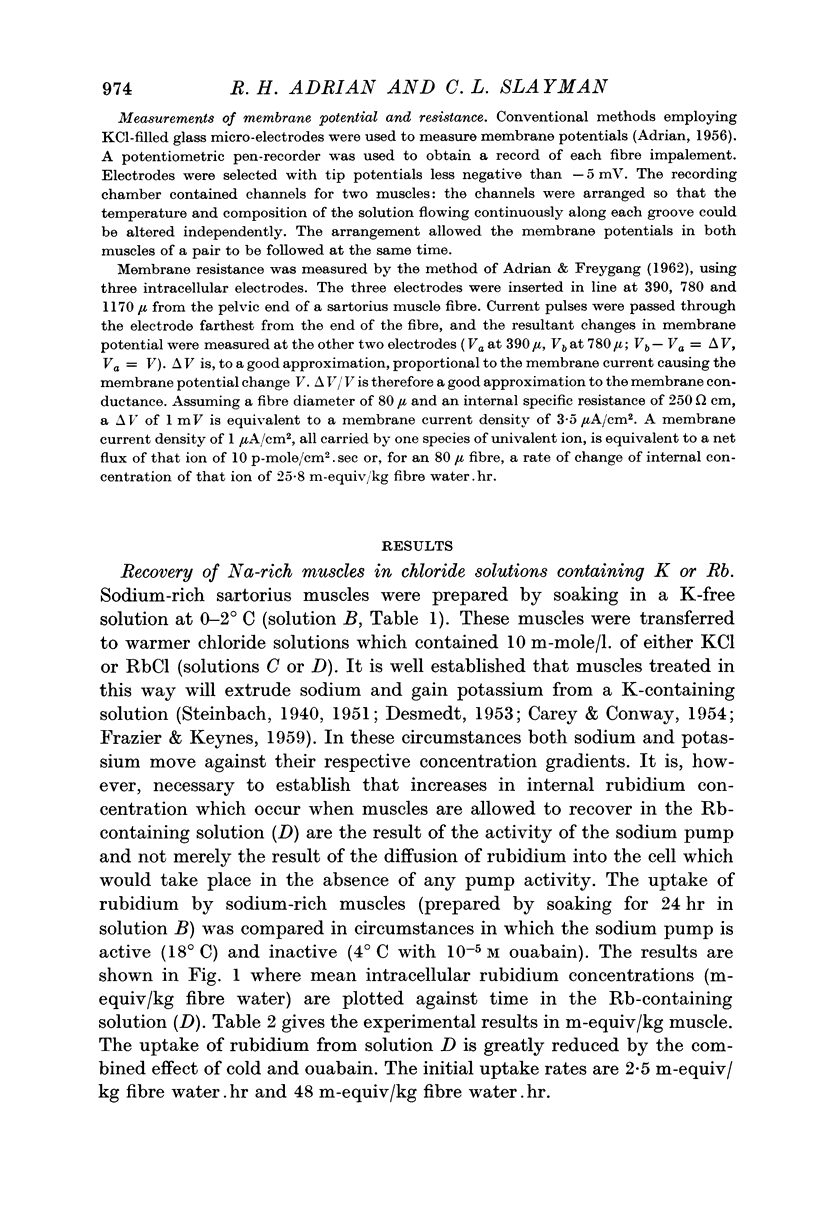
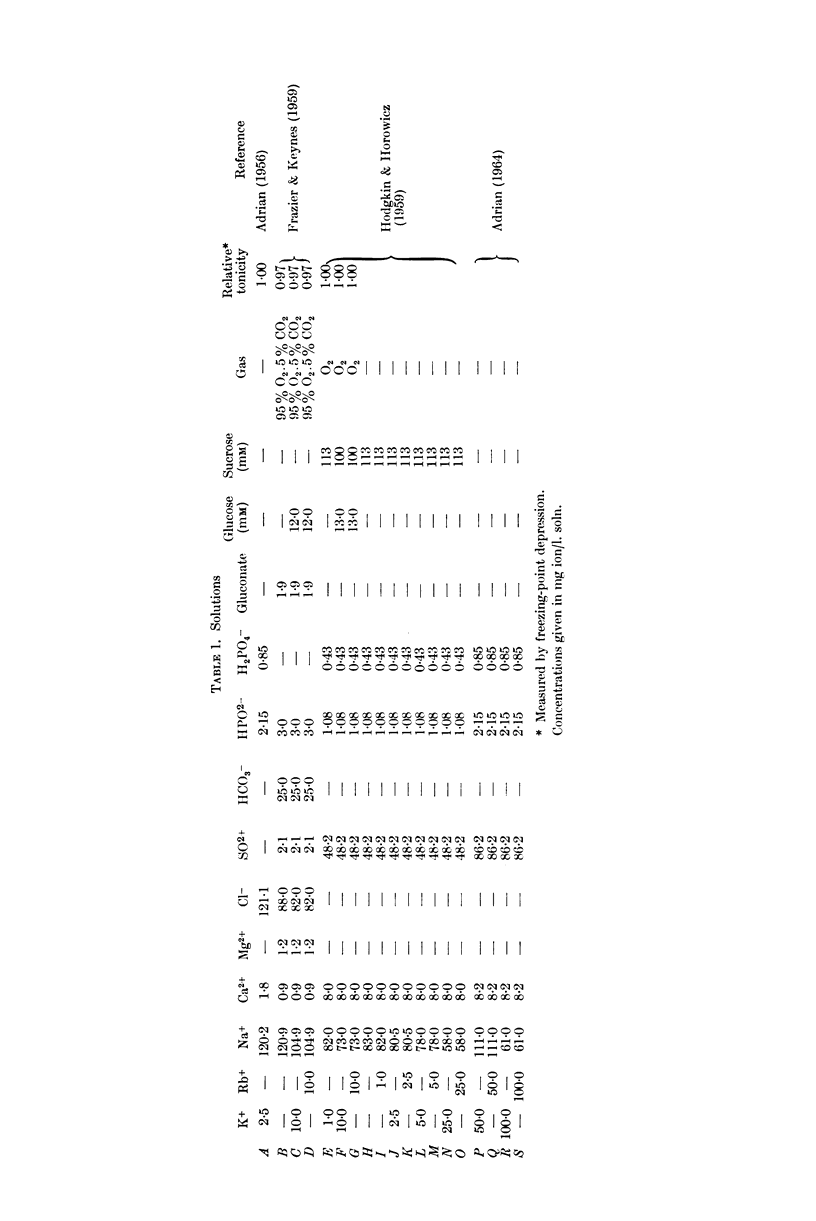
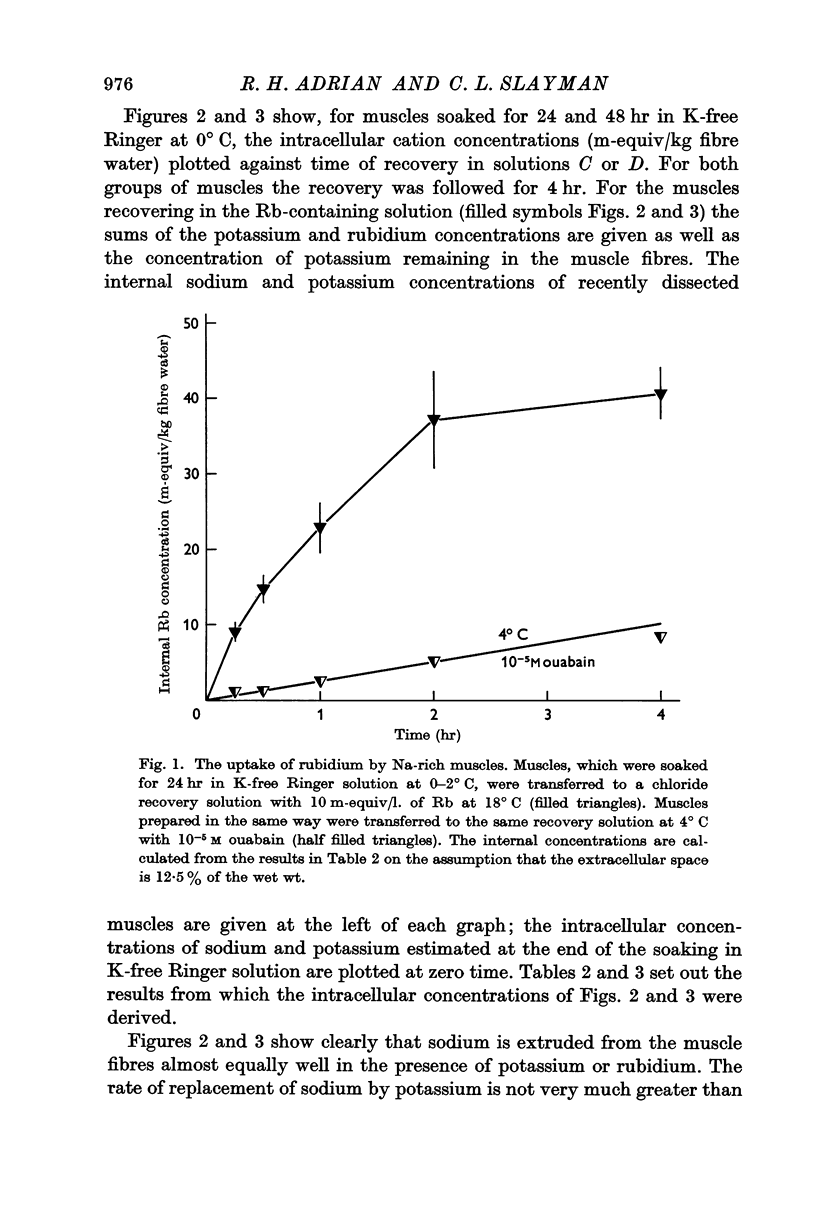
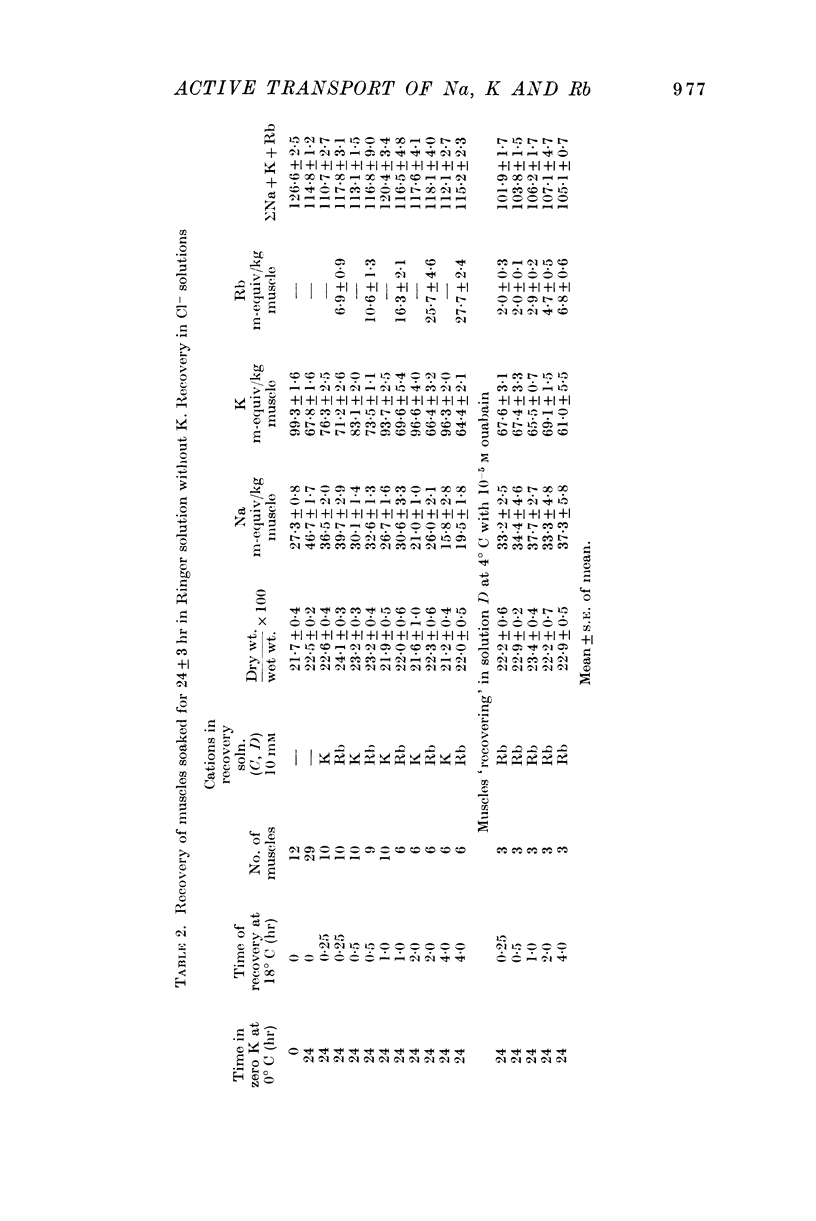
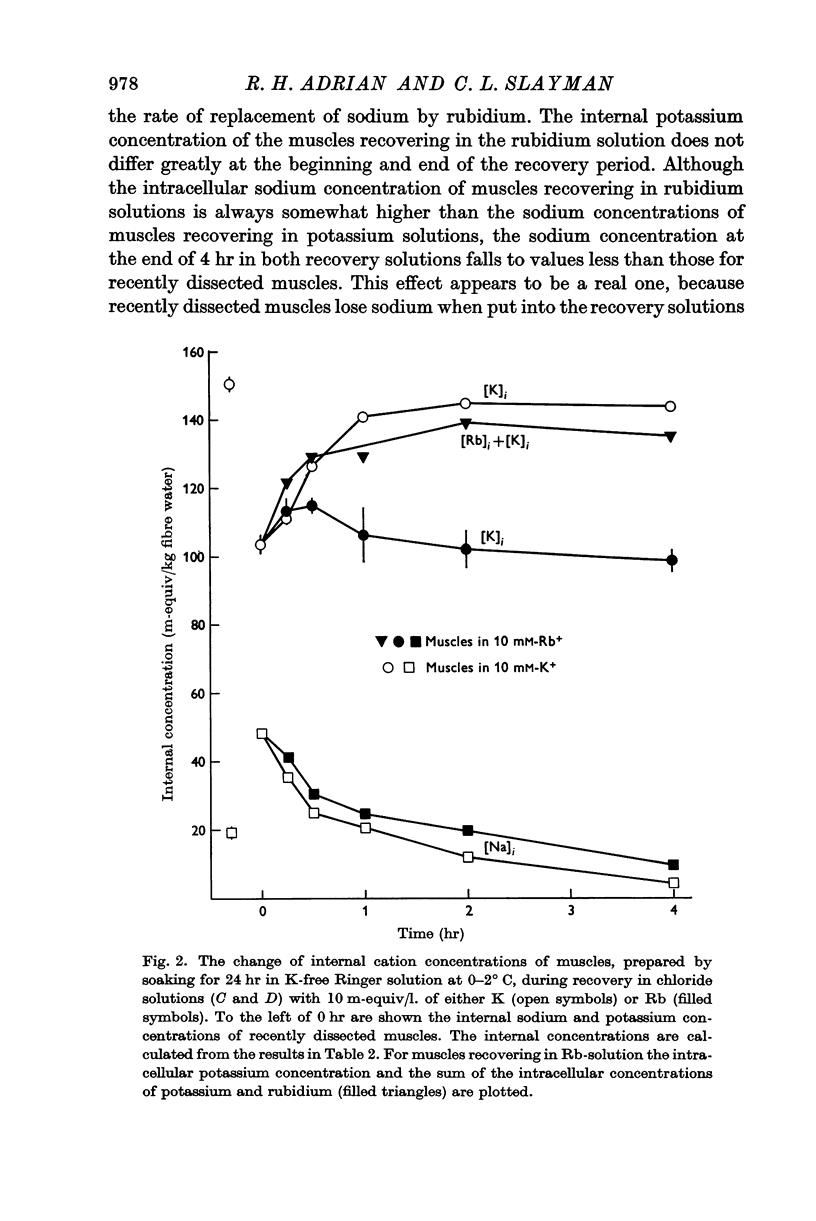
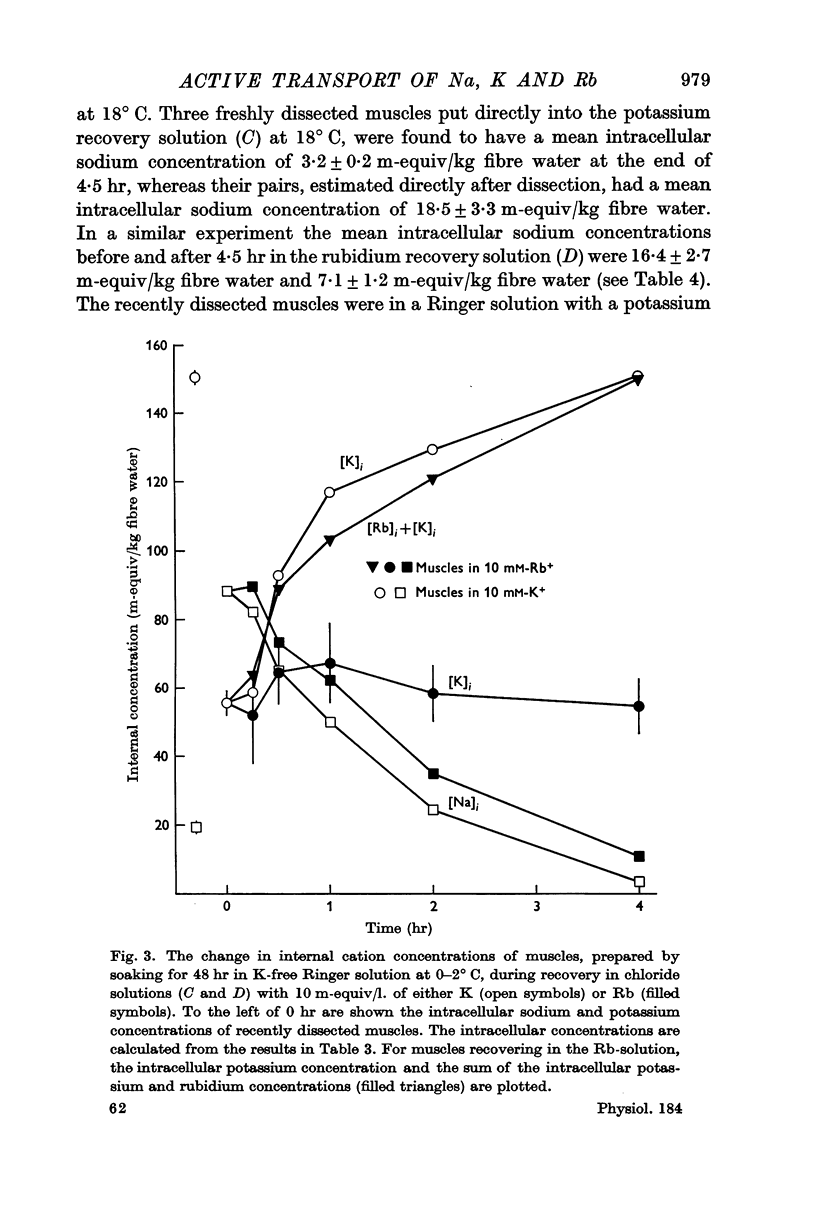
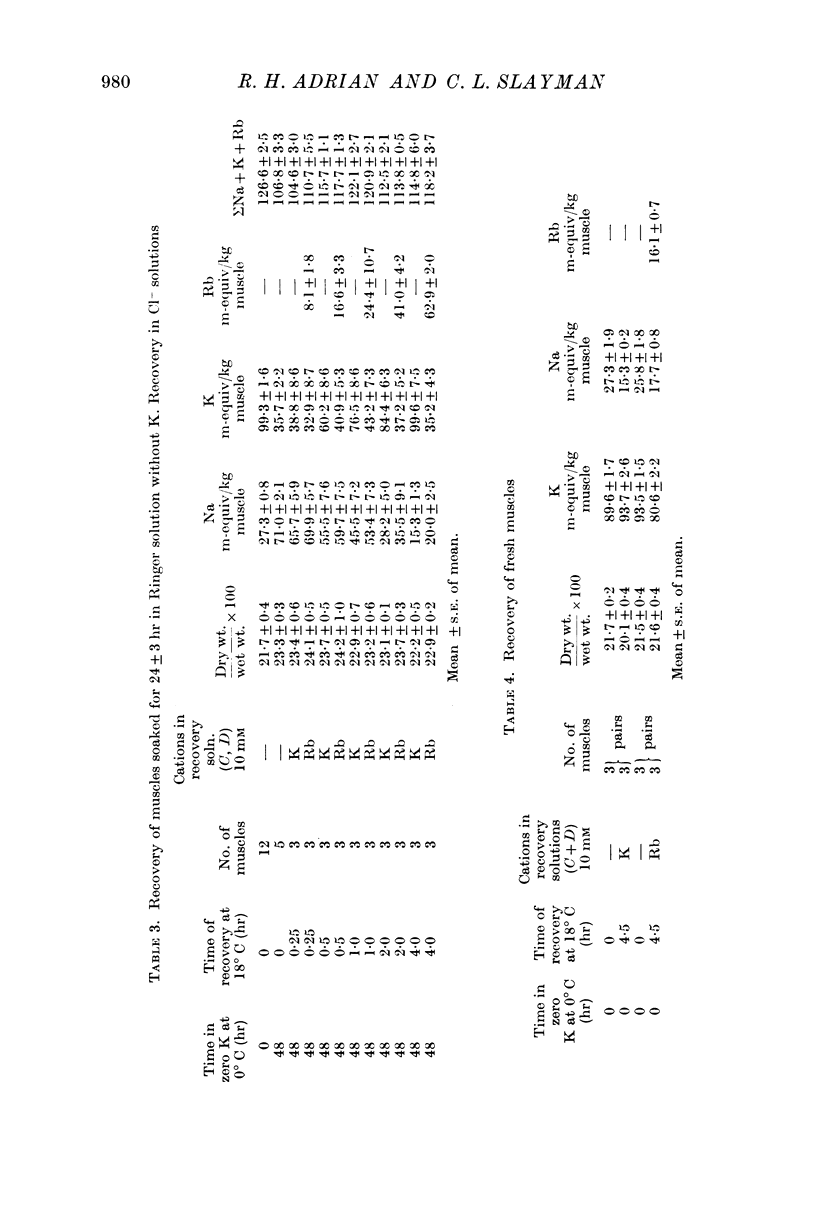
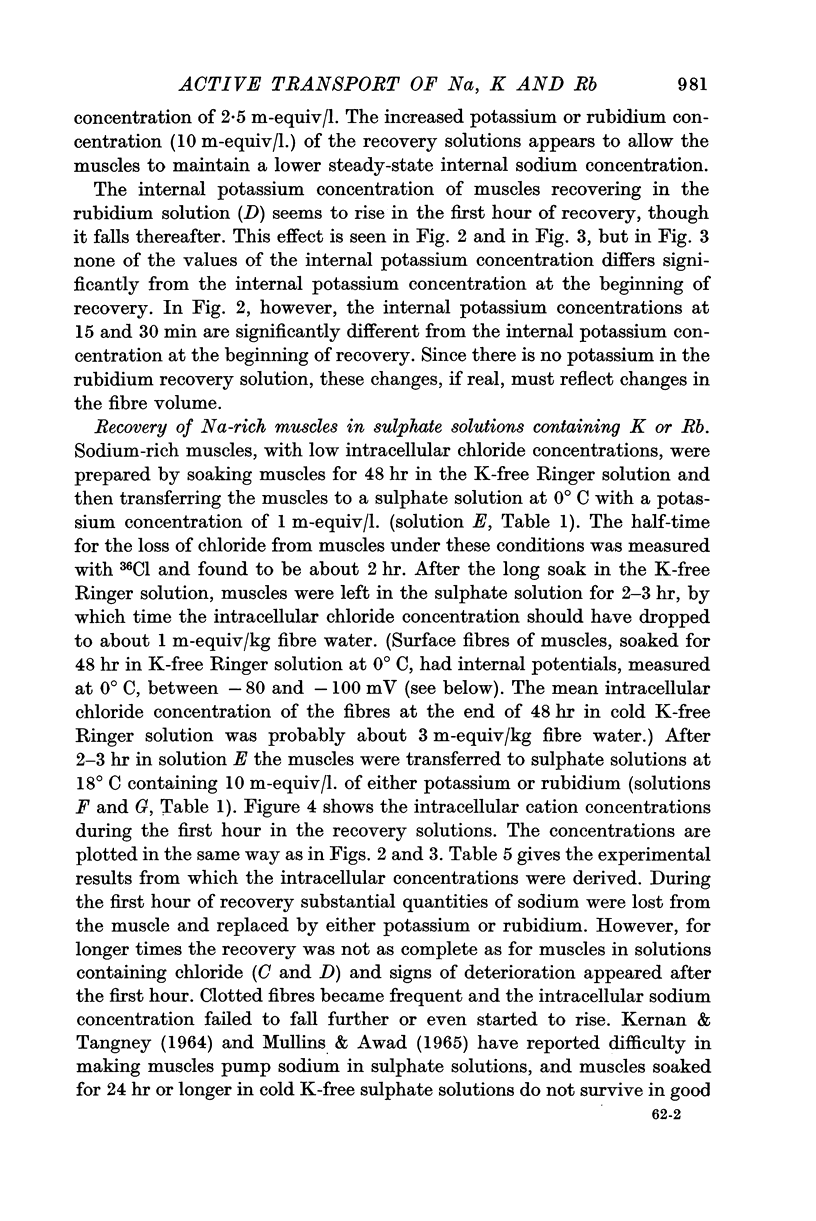
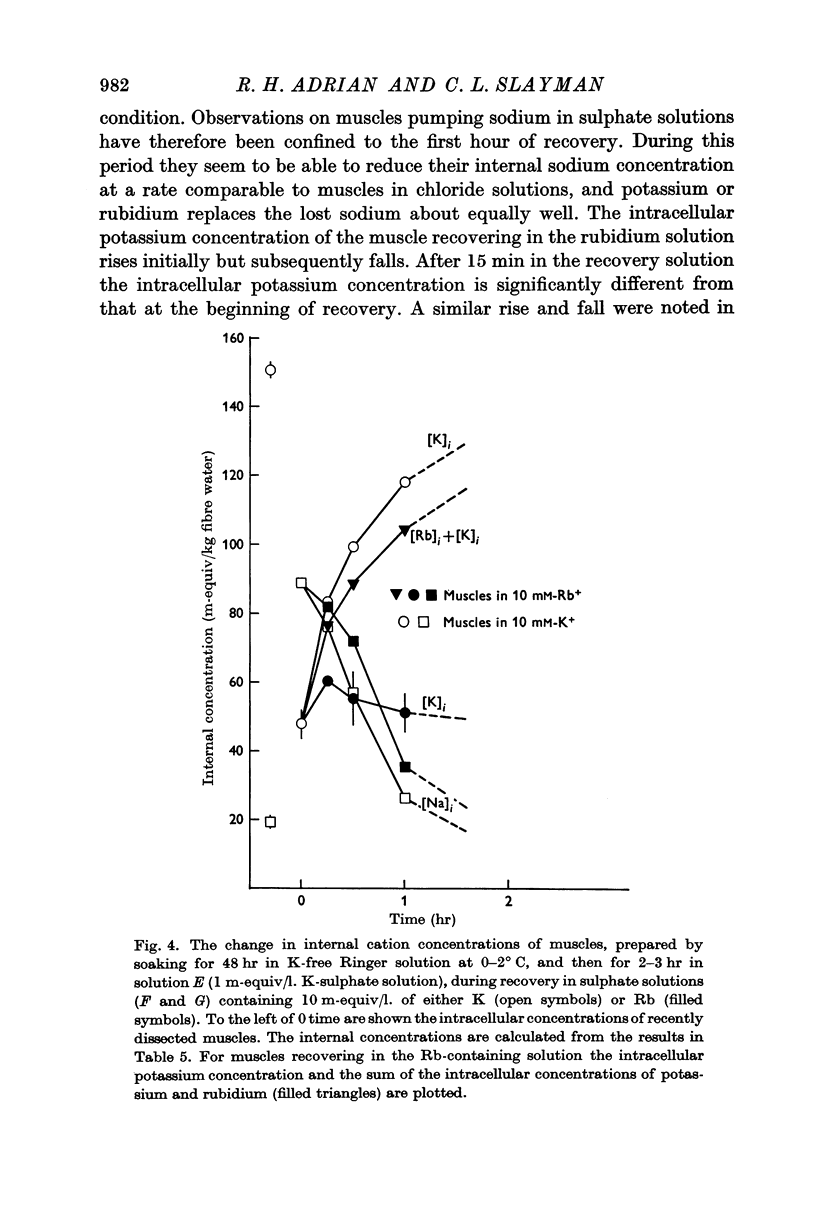
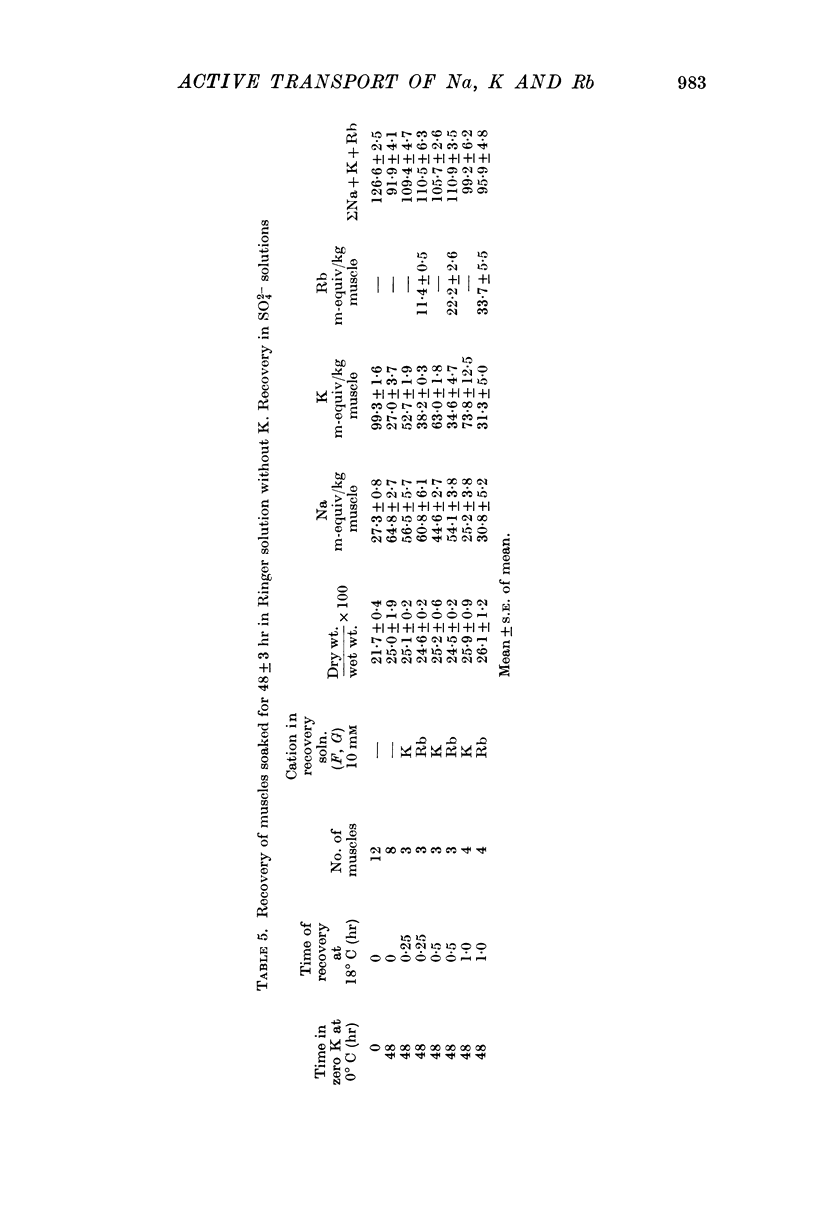
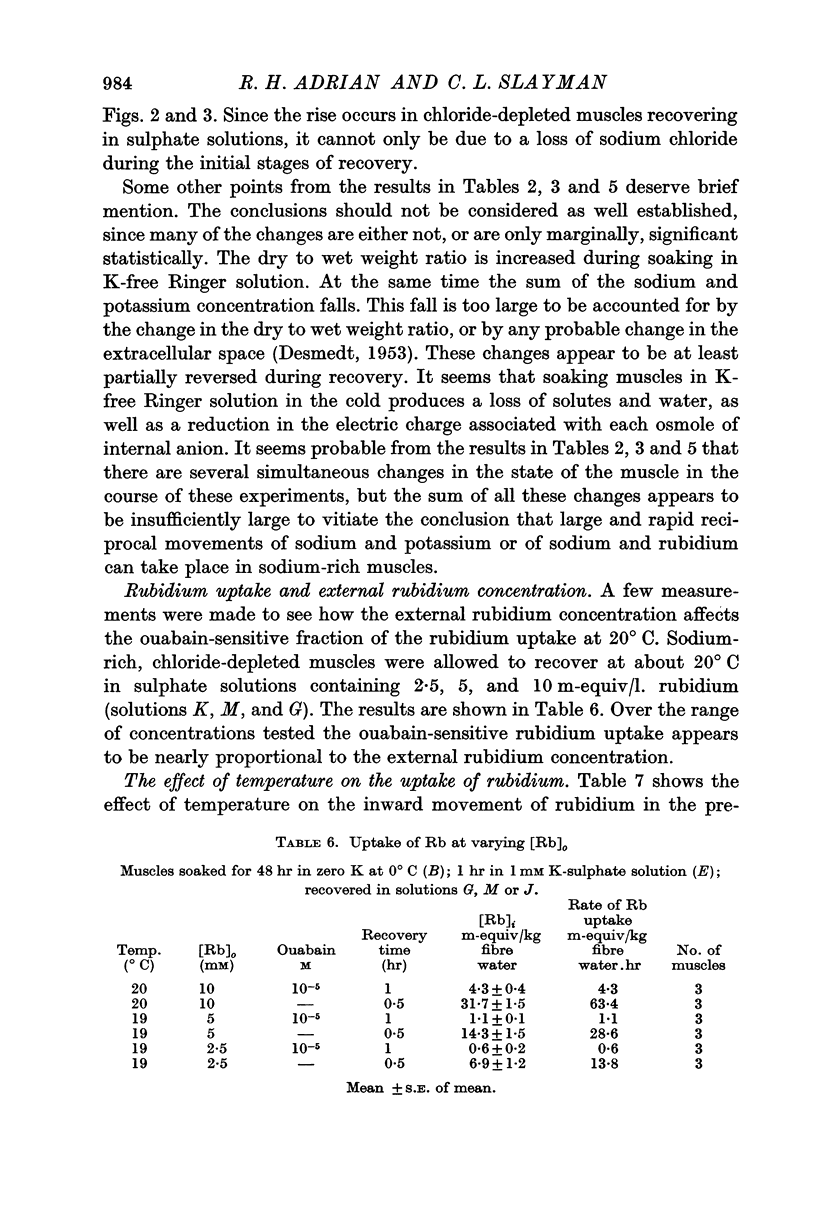
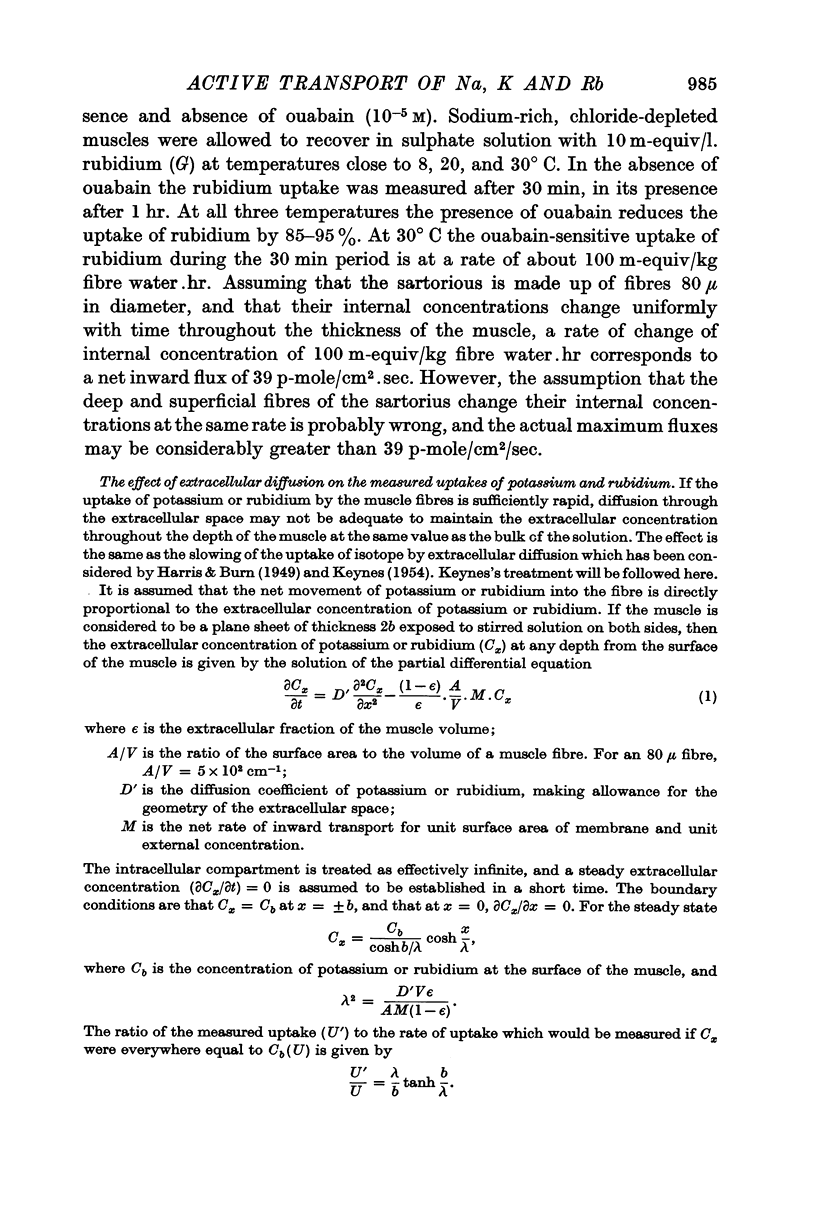
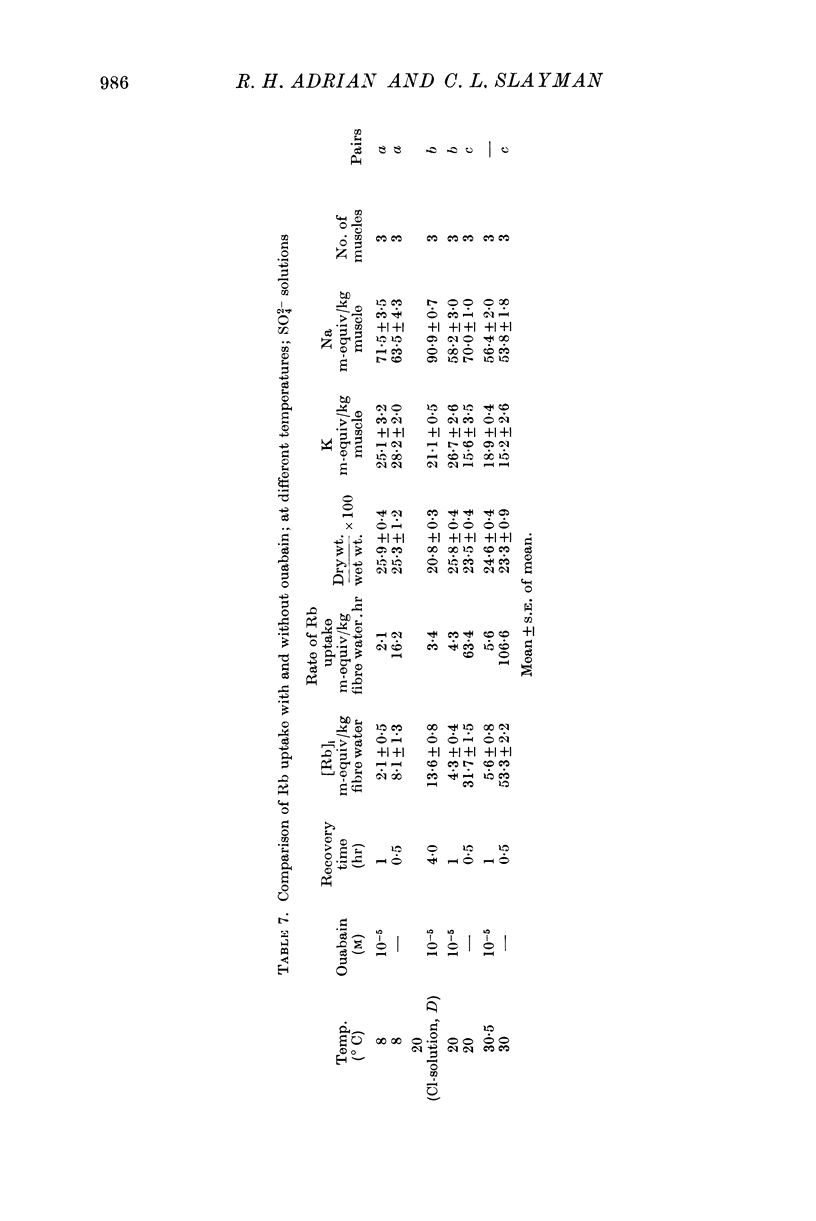
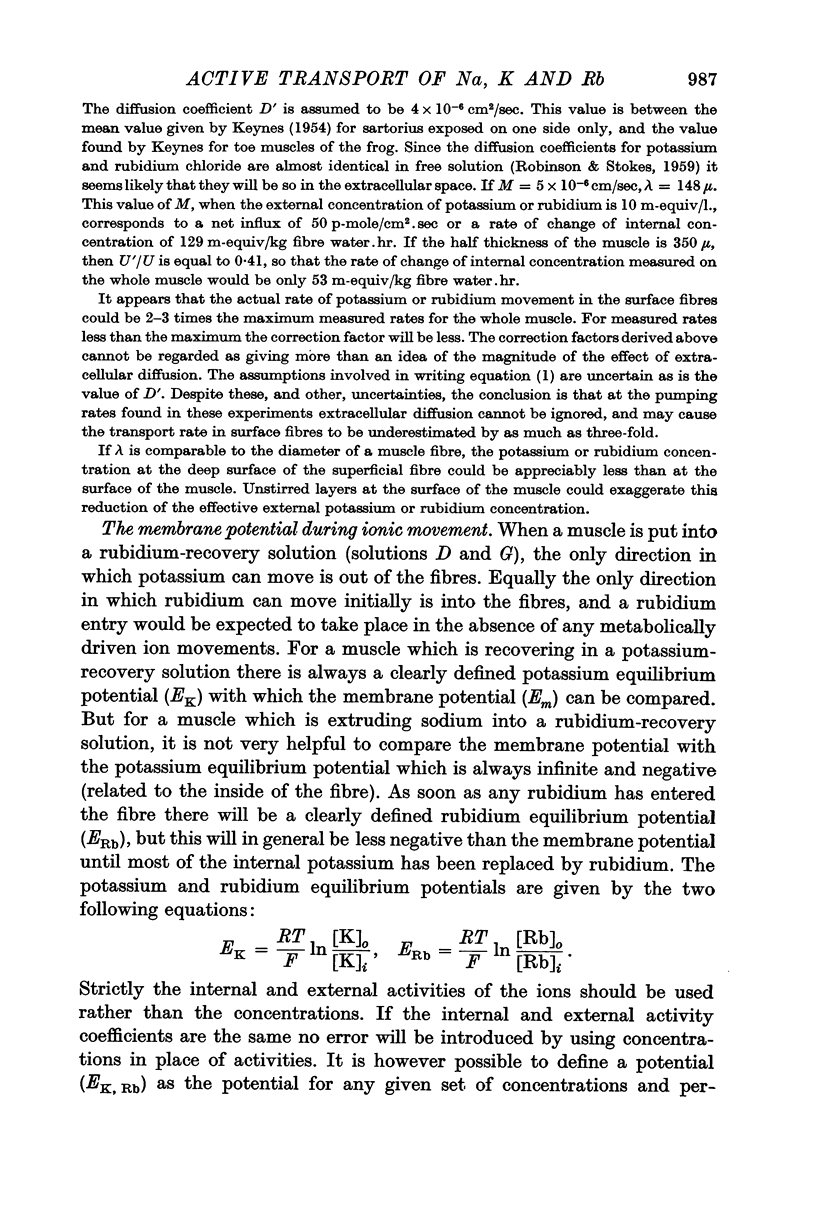
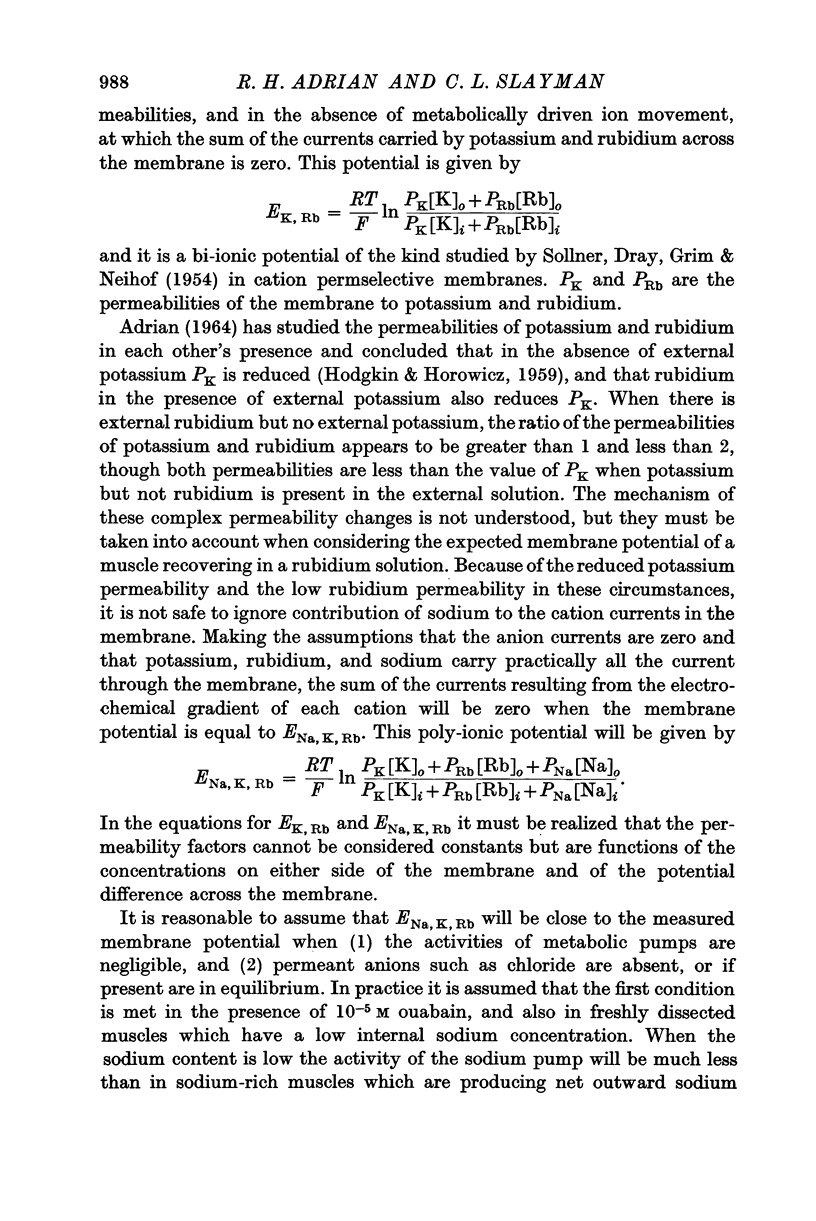
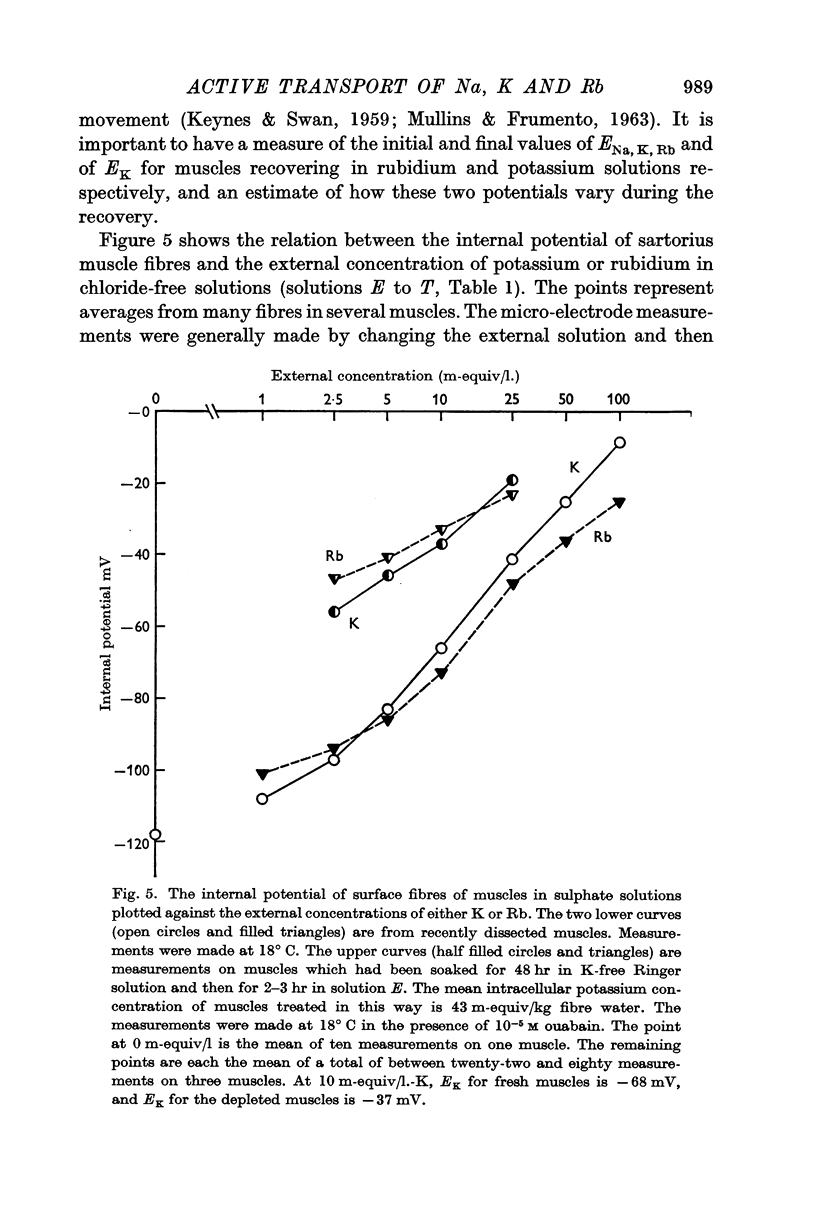
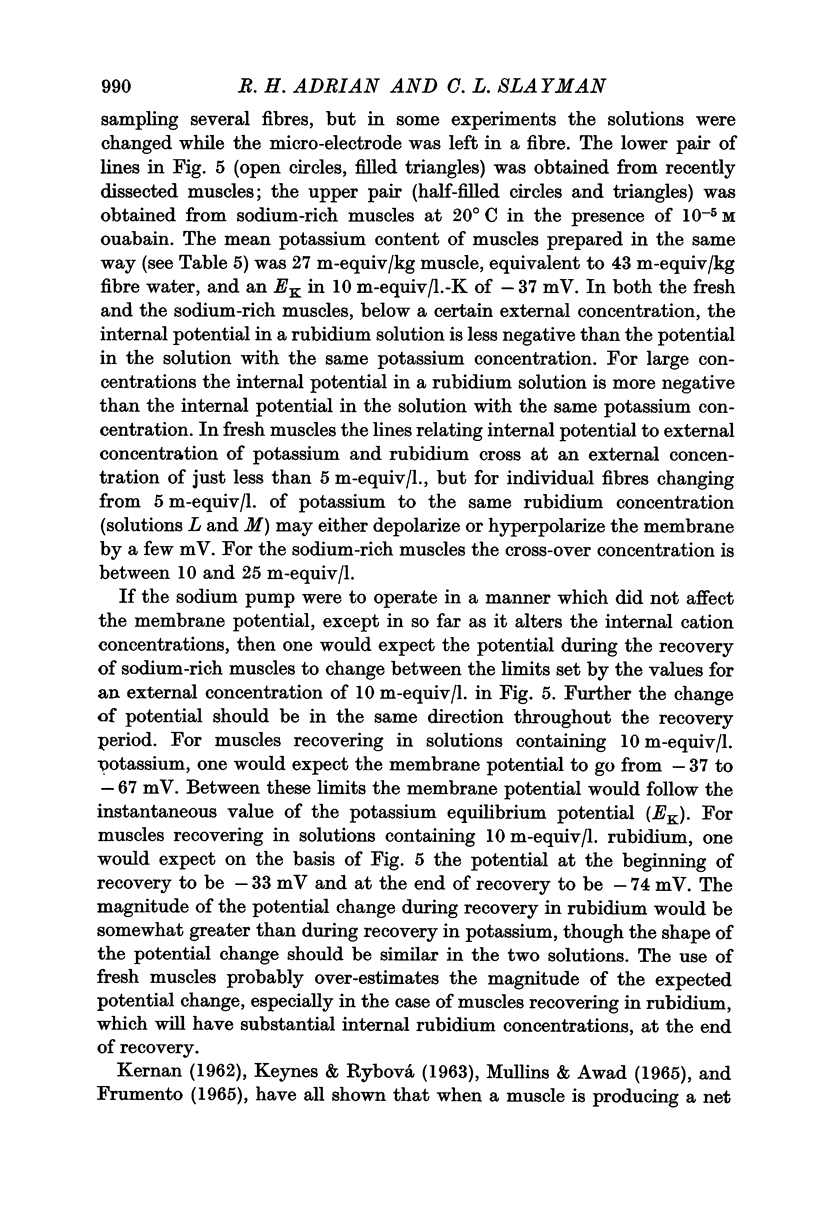
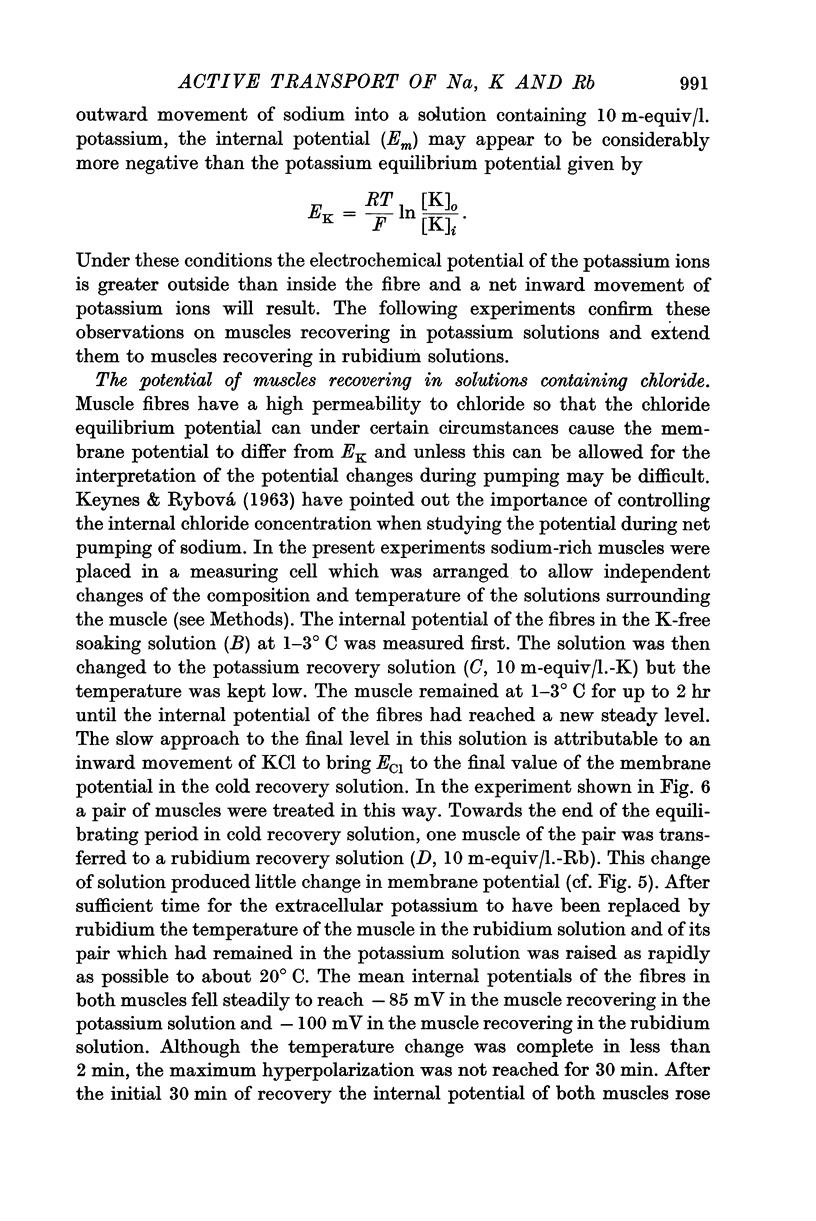
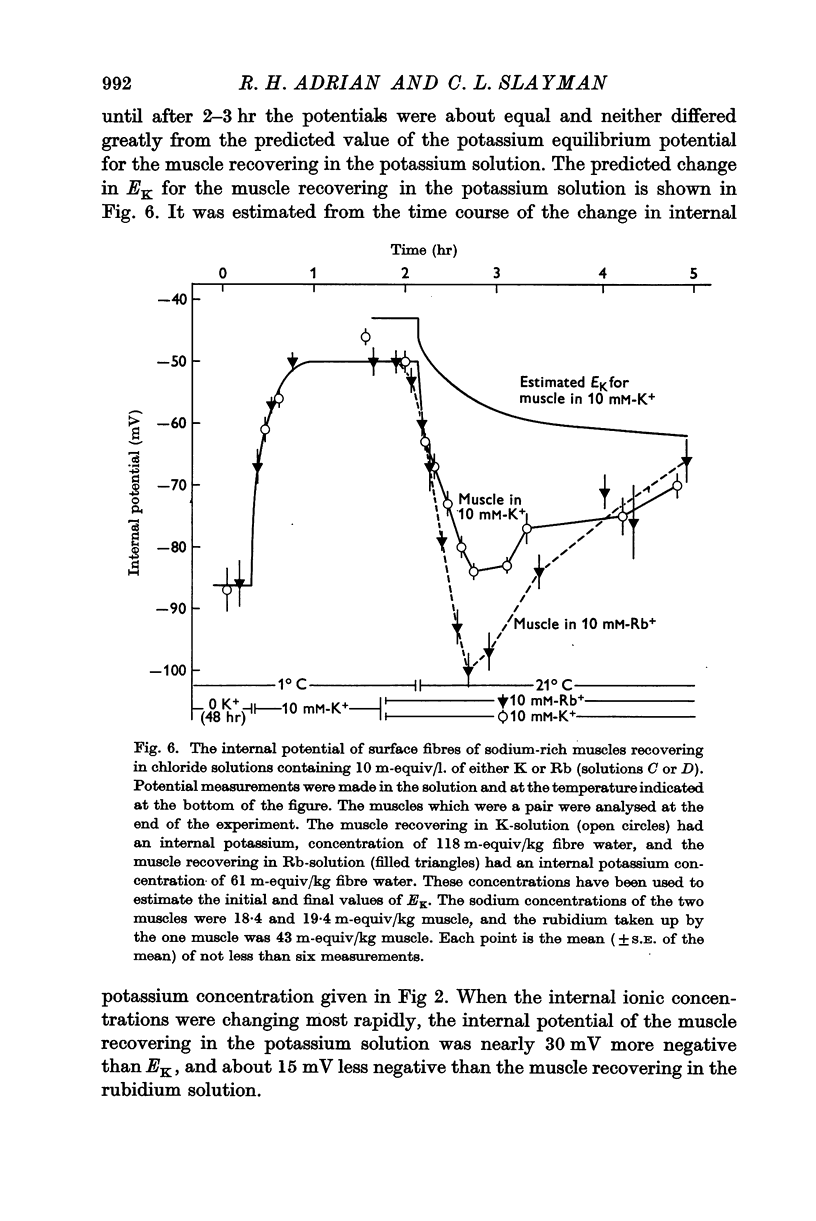
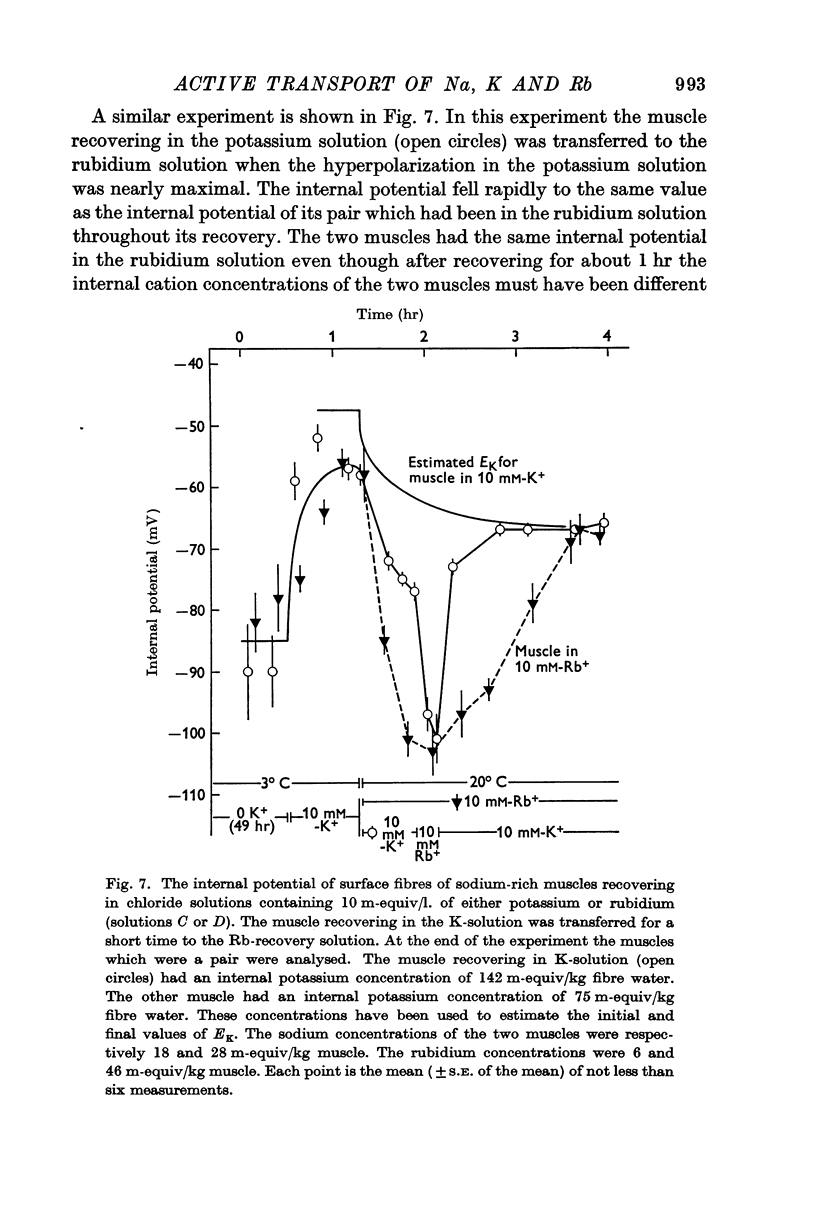
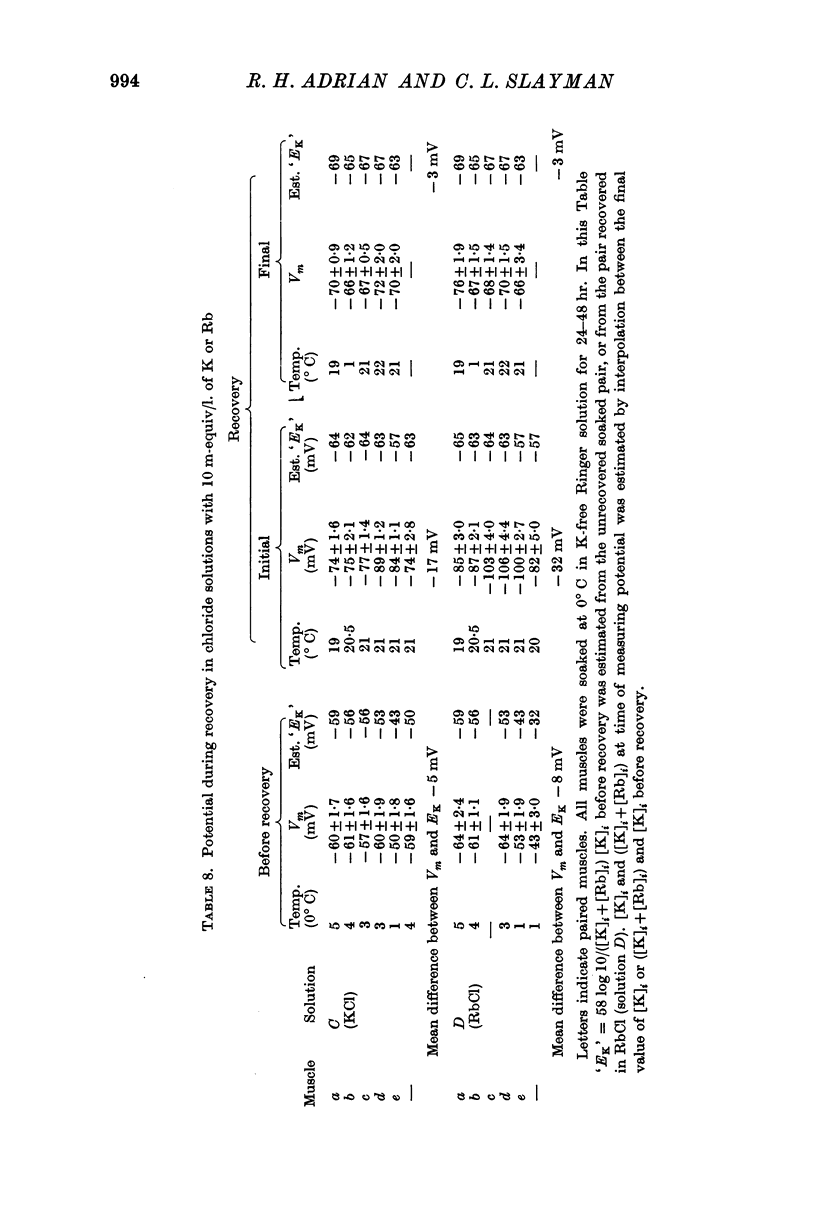
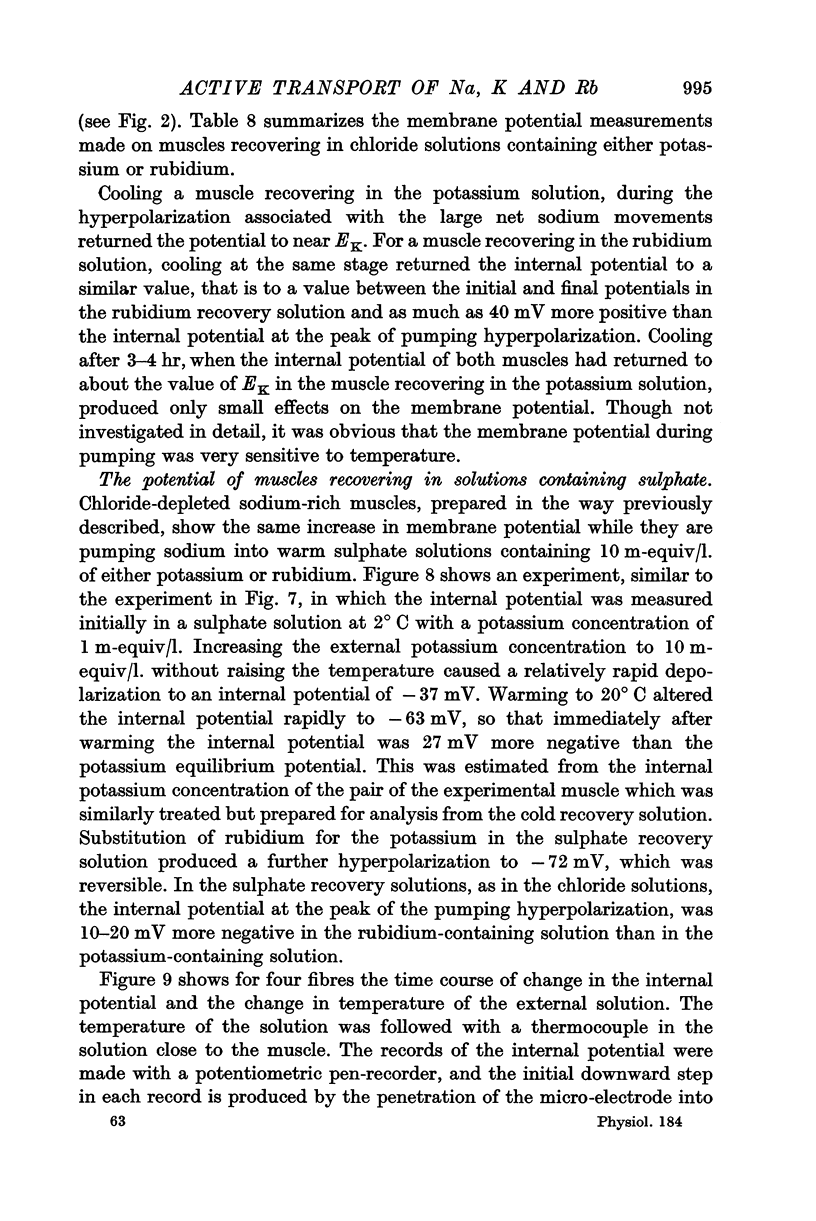
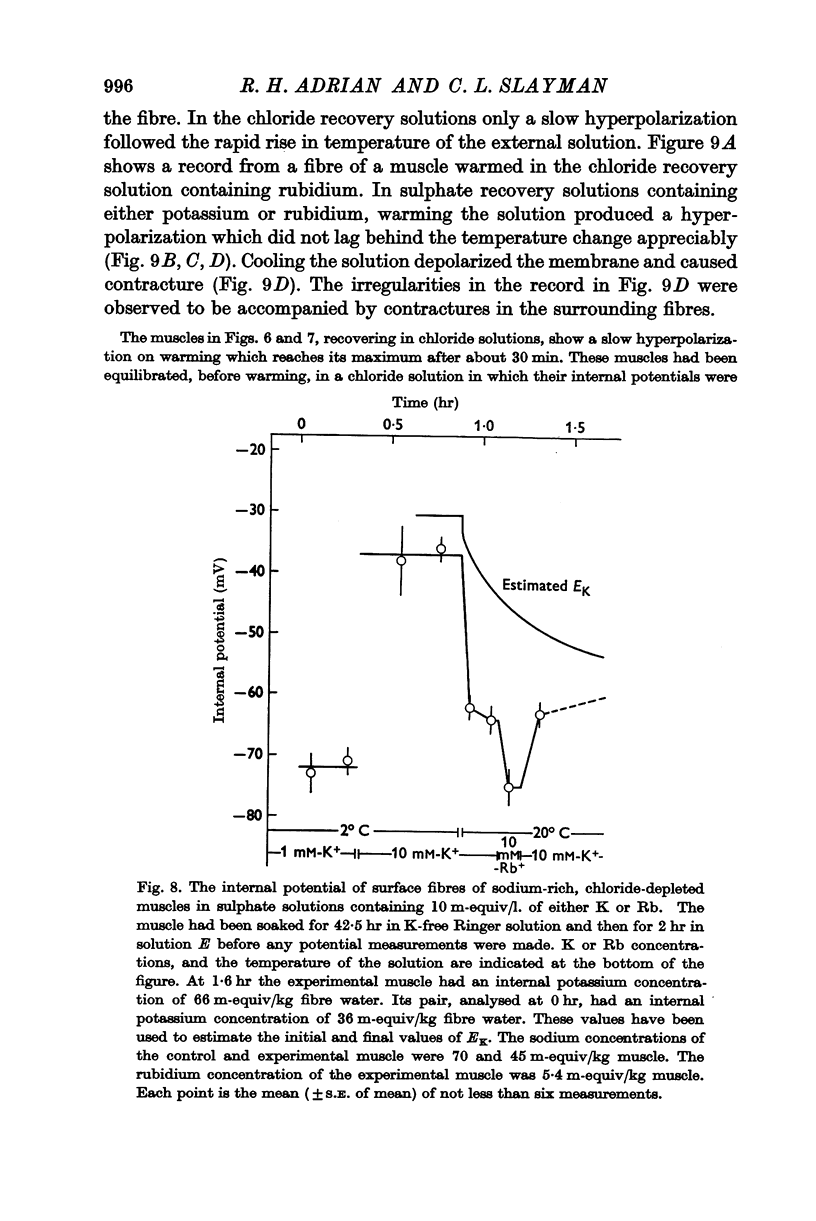
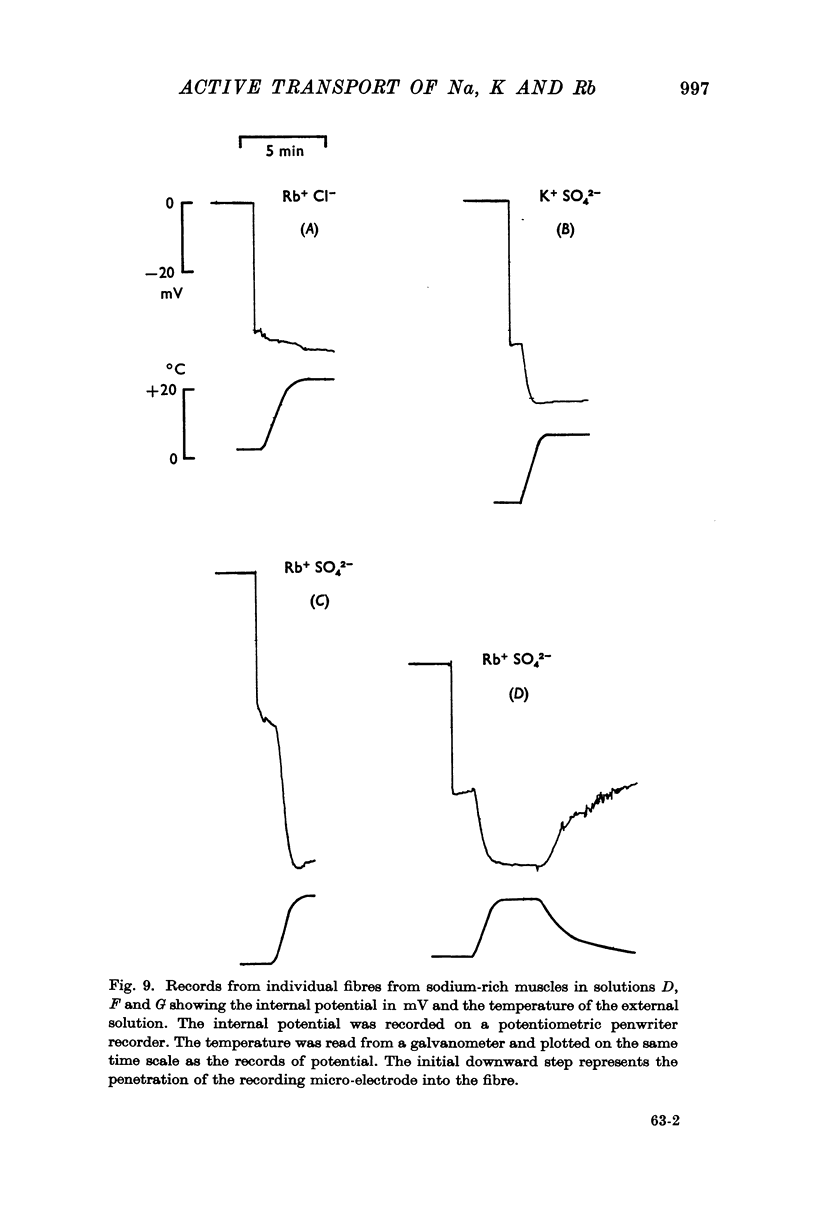
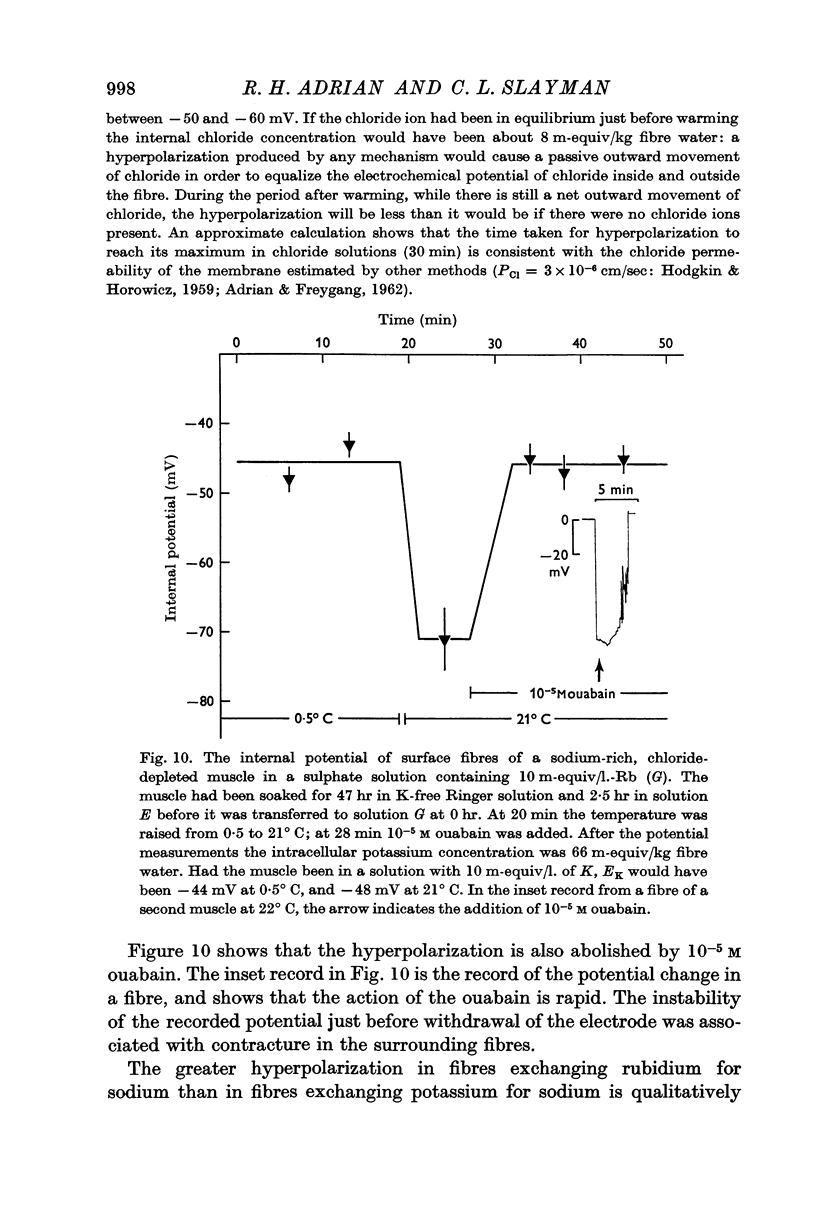
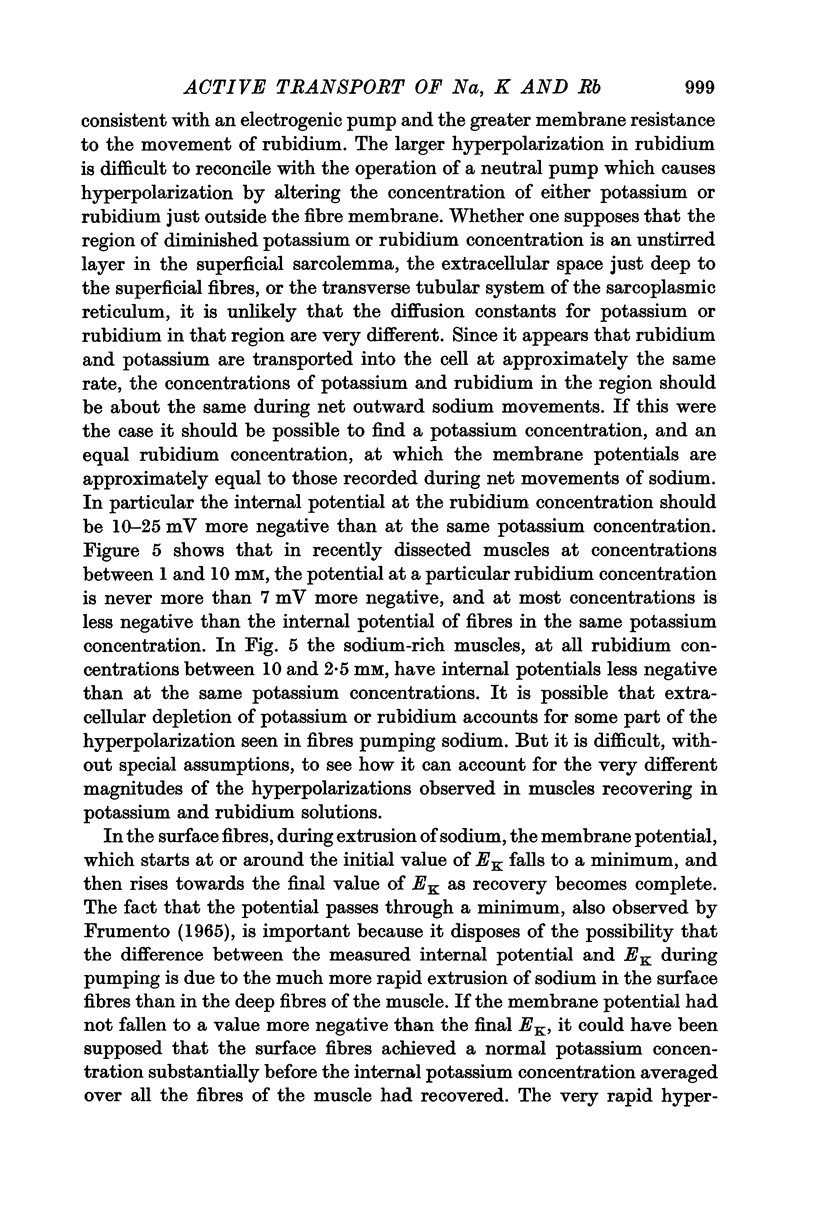
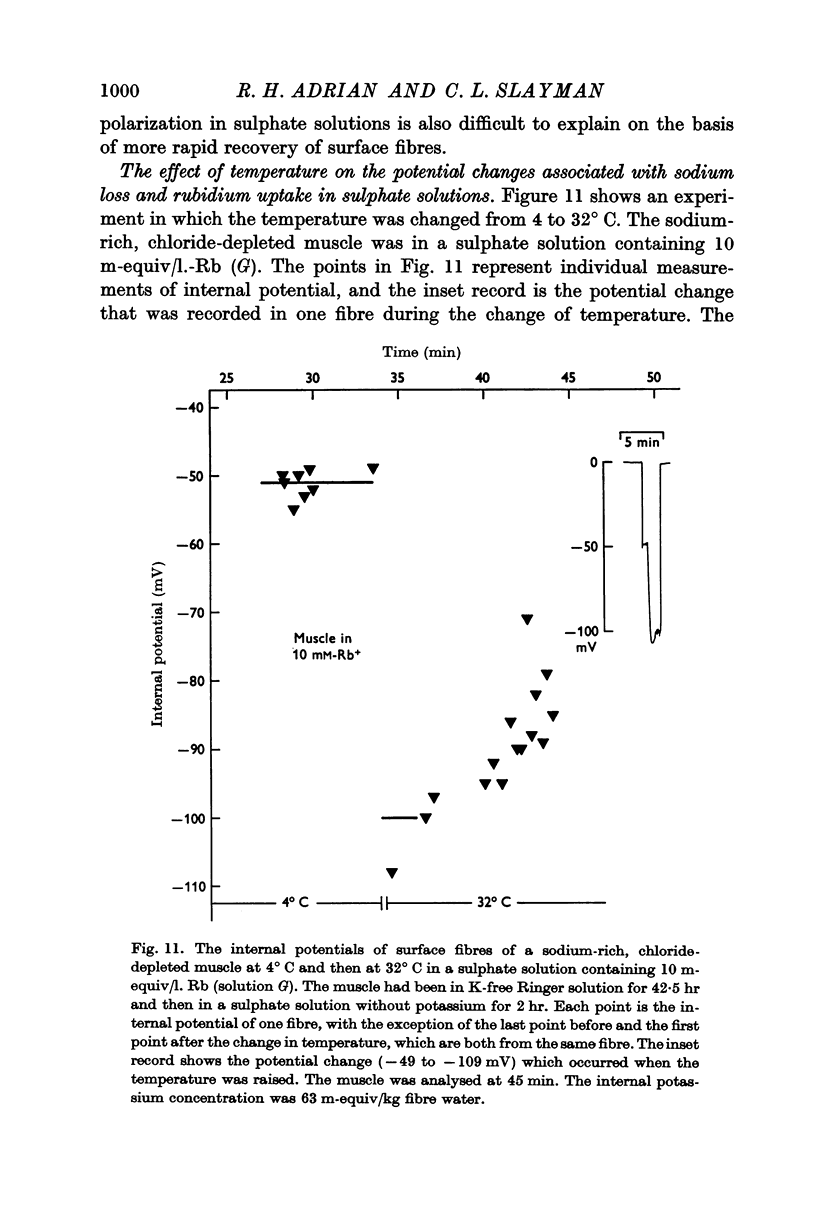
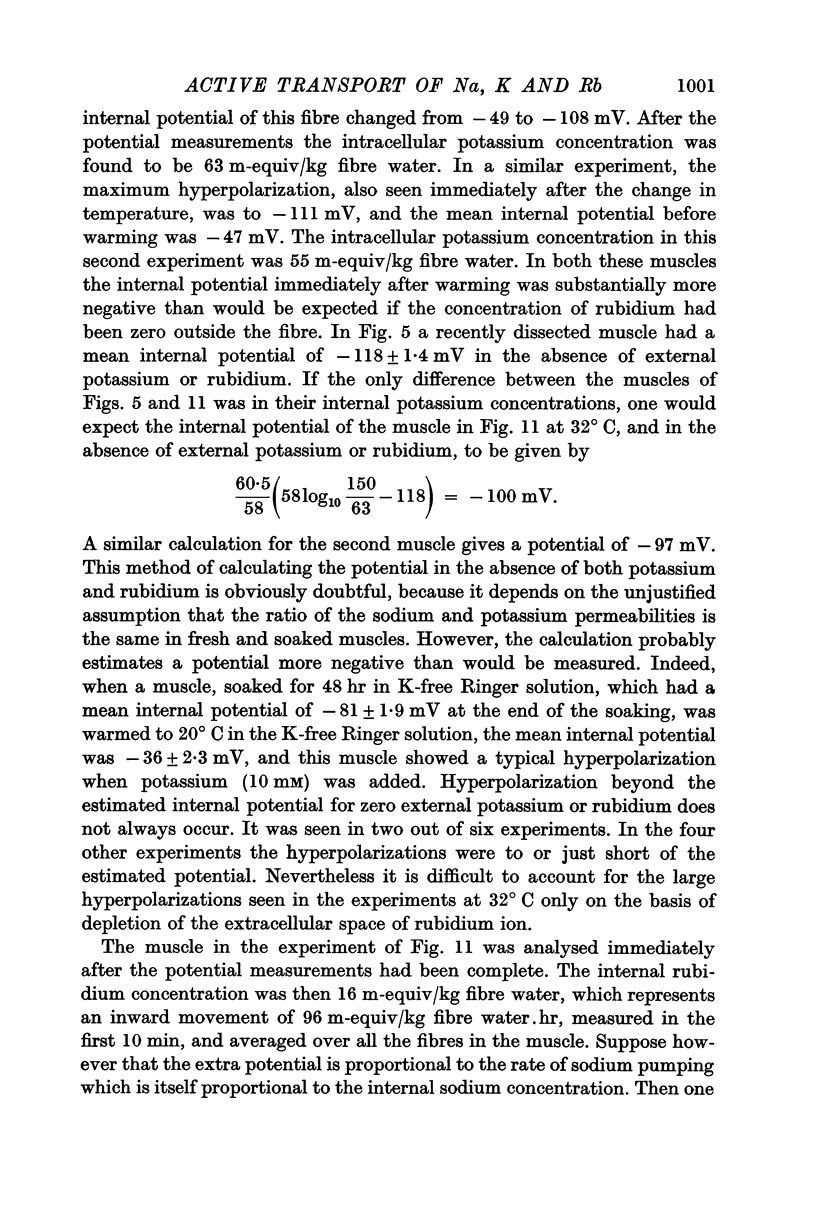
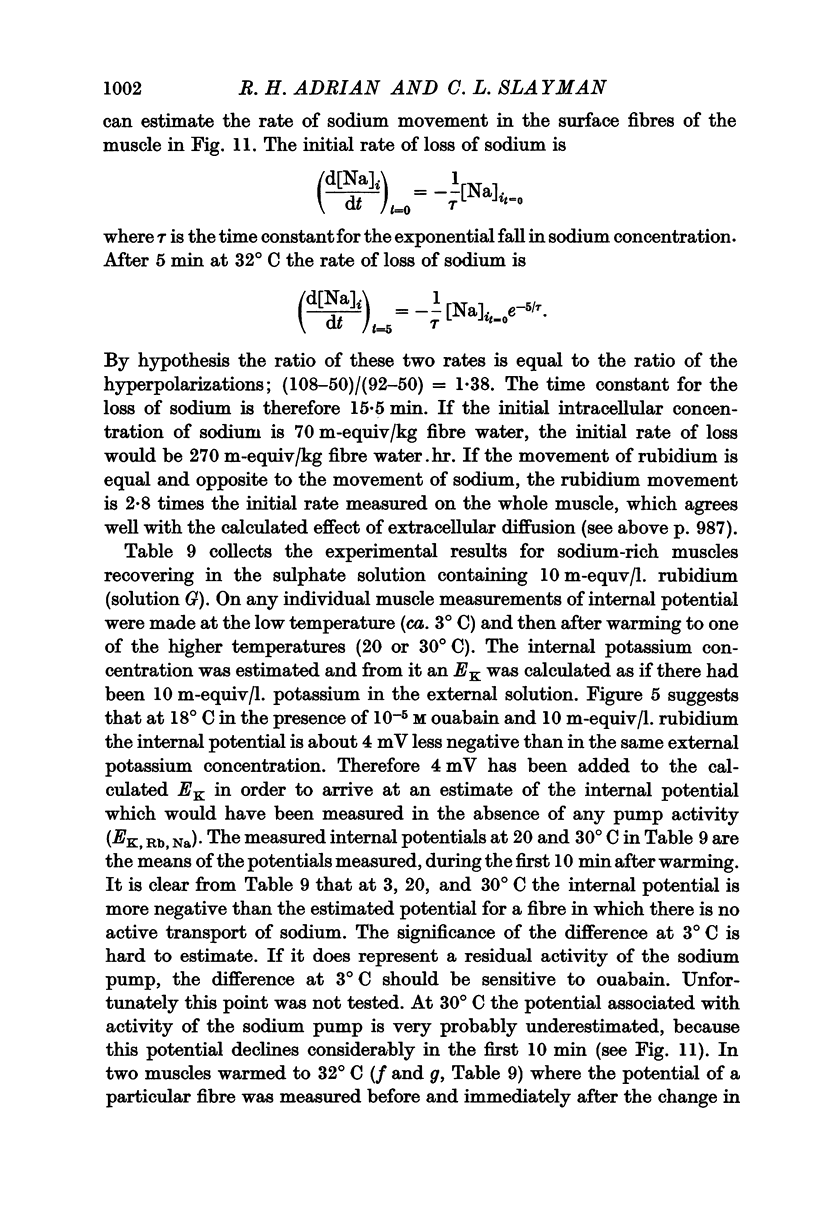
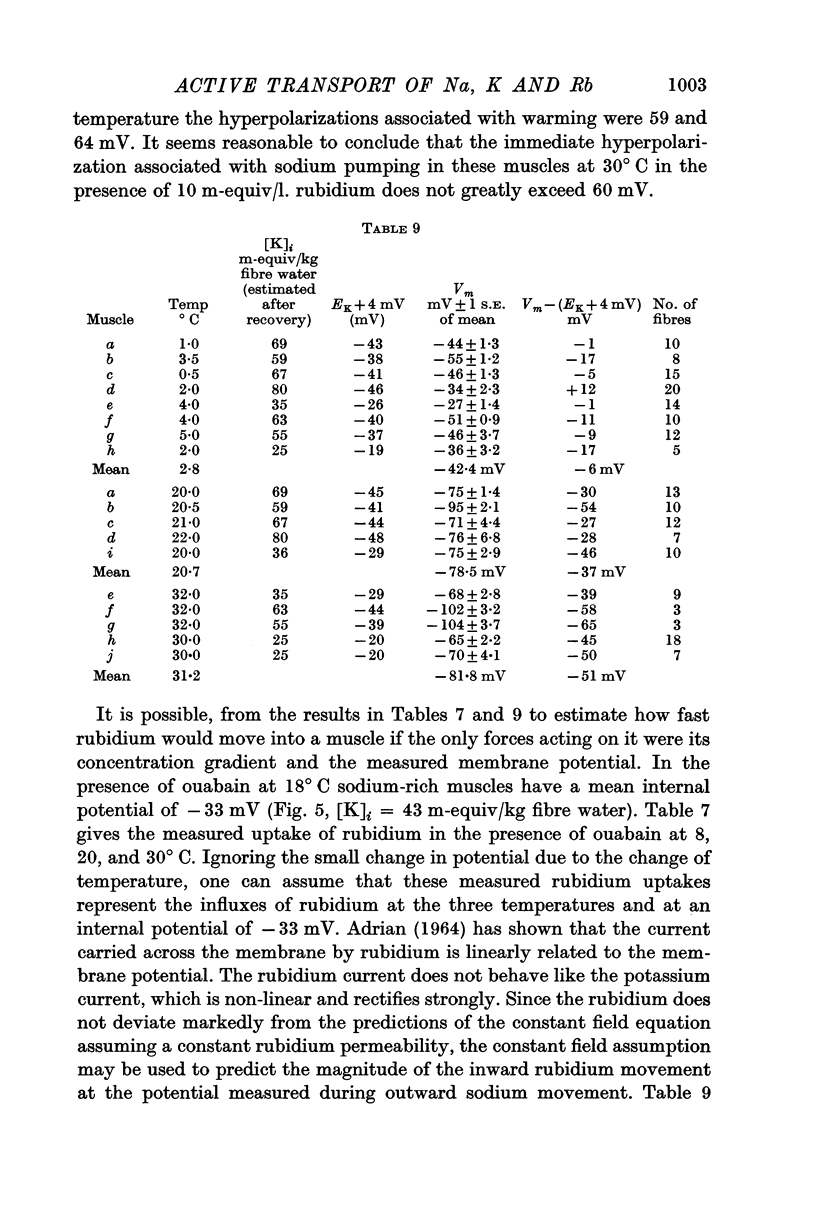
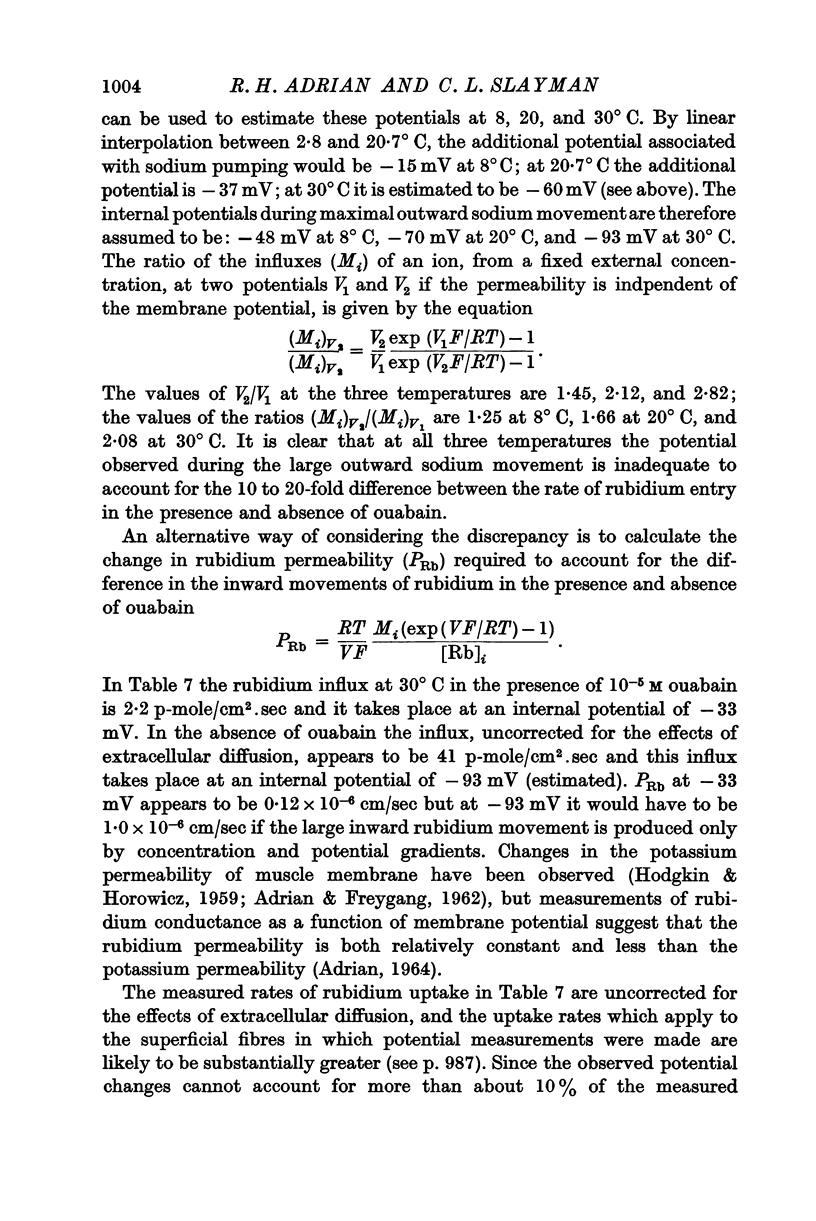
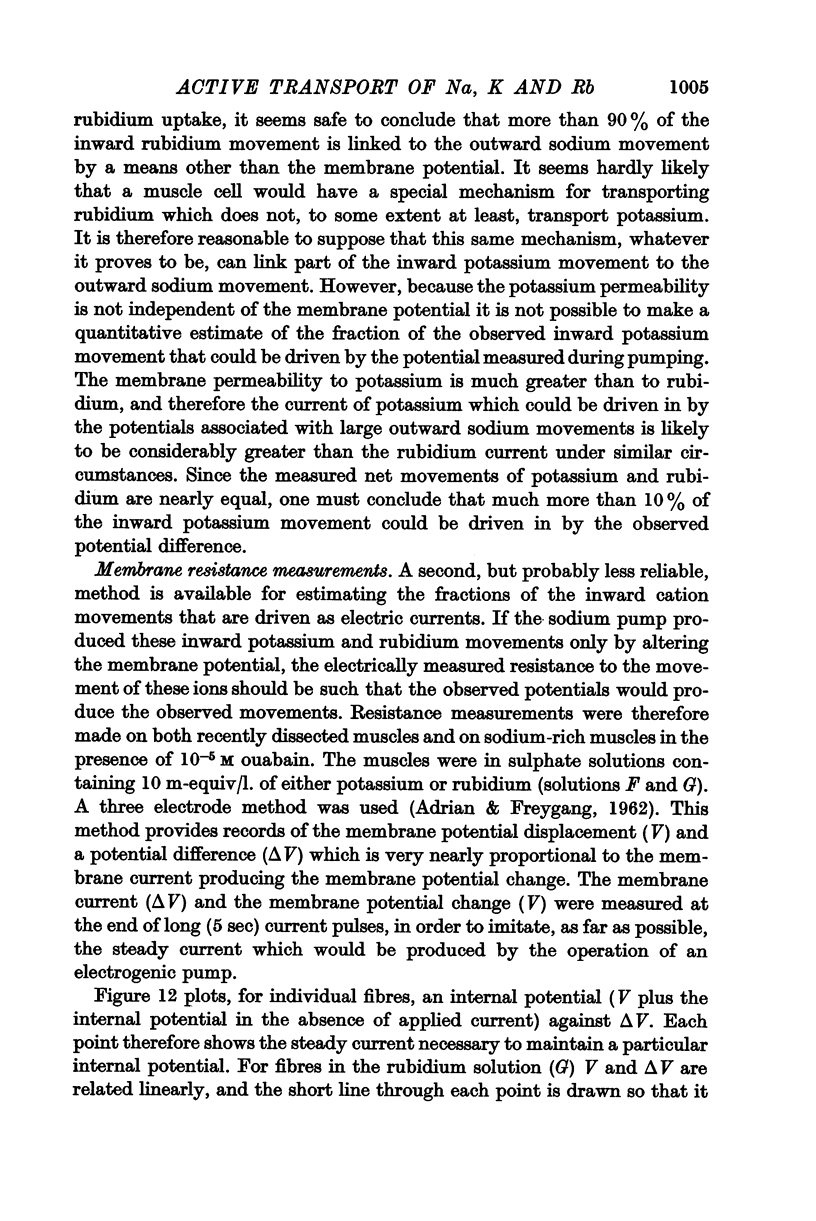
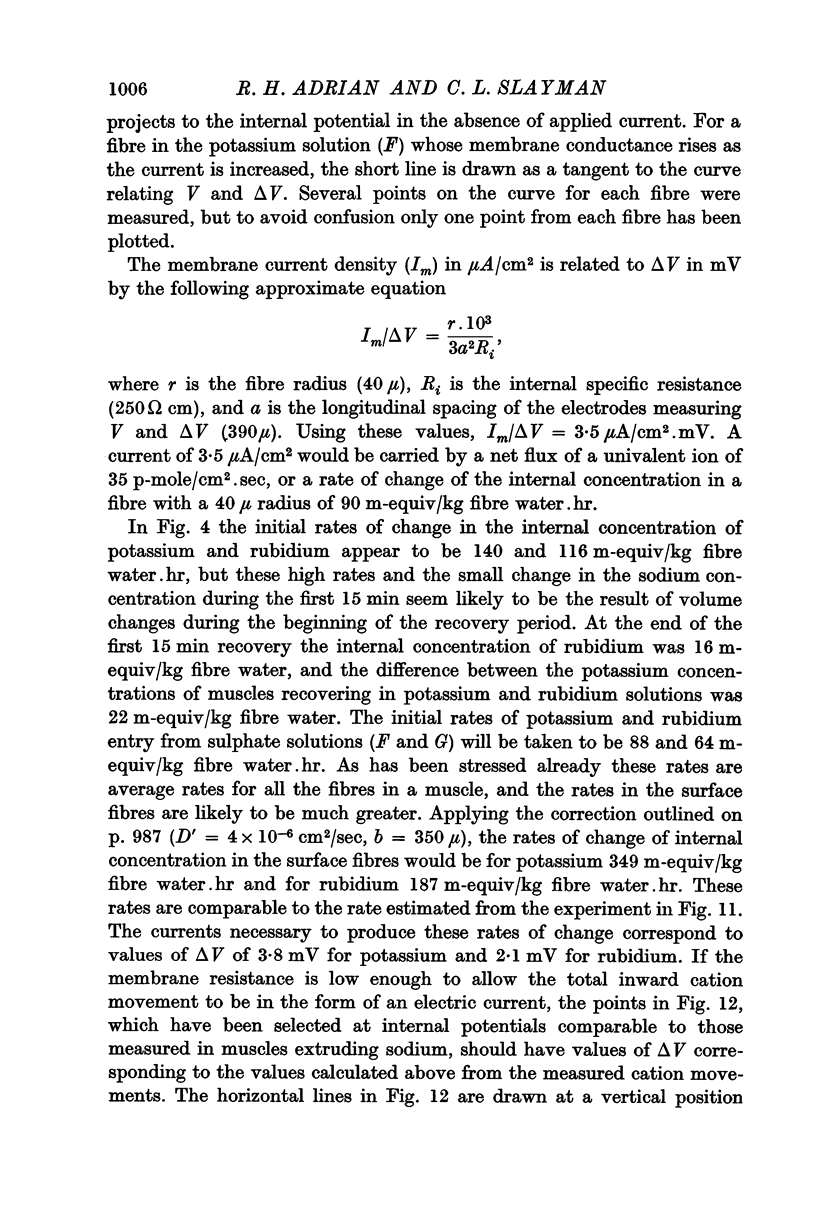
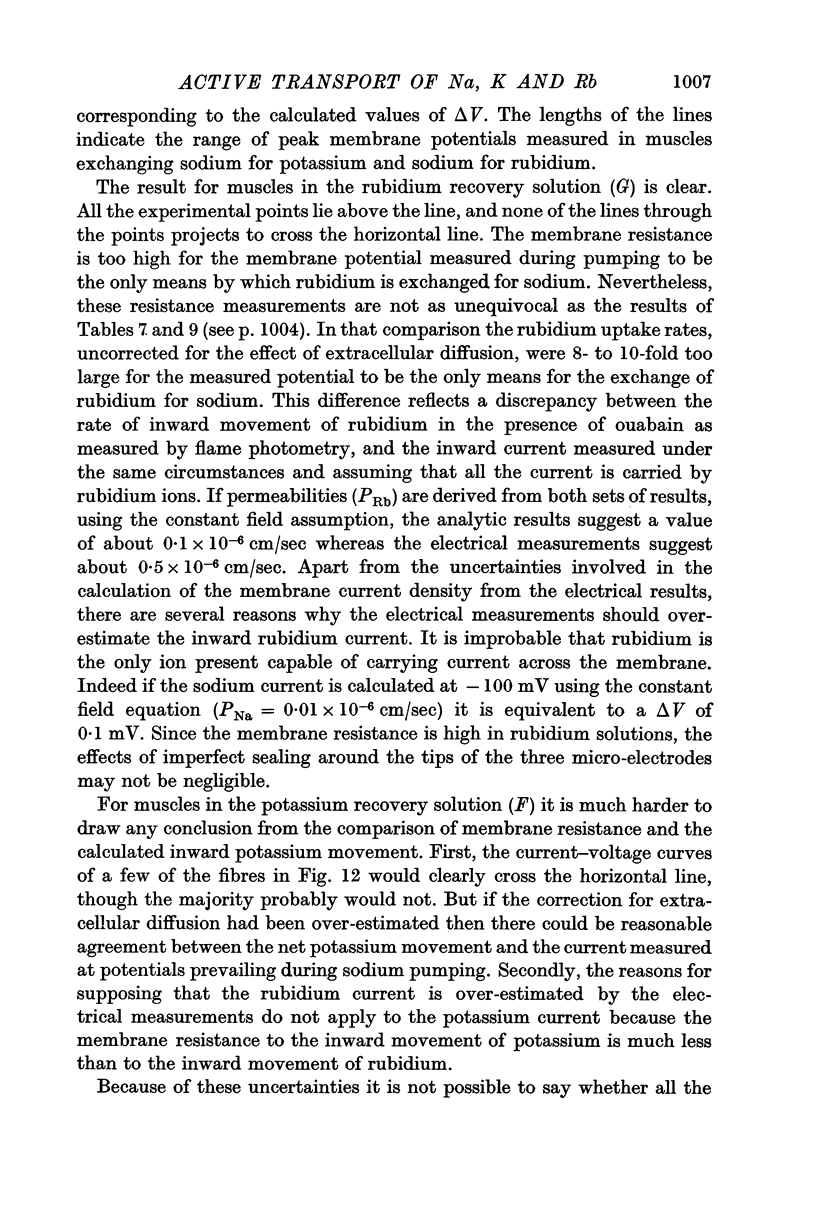
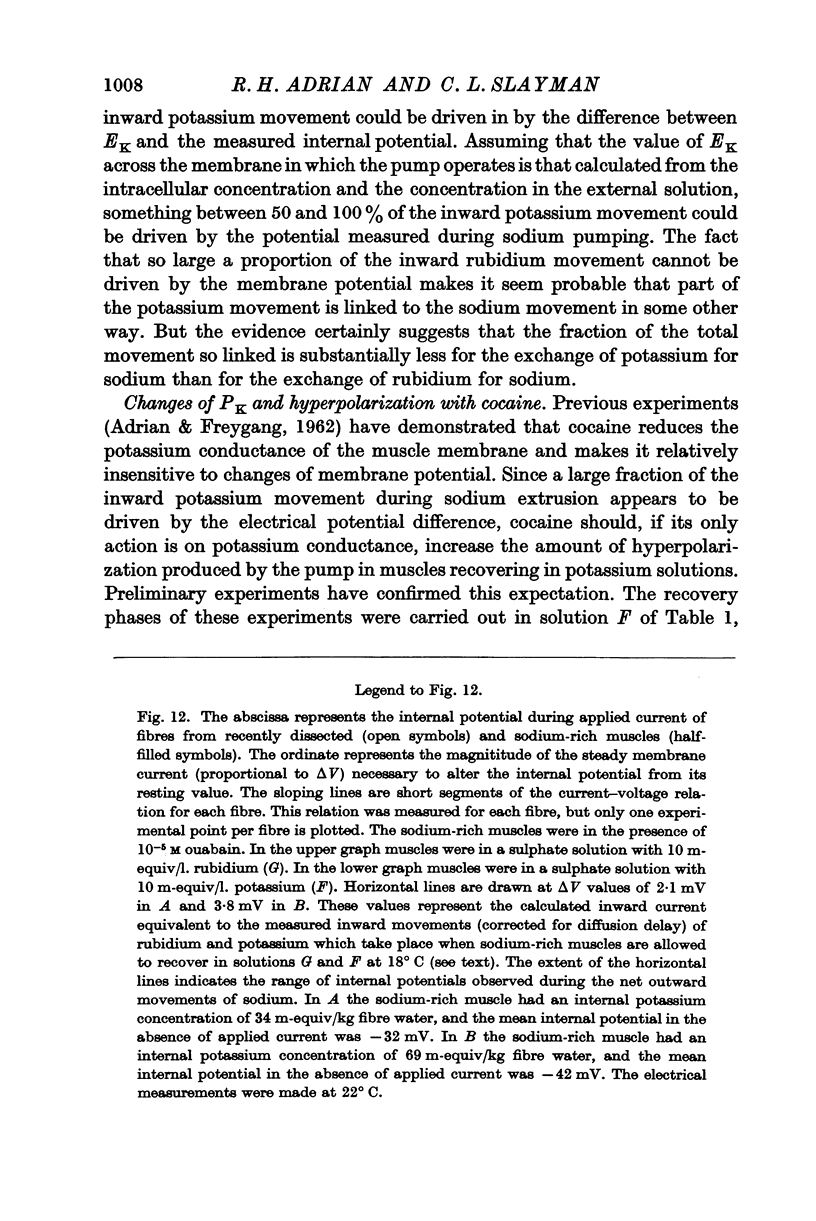
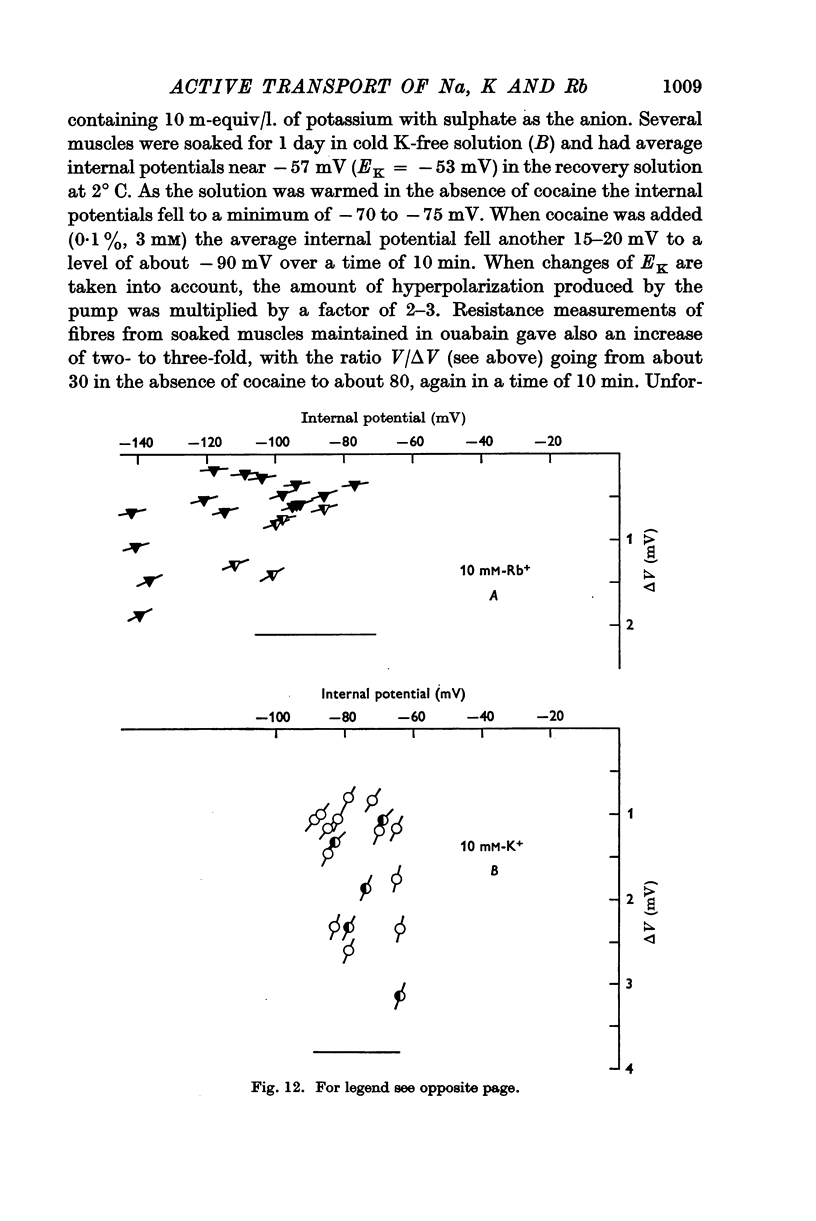
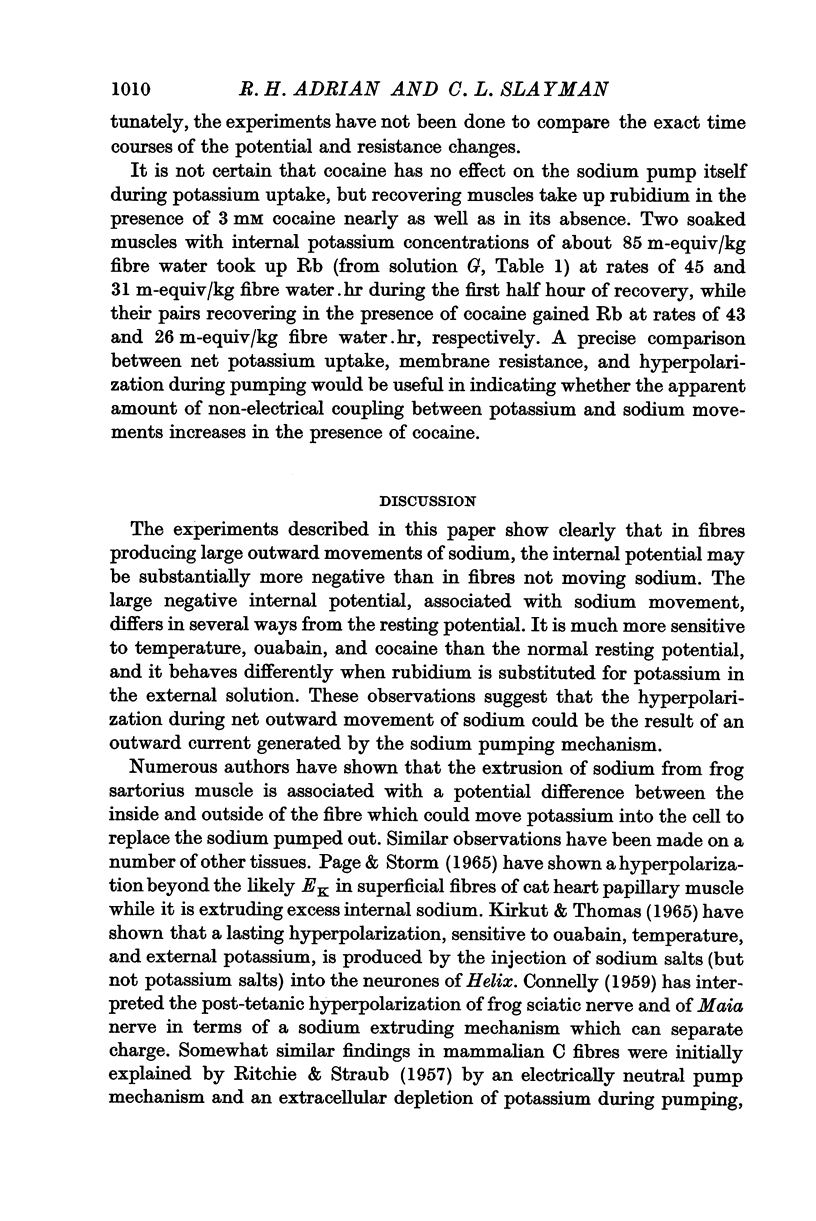
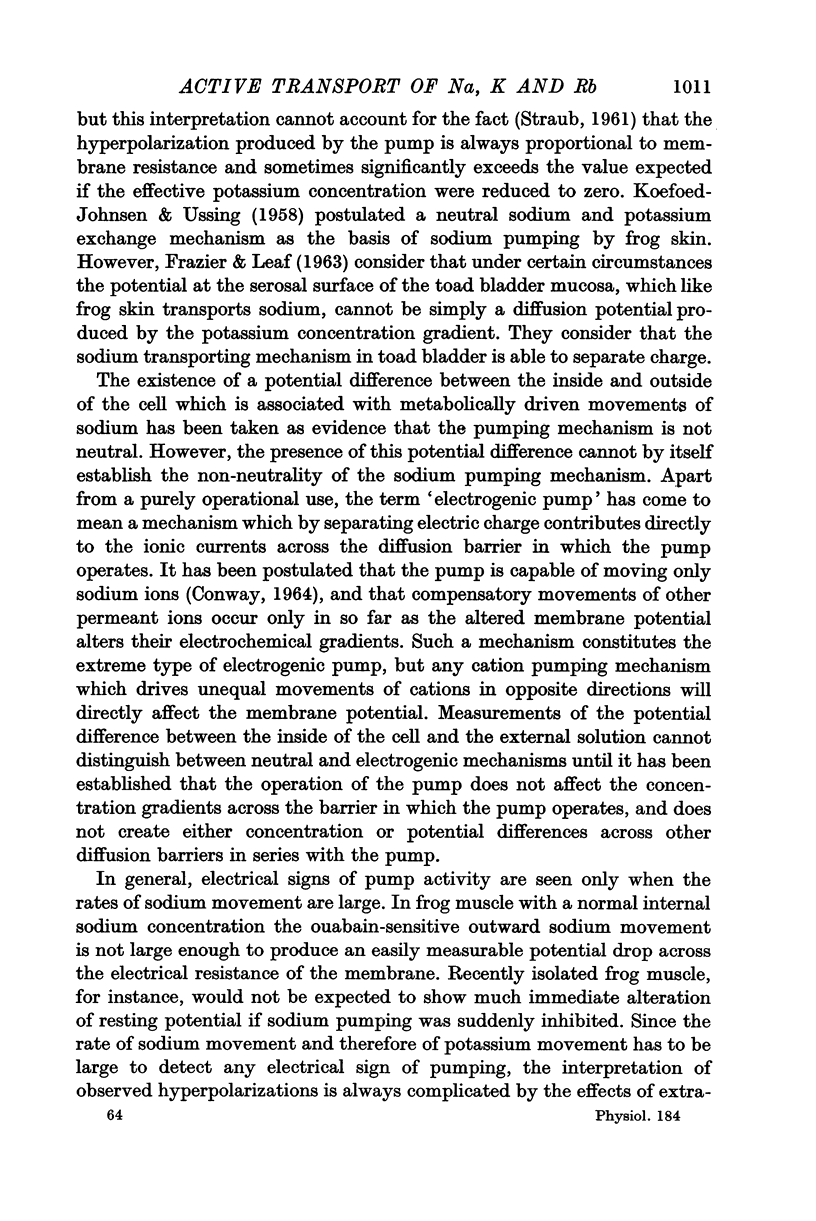
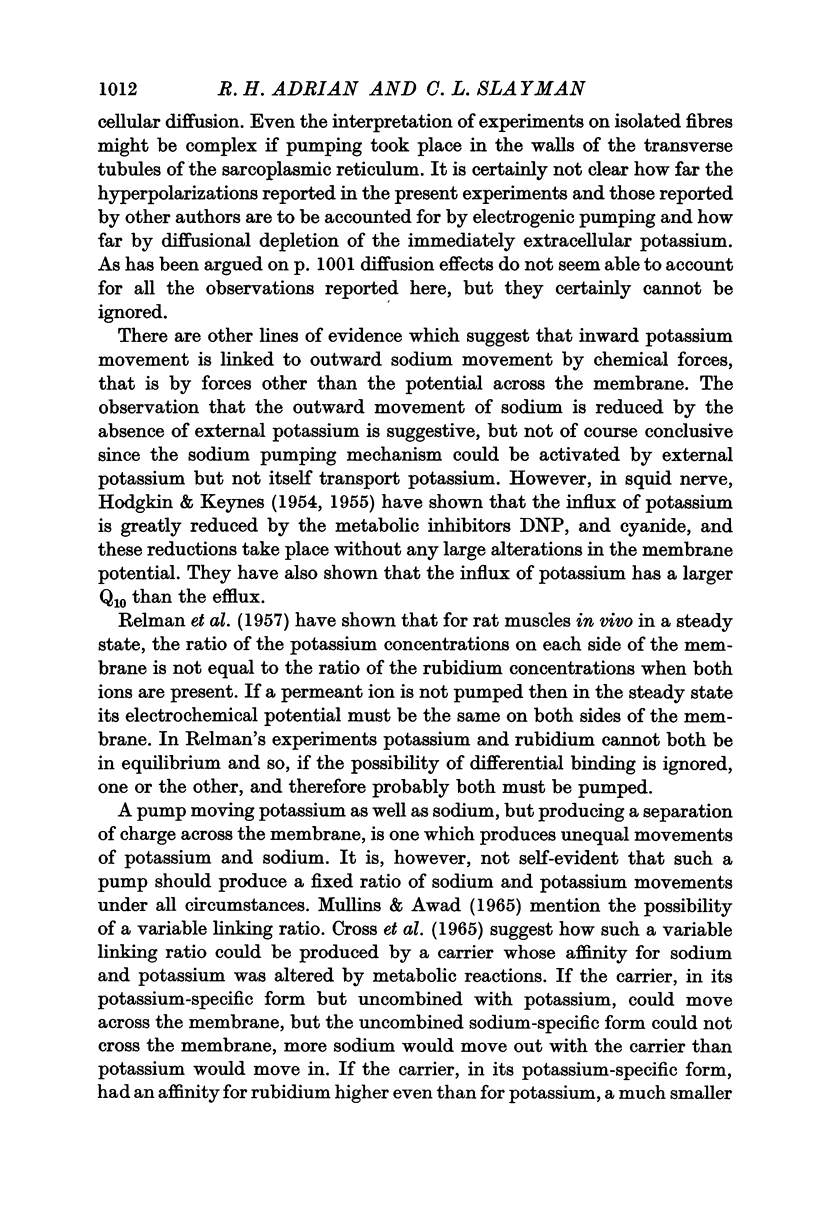
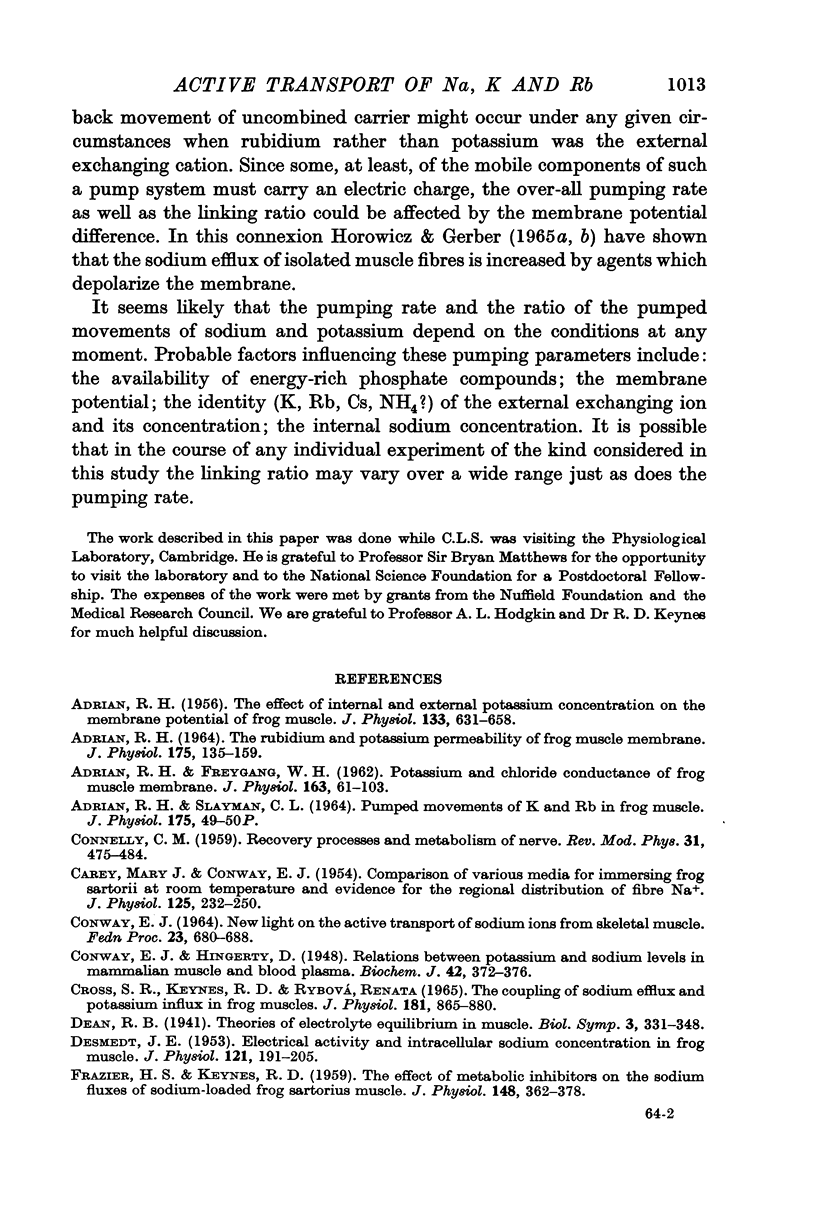
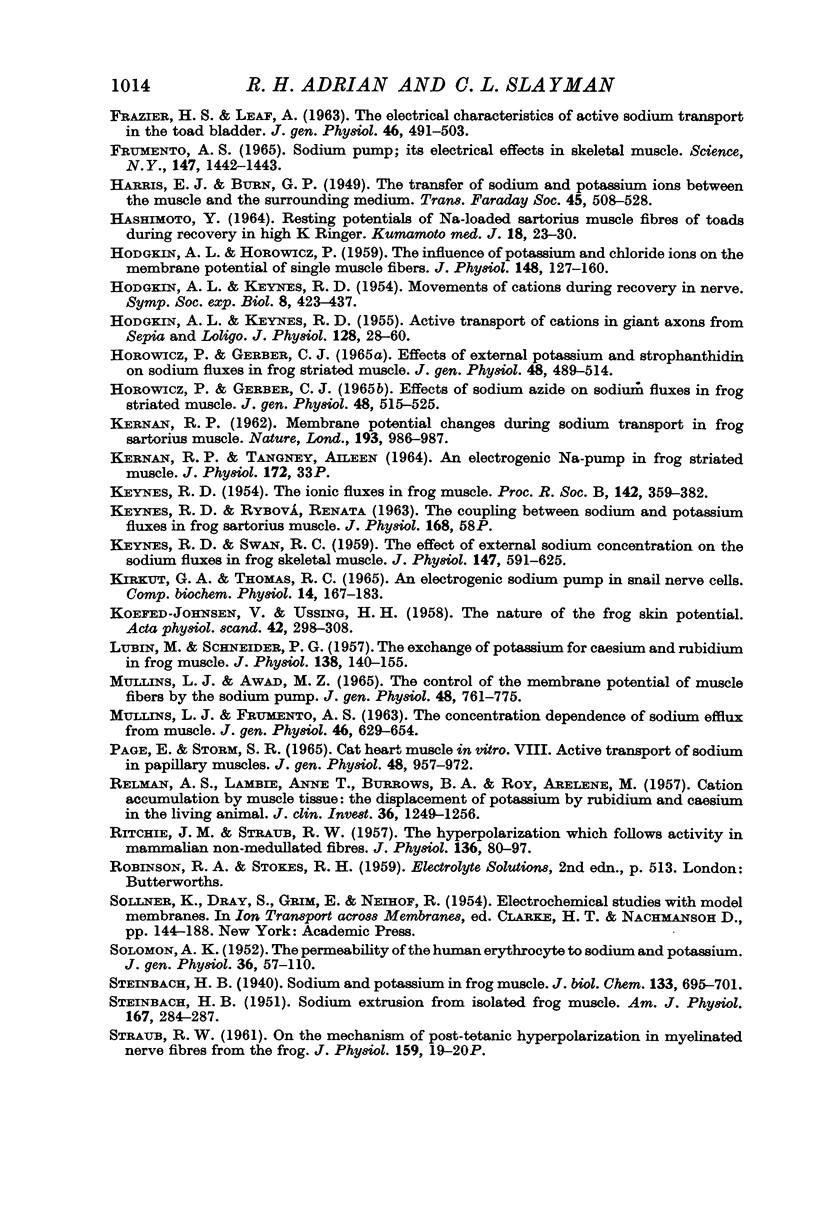
Selected References
These references are in PubMed. This may not be the complete list of references from this article.
- ADRIAN R. H. THE RUBIDIUM AND POTASSIUM PERMEABILITY OF FROG MUSCLE MEMBRANE. J Physiol. 1964 Dec;175:134–159. doi: 10.1113/jphysiol.1964.sp007508. [DOI] [PMC free article] [PubMed] [Google Scholar]
- ADRIAN R. H. The effect of internal and external potassium concentration on the membrane potential of frog muscle. J Physiol. 1956 Sep 27;133(3):631–658. doi: 10.1113/jphysiol.1956.sp005615. [DOI] [PMC free article] [PubMed] [Google Scholar]
- Adrian R. H., Freygang W. H. The potassium and chloride conductance of frog muscle membrane. J Physiol. 1962 Aug;163(1):61–103. doi: 10.1113/jphysiol.1962.sp006959. [DOI] [PMC free article] [PubMed] [Google Scholar]
- CAREY M. J., CONWAY E. J. Comparison of various media for immersing frog sartorii at room temperature, and evidence for the regional distribution of fibre Na+. J Physiol. 1954 Aug 27;125(2):232–250. doi: 10.1113/jphysiol.1954.sp005154. [DOI] [PMC free article] [PubMed] [Google Scholar]
- CONWAY E. J. NEW LIGHT ON THE ACTIVE TRANSPORT OF SODIUM IONS FROM SKELETAL MUSCLE. Fed Proc. 1964 May-Jun;23:680–688. [PubMed] [Google Scholar]
- Conway E. J., Hingerty D. Relations between potassium and sodium levels in mammalian muscle and blood plasma. Biochem J. 1948;42(3):372–376. doi: 10.1042/bj0420372. [DOI] [PMC free article] [PubMed] [Google Scholar]
- Cross S. B., Keynes R. D., Rybová R. The coupling of sodium efflux and potassium influx in frog muscle. J Physiol. 1965 Dec;181(4):865–880. doi: 10.1113/jphysiol.1965.sp007802. [DOI] [PMC free article] [PubMed] [Google Scholar]
- DESMEDT J. E. Electrical activity and intracellular sodium concentration in frog muscle. J Physiol. 1953 Jul;121(1):191–205. doi: 10.1113/jphysiol.1953.sp004940. [DOI] [PMC free article] [PubMed] [Google Scholar]
- FRAZIER H. S., KEYNES R. D. The effect of metabolic inhibitors on the sodium fluxes in sodium-loaded frog sartorius muscle. J Physiol. 1959 Oct;148:362–378. doi: 10.1113/jphysiol.1959.sp006293. [DOI] [PMC free article] [PubMed] [Google Scholar]
- FRAZIER H. S., LEAF A. The electrical characteristics of active sodium transport in the toad bladder. J Gen Physiol. 1963 Jan;46:491–503. doi: 10.1085/jgp.46.3.491. [DOI] [PMC free article] [PubMed] [Google Scholar]
- FRUMENTO A. S. SODIUM PUMP: ITS ELECTRICAL EFFECTS IN SKELETAL MUSCLE. Science. 1965 Mar 19;147(3664):1442–1443. doi: 10.1126/science.147.3664.1442. [DOI] [PubMed] [Google Scholar]
- HODGKIN A. L., HOROWICZ P. The influence of potassium and chloride ions on the membrane potential of single muscle fibres. J Physiol. 1959 Oct;148:127–160. doi: 10.1113/jphysiol.1959.sp006278. [DOI] [PMC free article] [PubMed] [Google Scholar]
- HODGKIN A. L., KEYNES R. D. Active transport of cations in giant axons from Sepia and Loligo. J Physiol. 1955 Apr 28;128(1):28–60. doi: 10.1113/jphysiol.1955.sp005290. [DOI] [PMC free article] [PubMed] [Google Scholar]
- HOROWICZ P., GERBER C. J. EFFECTS OF EXTERNAL POTASSIUM AND STROPHANTHIDIN ON SODIUM FLUXES IN FROG STRIATED MUSCLE. J Gen Physiol. 1965 Jan;48:489–514. doi: 10.1085/jgp.48.3.489. [DOI] [PMC free article] [PubMed] [Google Scholar]
- HOROWICZ P., GERBER C. J. EFFECTS OF SODIUM AZIDE ON SODIUM FLUXES IN FROG STRIATED MUSCLE. J Gen Physiol. 1965 Jan;48:515–525. doi: 10.1085/jgp.48.3.515. [DOI] [PMC free article] [PubMed] [Google Scholar]
- Hashimoto Y. Resting potentials of Na-loaded sartorius muscle fibres of toads during recovery in high K ringer. Kumamoto Med J. 1965 Mar 31;18(1):23–30. [PubMed] [Google Scholar]
- KERKUT G. A., THOMAS R. C. AN ELECTROGENIC SODIUM PUMP IN SNAIL NERVE CELLS. Comp Biochem Physiol. 1965 Jan;14:167–183. doi: 10.1016/0010-406x(65)90017-4. [DOI] [PubMed] [Google Scholar]
- KERNAN R. P. Membrane potential changes during sodium transport in frog sartorius muscle. Nature. 1962 Mar 10;193:986–987. doi: 10.1038/193986a0. [DOI] [PubMed] [Google Scholar]
- KEYNES R. D., SWAN R. C. The effect of external sodium concentration on the sodium fluxes in frog skeletal muscle. J Physiol. 1959 Oct;147:591–625. doi: 10.1113/jphysiol.1959.sp006264. [DOI] [PMC free article] [PubMed] [Google Scholar]
- KEYNES R. D. The ionic fluxes in frog muscle. Proc R Soc Lond B Biol Sci. 1954 May 27;142(908):359–382. doi: 10.1098/rspb.1954.0030. [DOI] [PubMed] [Google Scholar]
- KOEFOED-JOHNSEN V., USSING H. H. The nature of the frog skin potential. Acta Physiol Scand. 1958 Jun 2;42(3-4):298–308. doi: 10.1111/j.1748-1716.1958.tb01563.x. [DOI] [PubMed] [Google Scholar]
- LUBIN M., SCHNEIDER P. B. The exchange of potassium for caesium and rubidium in frog muscle. J Physiol. 1957 Aug 29;138(1):140–155. doi: 10.1113/jphysiol.1957.sp005842. [DOI] [PMC free article] [PubMed] [Google Scholar]
- MULLINS L. J., AWAD M. Z. THE CONTROL OF THE MEMBRANE POTENTIAL OF MUSCLE FIBERS BY THE SODIUM PUMP. J Gen Physiol. 1965 May;48:761–775. doi: 10.1085/jgp.48.5.761. [DOI] [PMC free article] [PubMed] [Google Scholar]
- MULLINS L. J., FRUMENTO A. S. The concentration dependence of sodium efflux from muscle. J Gen Physiol. 1963 Mar;46:629–654. doi: 10.1085/jgp.46.4.629. [DOI] [PMC free article] [PubMed] [Google Scholar]
- PAGE E., STORN S. R. CAT HEART MUSCLE IN VITRO. 8. ACTIVE TRANSPORT OF SODIUM IN PAPILLARY MUSCLES. J Gen Physiol. 1965 May;48:957–972. doi: 10.1085/jgp.48.5.957. [DOI] [PMC free article] [PubMed] [Google Scholar]
- RELMAN A. S., LAMBIE A. T., BURROWS B. A., ROY A. M. Cation accumulation by muscle tissue: the displacement of potassium by rubidium and cesium in the living animal. J Clin Invest. 1957 Aug;36(8):1249–1256. doi: 10.1172/JCI103522. [DOI] [PMC free article] [PubMed] [Google Scholar]
- RITCHIE J. M., STRAUB R. W. The hyperpolarization which follows activity in mammalian non-medullated fibres. J Physiol. 1957 Apr 3;136(1):80–97. doi: 10.1113/jphysiol.1957.sp005744. [DOI] [PMC free article] [PubMed] [Google Scholar]
- SOLOMON A. K. The permeability of the human erythrocyte to sodium and potassium. J Gen Physiol. 1952 May;36(1):57–110. doi: 10.1085/jgp.36.1.57. [DOI] [PMC free article] [PubMed] [Google Scholar]
- STEINBACH H. B. Sodium extrusion from isolated frog muscle. Am J Physiol. 1951 Oct;167(1):284–287. doi: 10.1152/ajplegacy.1951.167.1.284. [DOI] [PubMed] [Google Scholar]