Abstract
1. A voltage clamp for single myelinated nerve fibres was developed from commercially available operational amplifiers.
2. Under voltage clamp conditions steady-state currents were measured. The currents reached a maximum value at about E = + 100 mV. Currents for greater potentials decreased.
3. The preceding polarization markedly affected the magnitude of the delayed current at high cathodal potentials; however, the currents continued to reach maximum values.
4. As predicted by the constant field theory, currents at high cathodal polarizations were essentially independent of the external potassium concentration. Also, these currents were independent of the external calcium concentration.
5. Two pulse voltage clamp experiments showed that instantaneous outward currents did not reach a limiting value. The limiting steady-state current was reached with an exponential time constant of about 1 msec.
Full text
PDF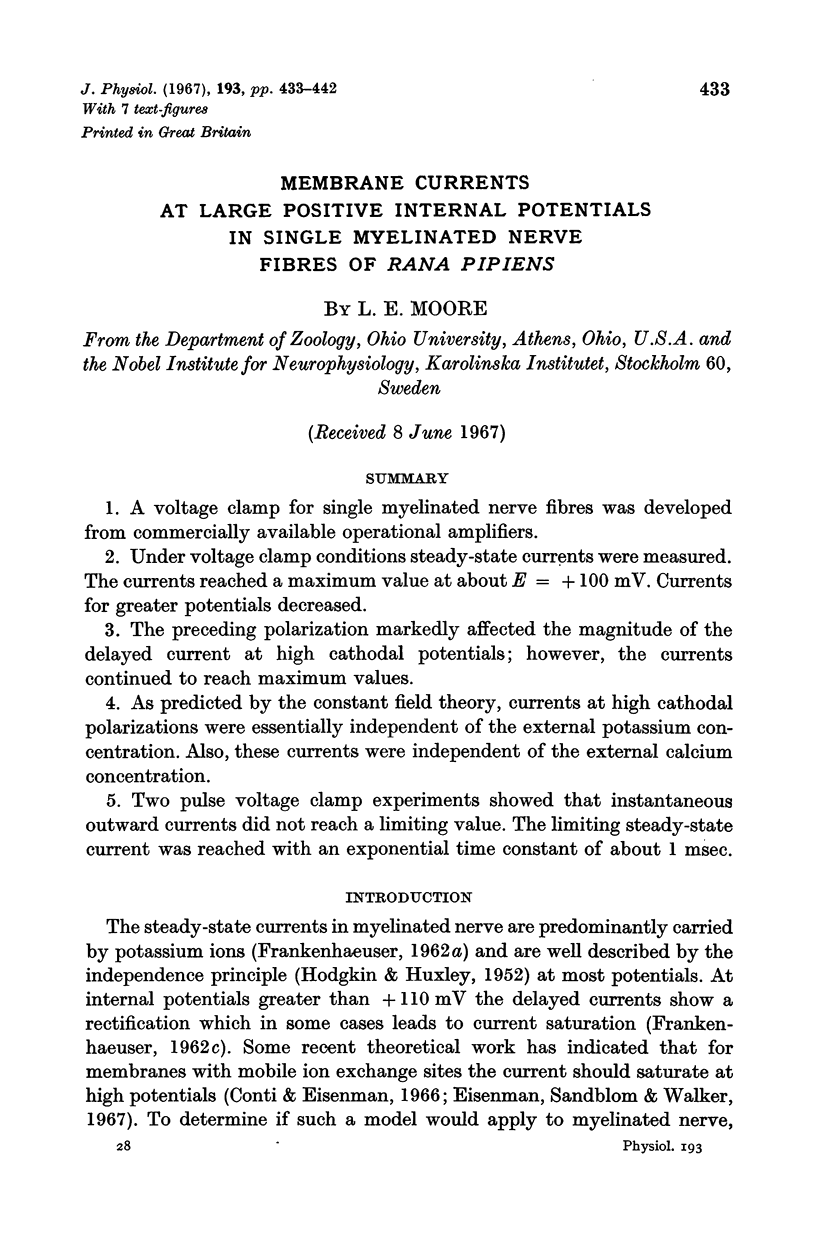
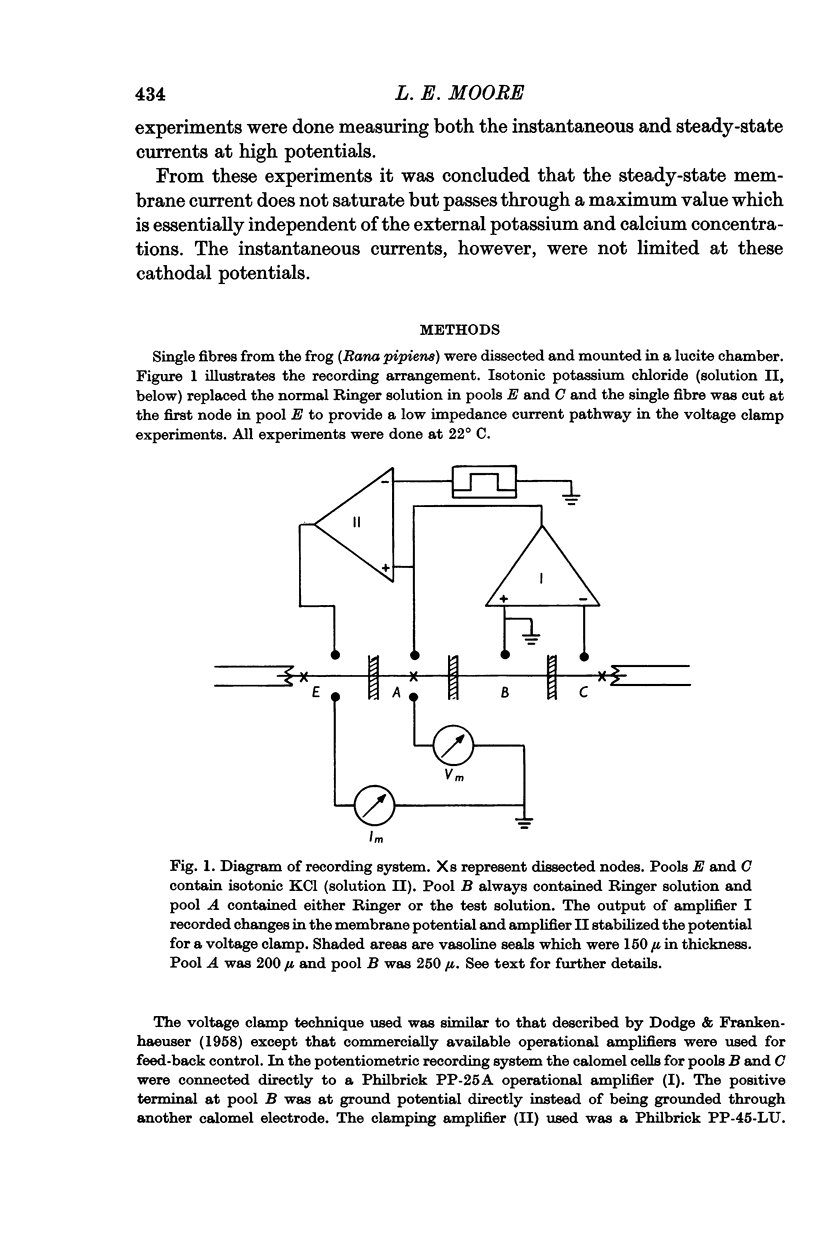
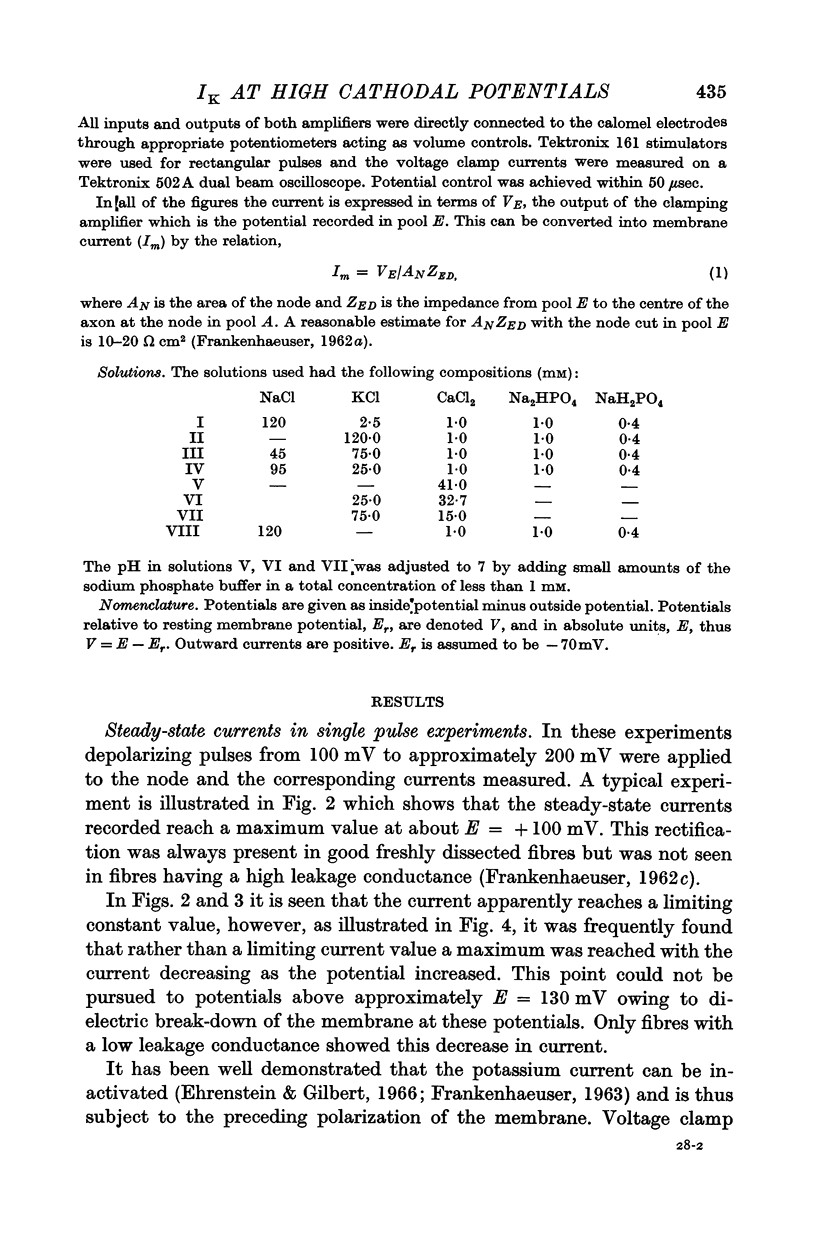
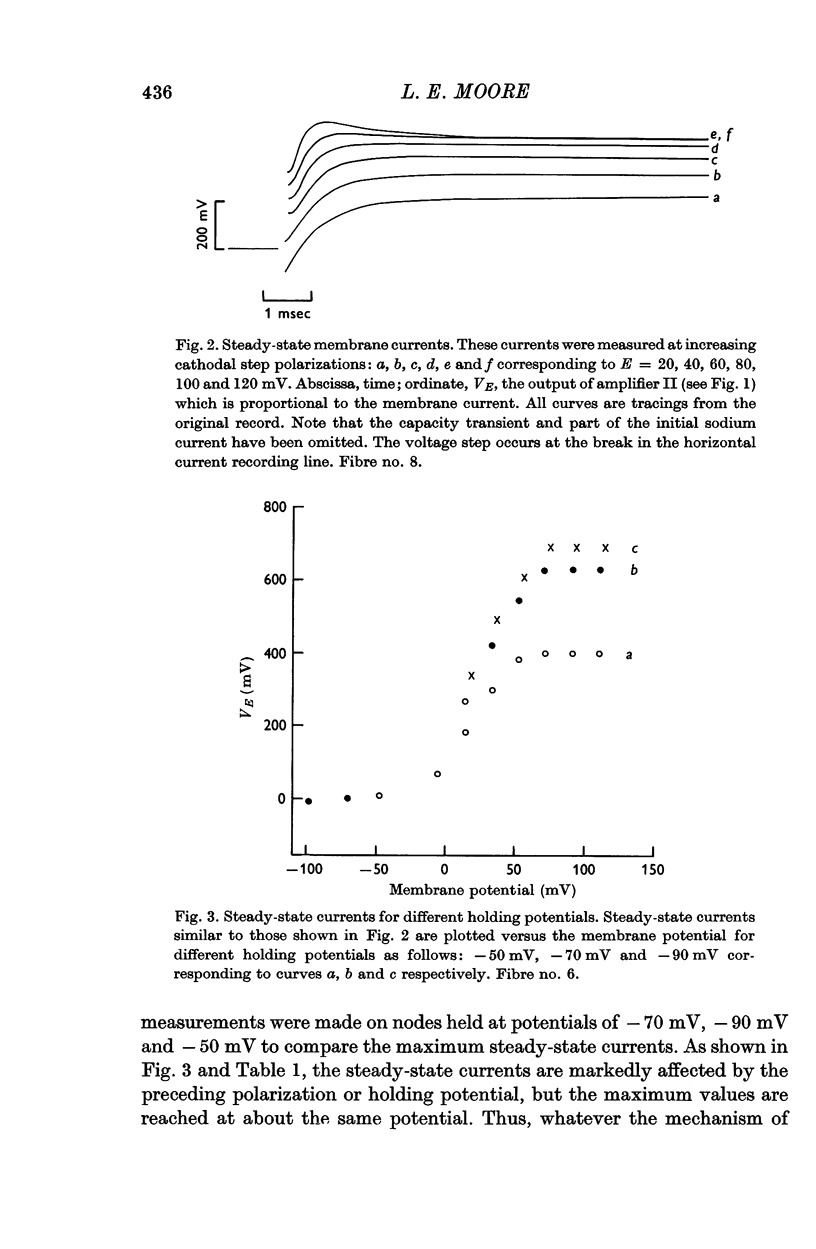
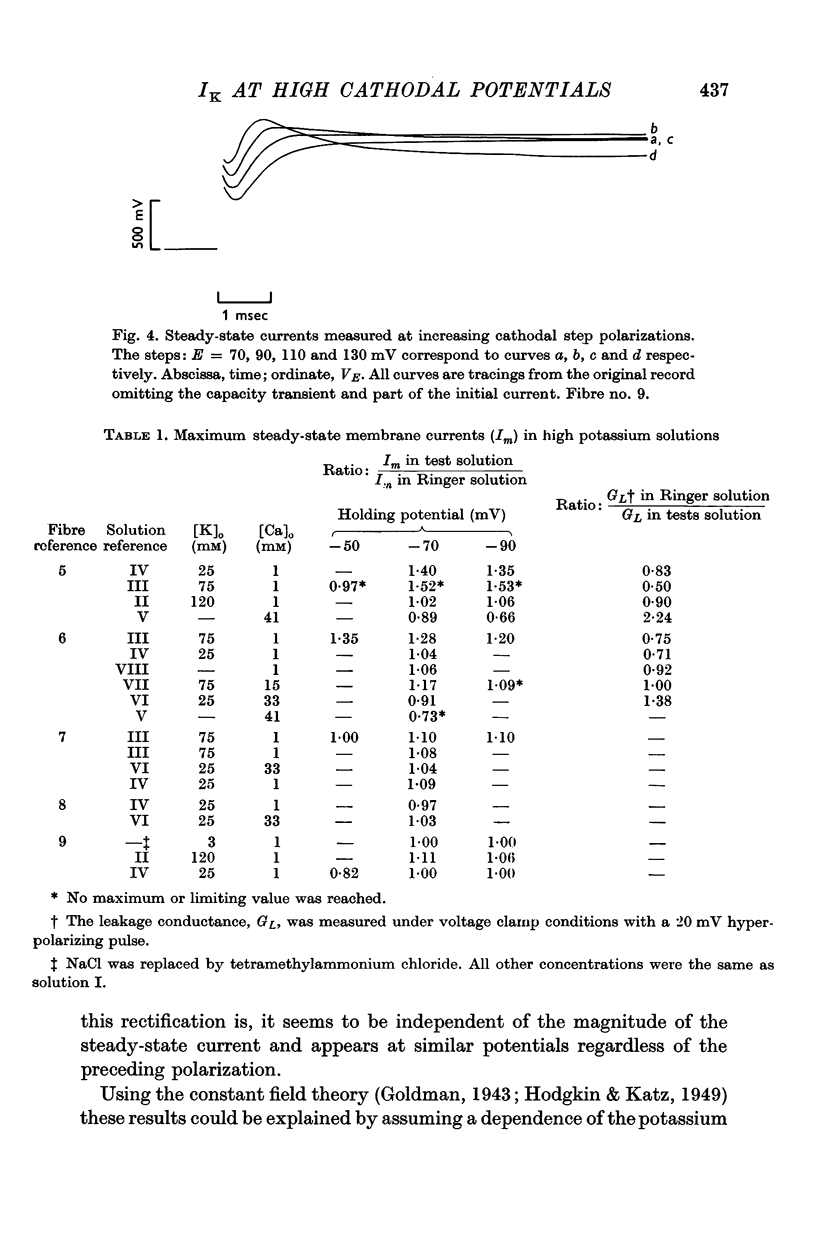
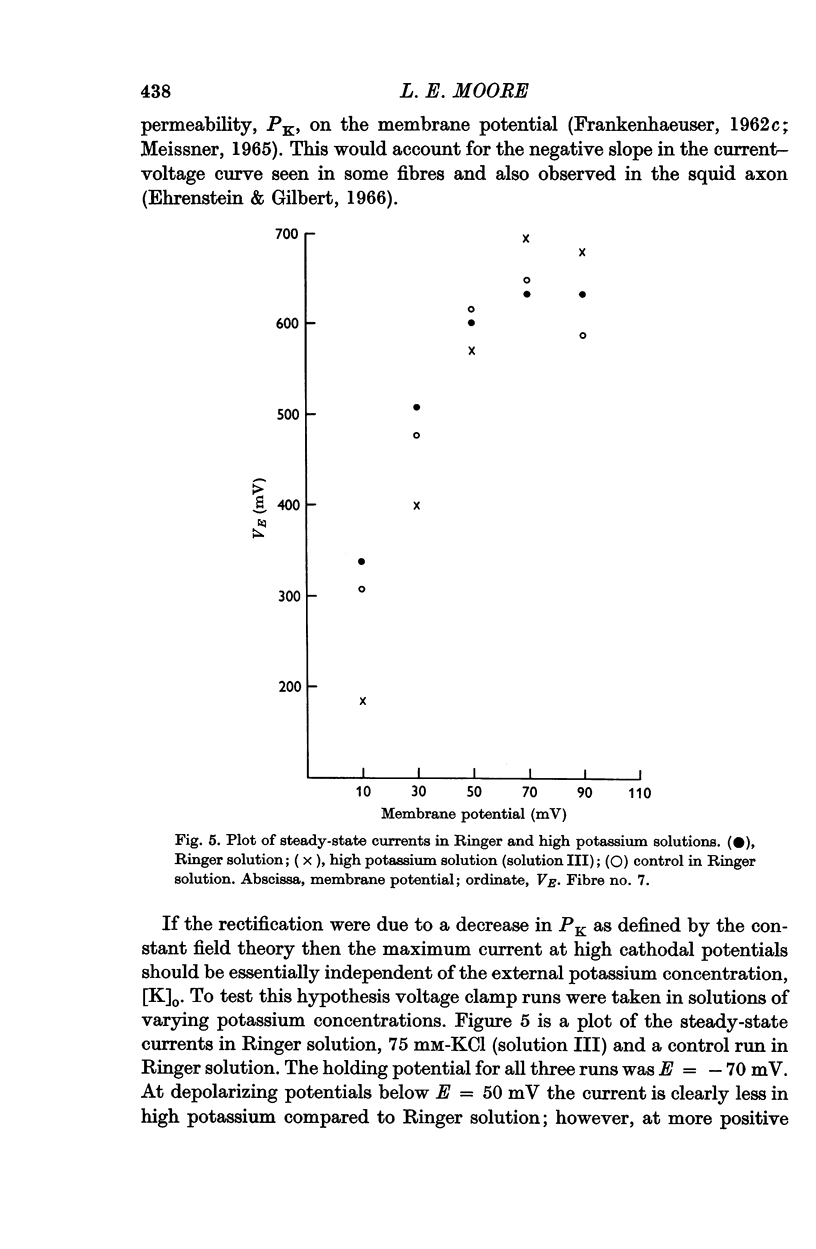
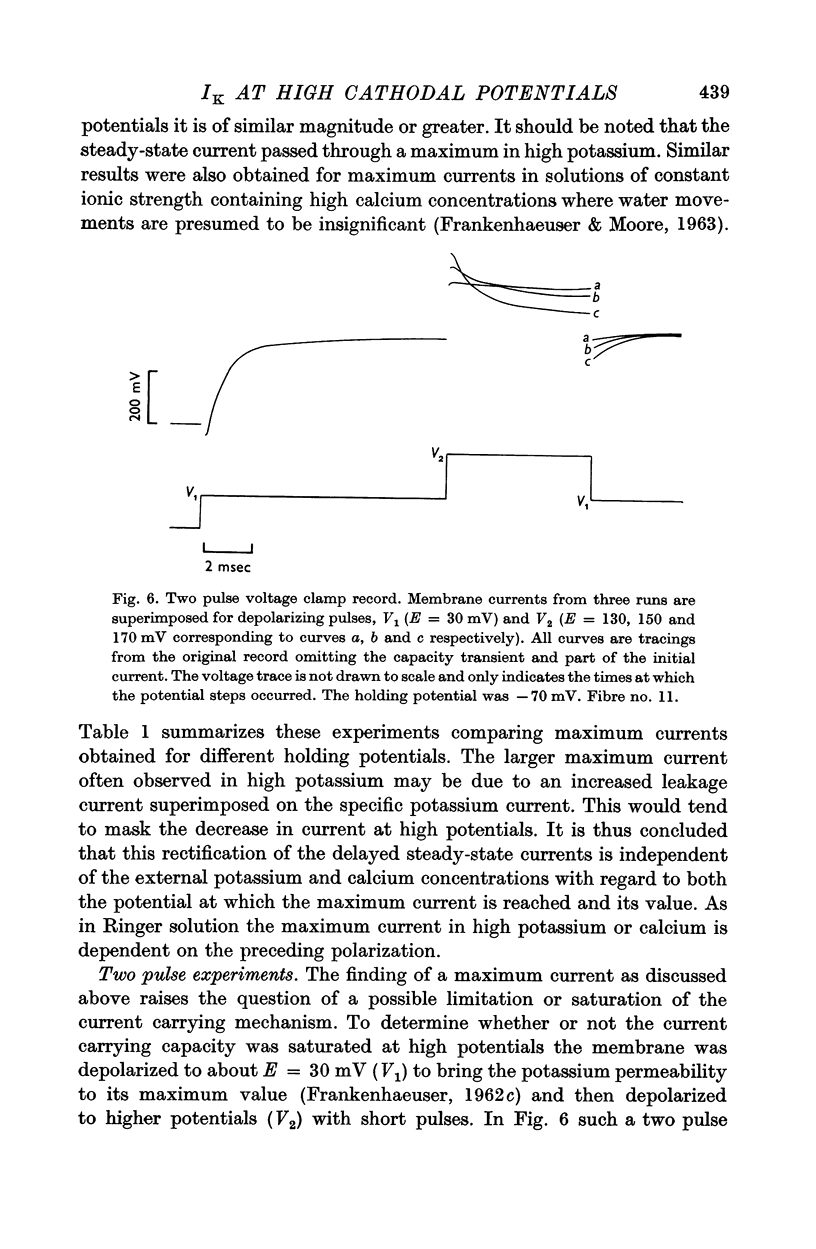
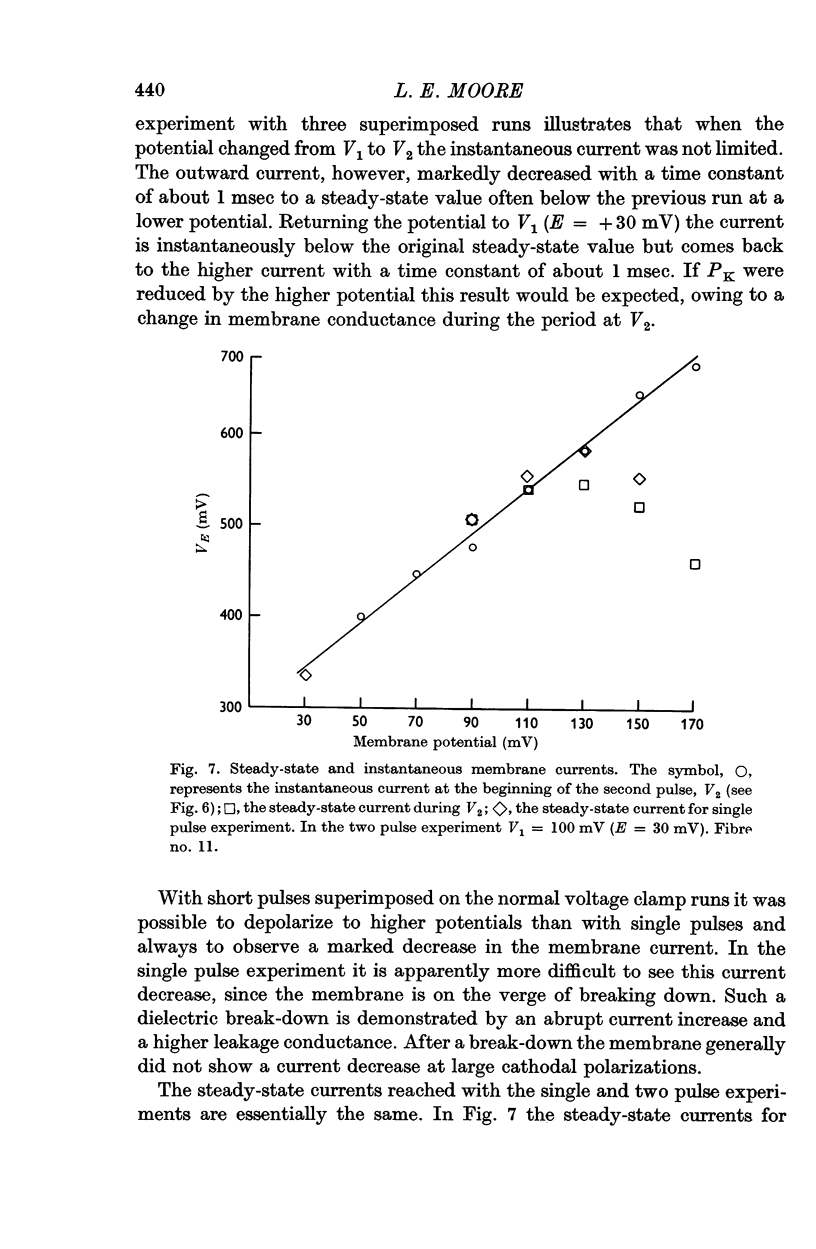
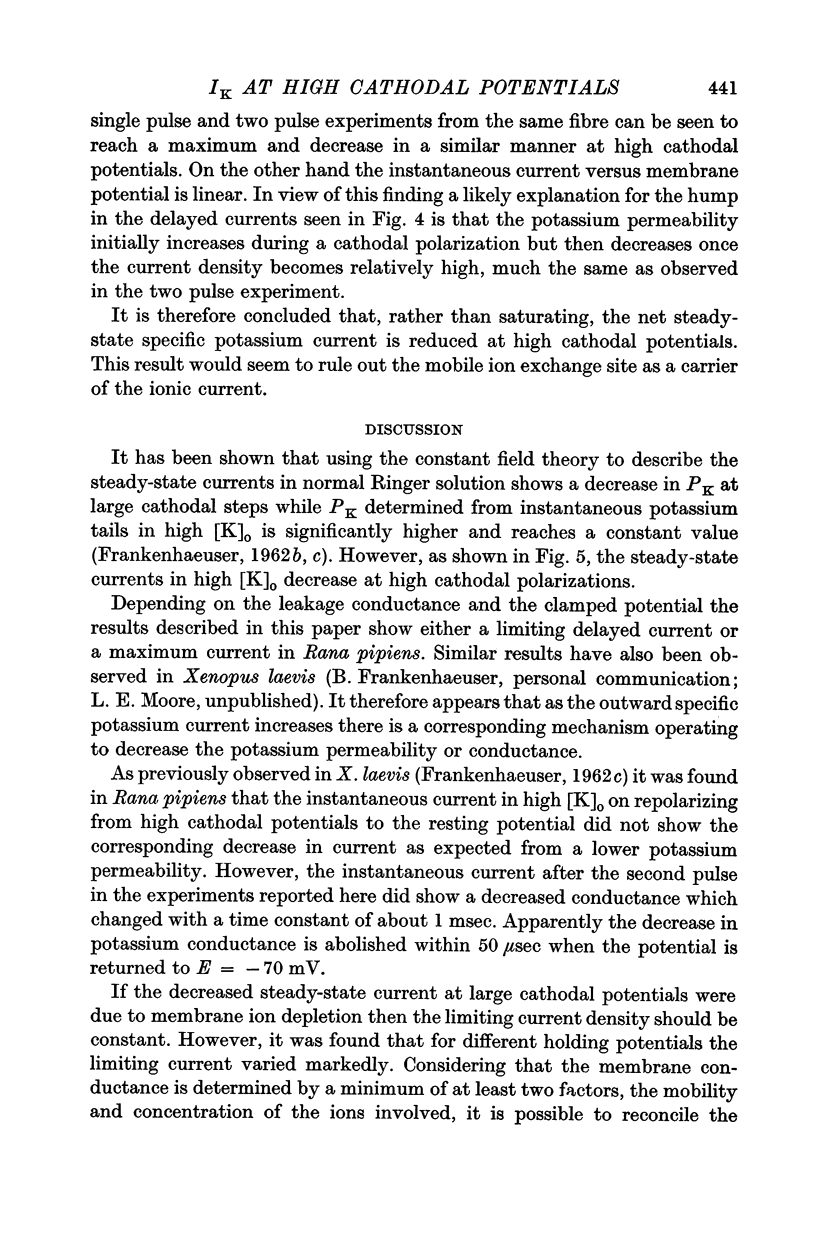
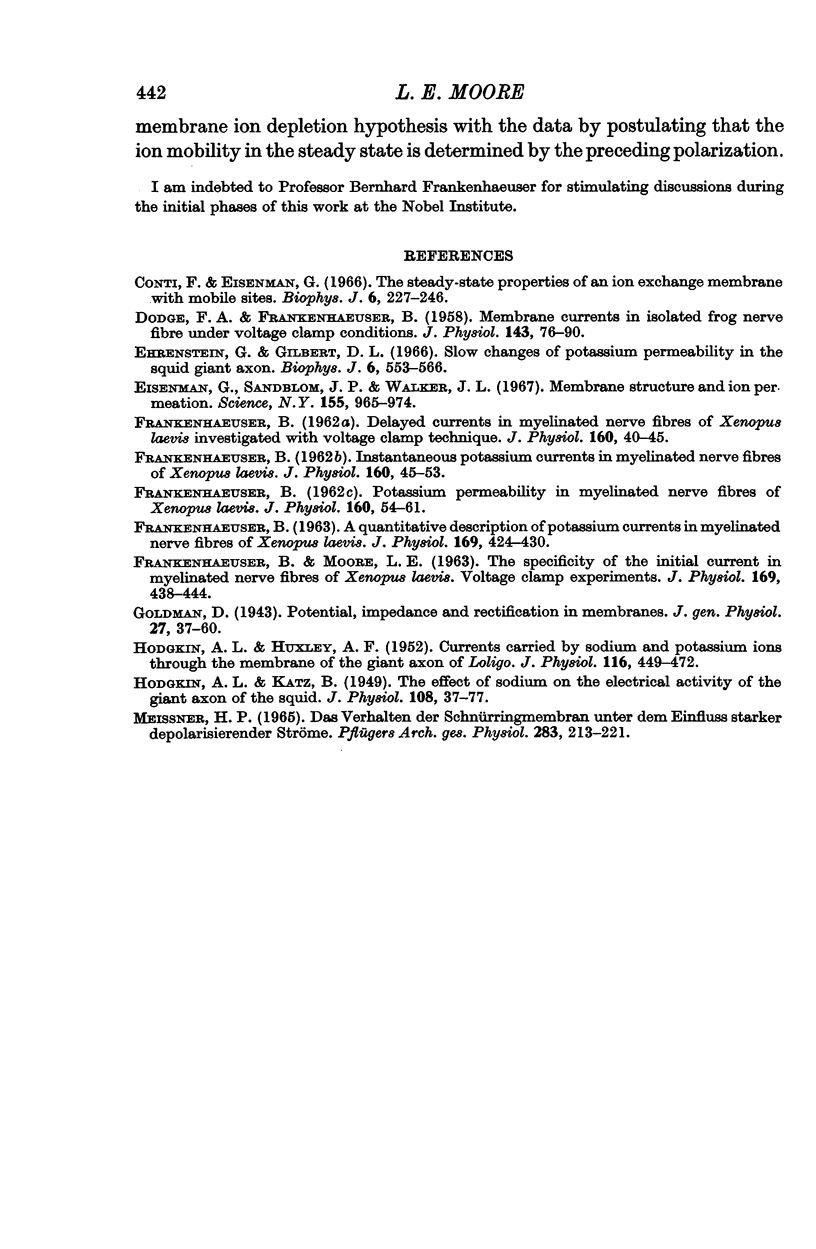
Selected References
These references are in PubMed. This may not be the complete list of references from this article.
- Conti F., Eisenman G. The steady-state properties of an ion exchange membrane with mobile sites. Biophys J. 1966 May;6(3):227–246. doi: 10.1016/S0006-3495(66)86653-5. [DOI] [PMC free article] [PubMed] [Google Scholar]
- DODGE F. A., FRANKENHAEUSER B. Membrane currents in isolated frog nerve fibre under voltage clamp conditions. J Physiol. 1958 Aug 29;143(1):76–90. doi: 10.1113/jphysiol.1958.sp006045. [DOI] [PMC free article] [PubMed] [Google Scholar]
- Ehrenstein G., Gilbert D. L. Slow changes of potassium permeability in the squid giant axon. Biophys J. 1966 Sep;6(5):553–566. doi: 10.1016/S0006-3495(66)86677-8. [DOI] [PMC free article] [PubMed] [Google Scholar]
- Eisenman G., Sandblom J. P., Walker J. L., Jr Membrane structure and ion permeation. Study of ion exchange membrane structure and function is relevant to analysis of biological ion permeation. Science. 1967 Feb 24;155(3765):965–974. doi: 10.1126/science.155.3765.965. [DOI] [PubMed] [Google Scholar]
- FRANKENHAEUSER B. A QUANTITATIVE DESCRIPTION OF POTASSIUM CURRENTS IN MYELINATED NERVE FIBRES OF XENOPUS LAEVIS. J Physiol. 1963 Nov;169:424–430. doi: 10.1113/jphysiol.1963.sp007268. [DOI] [PMC free article] [PubMed] [Google Scholar]
- FRANKENHAEUSER B., MOORE L. E. THE SPECIFICITY OF THE INITIAL CURRENT IN MYELINATED NERVE FIBRES OF XENOPUS LAEVIS. VOLTAGE CLAMP EXPERIMENTS. J Physiol. 1963 Nov;169:438–444. doi: 10.1113/jphysiol.1963.sp007270. [DOI] [PMC free article] [PubMed] [Google Scholar]
- FRANKENHAEUSER B. Potassium permeability in myelinated nerve fibres of Xenopus laevis. J Physiol. 1962 Jan;160:54–61. doi: 10.1113/jphysiol.1962.sp006834. [DOI] [PMC free article] [PubMed] [Google Scholar]
- HODGKIN A. L., HUXLEY A. F. Currents carried by sodium and potassium ions through the membrane of the giant axon of Loligo. J Physiol. 1952 Apr;116(4):449–472. doi: 10.1113/jphysiol.1952.sp004717. [DOI] [PMC free article] [PubMed] [Google Scholar]
- HODGKIN A. L., KATZ B. The effect of sodium ions on the electrical activity of giant axon of the squid. J Physiol. 1949 Mar 1;108(1):37–77. doi: 10.1113/jphysiol.1949.sp004310. [DOI] [PMC free article] [PubMed] [Google Scholar]