Abstract
Discussions of active transport usually assume stoichiometry between the rate of transport J+ and the metabolic rate Jr. However, the observation of a linear relationship between J+ and Jr does not imply a stoichiometric relationship, i.e., complete coupling. Since coupling may possibly be incomplete, we examine systems of an arbitrary degree of coupling q, regarding stoichiometry as a limiting case. We consider a sodium pump, with J+ and Jr linear functions of the electrochemical potential difference, -X+, and the chemical affinity of the metabolic driving reaction, A. The affinity is well defined even for various complex reaction pathways. Incorporation of a series barrier and a parallel leak does not affect the linearity of the composite observable system. The affinity of some region of the metabolic chain may be maintained constant, either by large pools of reactants or by regulation. If so, this affinity can be evaluated by two independent methods. Sodium transport is conveniently characterized by the open-circuit potential (Δψ)I=0 and the natural limits, level flow (J+)X+=0, and static head X0+ = (X+)J+=0. With high degrees of coupling -X0+/F approaches the electromotive force ENa (Ussing); -X0+/F cannot be identified with ((RT/F) ln f)X+=0, where f is the flux ratio. The efficiency η = -J+X+/JrA is of significance only when appreciable energy is being converted from one form to another. When either J+ or -X+ is small η is low; the significant parameters are then the efficacies εJ+ = J+/JrA and εX+ = -X+/JrA, respectively maximal at level flow and static head. Leak increases both J+ and εJ+ for isotonic saline reabsorption, but diminishes -X0+ and εX♀. Electrical resistance reflects both passive parameters and metabolism. Various fundamental relations are preserved despite coupling of passive ion and water flows.
Full text
PDF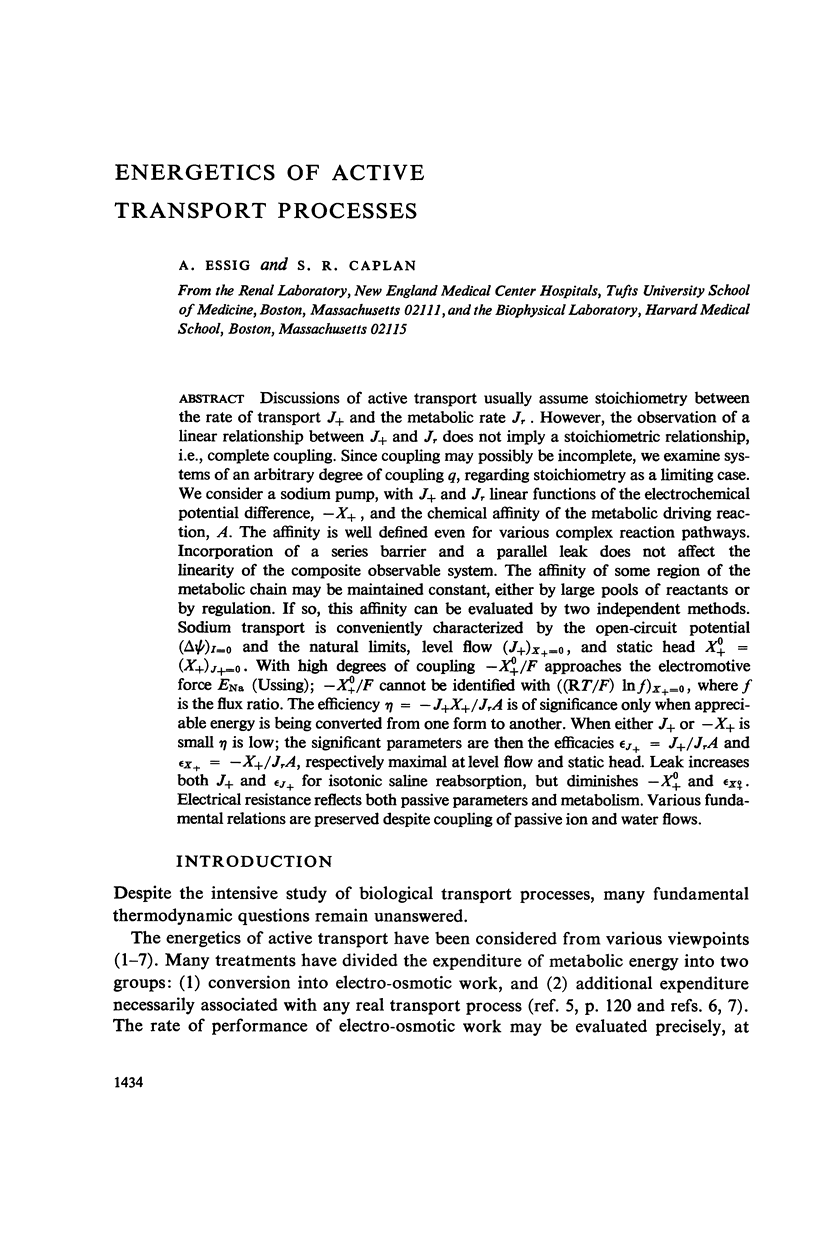
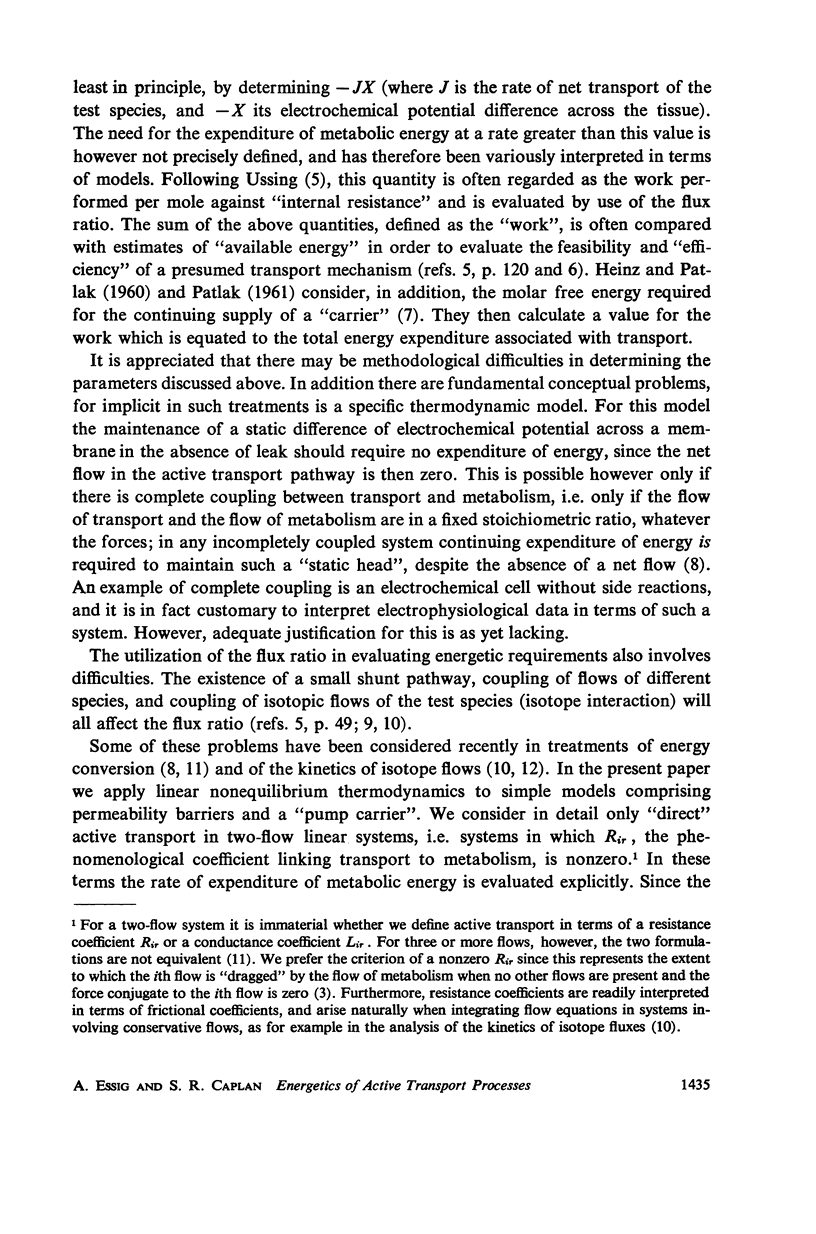
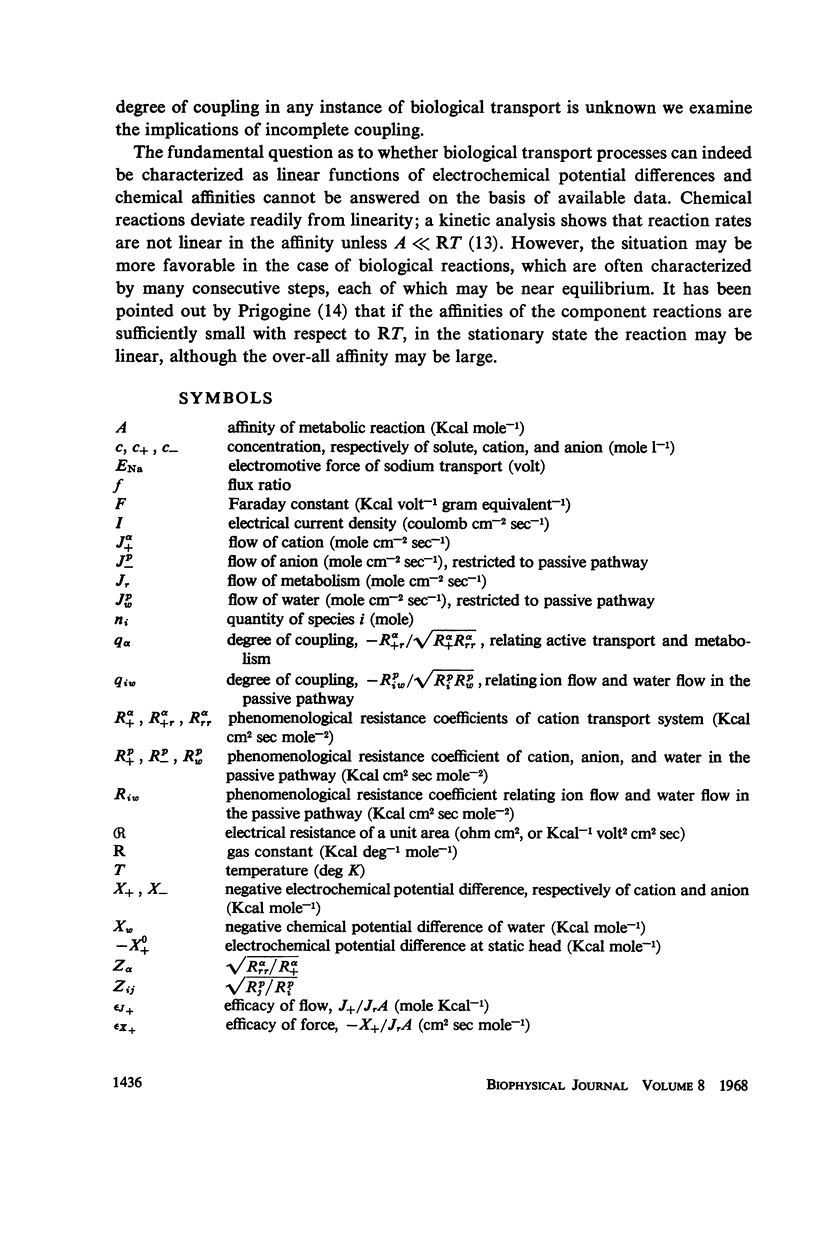
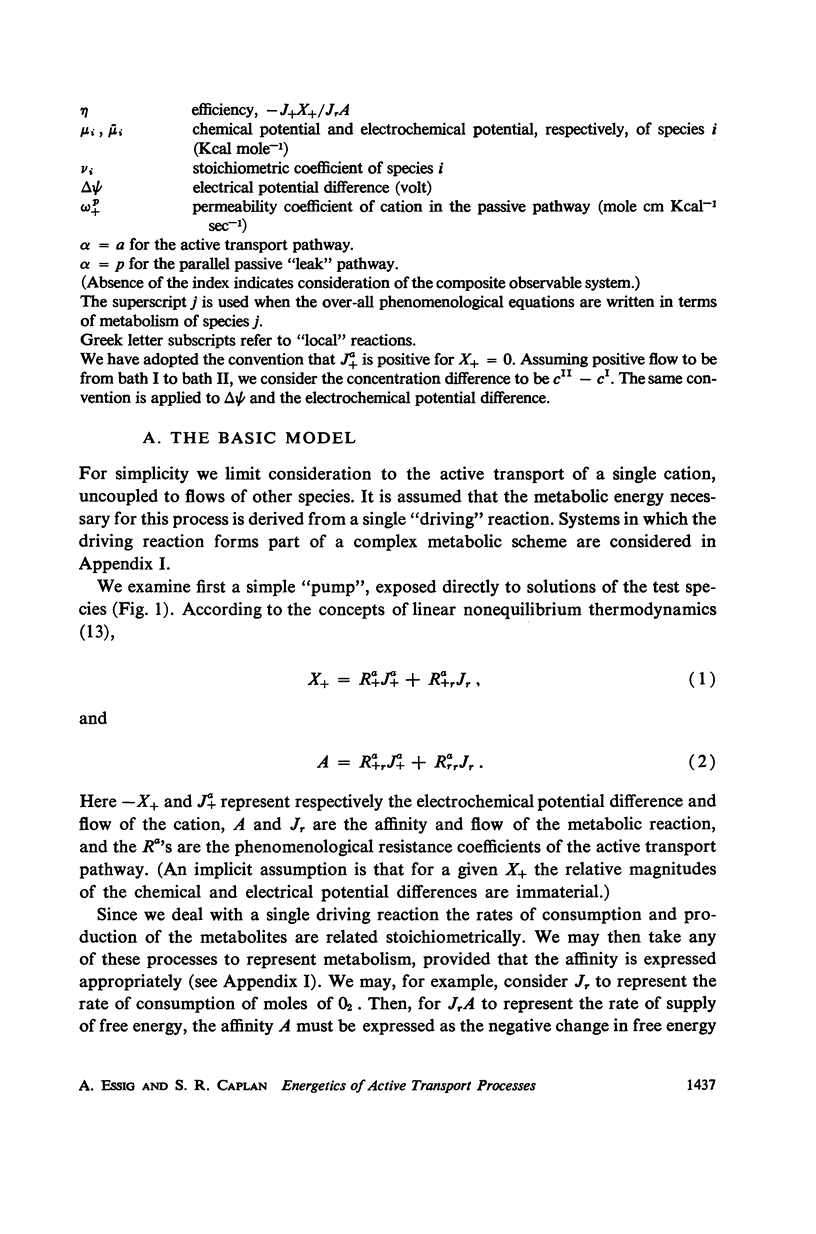
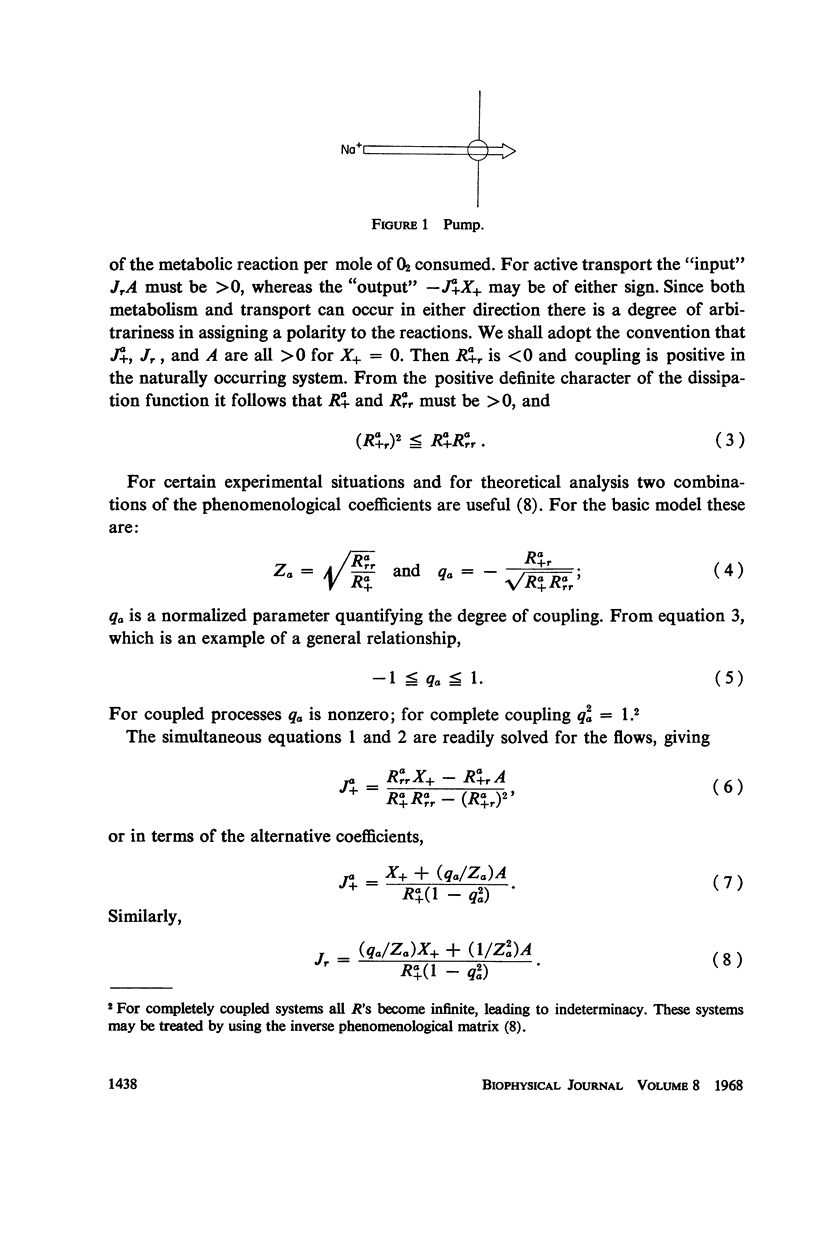
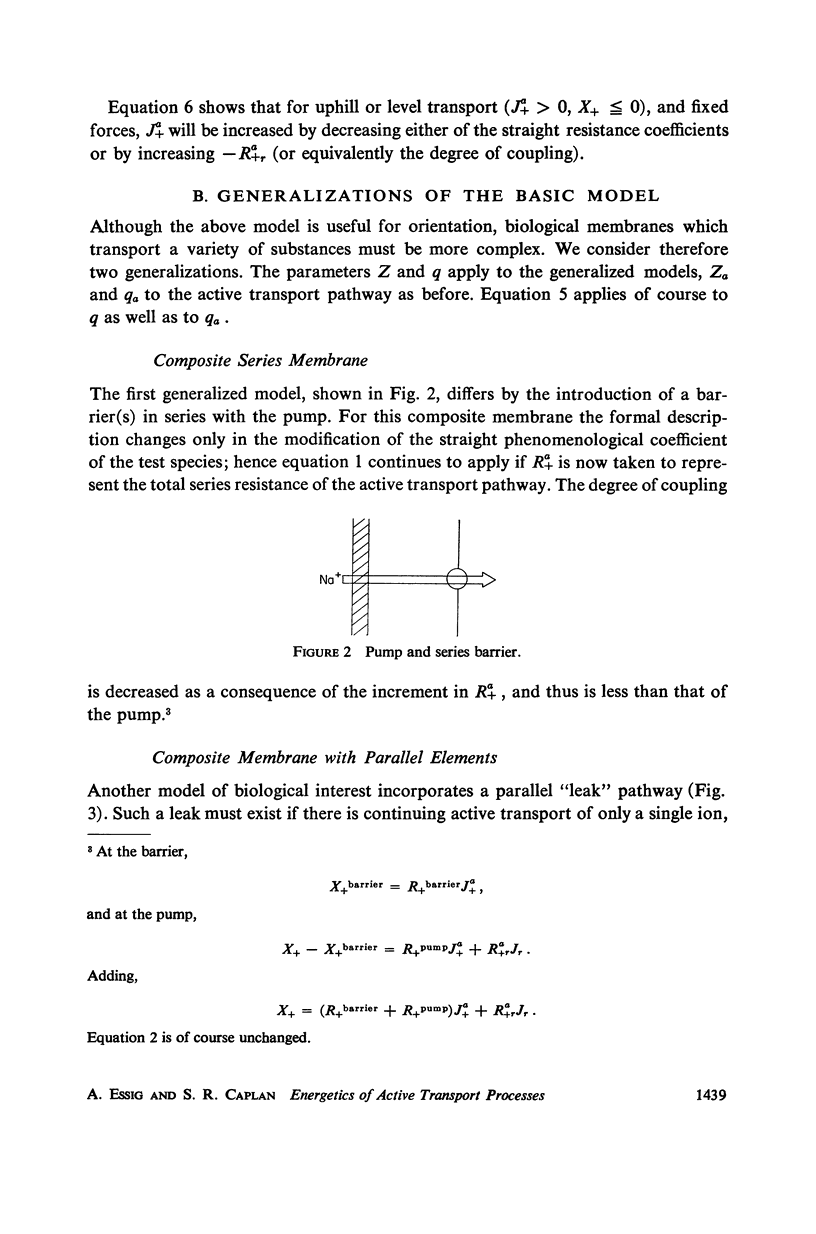
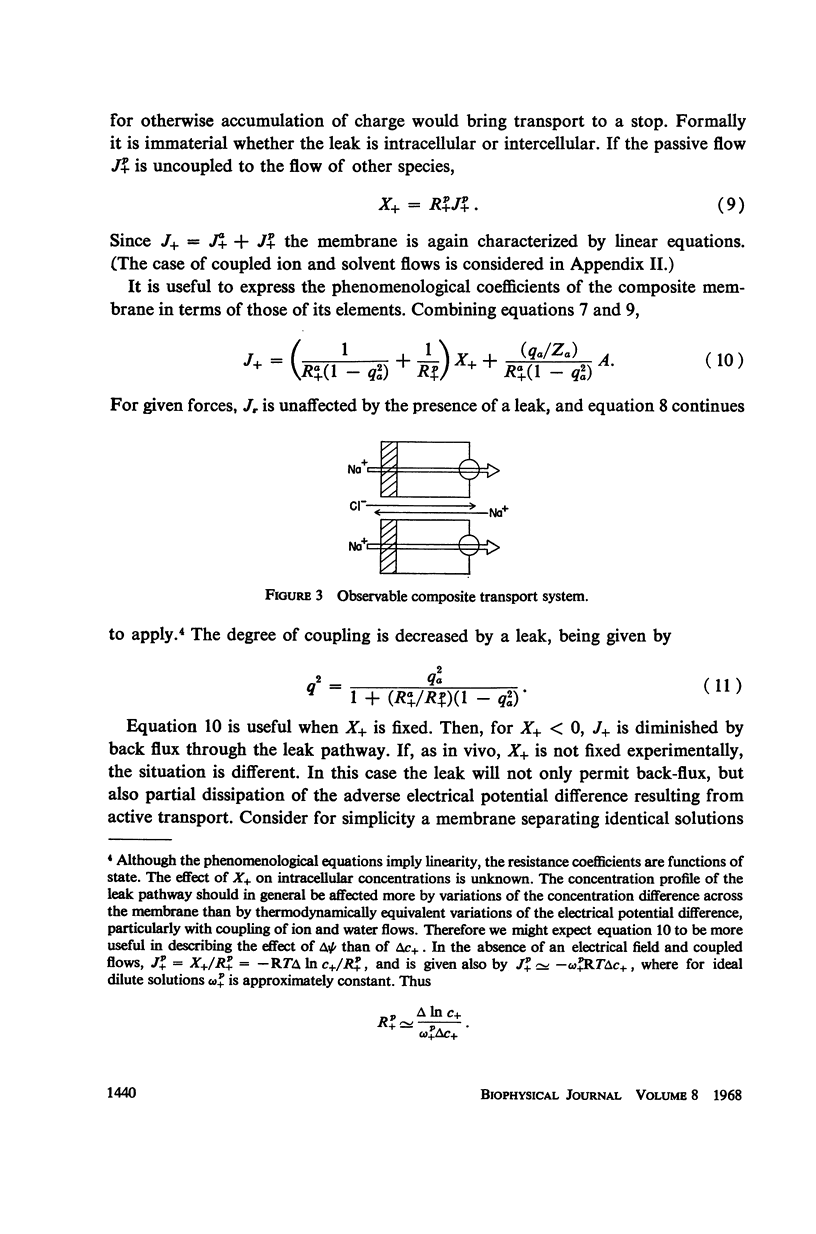
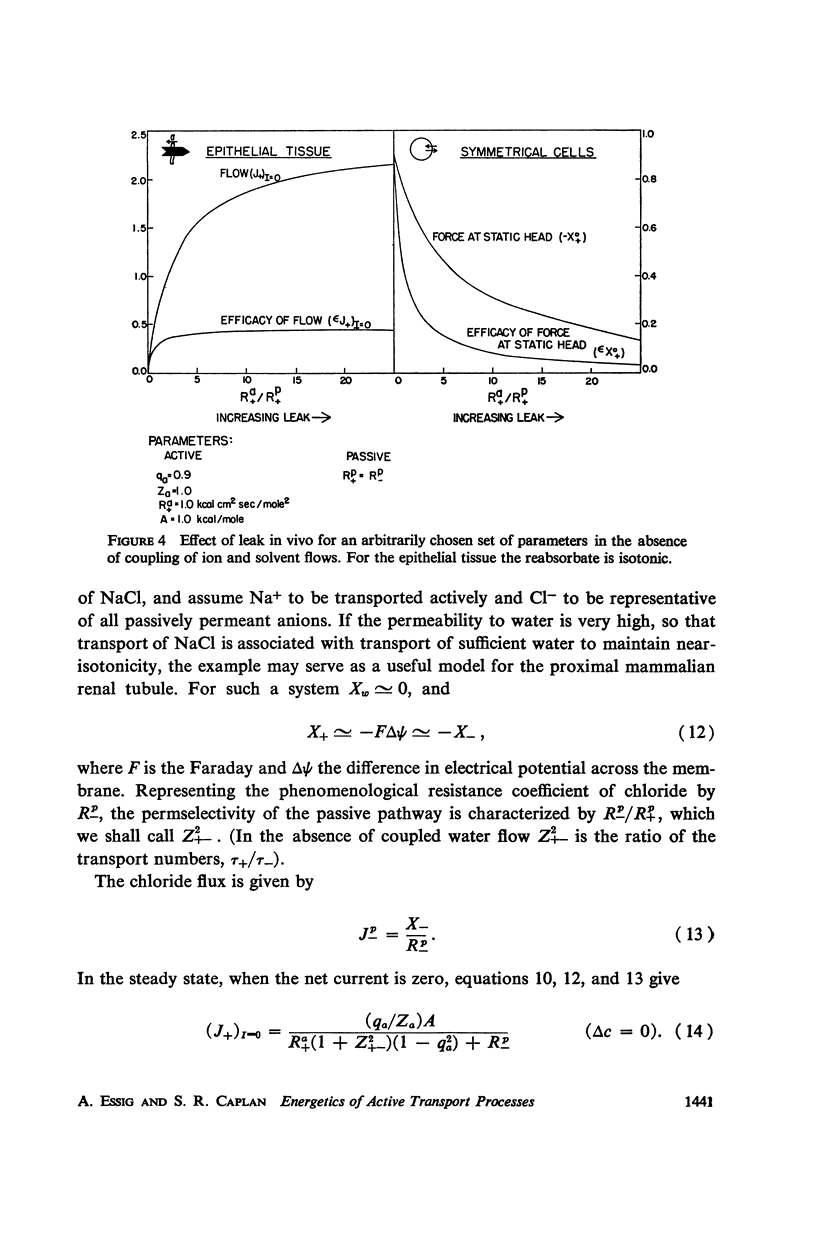
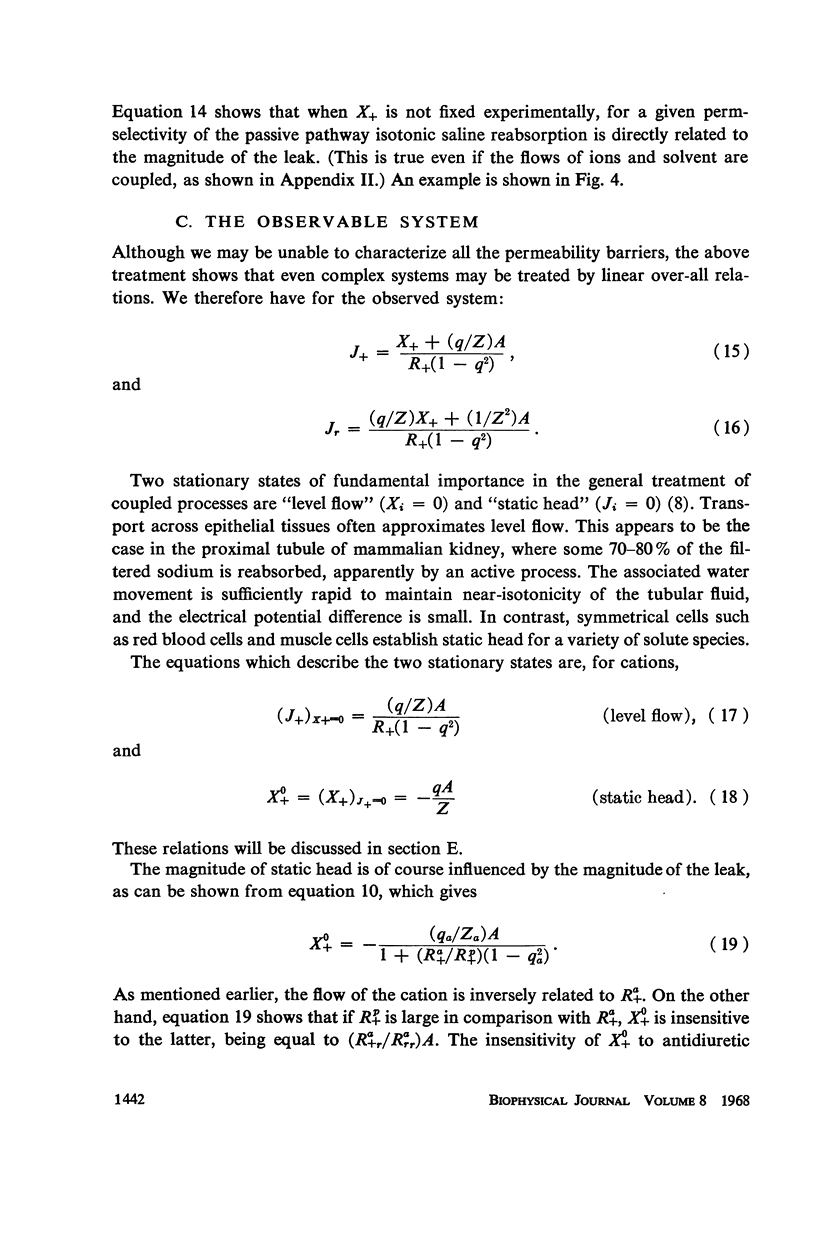
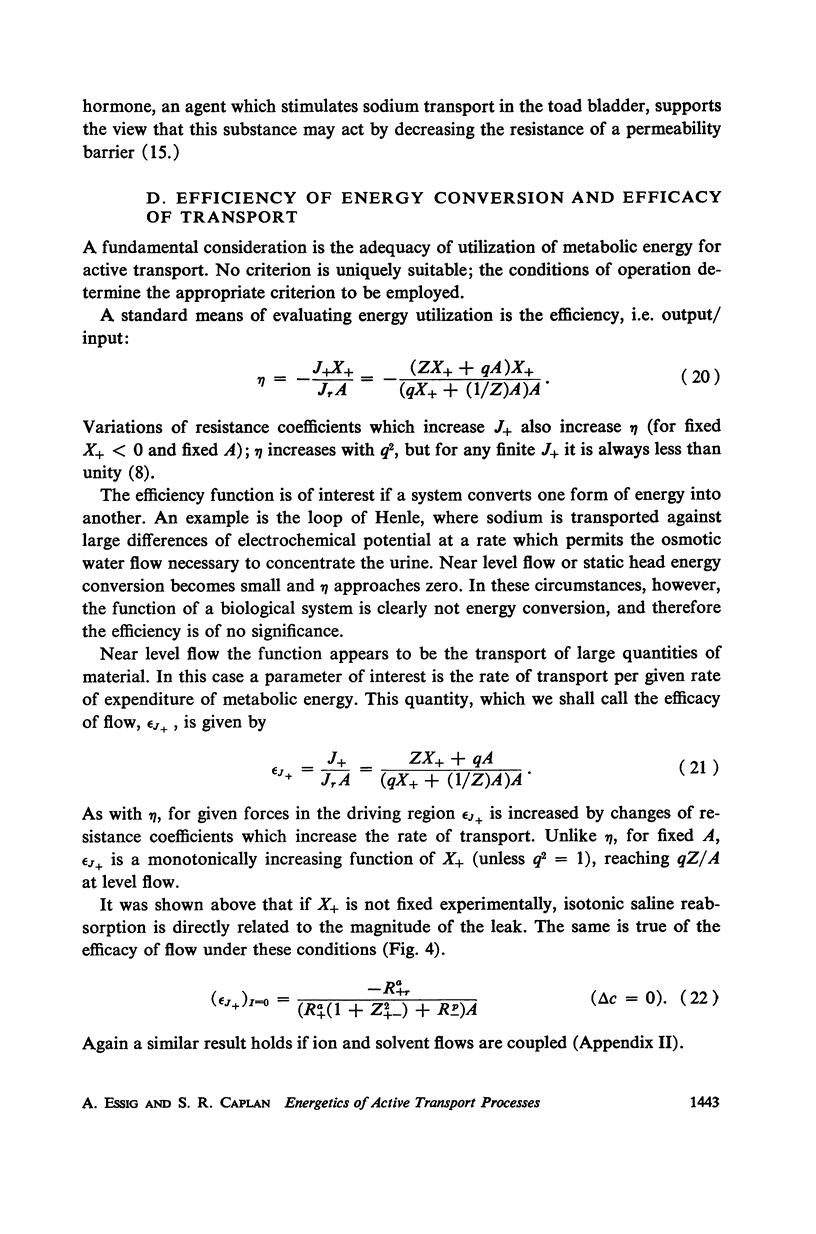
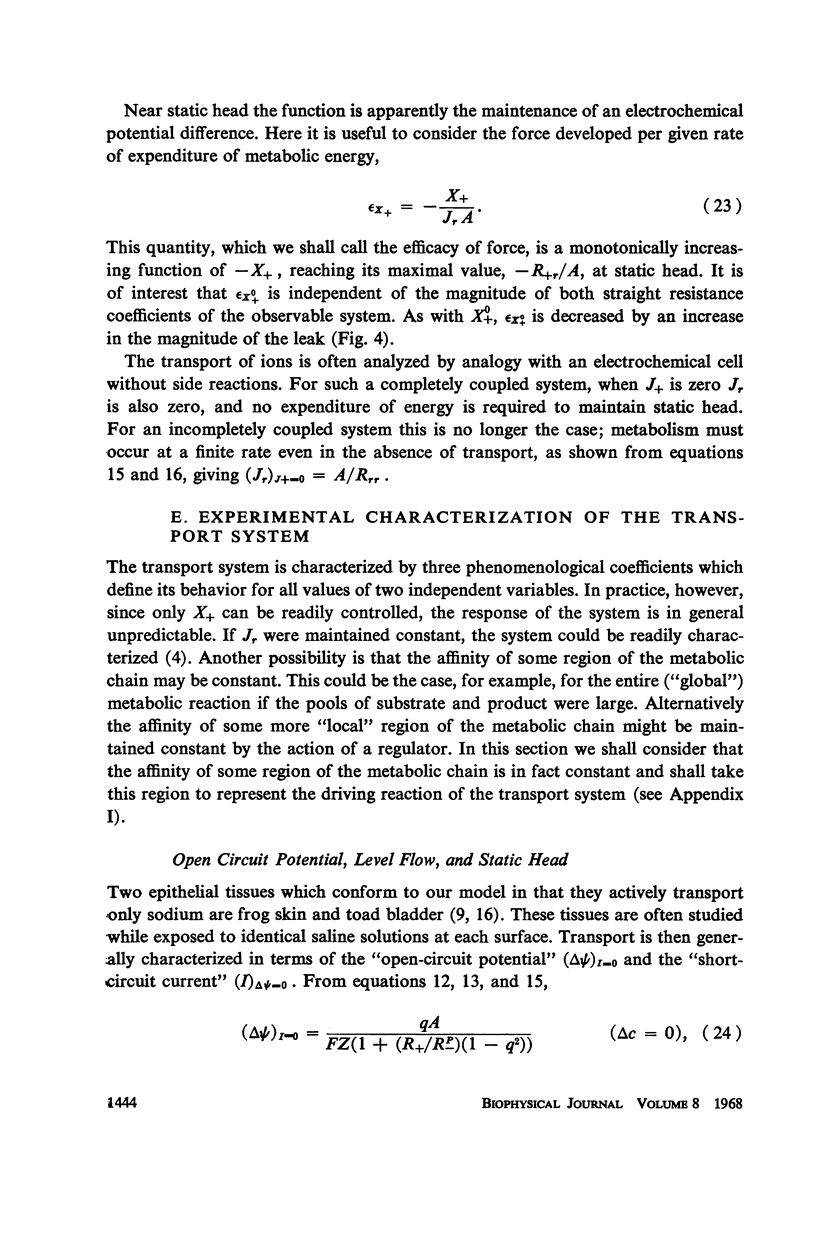
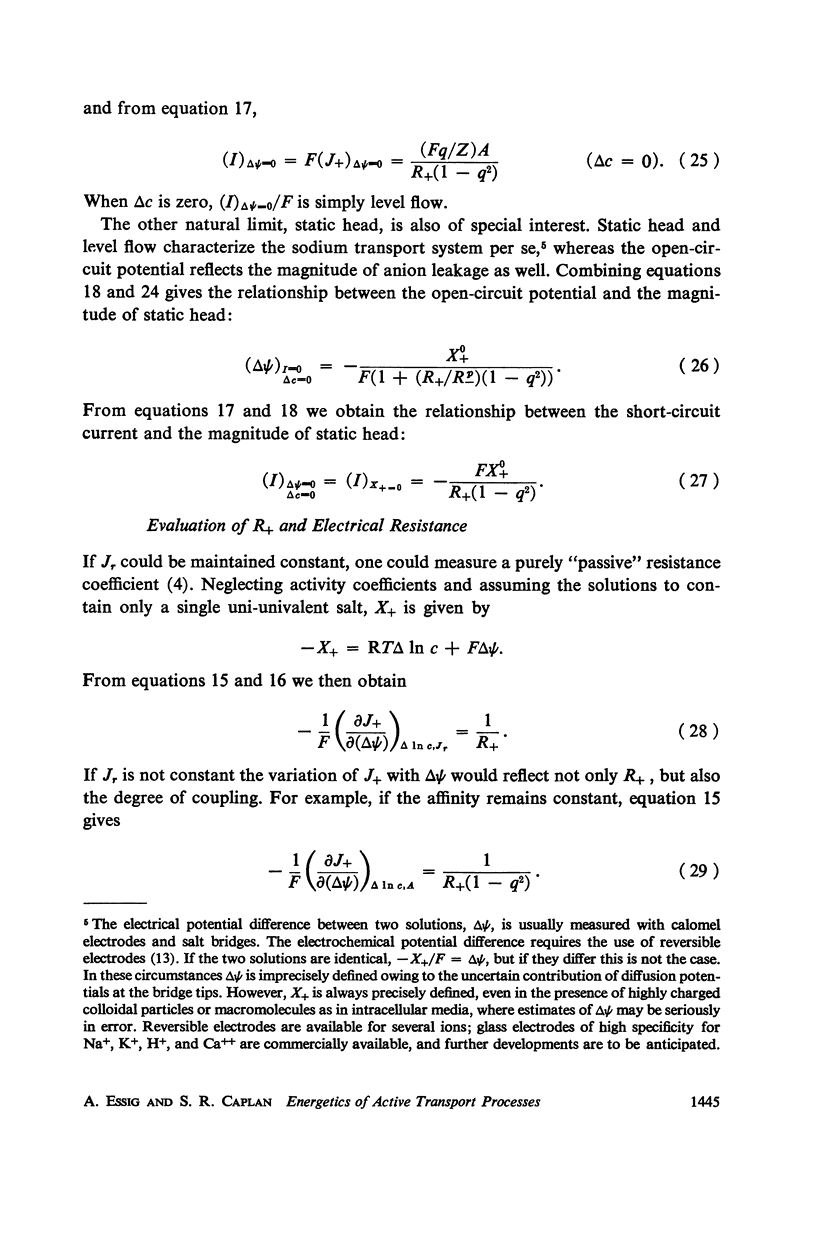
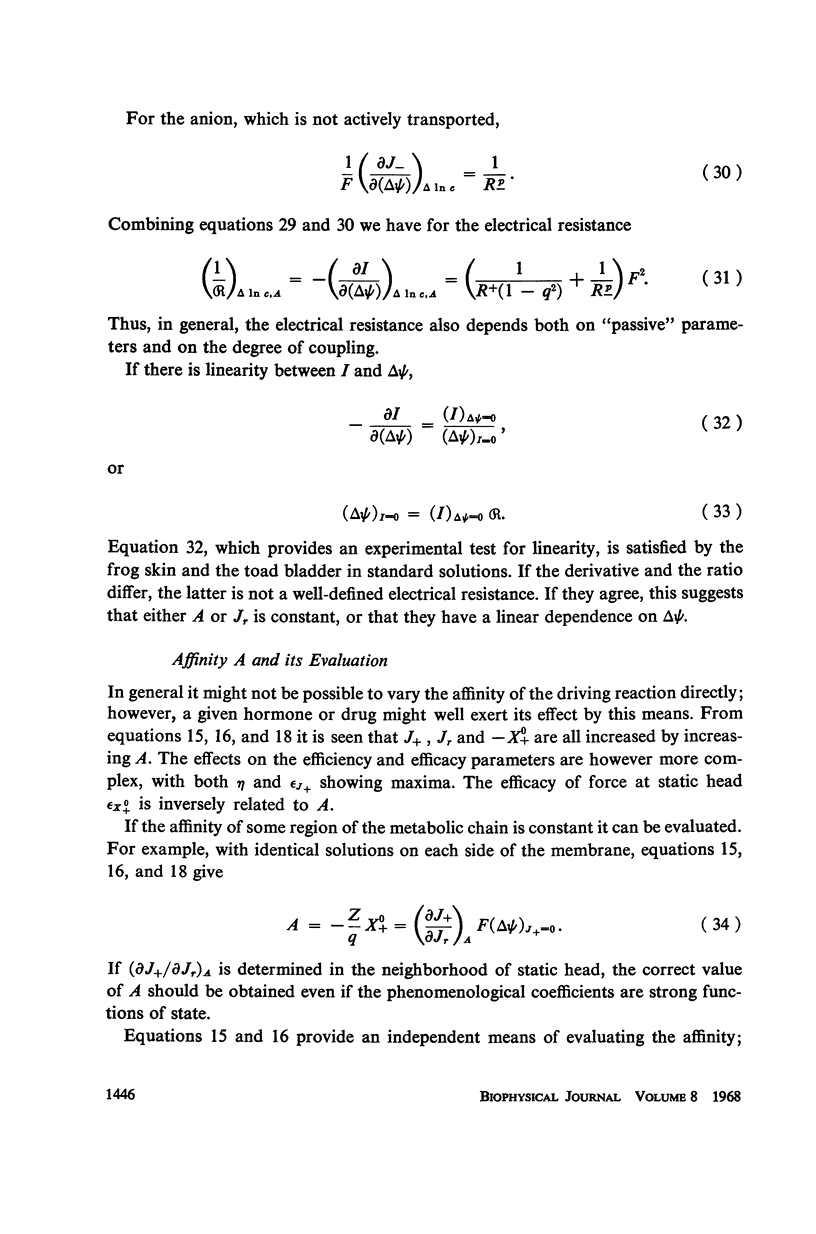
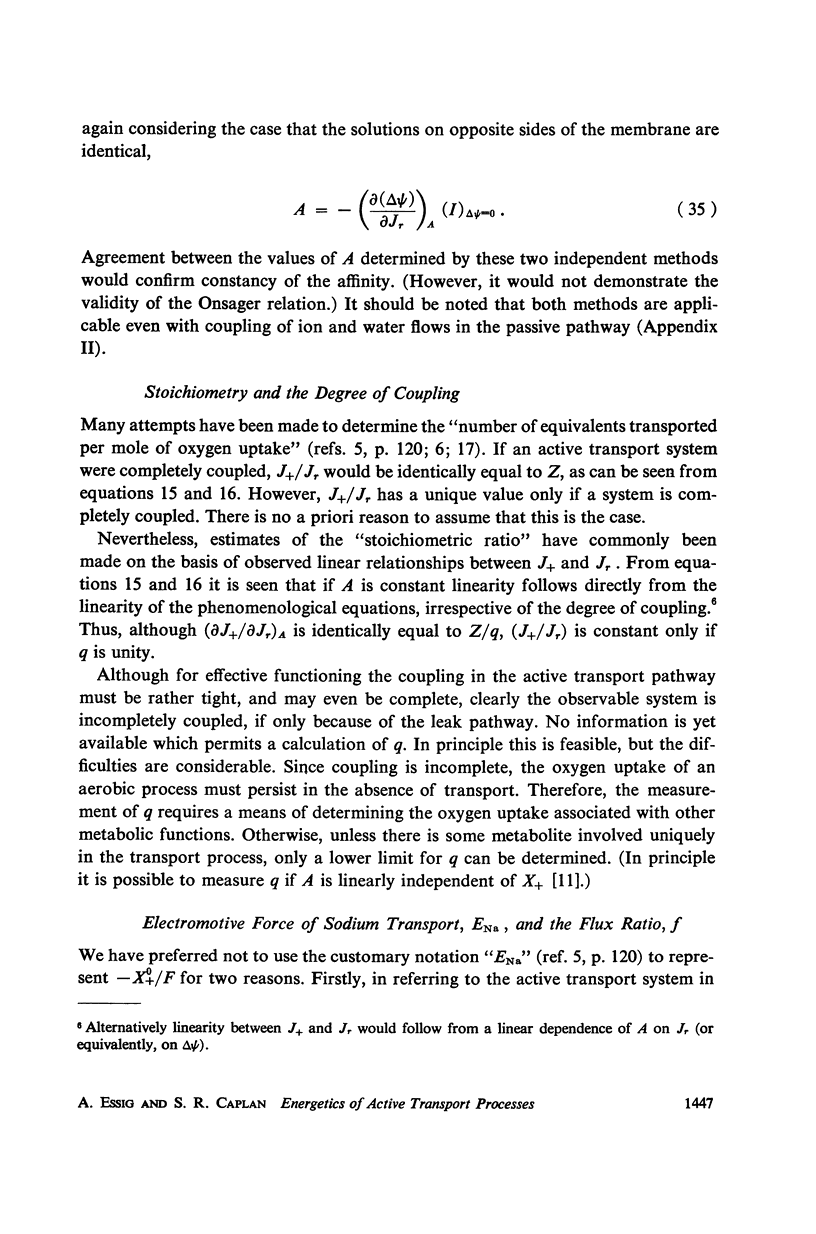
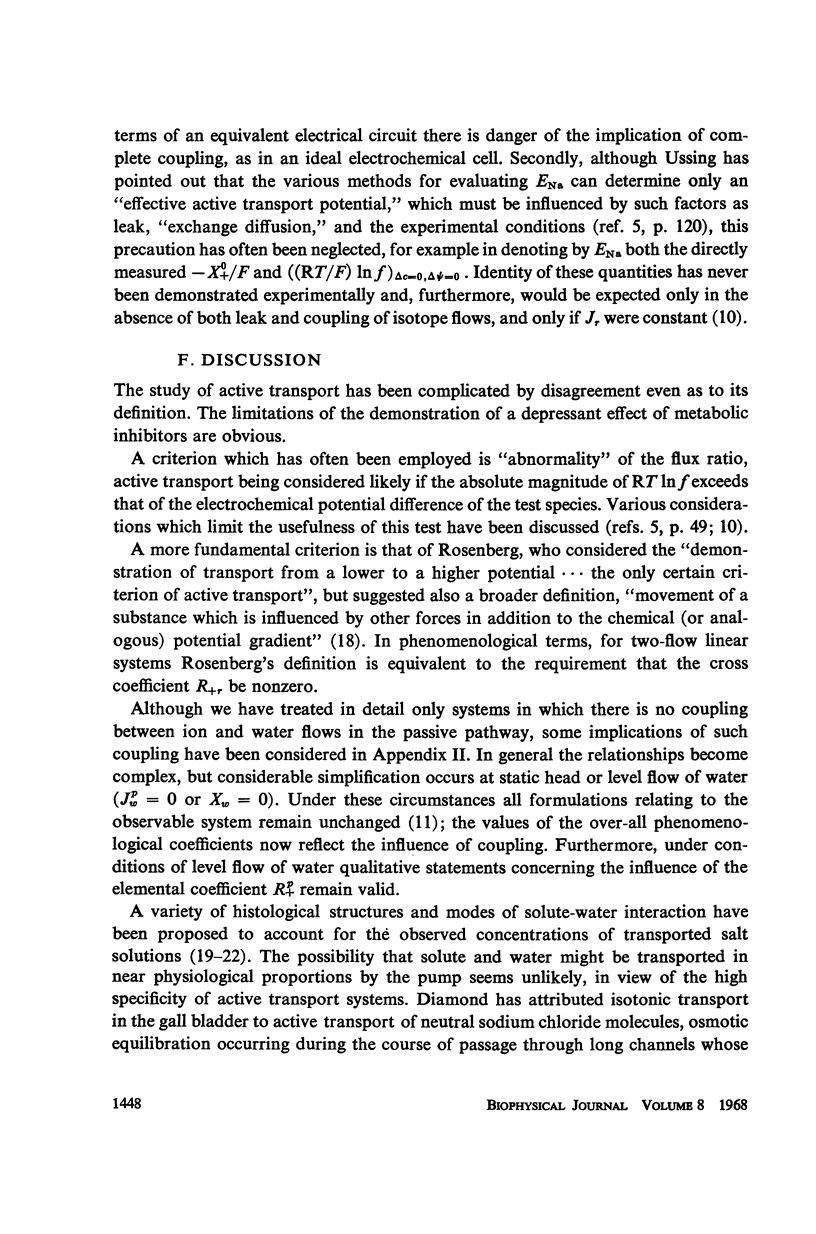
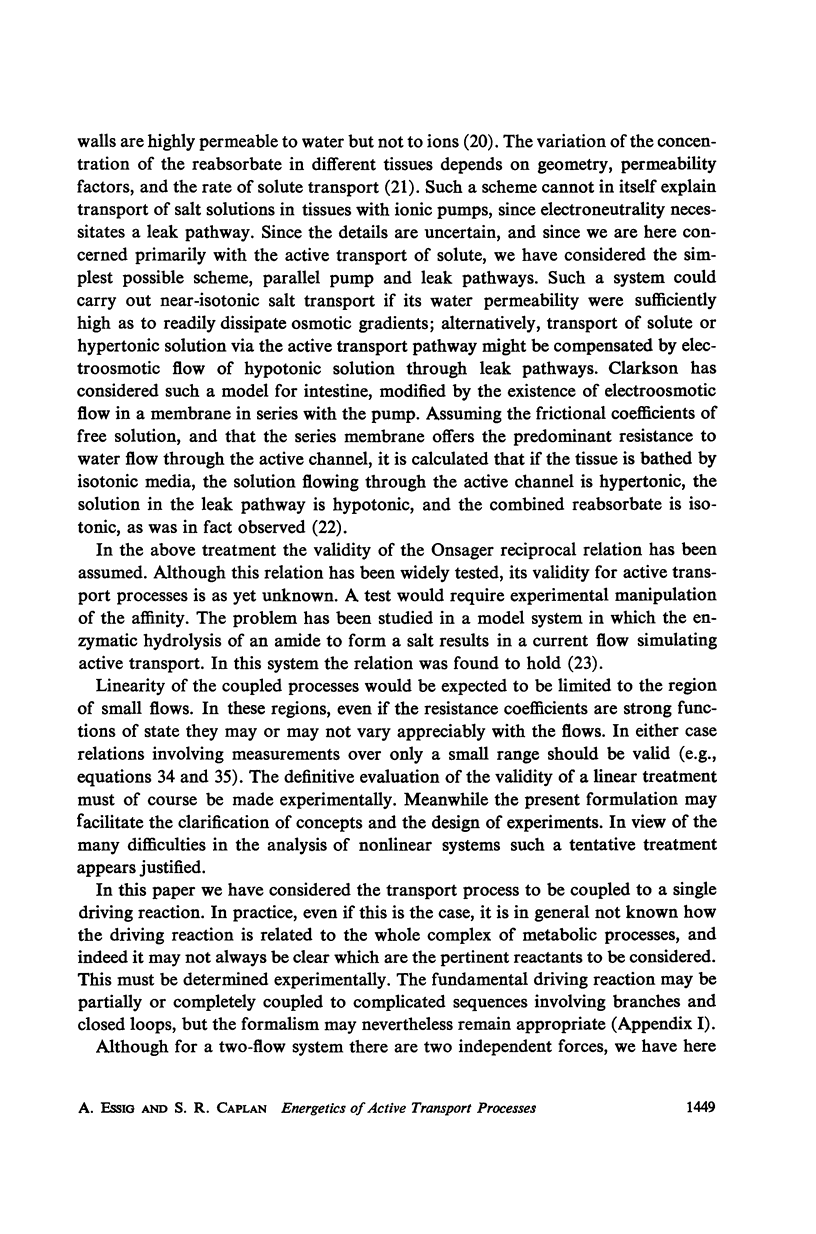
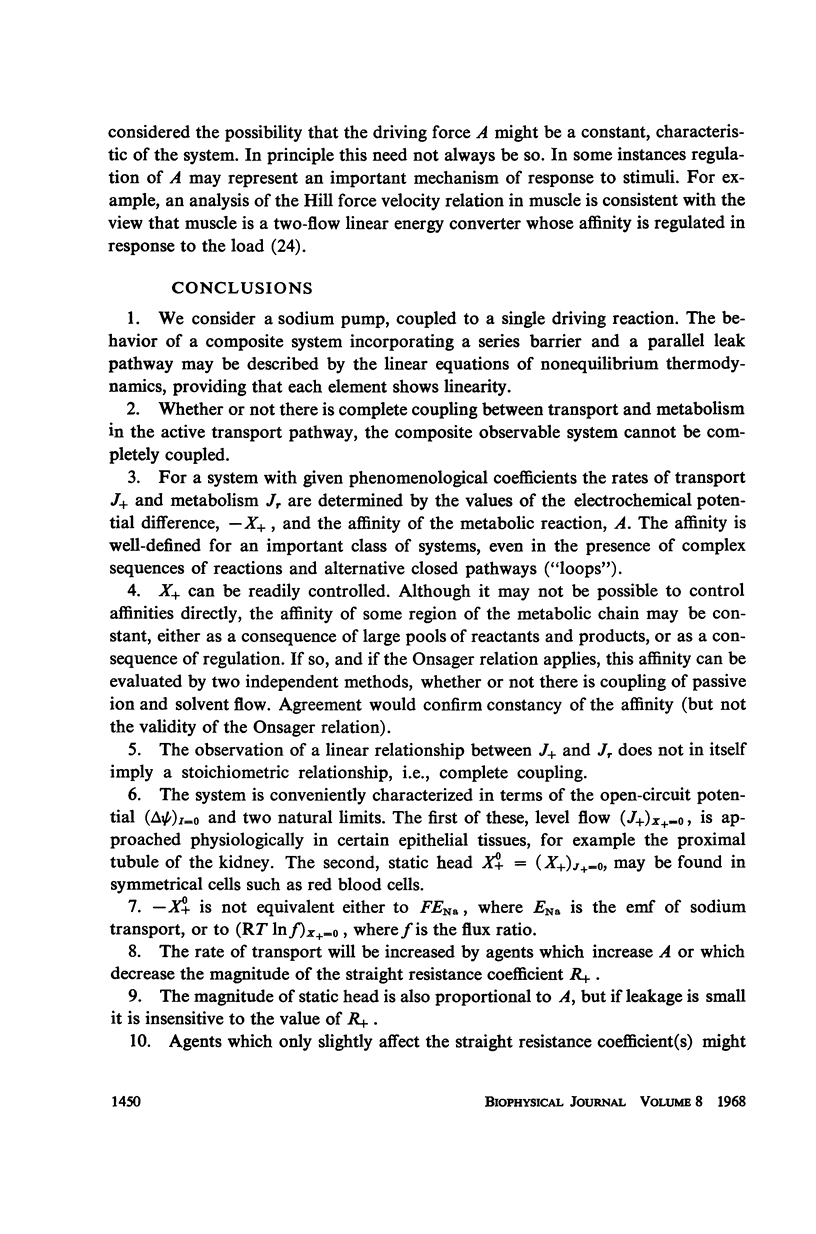
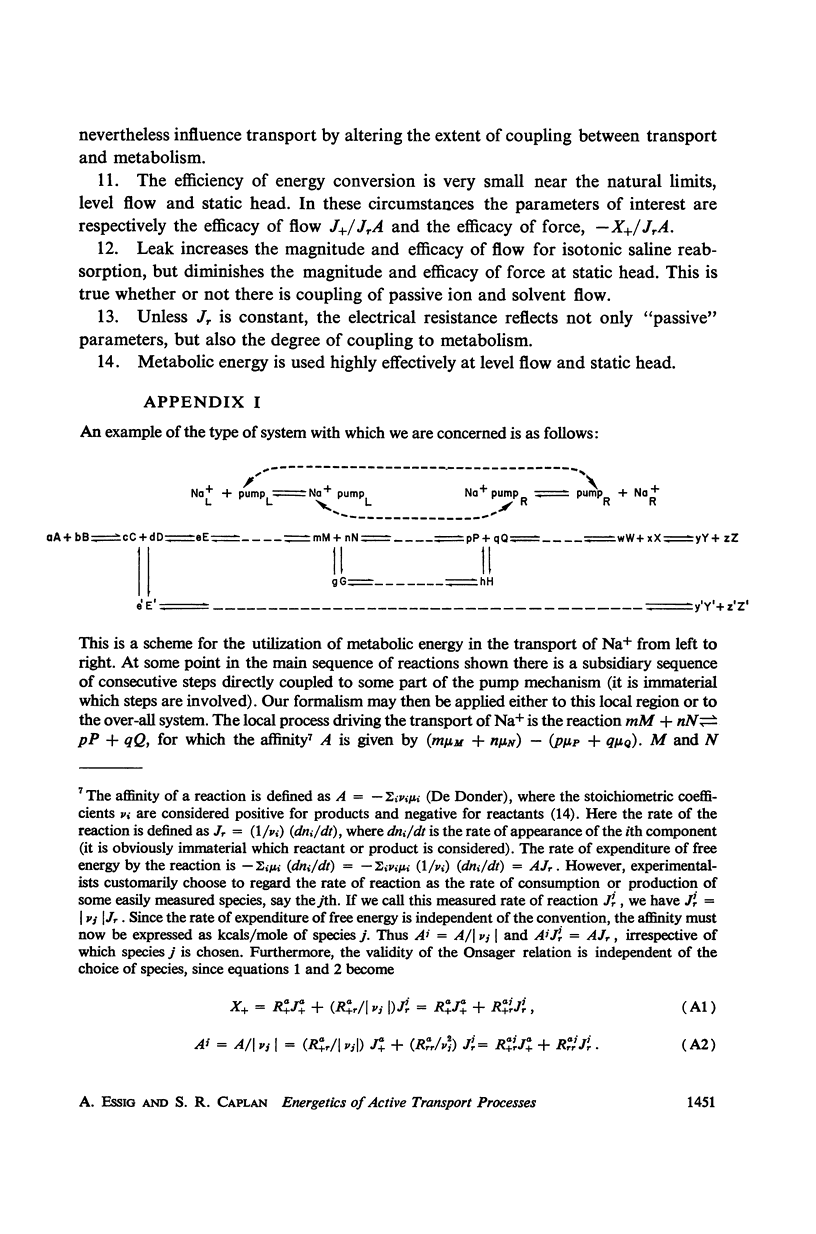
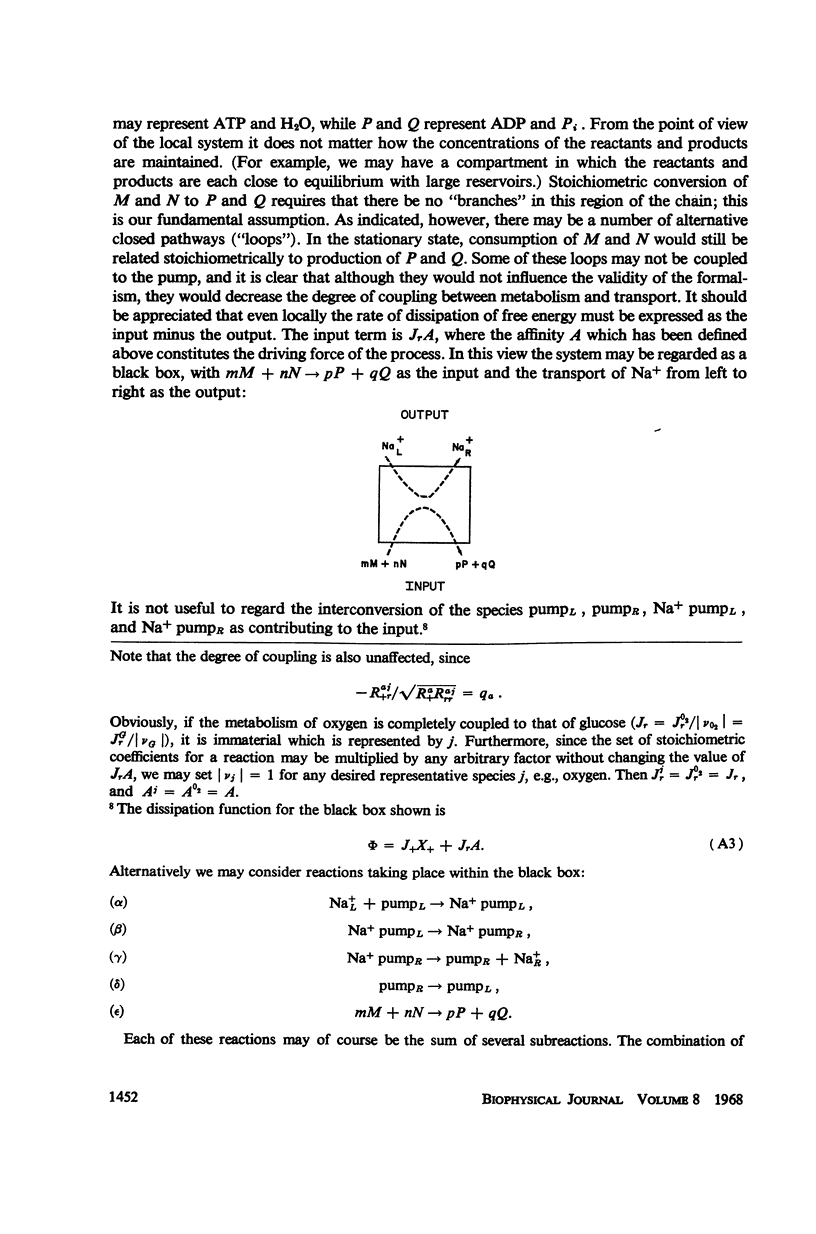
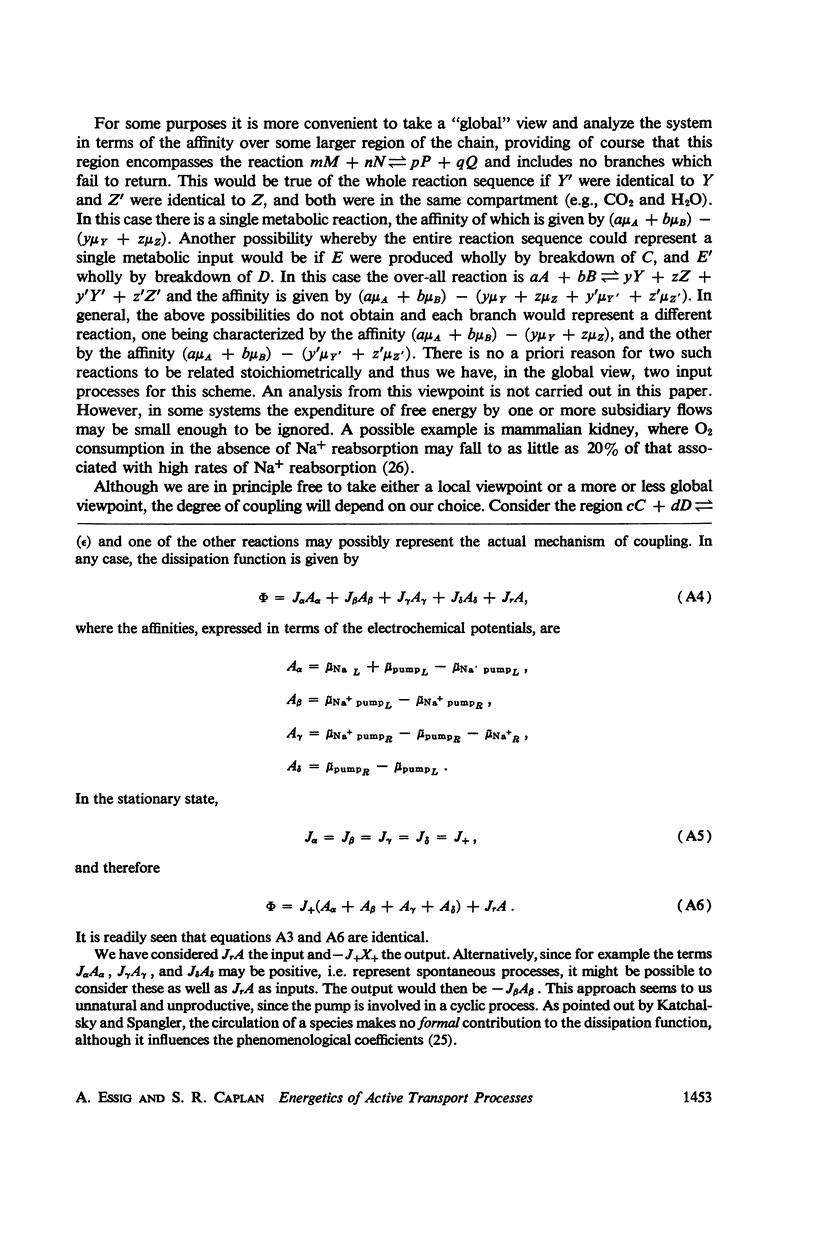
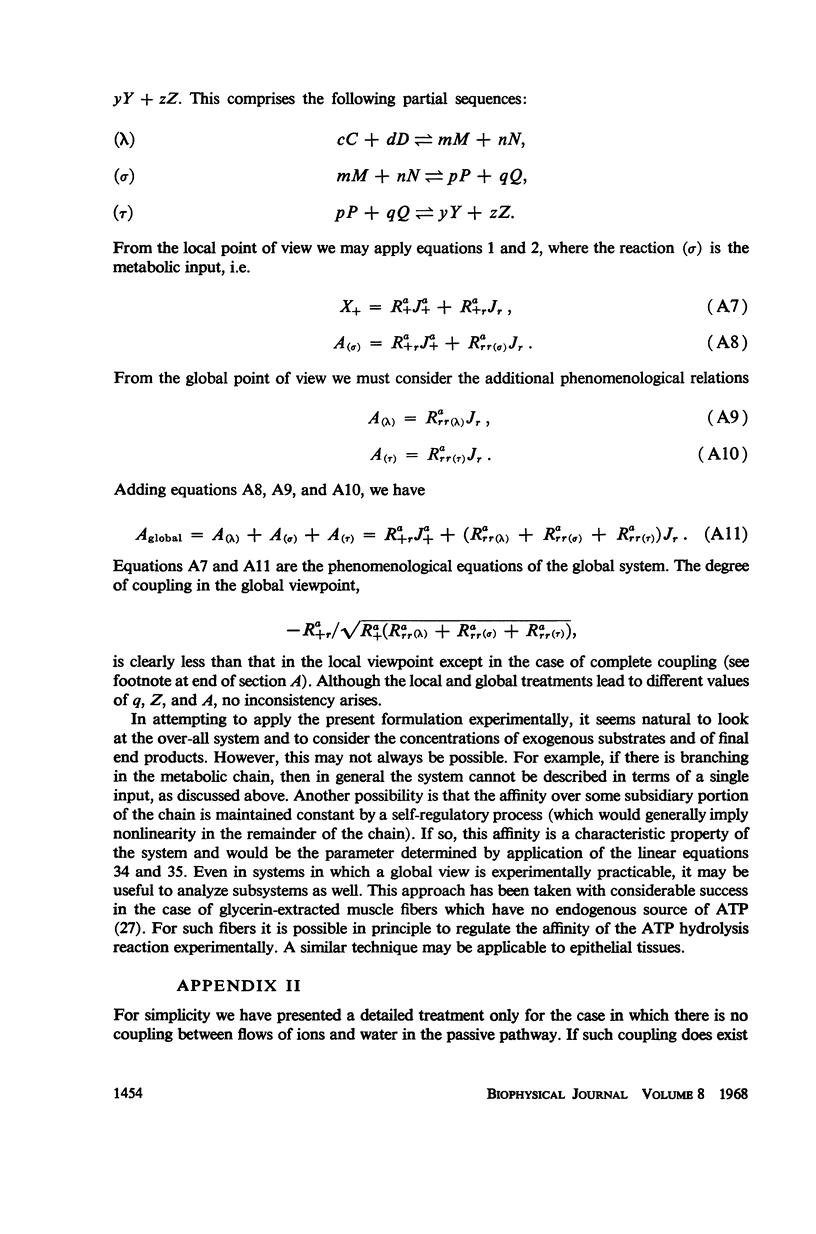
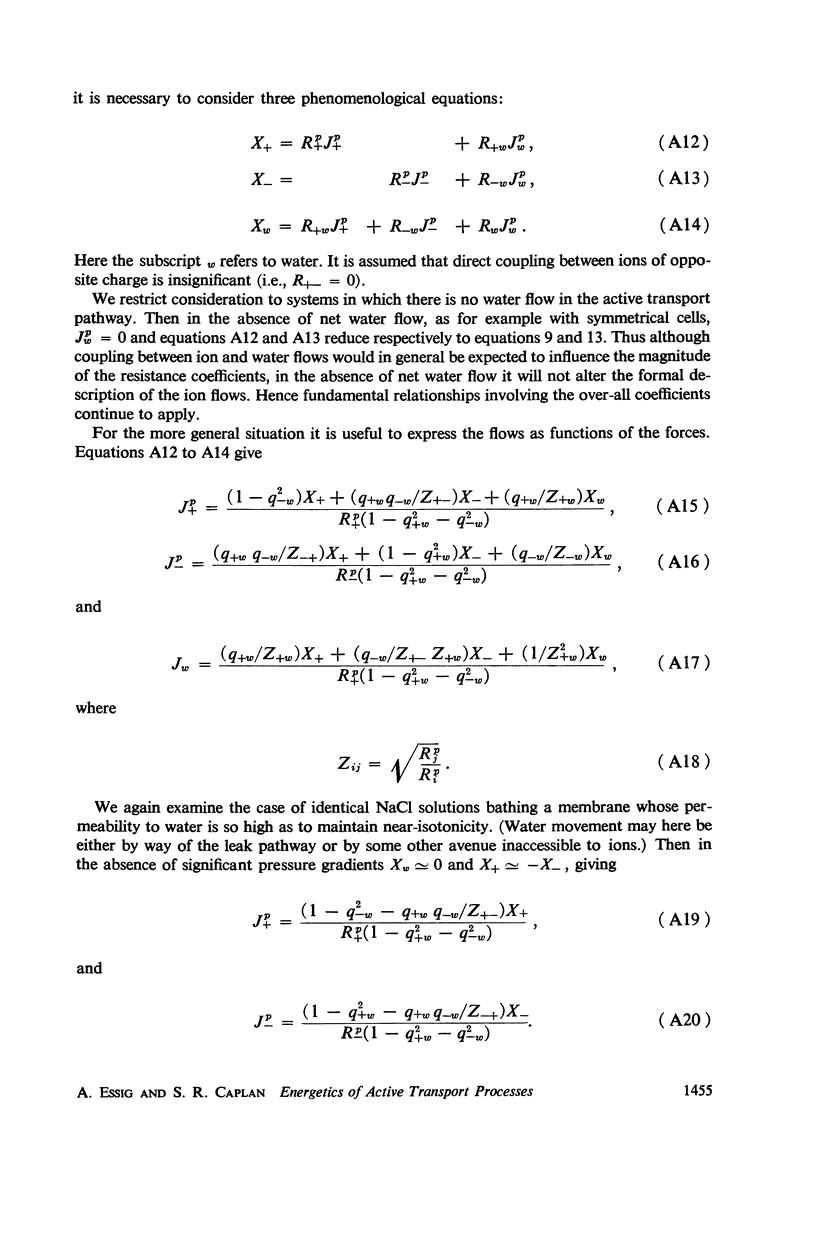
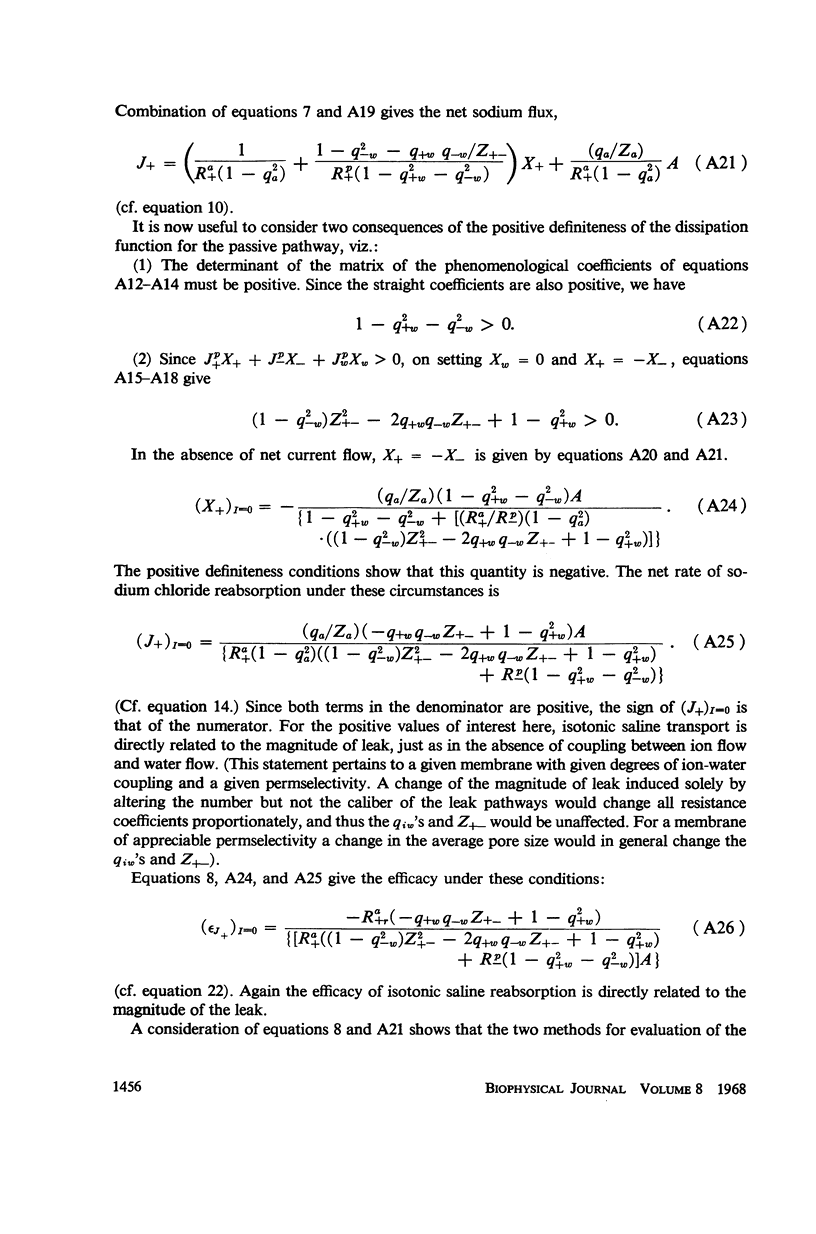
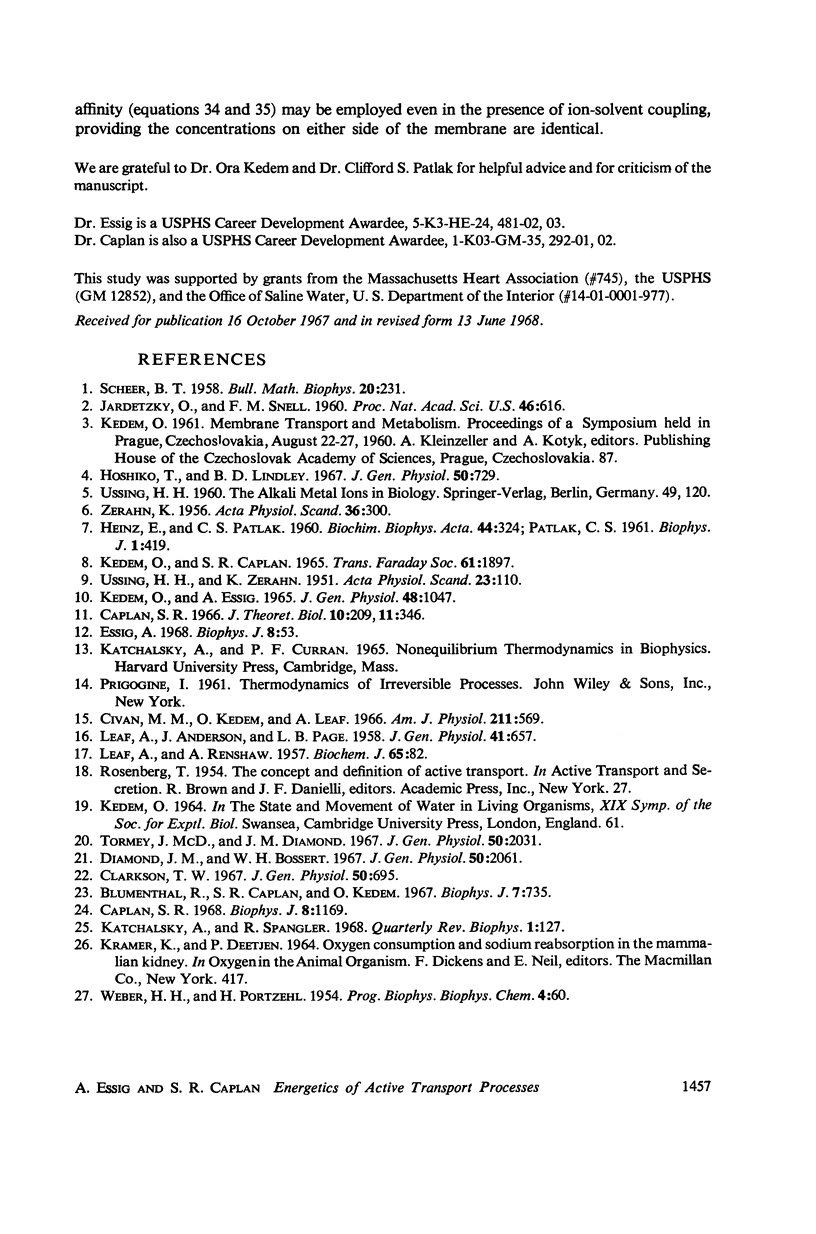
Selected References
These references are in PubMed. This may not be the complete list of references from this article.
- Blumenthal R., Caplan S. R., Kedem O. The coupling of an enzymatic reaction to transmembrane flow of electric current in a synthetic "active transport" system. Biophys J. 2008 Dec 31;7(6):735–757. doi: 10.1016/S0006-3495(67)86620-7. [DOI] [PMC free article] [PubMed] [Google Scholar]
- Civan M. M., Kedem O., Leaf A. Effect of vasopressin on toad bladder under conditions of zero net sodium transport. Am J Physiol. 1966 Sep;211(3):569–575. doi: 10.1152/ajplegacy.1966.211.3.569. [DOI] [PubMed] [Google Scholar]
- Clarkson T. W. The transport of salt and water across isolated rat ileum. Evidence for at least two distinct pathways. J Gen Physiol. 1967 Jan;50(3):695–727. doi: 10.1085/jgp.50.3.695. [DOI] [PMC free article] [PubMed] [Google Scholar]
- Diamond J. M., Bossert W. H. Standing-gradient osmotic flow. A mechanism for coupling of water and solute transport in epithelia. J Gen Physiol. 1967 Sep;50(8):2061–2083. doi: 10.1085/jgp.50.8.2061. [DOI] [PMC free article] [PubMed] [Google Scholar]
- HEINZ E., PATLAK C. S. Energy expenditure by active transport mechanisms. Biochim Biophys Acta. 1960 Nov 4;44:324–334. doi: 10.1016/0006-3002(60)91568-7. [DOI] [PubMed] [Google Scholar]
- Hoshiko T., Lindley B. D. Phenomenological description of active transport of salt and water. J Gen Physiol. 1967 Jan;50(3):729–758. doi: 10.1085/jgp.50.3.729. [DOI] [PMC free article] [PubMed] [Google Scholar]
- Jardetzky O., Snell F. M. THEORETICAL ANALYSIS OF TRANSPORT PROCESSES IN LIVING SYSTEMS. Proc Natl Acad Sci U S A. 1960 May;46(5):616–622. doi: 10.1073/pnas.46.5.616. [DOI] [PMC free article] [PubMed] [Google Scholar]
- Katchalsky A., Spangler R. Dynamics of membrane processes. Q Rev Biophys. 1968 Jun;1(2):127–175. doi: 10.1017/s0033583500000524. [DOI] [PubMed] [Google Scholar]
- Kedem O., Essig A. Isotope flows and flux ratios in biological membranes. J Gen Physiol. 1965 Jul;48(6):1047–1070. doi: 10.1085/jgp.48.6.1047. [DOI] [PMC free article] [PubMed] [Google Scholar]
- LEAF A., ANDERSON J., PAGE L. B. Active sodium transport by the isolated toad bladder. J Gen Physiol. 1958 Mar 20;41(4):657–668. doi: 10.1085/jgp.41.4.657. [DOI] [PMC free article] [PubMed] [Google Scholar]
- LEAF A., RENSHAW A. Ion transport and respiration of isolated frog skin. Biochem J. 1957 Jan;65(1):82–90. doi: 10.1042/bj0650082. [DOI] [PMC free article] [PubMed] [Google Scholar]
- Tormey J. M., Diamond J. M. The ultrastructural route of fluid transport in rabbit gall bladder. J Gen Physiol. 1967 Sep;50(8):2031–2060. doi: 10.1085/jgp.50.8.2031. [DOI] [PMC free article] [PubMed] [Google Scholar]
- USSING H. H., ZERAHN K. Active transport of sodium as the source of electric current in the short-circuited isolated frog skin. Acta Physiol Scand. 1951 Aug 25;23(2-3):110–127. doi: 10.1111/j.1748-1716.1951.tb00800.x. [DOI] [PubMed] [Google Scholar]
- ZERAHN K. Oxygen consumption and active sodium transport in the isolated and short-circuited frog skin. Acta Physiol Scand. 1956 May 31;36(4):300–318. doi: 10.1111/j.1748-1716.1956.tb01327.x. [DOI] [PubMed] [Google Scholar]