Abstract
1. When resealed ghosts containing adenosine triphosphate (ATP), magnesium and sodium were incubated in a medium containing potassium, ATP was hydrolysed vigorously by a ouabain-sensitive mechanism. If the ghosts contained potassium instead of or in addition to sodium, and the external solution contained sodium but no potassium, there was little ouabain-sensitive hydrolysis of ATP. As it is known that the ouabain-sensitive ATPase in fragmented ghosts requires both sodium and potassium ions, these results show that the ATPase is activated by potassium externally and by sodium internally, and suggest that the ions activating the ATPase are the ions that are transported.
2. Resealed ghosts containing ATP, magnesium and sodium were incubated in sodium-free media containing potassium, with and without ouabain, and the rate of loss of sodium and rate of hydrolysis of ATP were measured. The hydrolysis of 1 molecule of ATP by the ouabain-sensitive mechanism was accompanied by the ouabain-sensitive loss of about 3 sodium ions.
3. 24Na and 42K were used to measure sodium efflux and potassium influx in identical batches of fresh red cells under the same conditions and at the same time. Each flux was measured in the presence and absence of ouabain. The ratio (ouabain-sensitive sodium efflux)/(ouabain-sensitive potassium influx) was significantly greater than 1 (1·20 ± 0·01 and 1·35 ± 0·01 in two experiments). If a small fraction of the potassium influx represented a ouabain-sensitive potassium: potassium exchange, the ratio of the numbers of ions moved in the sodium: potassium exchange catalysed by the pump must have been even further from unity.
4. Resealed ghosts containing [γ-32P]ATP, magnesium, 24Na and orthophosphate were incubated in balanced salt solutions with and without potassium and with and without ouabain. A comparison of sodium efflux, estimated from 24Na loss, with ATP hydrolysis, estimated from the formation of [32P]orthophosphate, showed that the sodium:sodium exchange in a potassium-free medium was accompanied by little or no ouabain-sensitive hydrolysis of ATP.
5. Experiments on intact red cells loaded with 24Na showed that both sodium:sodium exchange in a potassium-free medium, and sodium:potassium exchange in a medium containing potassium, were partially inhibited by oligomycin (1-10 μg/ml.). Inhibition of the sodium:potassium exchange was not affected by raising the external potassium concentration.
Full text
PDF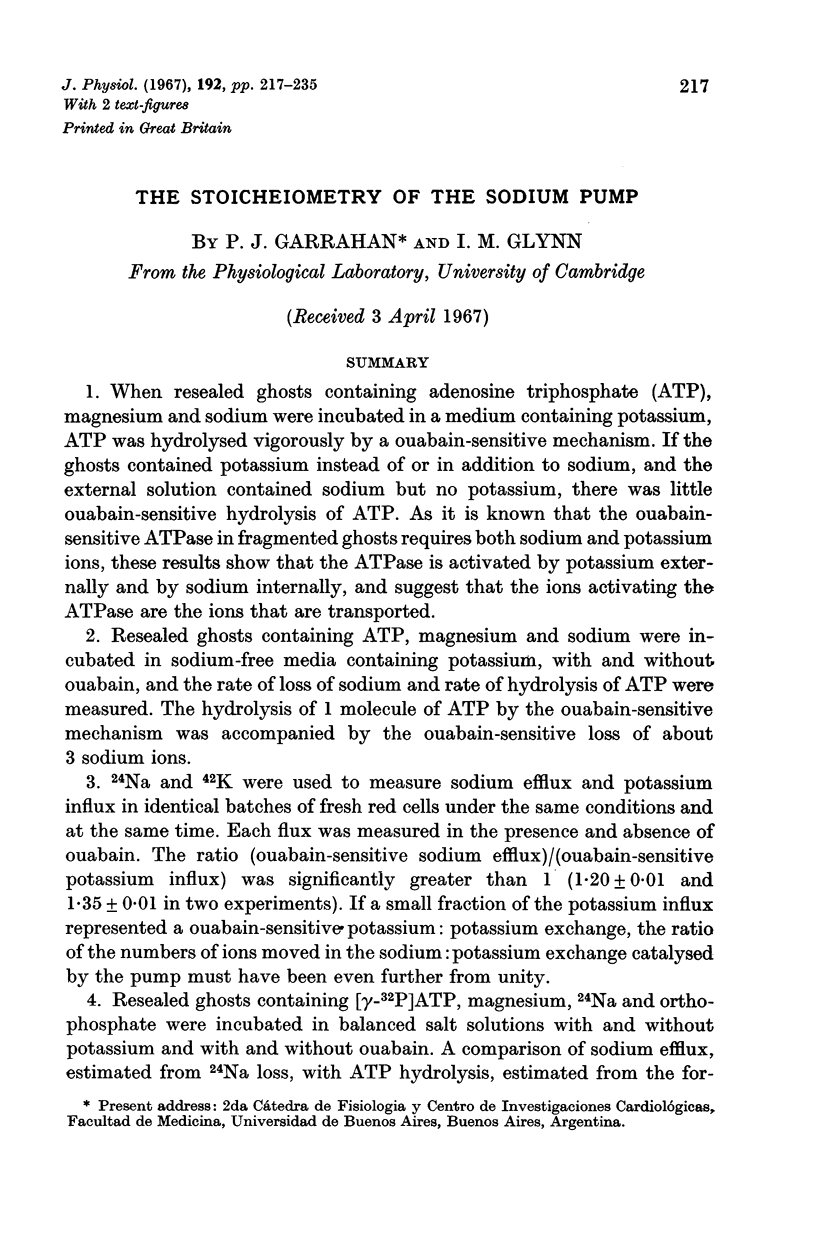
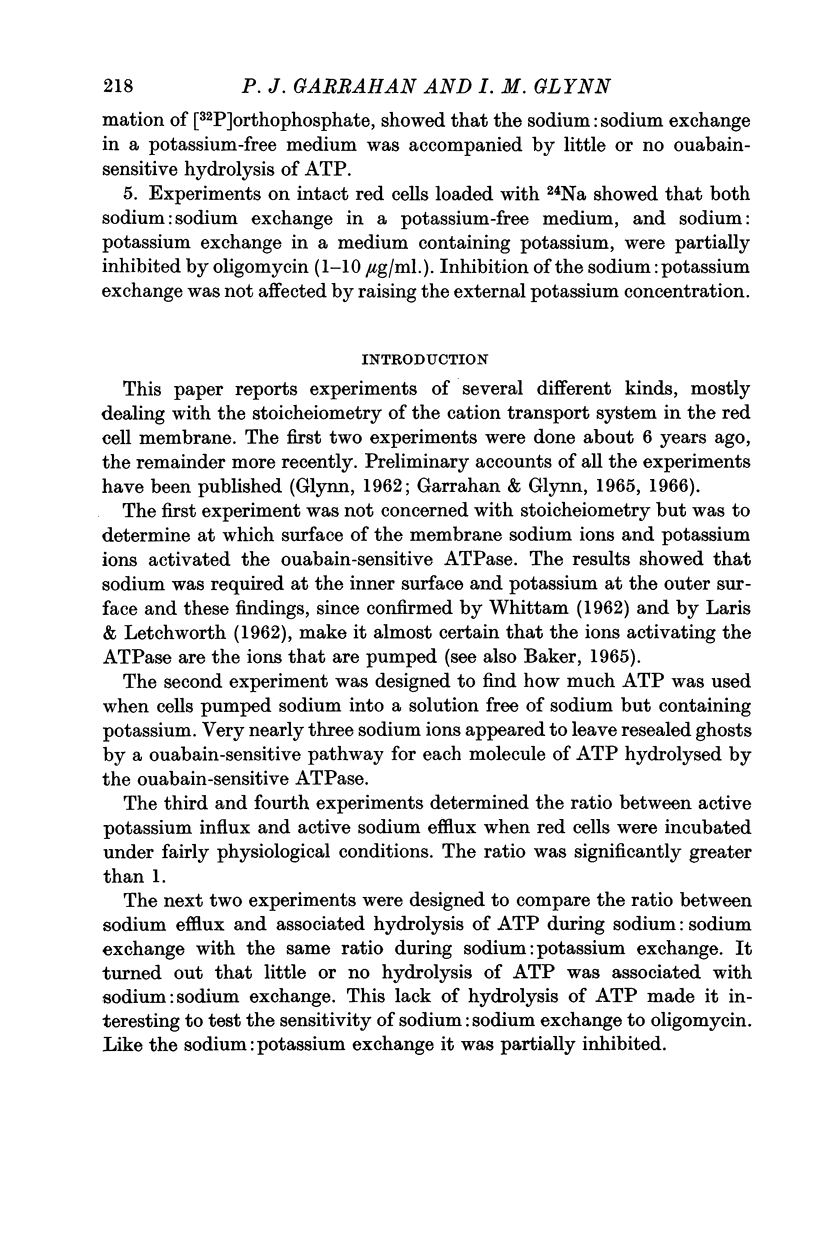
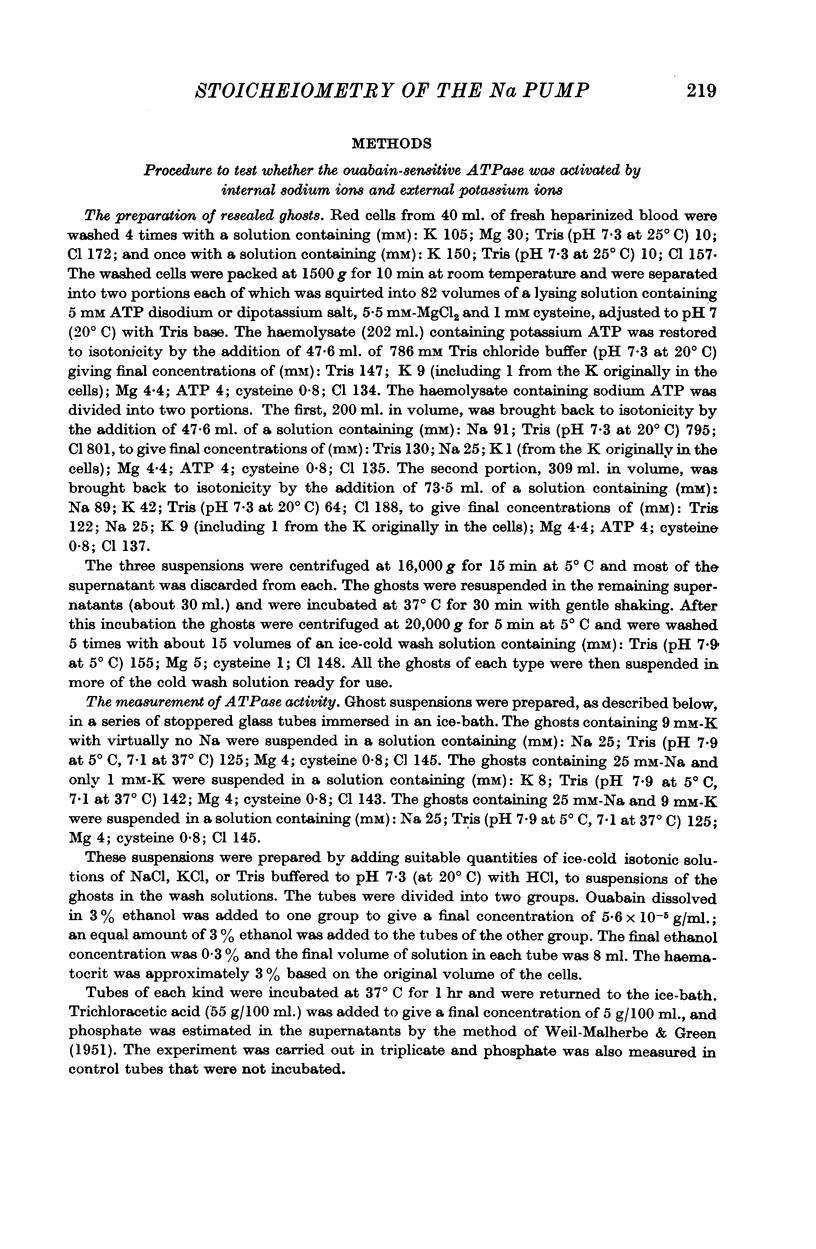
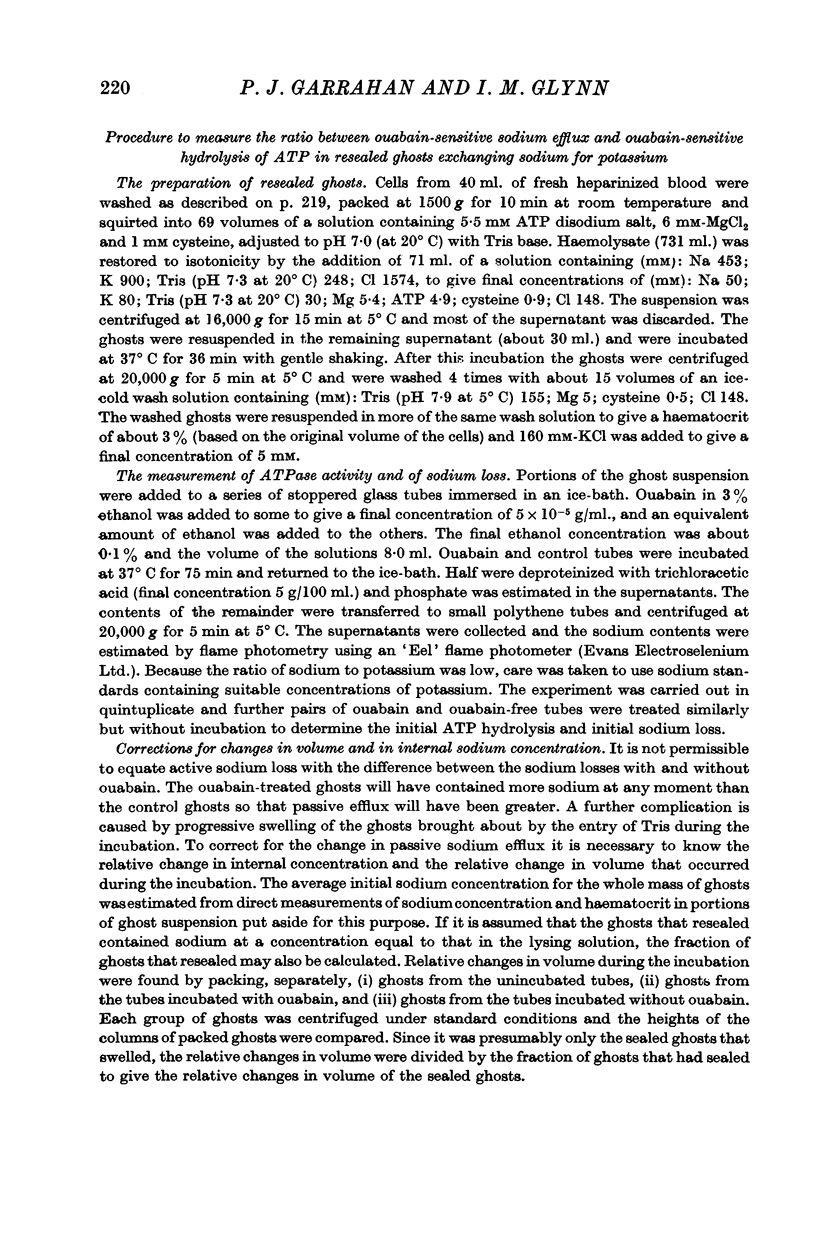
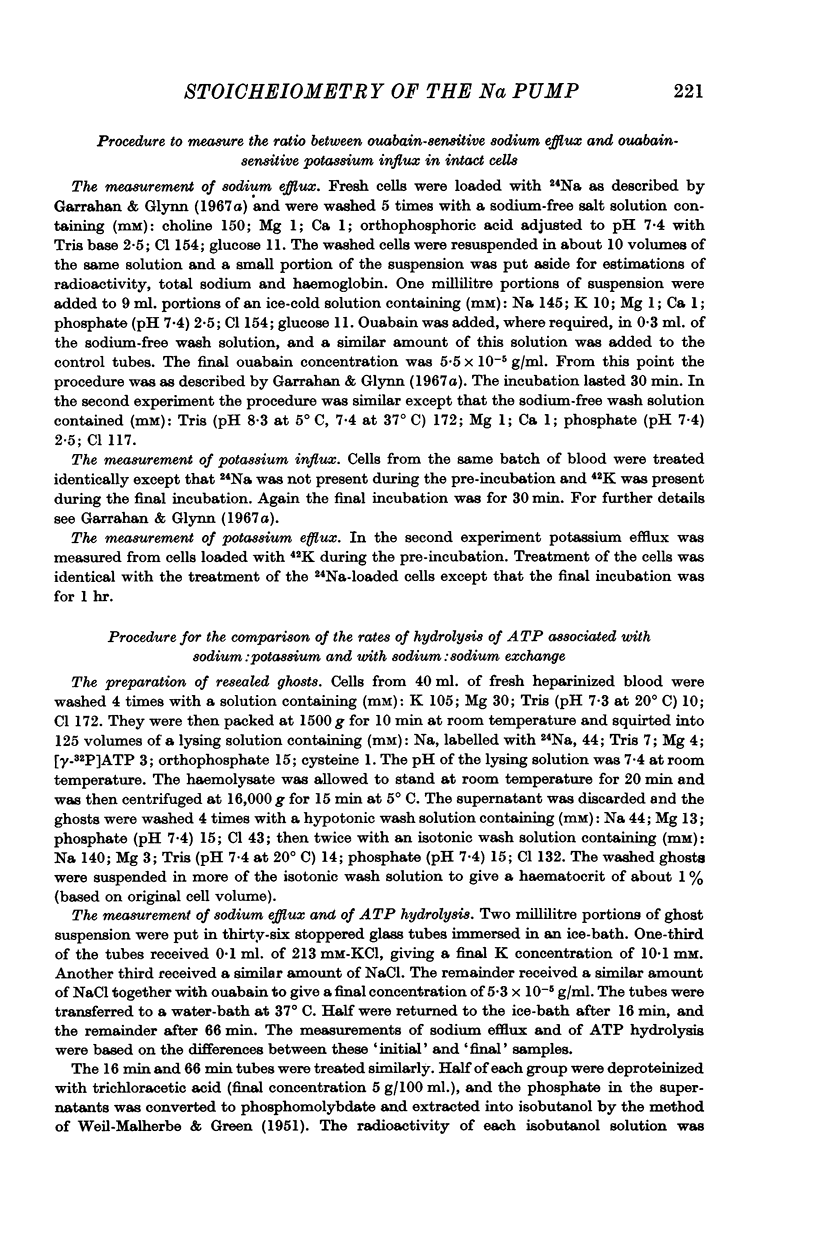
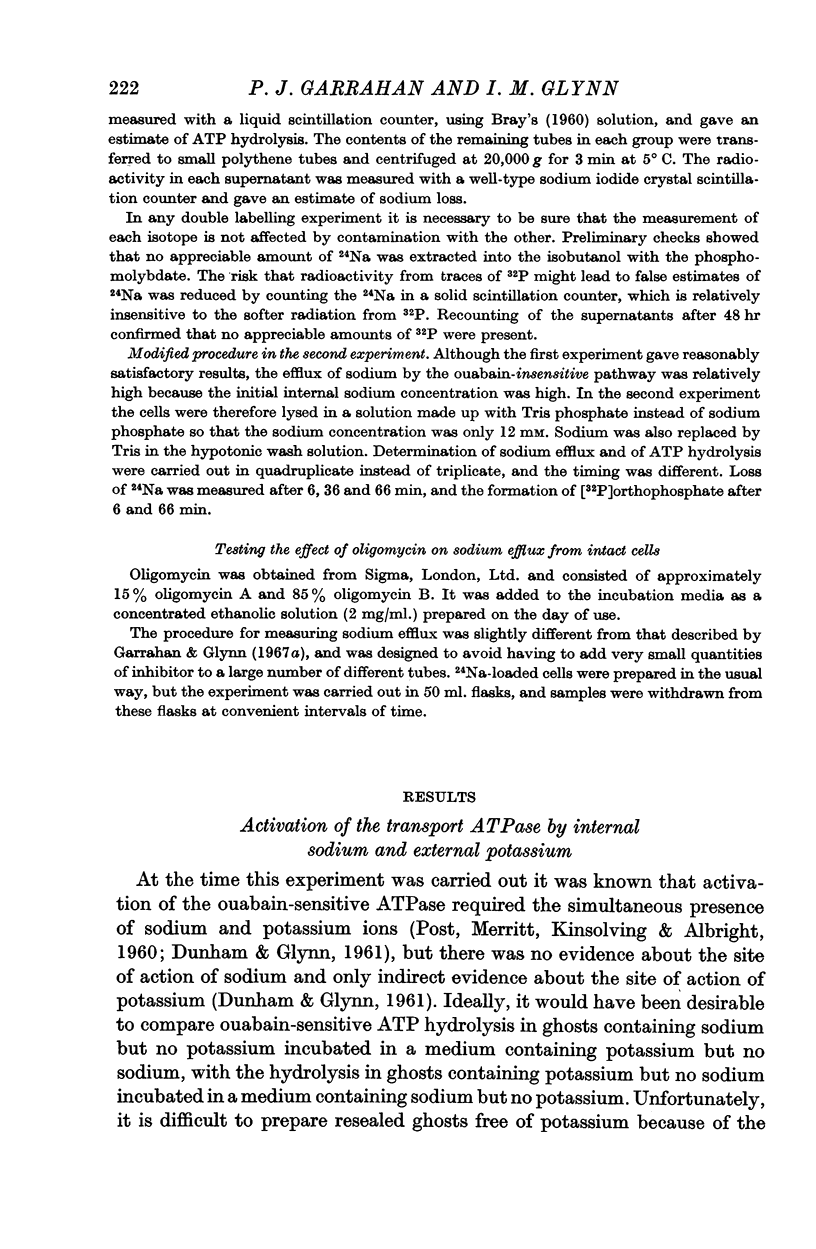
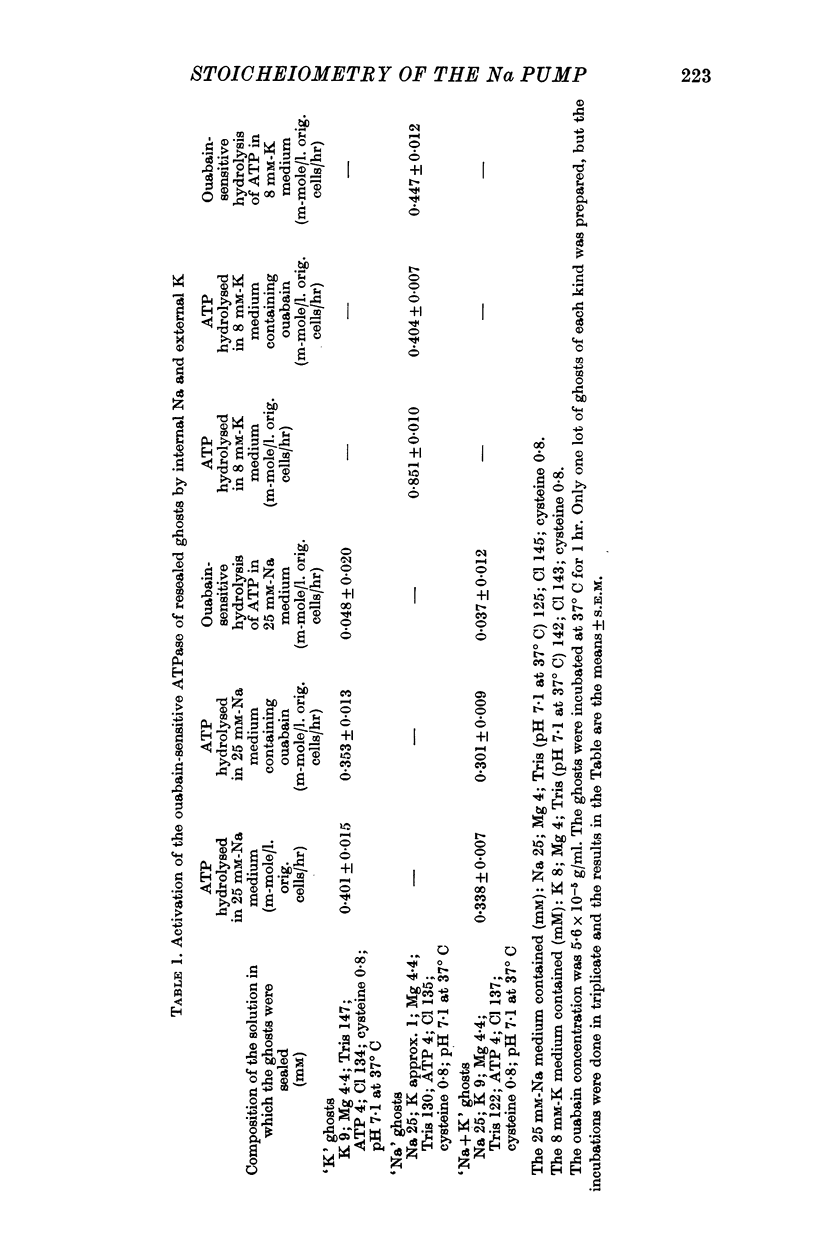
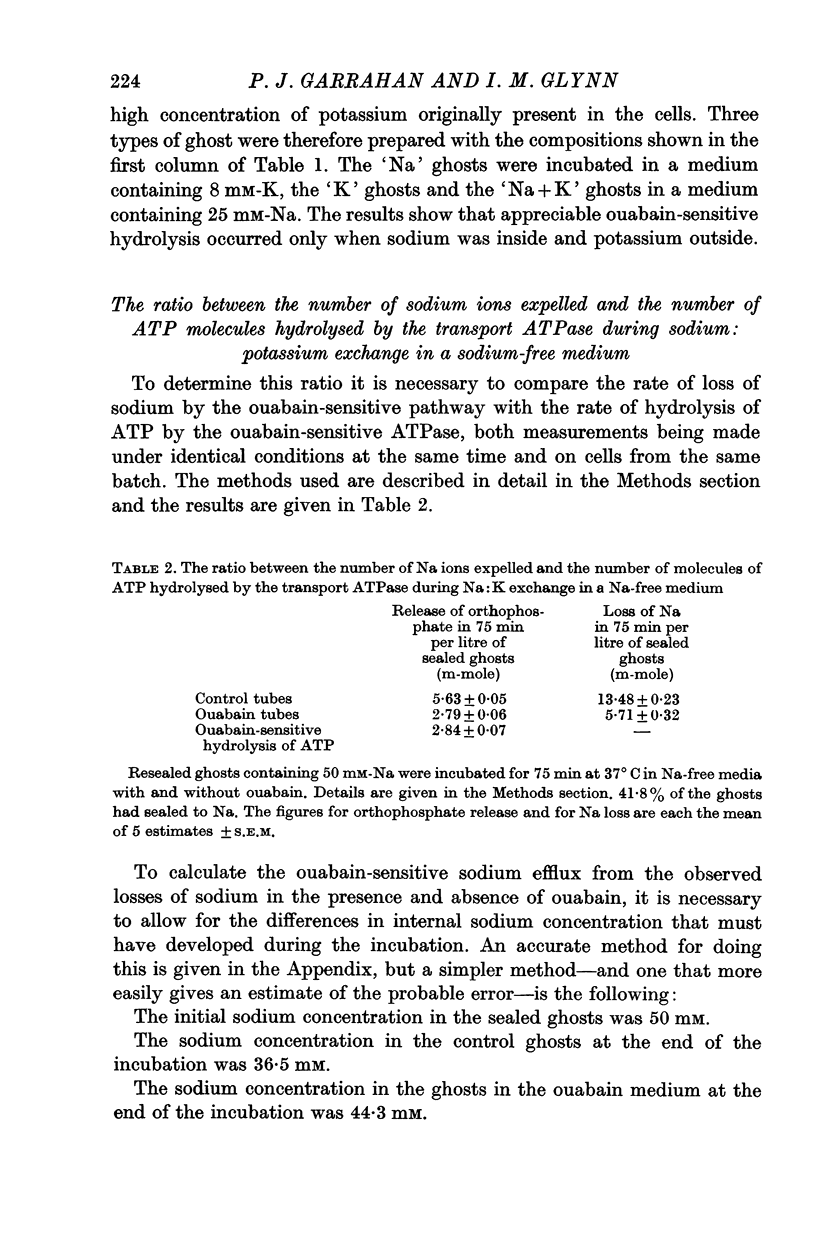
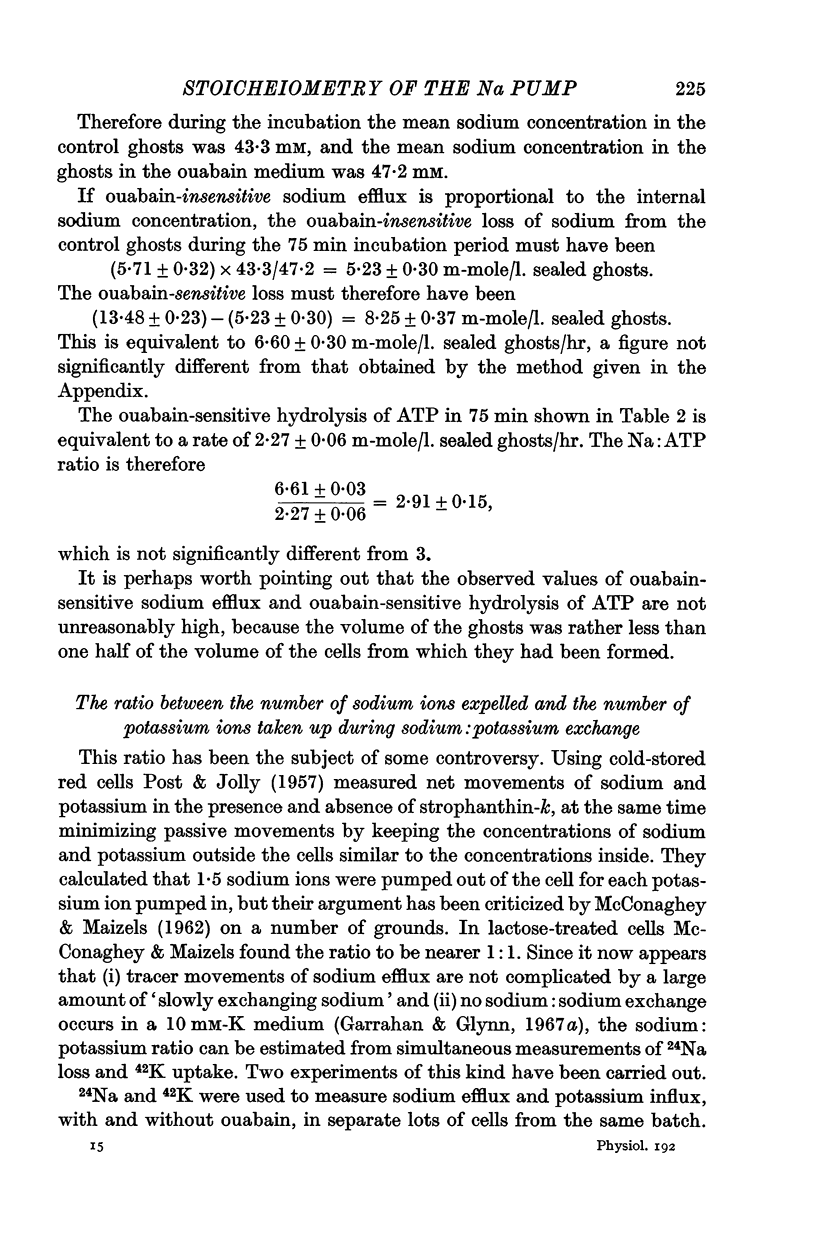
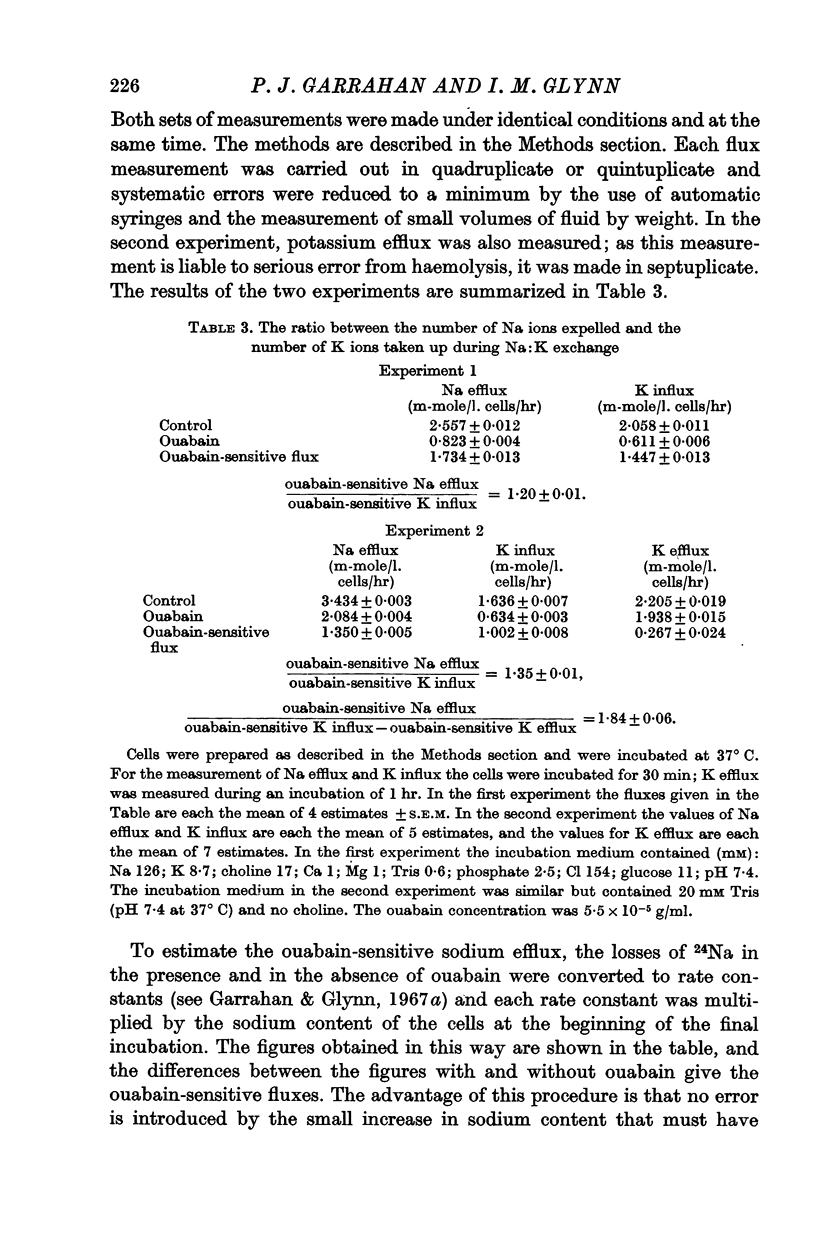
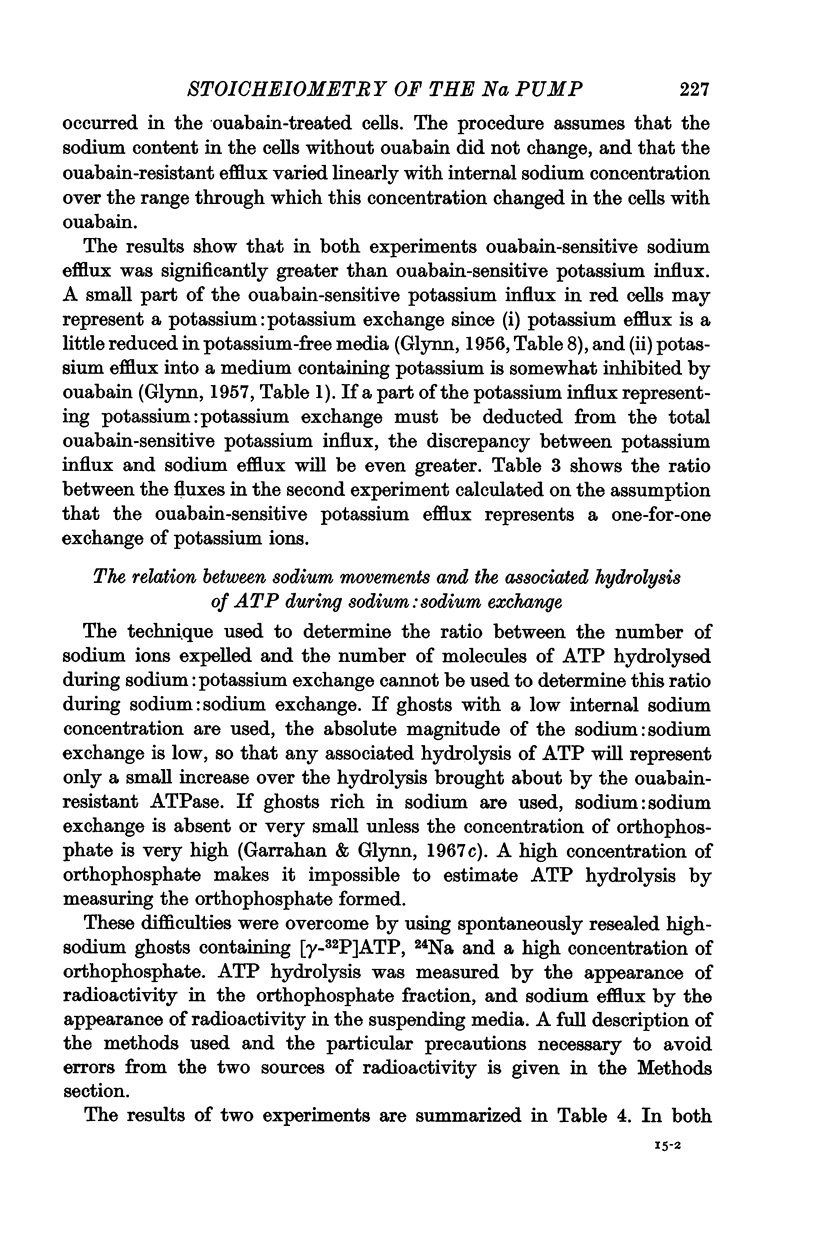
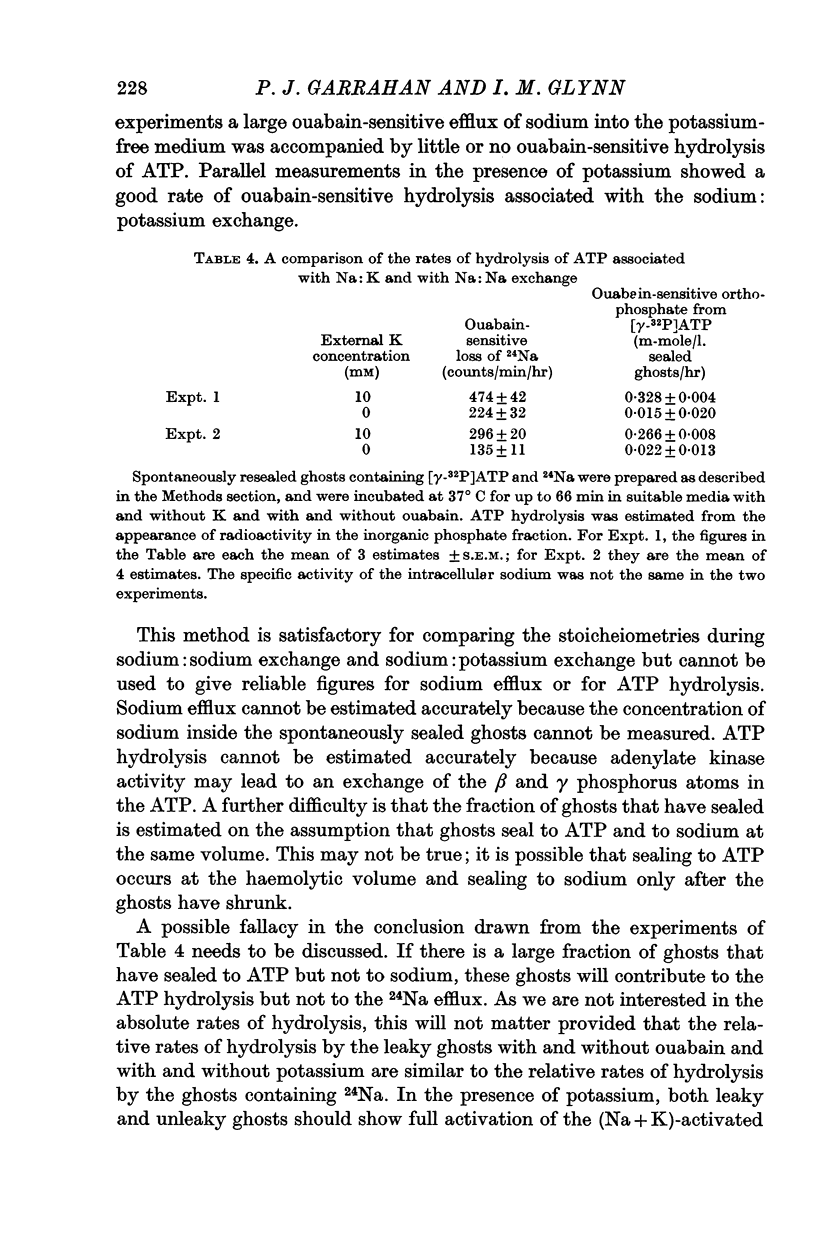
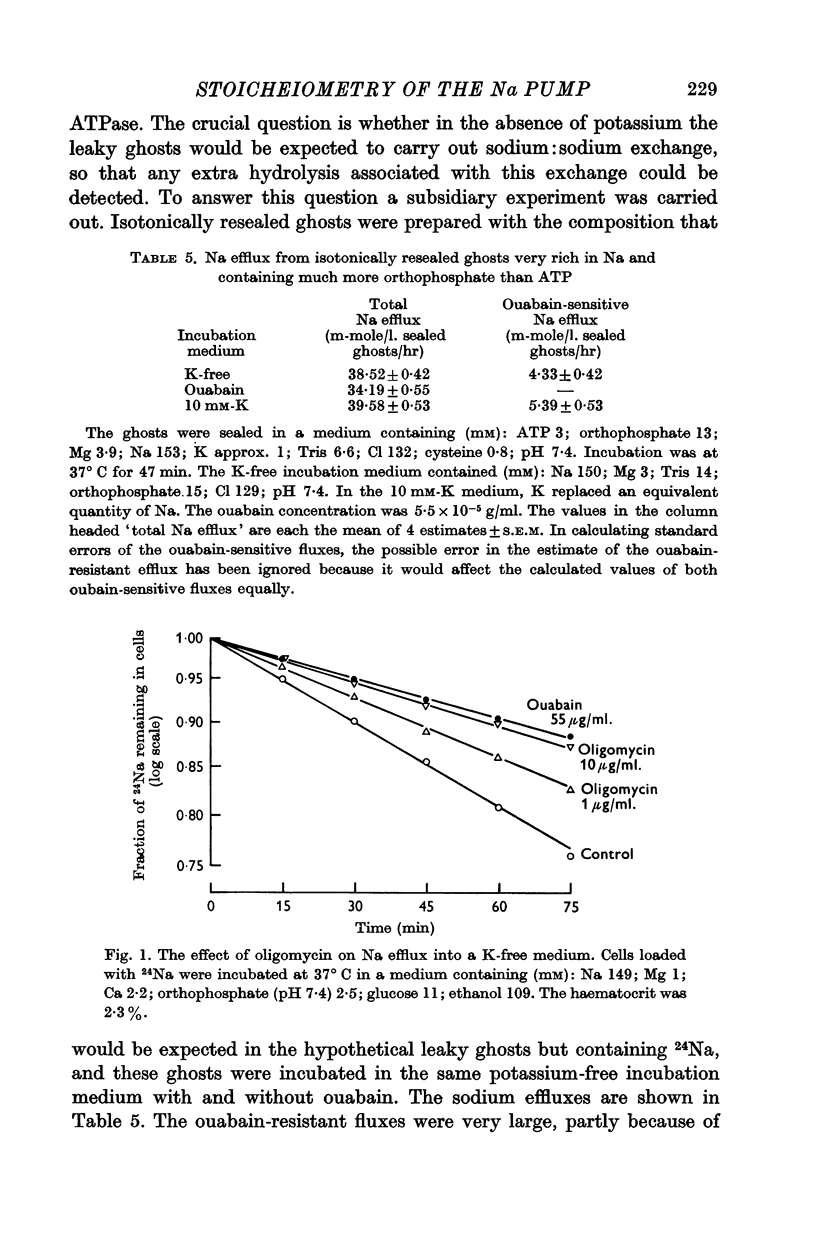
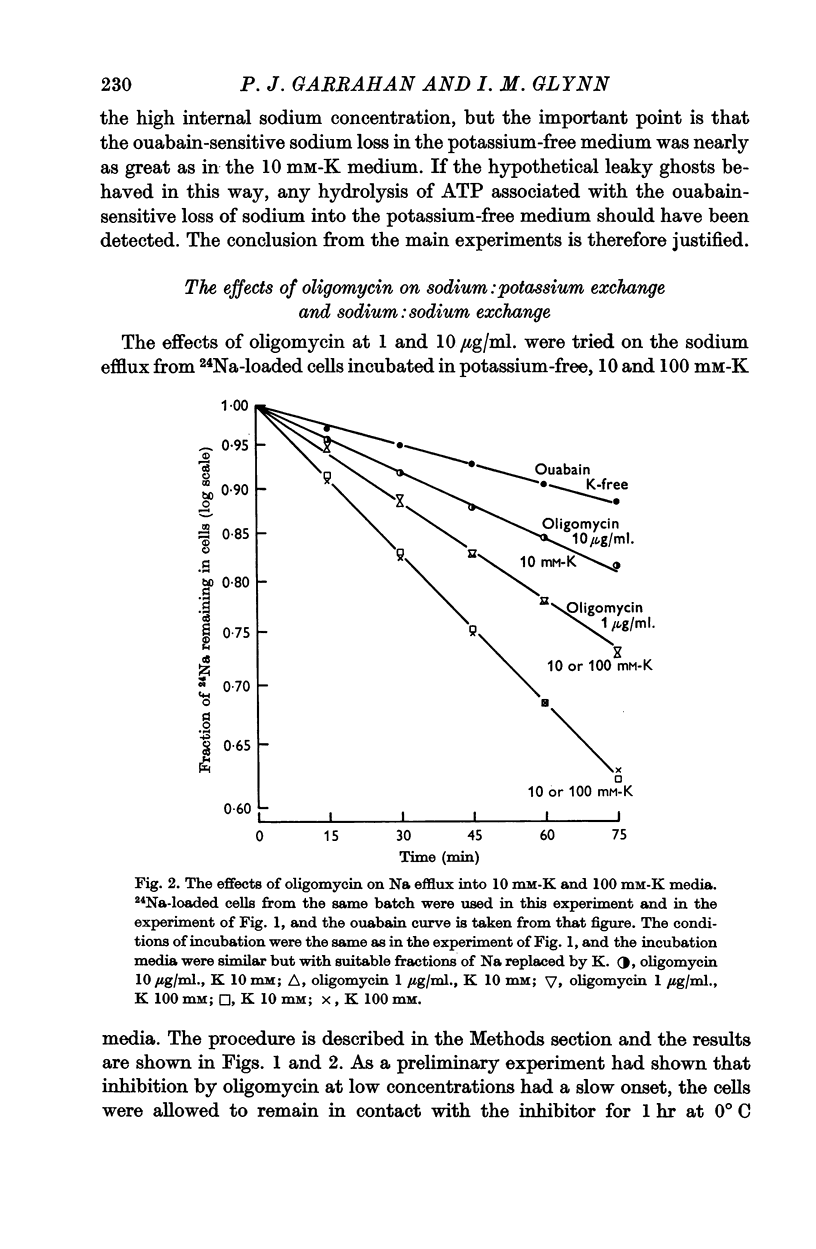
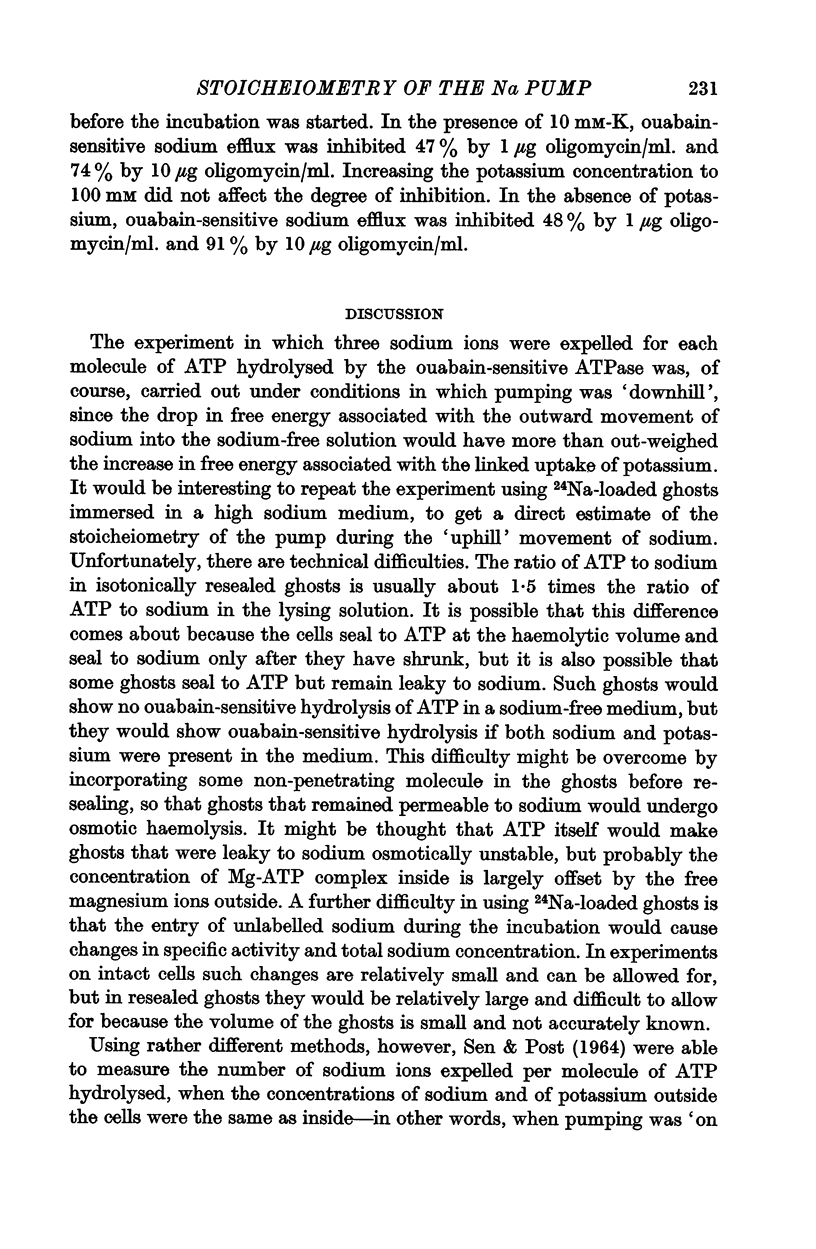
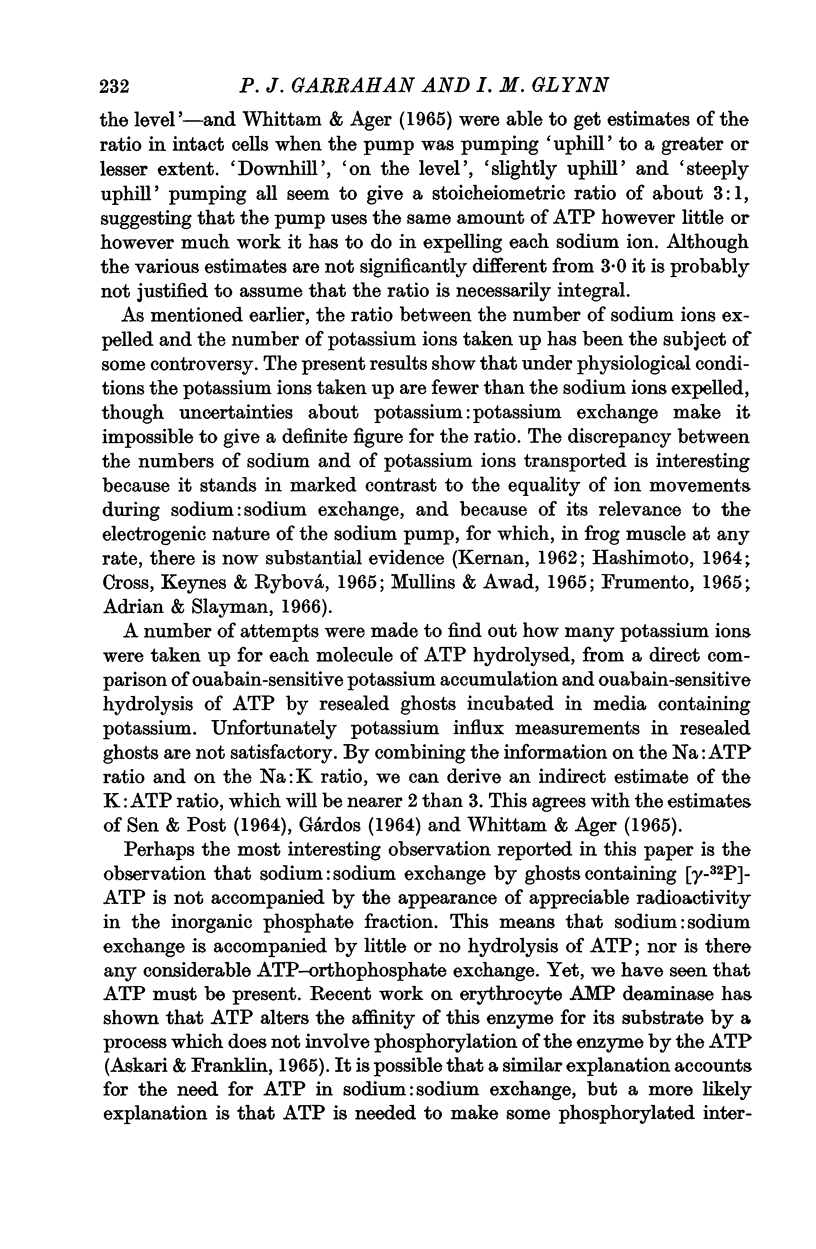
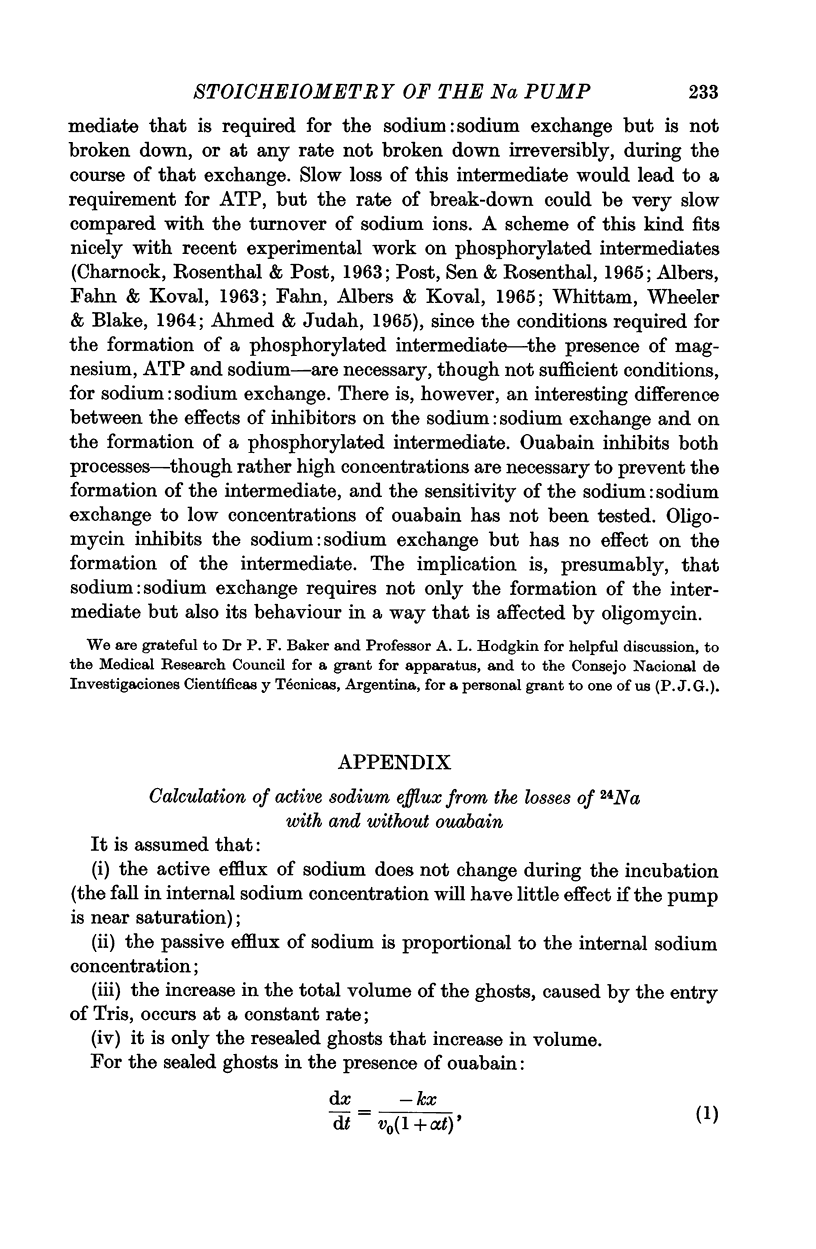
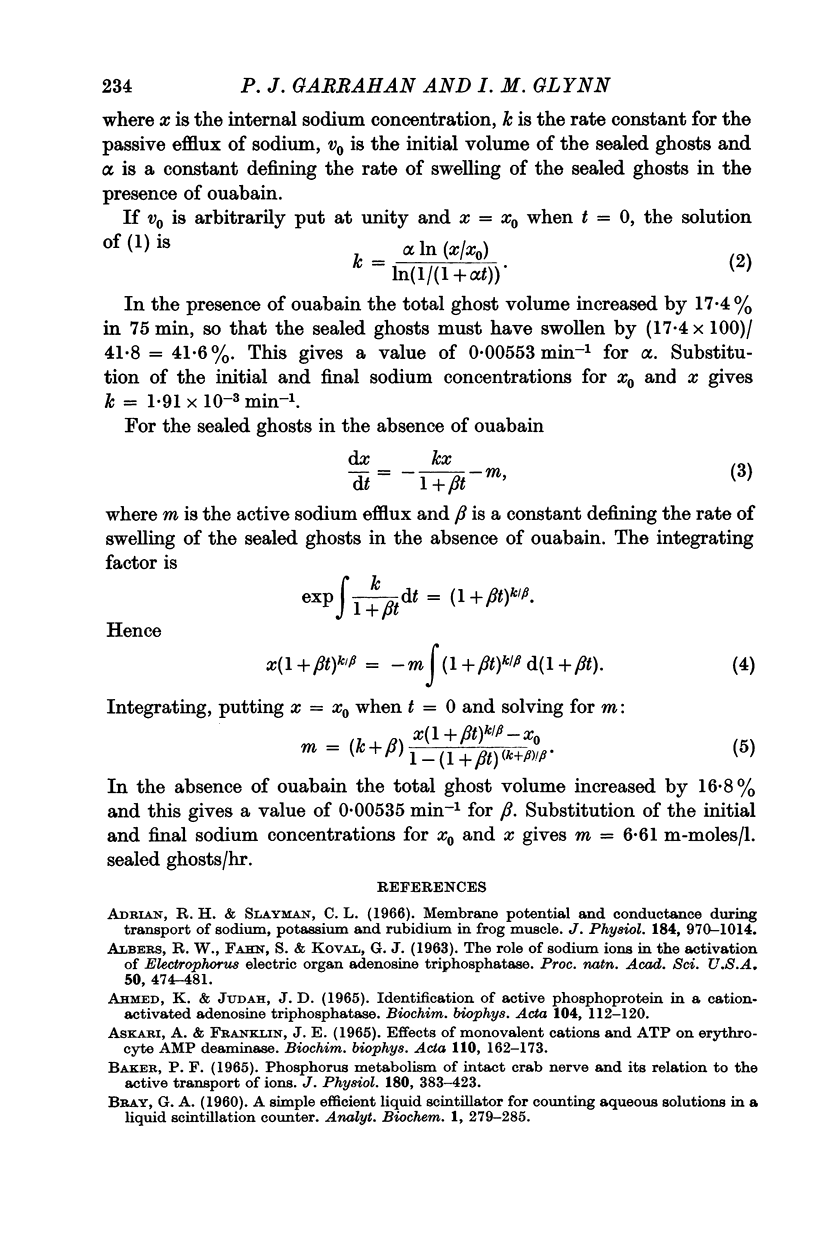
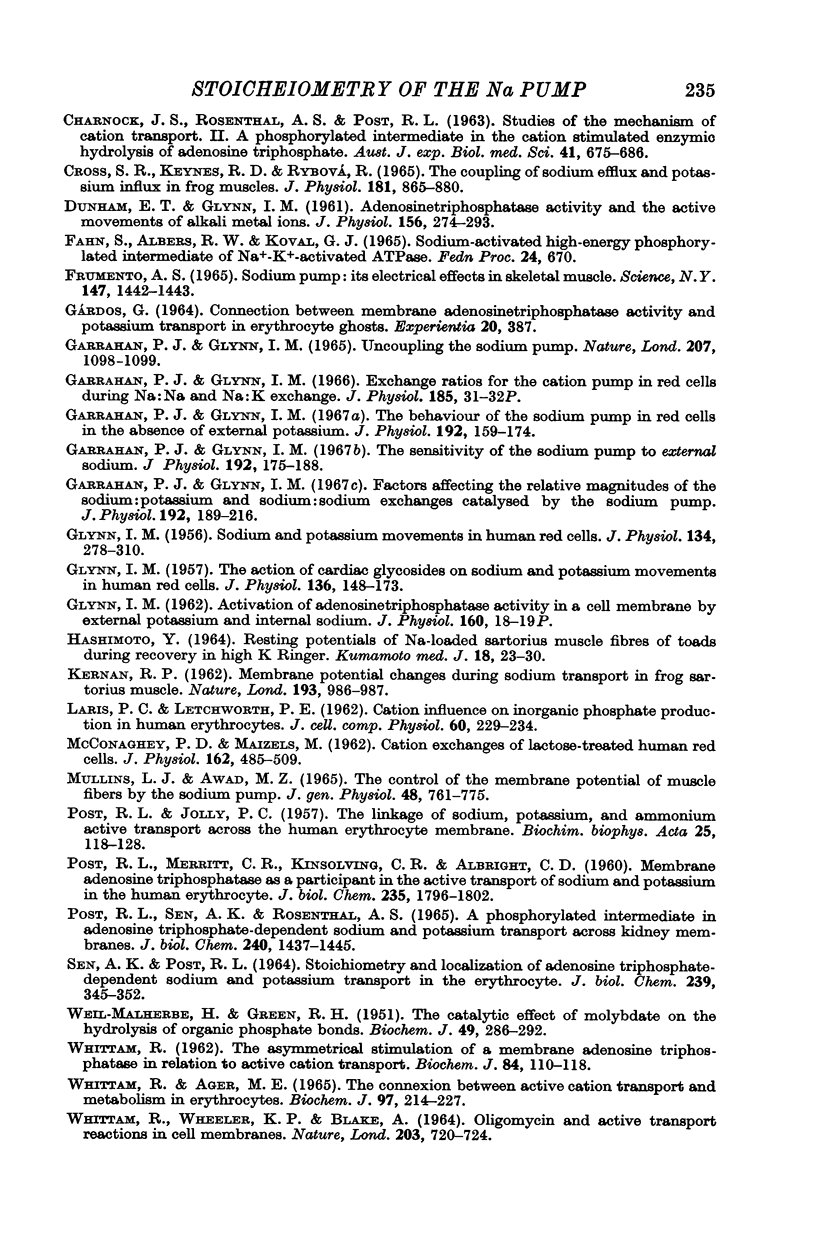
Selected References
These references are in PubMed. This may not be the complete list of references from this article.
- ALBERS R. W., FAHN S., KOVAL G. J. THE ROLE OF SODIUM IONS IN THE ACTIVATION OF ELECTROPHORUS ELECTRIC ORGAN ADENOSINE TRIPHOSPHATASE. Proc Natl Acad Sci U S A. 1963 Sep;50:474–481. doi: 10.1073/pnas.50.3.474. [DOI] [PMC free article] [PubMed] [Google Scholar]
- Adrian R. H., Slayman C. L. Membrane potential and conductance during transport of sodium, potassium and rubidium in frog muscle. J Physiol. 1966 Jun;184(4):970–1014. doi: 10.1113/jphysiol.1966.sp007961. [DOI] [PMC free article] [PubMed] [Google Scholar]
- Ahmed K., Judah J. D. Identification of active phosphoprotein in a cation-activated adenosine triphosphatase. Biochim Biophys Acta. 1965 Jun 15;104(1):112–120. doi: 10.1016/0304-4165(65)90227-8. [DOI] [PubMed] [Google Scholar]
- Baker P. F. Phosphorus metabolism of intact crab nerve and its relation to the active transport of ions. J Physiol. 1965 Sep;180(2):383–423. doi: 10.1113/jphysiol.1965.sp007709. [DOI] [PMC free article] [PubMed] [Google Scholar]
- CHARNOCK J. S., ROSENTHAL A. S., POST R. L. STUDIES OF THE MECHANISM OF CATION TRANSPORT. II. A PHOSPHORLATED INTERMEDIATE IN THE CATION STIMULATED ENZYMIC HYDROLYSIS OF ADENOSINE TRIPHOSPHATE. Aust J Exp Biol Med Sci. 1963 Dec;41:675–686. doi: 10.1038/icb.1963.56. [DOI] [PubMed] [Google Scholar]
- Cross S. B., Keynes R. D., Rybová R. The coupling of sodium efflux and potassium influx in frog muscle. J Physiol. 1965 Dec;181(4):865–880. doi: 10.1113/jphysiol.1965.sp007802. [DOI] [PMC free article] [PubMed] [Google Scholar]
- DUNHAM E. T., GLYNN I. M. Adenosinetriphosphatase activity and the active movements of alkali metal ions. J Physiol. 1961 Apr;156:274–293. doi: 10.1113/jphysiol.1961.sp006675. [DOI] [PMC free article] [PubMed] [Google Scholar]
- GLYNN I. M. Sodium and potassium movements in human red cells. J Physiol. 1956 Nov 28;134(2):278–310. doi: 10.1113/jphysiol.1956.sp005643. [DOI] [PMC free article] [PubMed] [Google Scholar]
- GLYNN I. M. The action of cardiac glycosides on sodium and potassium movements in human red cells. J Physiol. 1957 Apr 3;136(1):148–173. doi: 10.1113/jphysiol.1957.sp005749. [DOI] [PMC free article] [PubMed] [Google Scholar]
- Garrahan P. J., Glynn I. M. Facftors affecting the relative magnitudes of the sodium:potassium and sodium:sodium exchanges catalysed by the sodium pump. J Physiol. 1967 Sep;192(1):189–216. doi: 10.1113/jphysiol.1967.sp008296. [DOI] [PMC free article] [PubMed] [Google Scholar]
- Garrahan P. J., Glynn I. M. The behaviour of the sodium pump in red cells in the absence of external potassium. J Physiol. 1967 Sep;192(1):159–174. doi: 10.1113/jphysiol.1967.sp008294. [DOI] [PMC free article] [PubMed] [Google Scholar]
- Garrahan P. J., Glynn I. M. The sensitivity of the sodium pump to external sodium. J Physiol. 1967 Sep;192(1):175–188. doi: 10.1113/jphysiol.1967.sp008295. [DOI] [PMC free article] [PubMed] [Google Scholar]
- Garrahan P. J., Glynn I. M. Uncoupling the sodium pump. Nature. 1965 Sep 4;207(5001):1098–1099. doi: 10.1038/2071098a0. [DOI] [PubMed] [Google Scholar]
- Gárdos G. Connection between membrane adenosine triphosphatase activity and potassium transport in erythrocyte ghosts. Experientia. 1964 Jul 15;20(7):387–387. doi: 10.1007/BF02147979. [DOI] [PubMed] [Google Scholar]
- Hashimoto Y. Resting potentials of Na-loaded sartorius muscle fibres of toads during recovery in high K ringer. Kumamoto Med J. 1965 Mar 31;18(1):23–30. [PubMed] [Google Scholar]
- KERNAN R. P. Membrane potential changes during sodium transport in frog sartorius muscle. Nature. 1962 Mar 10;193:986–987. doi: 10.1038/193986a0. [DOI] [PubMed] [Google Scholar]
- MULLINS L. J., AWAD M. Z. THE CONTROL OF THE MEMBRANE POTENTIAL OF MUSCLE FIBERS BY THE SODIUM PUMP. J Gen Physiol. 1965 May;48:761–775. doi: 10.1085/jgp.48.5.761. [DOI] [PMC free article] [PubMed] [Google Scholar]
- McConaghey P. D., Maizels M. Cation exchanges of lactose-treated human red cells. J Physiol. 1962 Aug;162(3):485–509. doi: 10.1113/jphysiol.1962.sp006946. [DOI] [PMC free article] [PubMed] [Google Scholar]
- POST R. L., JOLLY P. C. The linkage of sodium, potassium, and ammonium active transport across the human erythrocyte membrane. Biochim Biophys Acta. 1957 Jul;25(1):118–128. doi: 10.1016/0006-3002(57)90426-2. [DOI] [PubMed] [Google Scholar]
- POST R. L., MERRITT C. R., KINSOLVING C. R., ALBRIGHT C. D. Membrane adenosine triphosphatase as a participant in the active transport of sodium and potassium in the human erythrocyte. J Biol Chem. 1960 Jun;235:1796–1802. [PubMed] [Google Scholar]
- POST R. L., SEN A. K., ROSENTHAL A. S. A PHOSPHORYLATED INTERMEDIATE IN ADENOSINE TRIPHOSPHATE-DEPENDENT SODIUM AND POTASSIUM TRANSPORT ACROSS KIDNEY MEMBRANES. J Biol Chem. 1965 Mar;240:1437–1445. [PubMed] [Google Scholar]
- SEN A. K., POST R. L. STOICHIOMETRY AND LOCALIZATION OF ADENOSINE TRIPHOSPHATE-DEPENDENT SODIUM AND POTASSIUM TRANSPORT IN THE ERYTHROCYTE. J Biol Chem. 1964 Jan;239:345–352. [PubMed] [Google Scholar]
- WEIL-MALHERBE H., GREEN R. H. The catalytic effect of molybdate on the hydrolysis of organic phosphate bonds. Biochem J. 1951 Aug;49(3):286–292. [PMC free article] [PubMed] [Google Scholar]
- WHITTAM R. The asymmetrical stimulation of a membrane adenosine triphosphatase in relation to active cation transport. Biochem J. 1962 Jul;84:110–118. doi: 10.1042/bj0840110. [DOI] [PMC free article] [PubMed] [Google Scholar]
- WHITTAM R., WHEELER K. P., BLAKE A. OLIGOMYCIN AND ACTIVE TRANSPORT REACTIONS IN CELL MEMBRANES. Nature. 1964 Aug 15;203:720–724. doi: 10.1038/203720a0. [DOI] [PubMed] [Google Scholar]
- Whittam R., Ager M. E. The connexion between active cation transport and metabolism in erythrocytes. Biochem J. 1965 Oct;97(1):214–227. doi: 10.1042/bj0970214. [DOI] [PMC free article] [PubMed] [Google Scholar]