Abstract
Cell depolarization in hemorrhagic shock has been attributed to hypoperfusion, but the mechanism remains unclear. Suspensions of single cell lines loaded with the potential-sensitive fluorescent dye bis-(1,3-dibutylbarbiturioc acid) trimethine oxonal (DIBAC) and exposed for 30 minutes to rat plasma drawn either before or after hemorrhagic shock (bled 20 mL/kg: mean arterial blood pressure less than 40 mmHg) were studied. Plasma drawn after, but not before, hemorrhage led to partial depolarization regardless of cell type (rat H9C2 skeletal muscle, A-10 smooth muscle, C-9 liver, adrenal, kidney, red blood cell [RBC], white blood cell [WBC]) or species (cat, dog, pig RBC; cat WBC; mouse C2C12 skeletal muscle; and human intestinal smooth muscle [HISM]). Dialysis did not remove the factor(s), suggesting a molecular weight of more than 10,000 daltons. The factor appeared within 5 minutes of shock. The depolarization amplitude increased as a function of plasma concentration and demonstrated saturation kinetics indicating specific receptor binding. Cells were equivalently oxygenated, excluding hypoperfusion as a necessary condition for depolarization. Tumor necrosis factor or platelet activating factor alone or in combination were not effective in this system. Stable measurements can be obtained with this noninvasive system that avoids cell injury consequent to cell impalement with electrodes. This system provides a sensitive in vitro bioassay that should permit identification of the plasma factors mediating cell depolarization, as well as definition of the responsible intracellular mechanisms.
Full text
PDF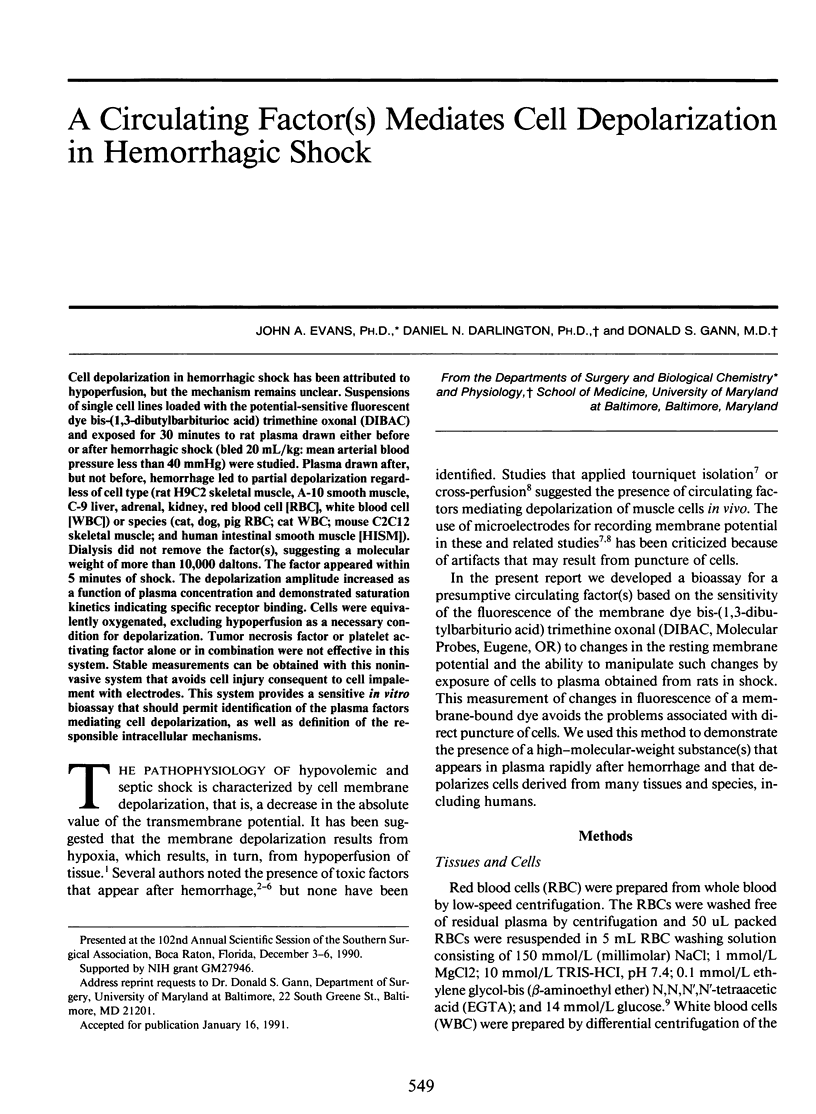
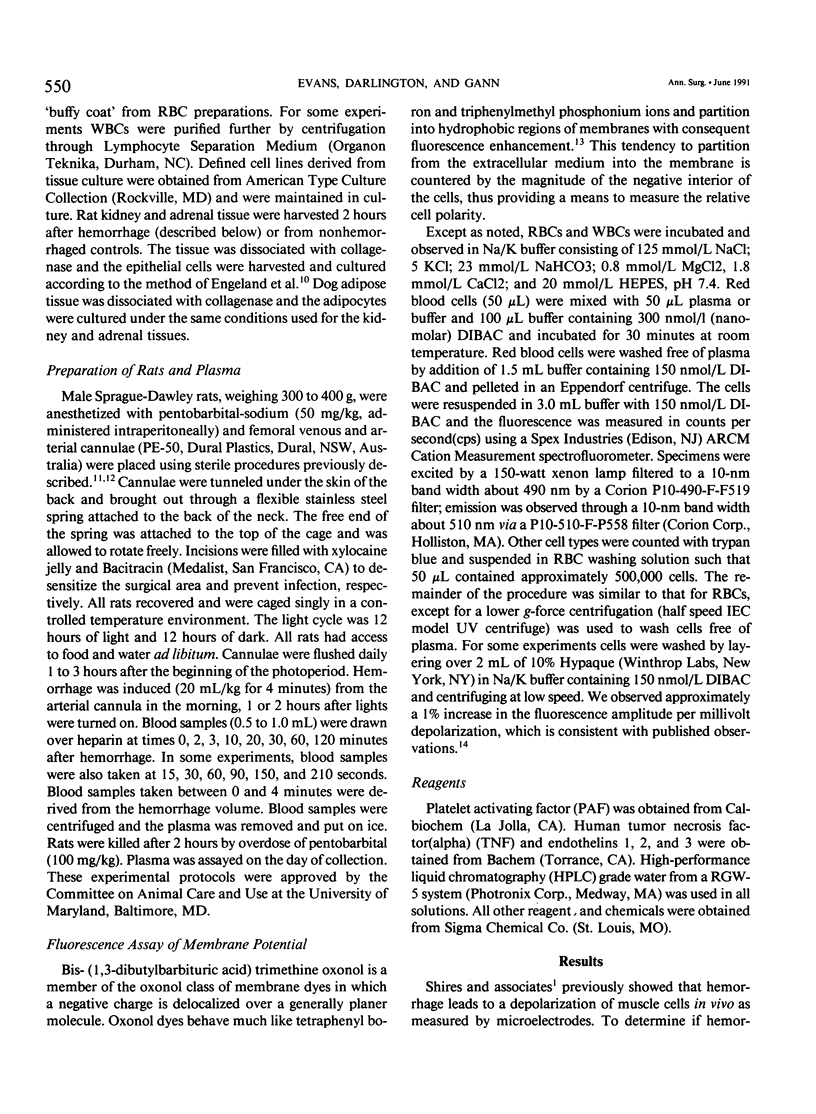
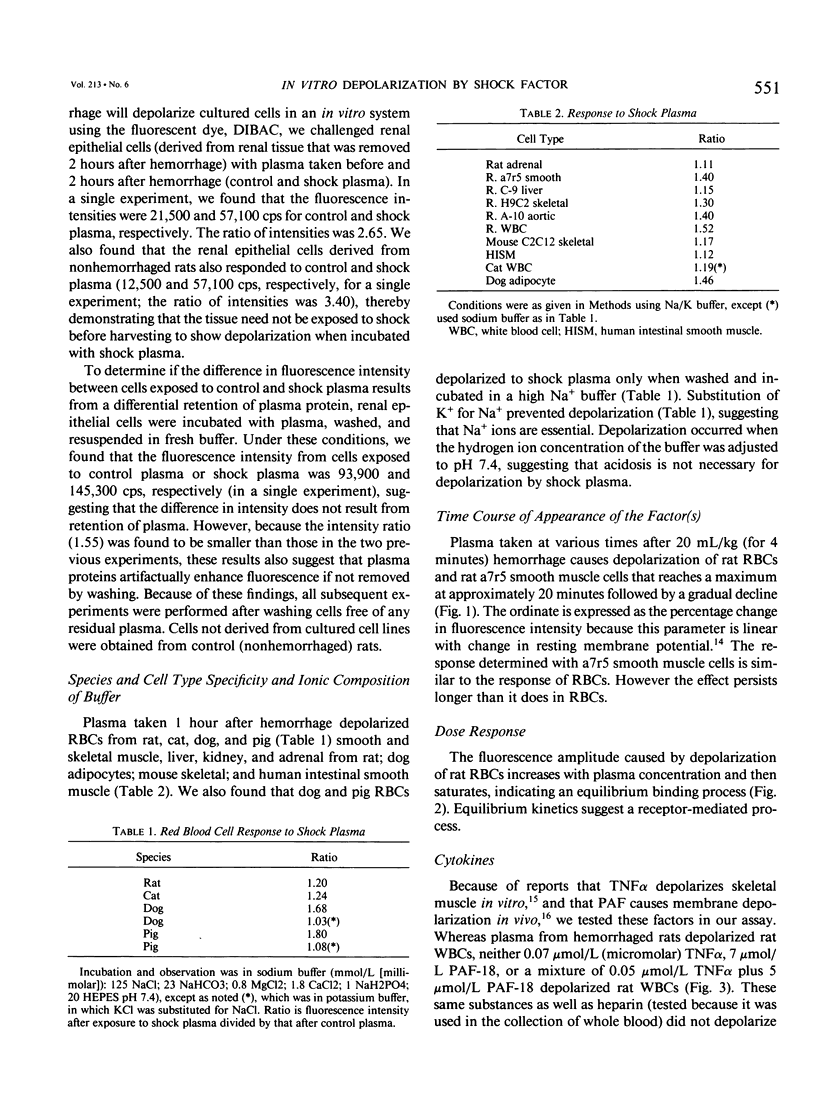
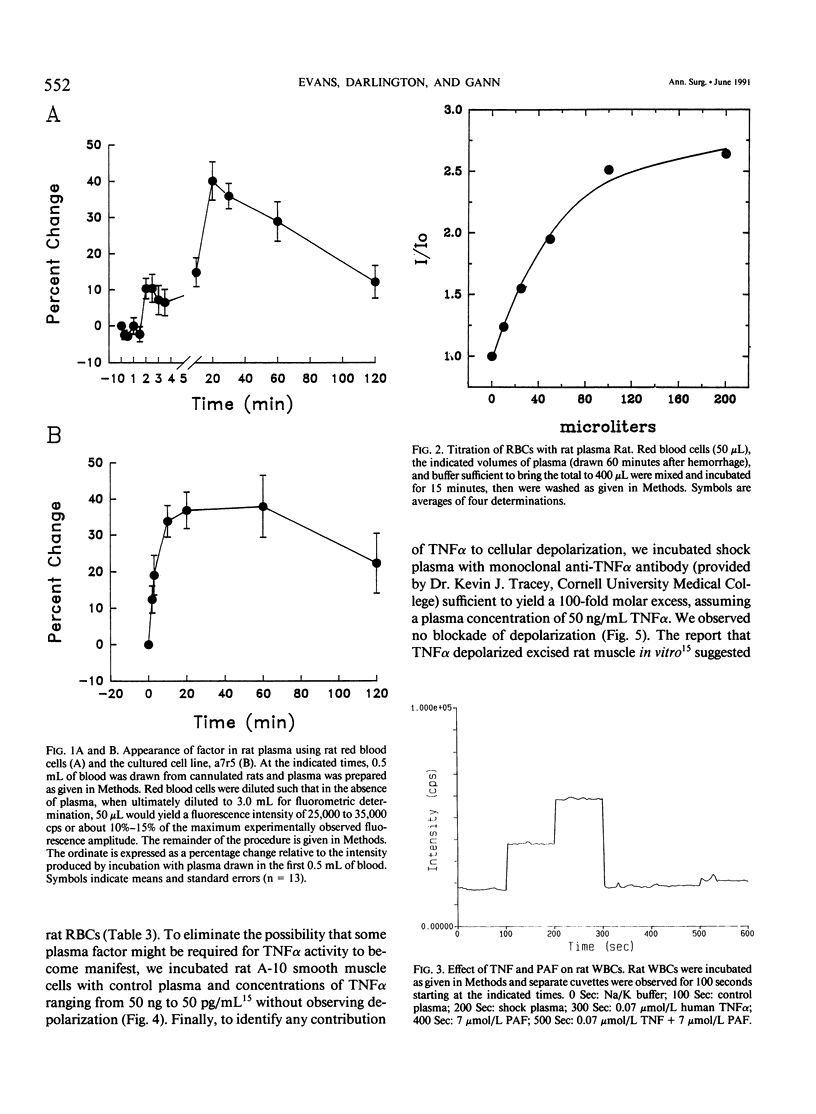
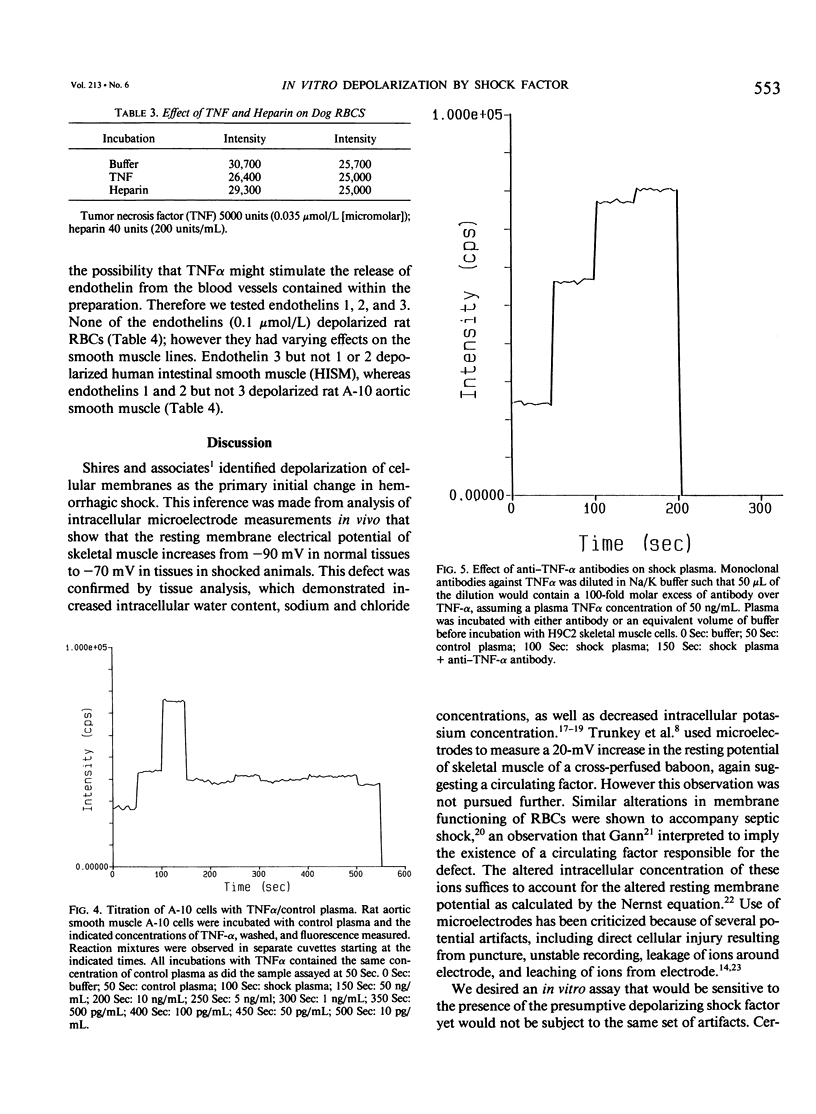
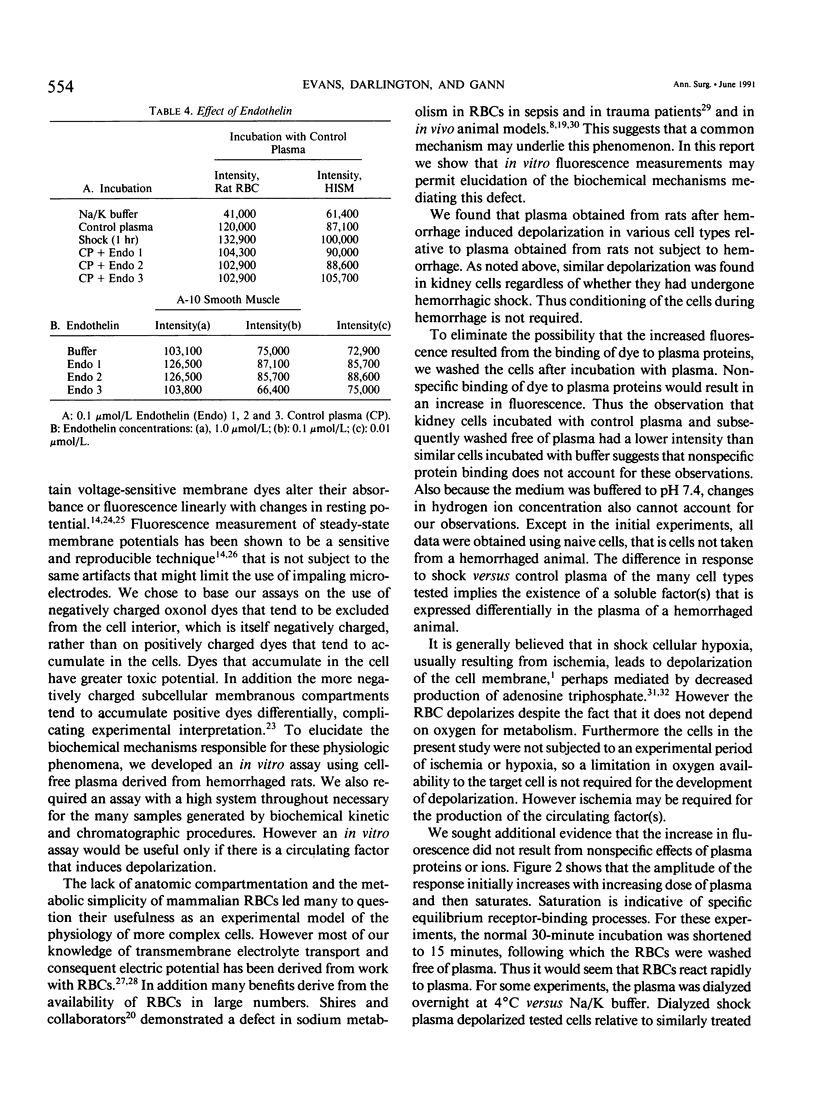
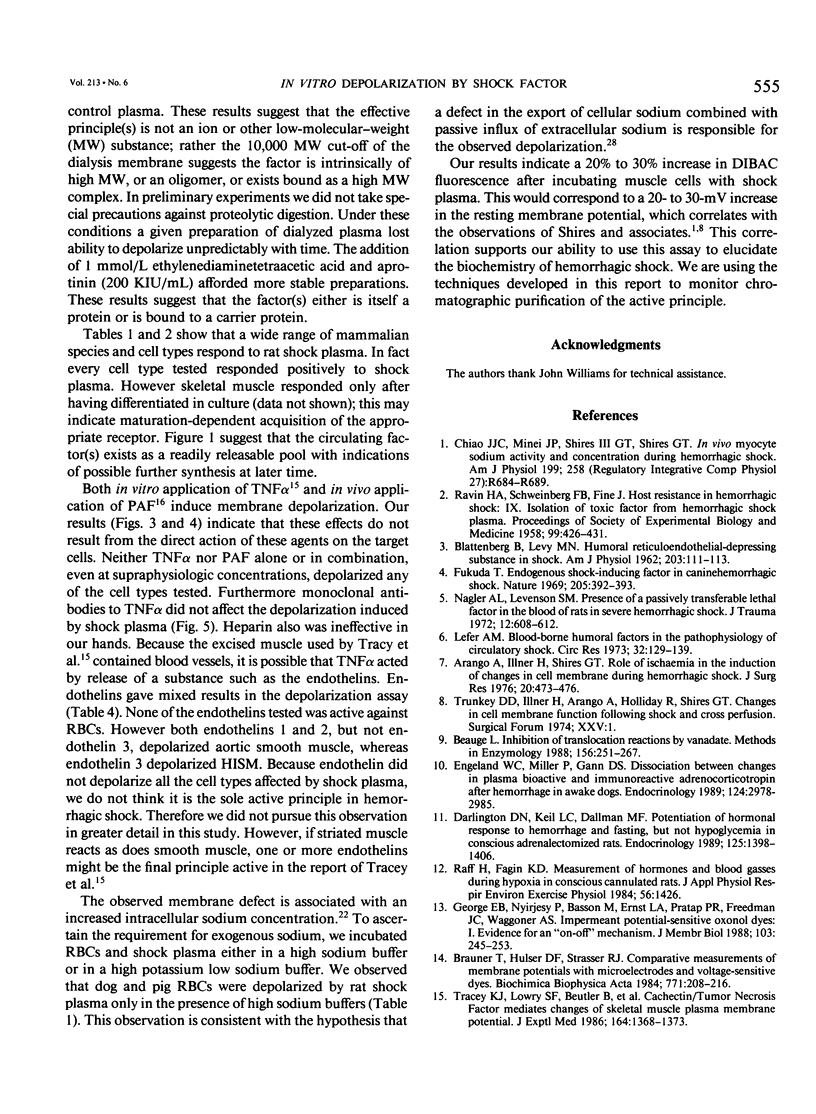
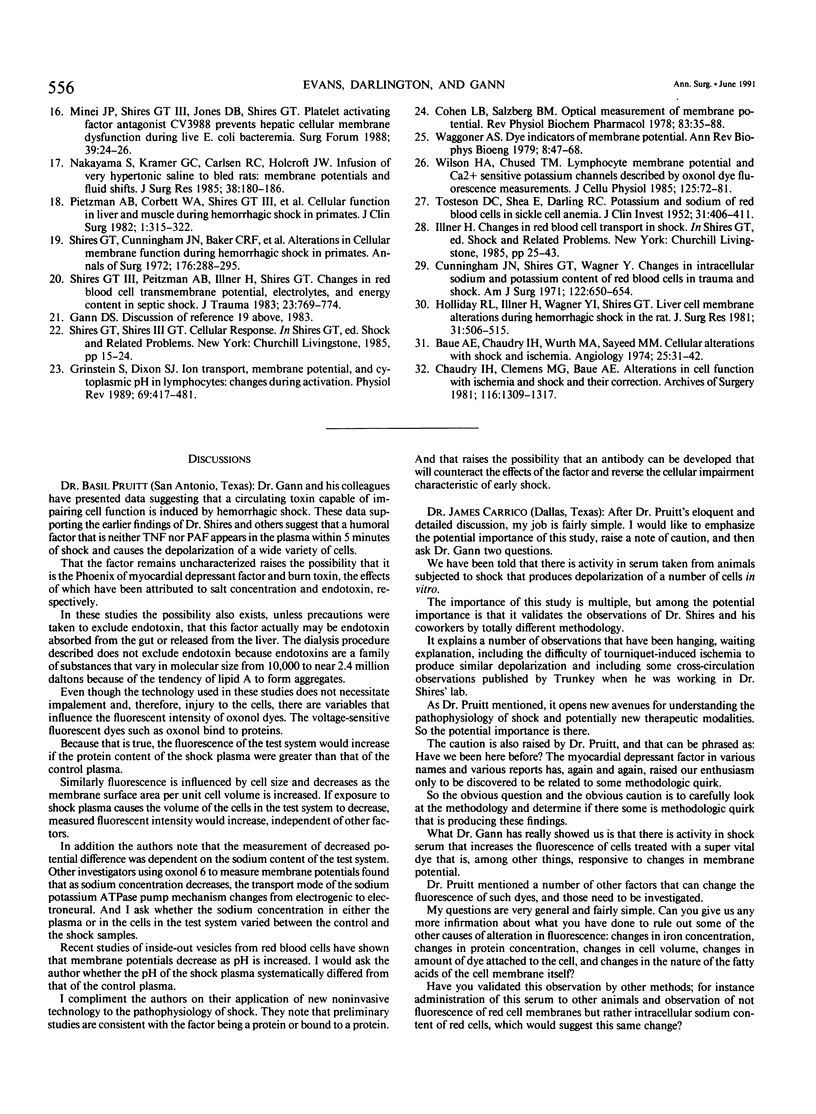
Selected References
These references are in PubMed. This may not be the complete list of references from this article.
- Arango A., Illner H., Shires G. T. Role of ischemia in the induction of changes in cell membrane during hemorrhagic shock. J Surg Res. 1976 May;20(5):473–476. doi: 10.1016/0022-4804(76)90122-0. [DOI] [PubMed] [Google Scholar]
- BLATTBERG B., LEVY M. N. Mechanism of depression of reticuloendothelial system in shock. Am J Physiol. 1962 Jul;203:111–113. doi: 10.1152/ajplegacy.1962.203.1.111. [DOI] [PubMed] [Google Scholar]
- Baue A. E., Chaudry I. H., Wurth M. A., Sayeed M. M. Cellular alterations with shock and ischemia. Angiology. 1974 Jan;25(1):31–42. doi: 10.1177/000331977402500106. [DOI] [PubMed] [Google Scholar]
- Beaugé L. Inhibition of translocation reactions by vanadate. Methods Enzymol. 1988;156:251–267. doi: 10.1016/0076-6879(88)56026-3. [DOI] [PubMed] [Google Scholar]
- Bräuner T., Hülser D. F., Strasser R. J. Comparative measurements of membrane potentials with microelectrodes and voltage-sensitive dyes. Biochim Biophys Acta. 1984 Apr 11;771(2):208–216. doi: 10.1016/0005-2736(84)90535-2. [DOI] [PubMed] [Google Scholar]
- Chaudry I. H., Clemens M. G., Baue A. E. Alterations in cell function with ischemia and shock and their correction. Arch Surg. 1981 Oct;116(10):1309–1317. doi: 10.1001/archsurg.1981.01380220053009. [DOI] [PubMed] [Google Scholar]
- Cohen L. B., Salzberg B. M. Optical measurement of membrane potential. Rev Physiol Biochem Pharmacol. 1978;83:35–88. doi: 10.1007/3-540-08907-1_2. [DOI] [PubMed] [Google Scholar]
- Cunningham J. N., Jr, Shires G. T., Wagner Y. Changes in intracellular sodium and potassium content of red blood cells in trauma and shock. Am J Surg. 1971 Nov;122(5):650–654. doi: 10.1016/0002-9610(71)90293-5. [DOI] [PubMed] [Google Scholar]
- Darlington D. N., Keil L. C., Dallman M. F. Potentiation of hormonal responses to hemorrhage and fasting, but not hypoglycemia in conscious adrenalectomized rats. Endocrinology. 1989 Sep;125(3):1398–1406. doi: 10.1210/endo-125-3-1398. [DOI] [PubMed] [Google Scholar]
- Engeland W. C., Miller P., Gann D. S. Dissociation between changes in plasma bioactive and immunoreactive adrenocorticotropin after hemorrhage in awake dogs. Endocrinology. 1989 Jun;124(6):2978–2985. doi: 10.1210/endo-124-6-2978. [DOI] [PubMed] [Google Scholar]
- FUKUDA T. ENDOGENOUS SHOCK-INDUCING FACTOR IN CANINE HAEMORRHAGIC SHOCK. Nature. 1965 Jan 23;205:392–393. doi: 10.1038/205392a0. [DOI] [PubMed] [Google Scholar]
- George E. B., Nyirjesy P., Basson M., Ernst L. A., Pratap P. R., Freedman J. C., Waggoner A. S. Impermeant potential-sensitive oxonol dyes: I. Evidence for an "on-off" mechanism. J Membr Biol. 1988 Aug;103(3):245–253. doi: 10.1007/BF01993984. [DOI] [PubMed] [Google Scholar]
- Grinstein S., Dixon S. J. Ion transport, membrane potential, and cytoplasmic pH in lymphocytes: changes during activation. Physiol Rev. 1989 Apr;69(2):417–481. doi: 10.1152/physrev.1989.69.2.417. [DOI] [PubMed] [Google Scholar]
- Holliday R. L., Illner H. P., Shires G. T. Liver cell membrane alterations during hemorrhagic shock in the rat. J Surg Res. 1981 Dec;31(6):506–515. doi: 10.1016/0022-4804(81)90189-x. [DOI] [PubMed] [Google Scholar]
- Lefer A. M. Blood-borne humoral factors in the pathophysiology of circulatory shock. Circ Res. 1973 Feb;32(2):129–139. doi: 10.1161/01.res.32.2.129. [DOI] [PubMed] [Google Scholar]
- Nagler A. L., Levenson S. M. Presence of a passively transferable lethal factor in the blood of rats in severe hemorrhagic shock. J Trauma. 1972 Jul;12(7):608–612. doi: 10.1097/00005373-197207000-00009. [DOI] [PubMed] [Google Scholar]
- Nakayama S., Kramer G. C., Carlsen R. C., Holcroft J. W. Infusion of very hypertonic saline to bled rats: membrane potentials and fluid shifts. J Surg Res. 1985 Feb;38(2):180–186. doi: 10.1016/0022-4804(85)90025-3. [DOI] [PubMed] [Google Scholar]
- Raff H., Fagin K. D. Measurement of hormones and blood gases during hypoxia in conscious cannulated rats. J Appl Physiol Respir Environ Exerc Physiol. 1984 May;56(5):1426–1430. doi: 10.1152/jappl.1984.56.5.1426. [DOI] [PubMed] [Google Scholar]
- Shires G. T., 3rd, Peitzman A. B., Illner H., Shires G. T. Changes in red blood cell transmembrane potential, electrolytes, and energy content in septic shock. J Trauma. 1983 Sep;23(9):769–774. doi: 10.1097/00005373-198309000-00001. [DOI] [PubMed] [Google Scholar]
- Shires G. T., Cunningham J. N., Backer C. R., Reeder S. F., Illner H., Wagner I. Y., Maher J. Alterations in cellular membrane function during hemorrhagic shock in primates. Ann Surg. 1972 Sep;176(3):288–295. doi: 10.1097/00000658-197209000-00004. [DOI] [PMC free article] [PubMed] [Google Scholar]
- TOSTESON D. C., SHEA E., DARLING R. C. Potassium and sodium of red blood cells in sickle cell anemia. J Clin Invest. 1952 Apr;31(4):406–411. doi: 10.1172/JCI102623. [DOI] [PMC free article] [PubMed] [Google Scholar]
- Tracey K. J., Lowry S. F., Beutler B., Cerami A., Albert J. D., Shires G. T. Cachectin/tumor necrosis factor mediates changes of skeletal muscle plasma membrane potential. J Exp Med. 1986 Oct 1;164(4):1368–1373. doi: 10.1084/jem.164.4.1368. [DOI] [PMC free article] [PubMed] [Google Scholar]
- Trunkey D. D., Illner H., Arango A., Holiday R., Shires G. T. Changes in cell membrane function following shock and cross-perfusion. Surg Forum. 1974;25(0):1–3. [PubMed] [Google Scholar]
- Waggoner A. S. Dye indicators of membrane potential. Annu Rev Biophys Bioeng. 1979;8:47–68. doi: 10.1146/annurev.bb.08.060179.000403. [DOI] [PubMed] [Google Scholar]
- Wilson H. A., Chused T. M. Lymphocyte membrane potential and Ca2+-sensitive potassium channels described by oxonol dye fluorescence measurements. J Cell Physiol. 1985 Oct;125(1):72–81. doi: 10.1002/jcp.1041250110. [DOI] [PubMed] [Google Scholar]