Abstract
The Na-K exchange pump is represented as a net stoichiometrically coupled reaction, r, involving ATP, Na+, and K+, and is located in the active region of the cell membrane. The reaction rate is Jr = Lrr (-ΔFr), where ΔFr is the free energy change of the reaction. ΔFr includes membrane potential ø2 in the absence of 1:1 coupling between Na+ and K+, and the reaction rate is potential dependent under these conditions. At the same time the pump will produce a potential H which is the difference between membrane potential and the diffusion potential as calculated with constant field assumptions. In the absence of 1:1 coupling, the pump is electrogenic. The feedback relation between reaction rate and membrane potential makes the membrane resistance in the presence of the pump less than or equal to the resistance in its absence, at the same membrane potential. H depends on stoichiometry, reaction rate, and passive ionic conductances. Experimental verification of the model will depend on the accuracy of permeability determinations. Dissipation and efficiency of transport can be calculated also.
Full text
PDF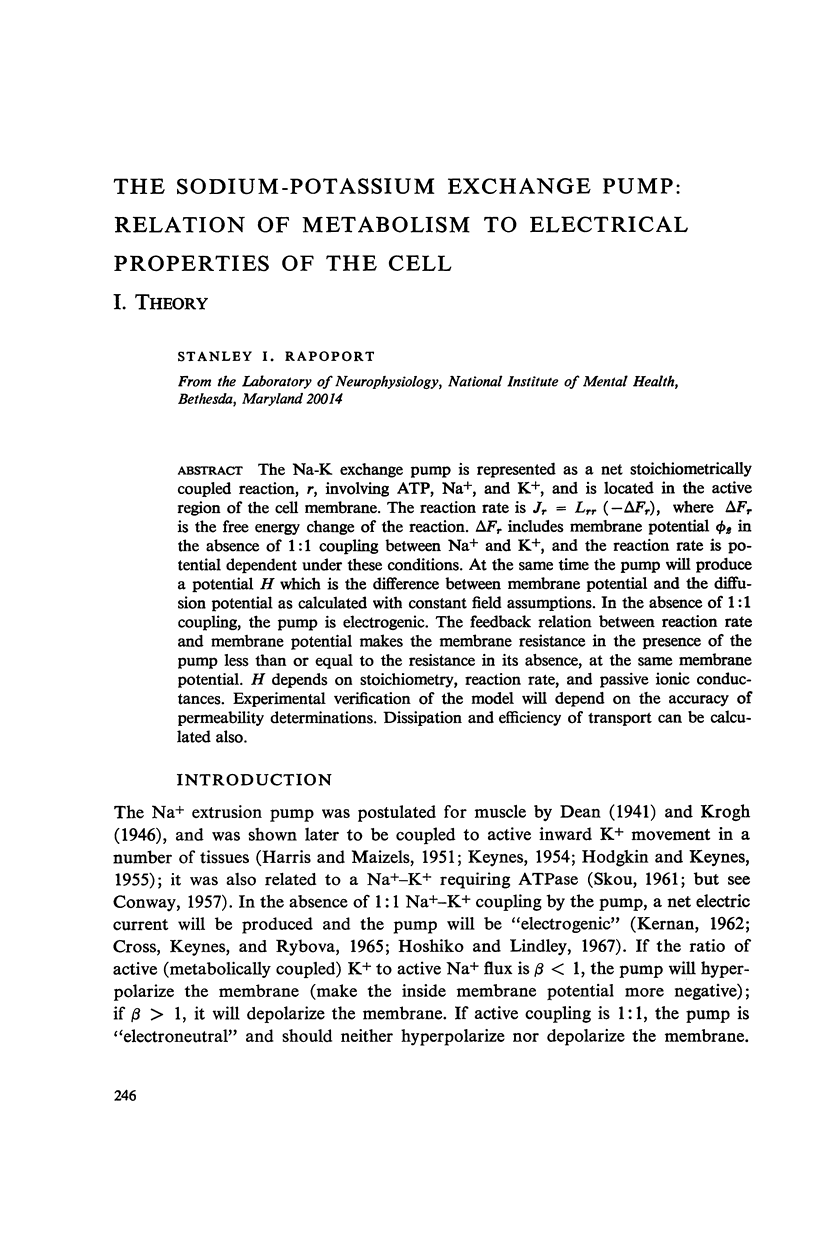
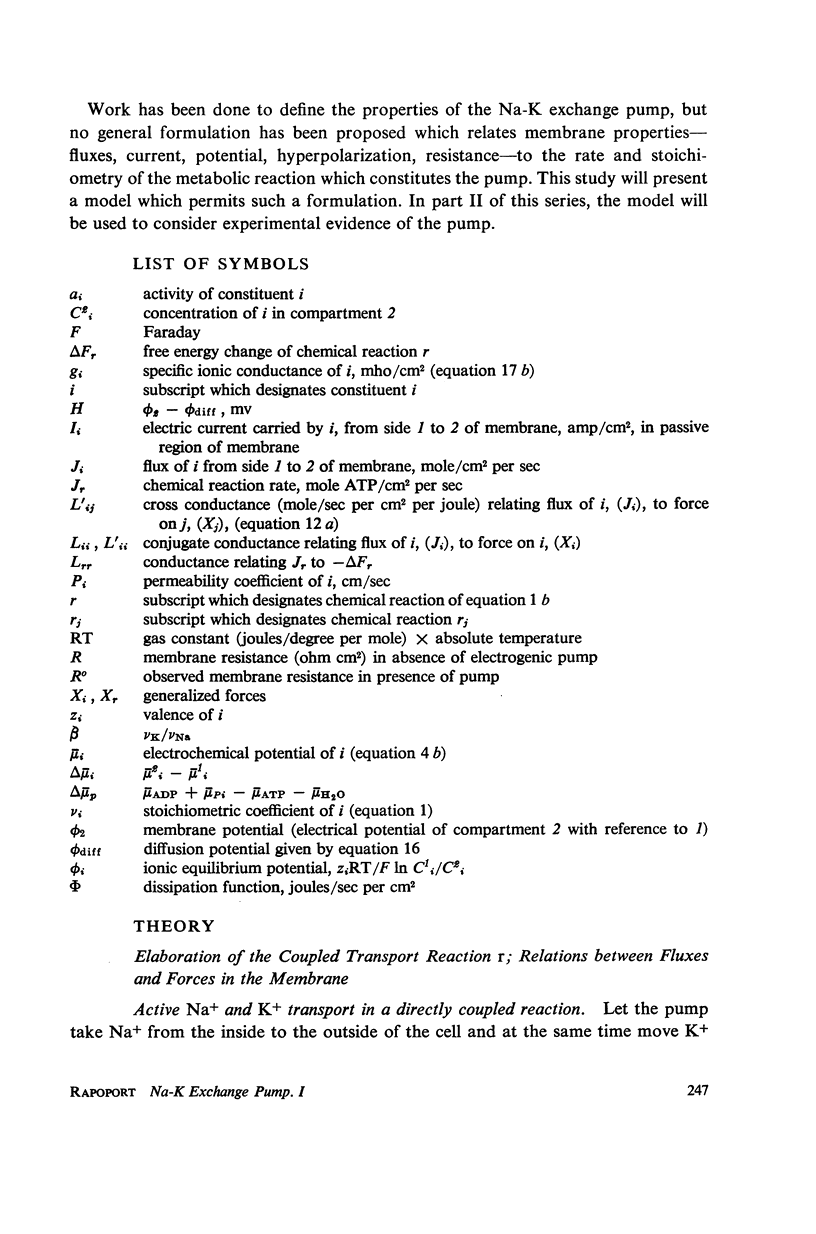
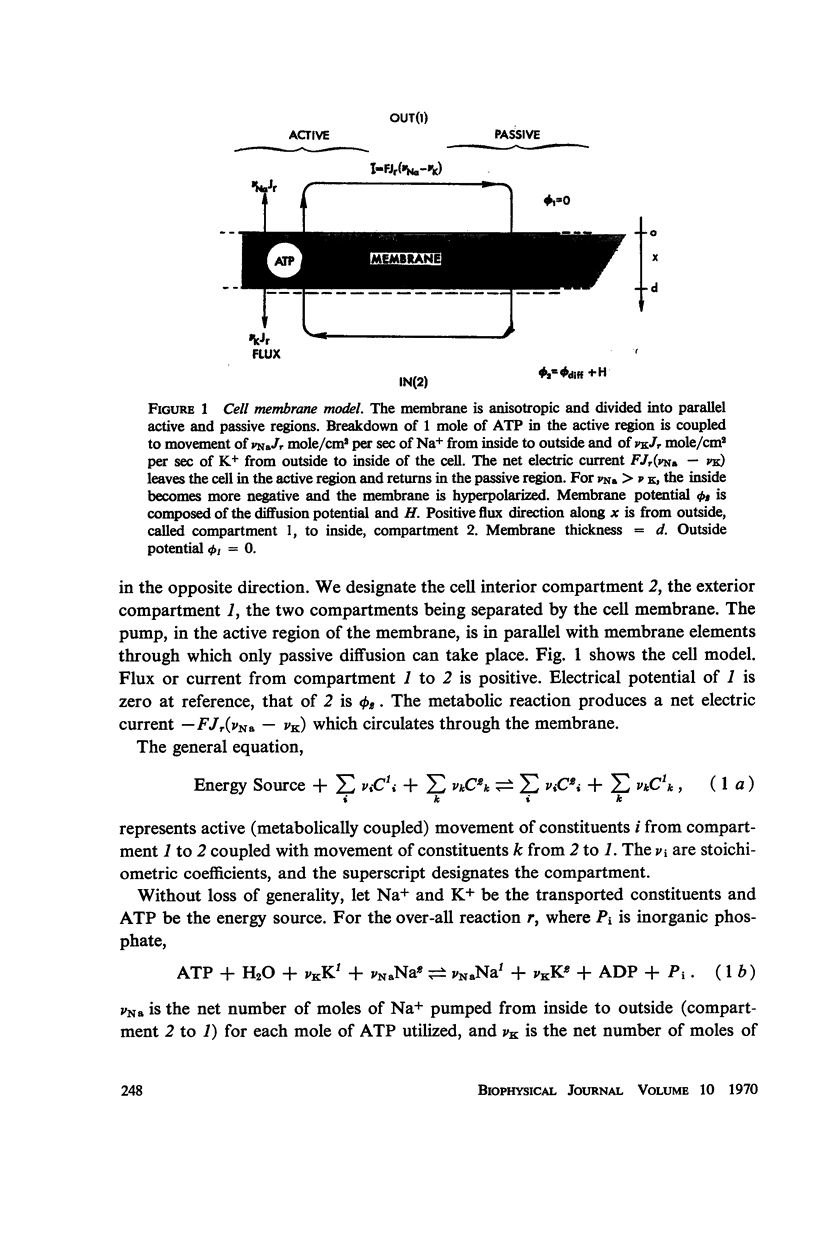
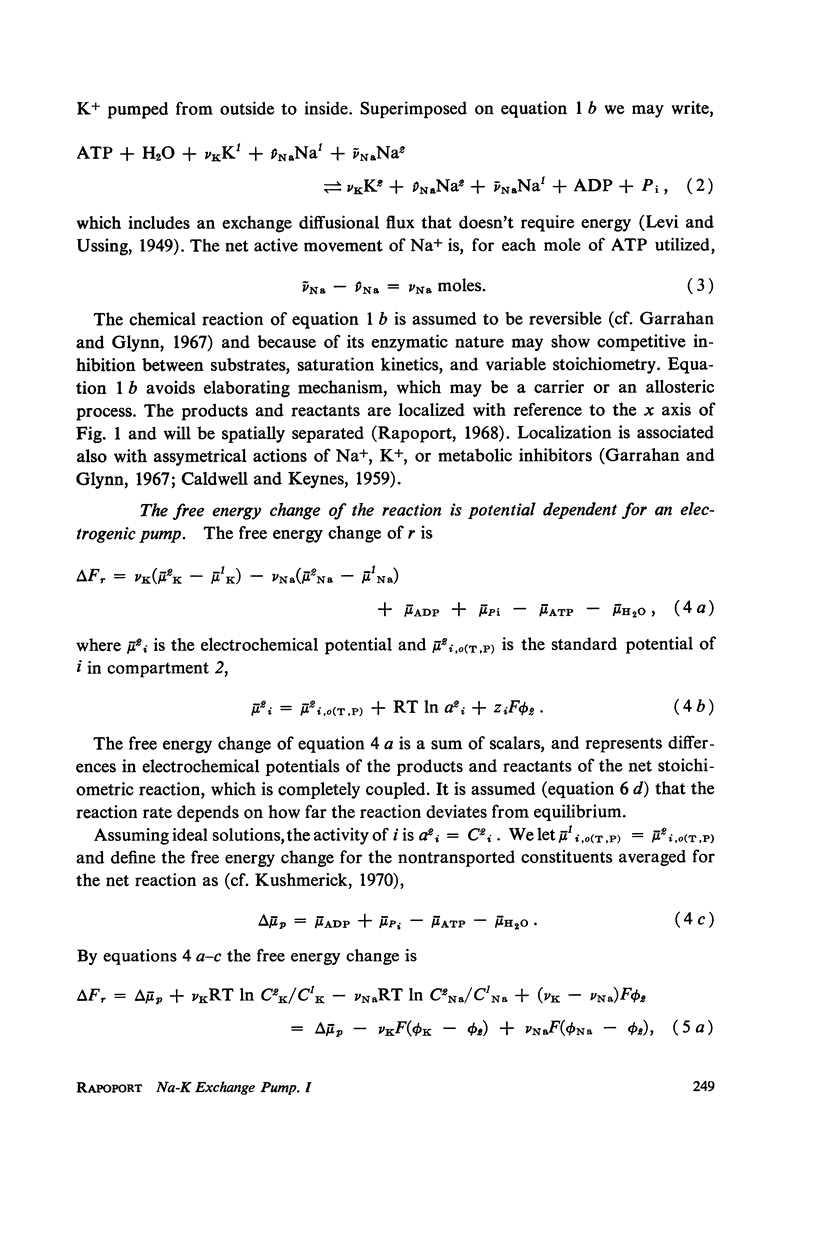
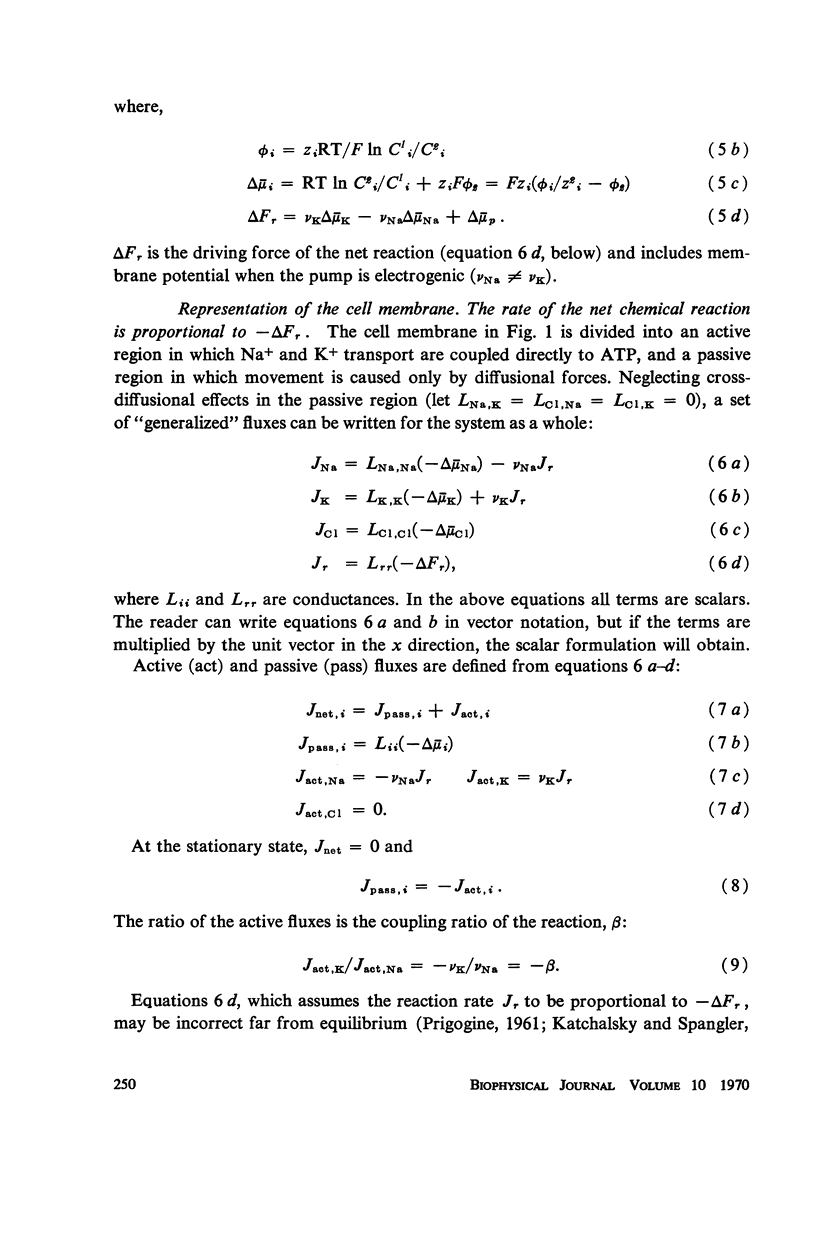
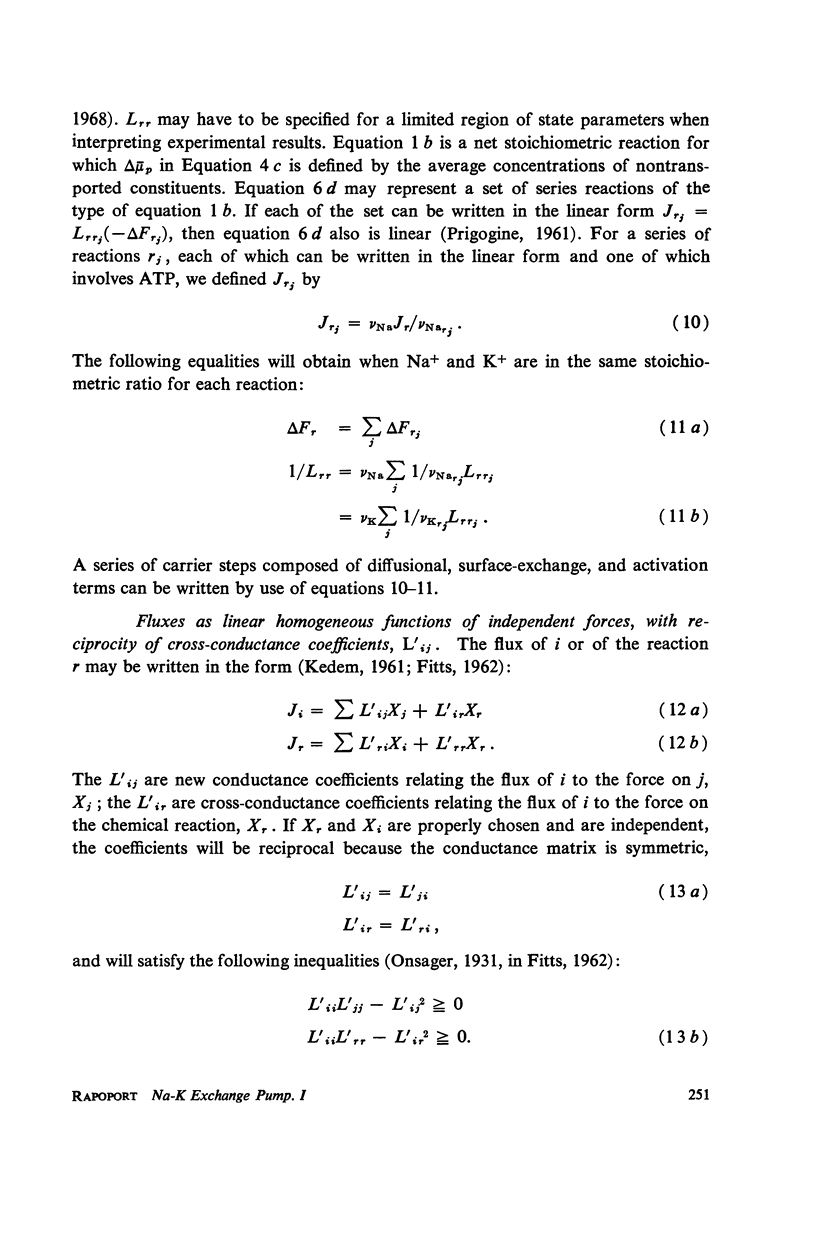
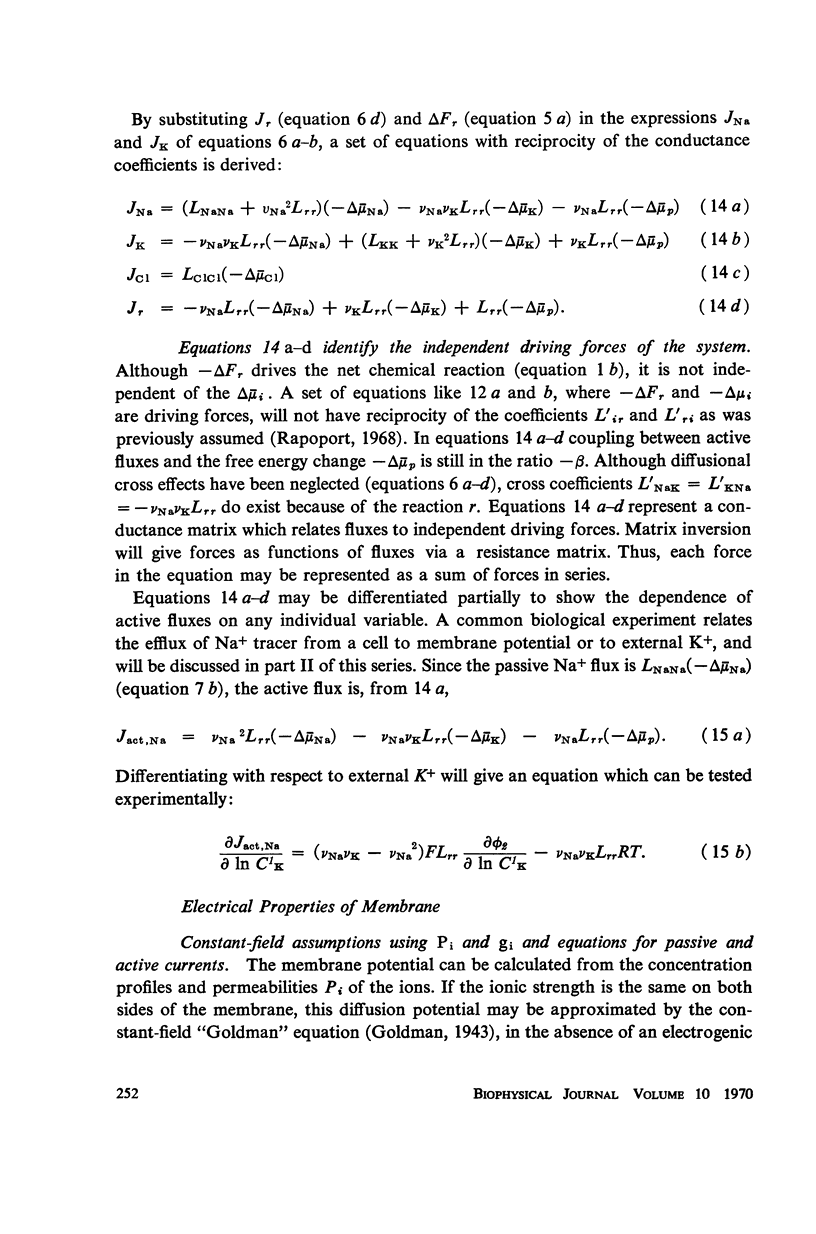
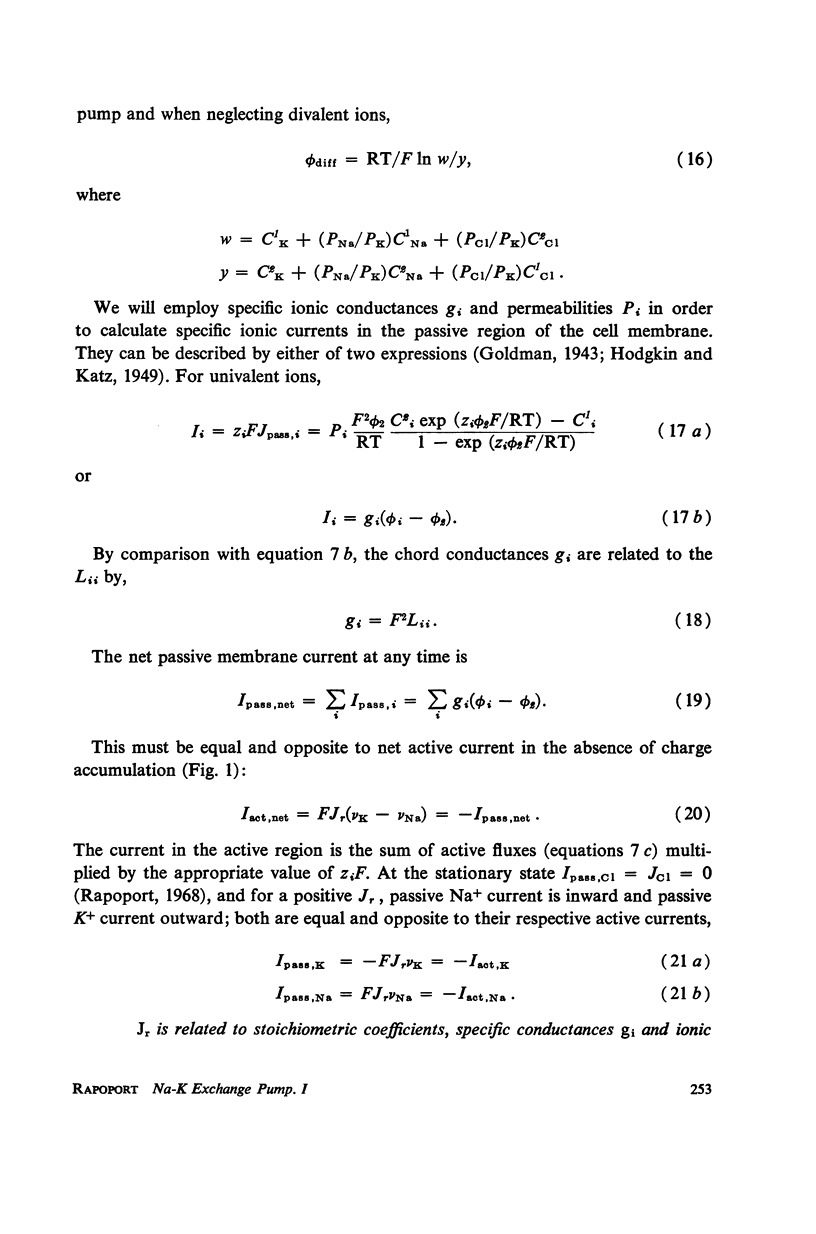
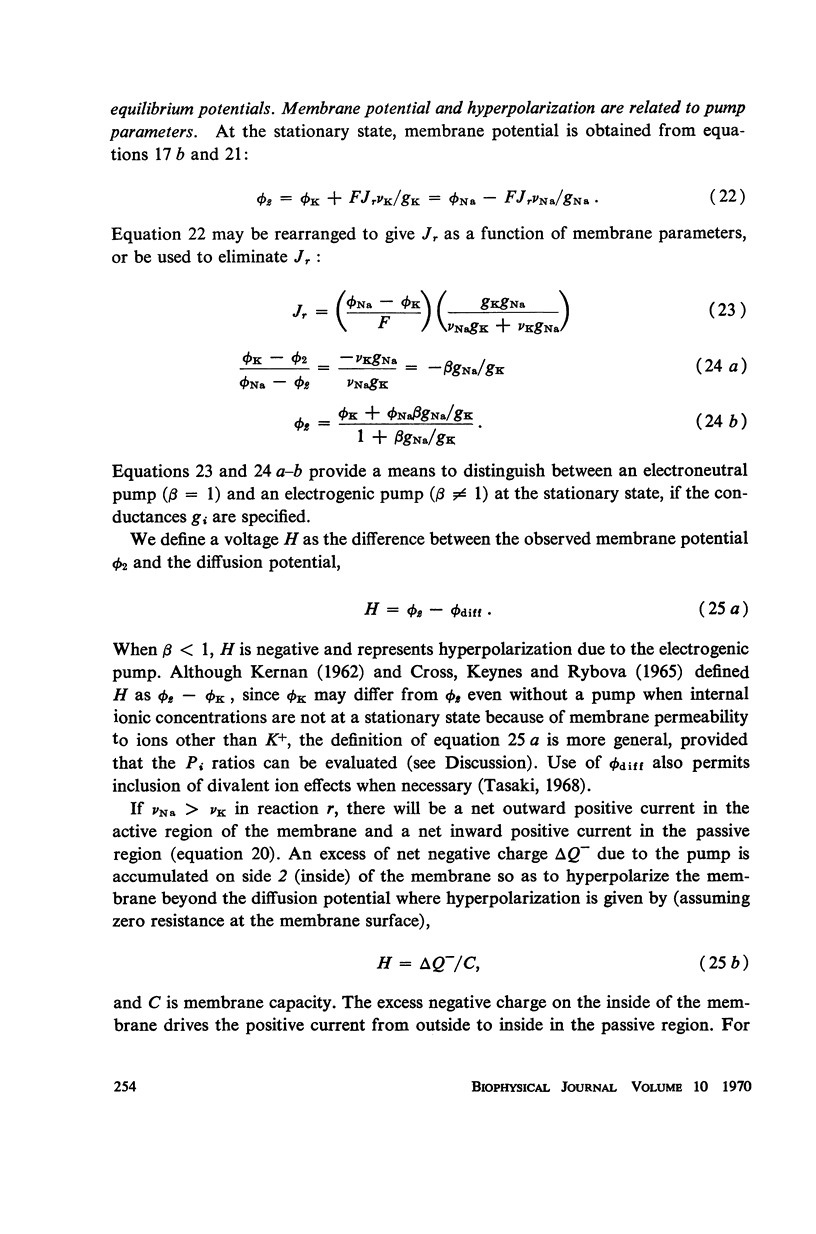
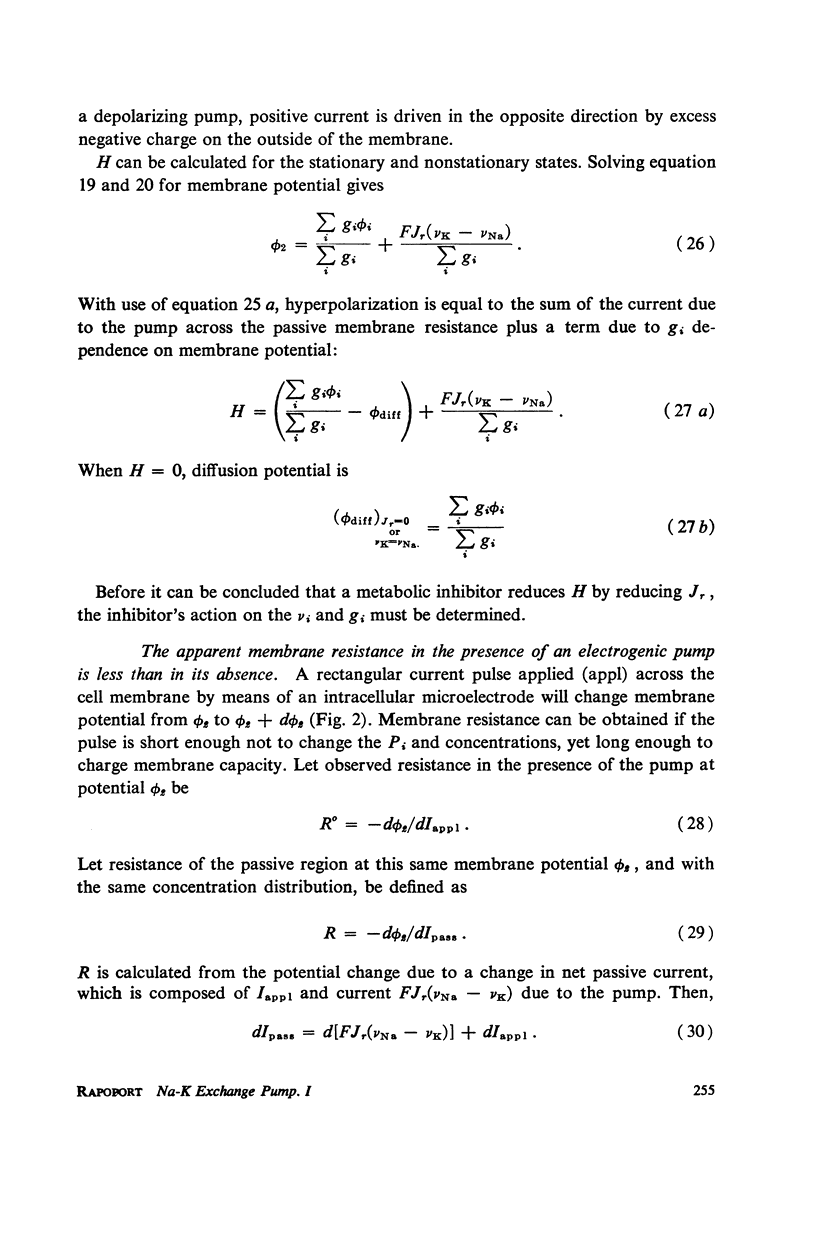
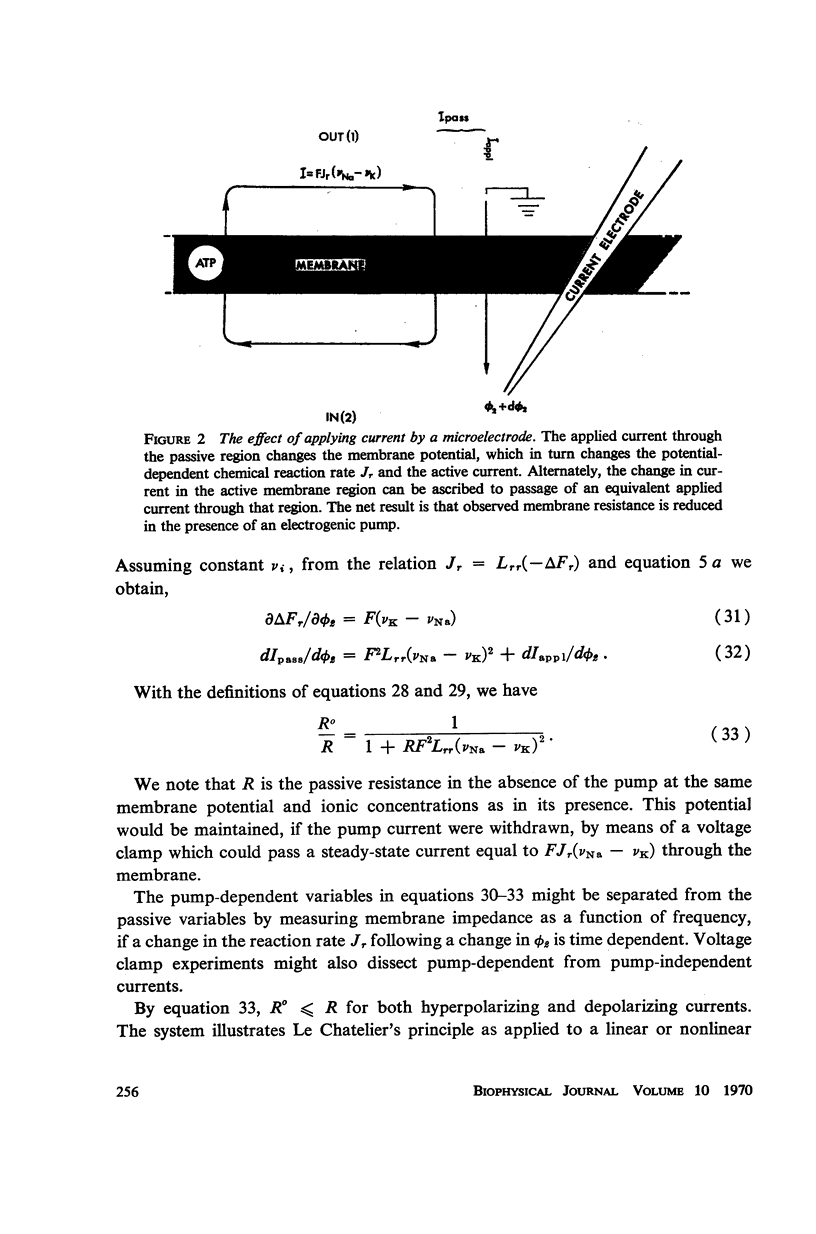
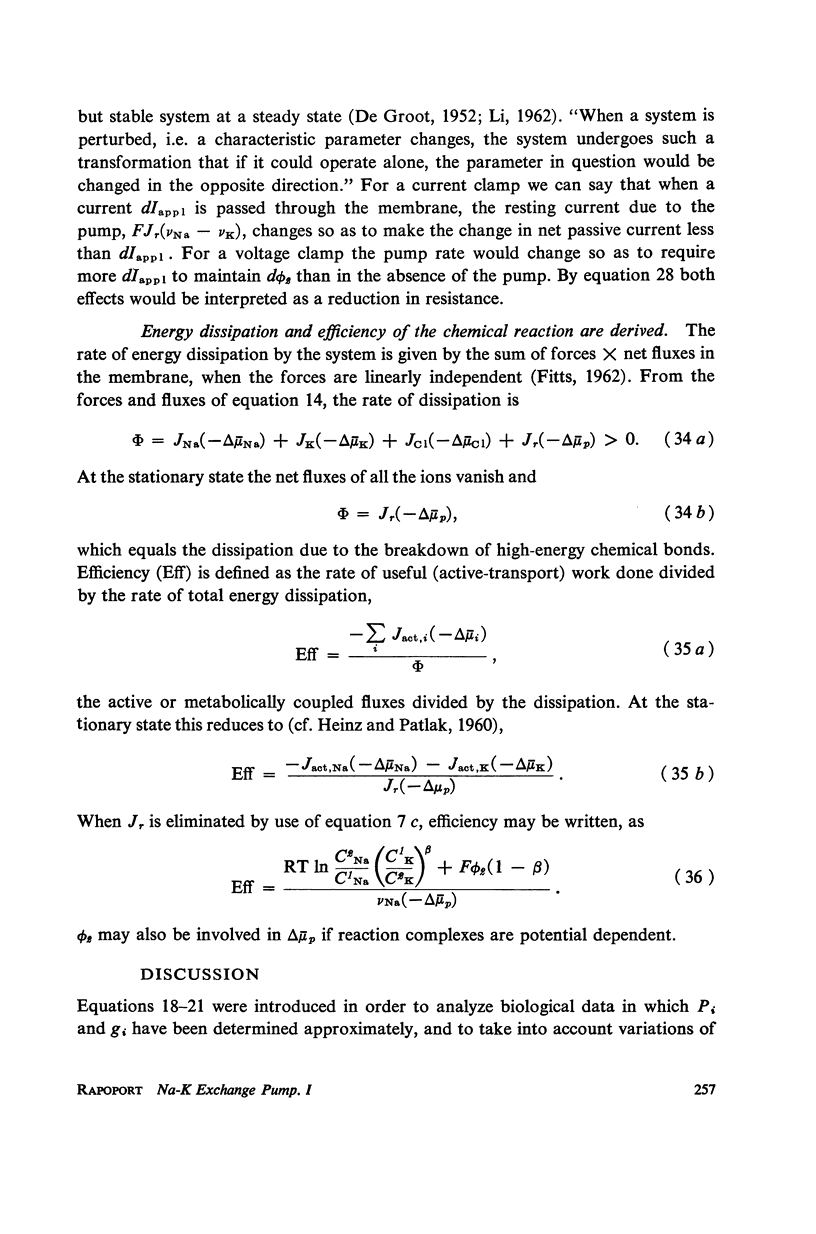
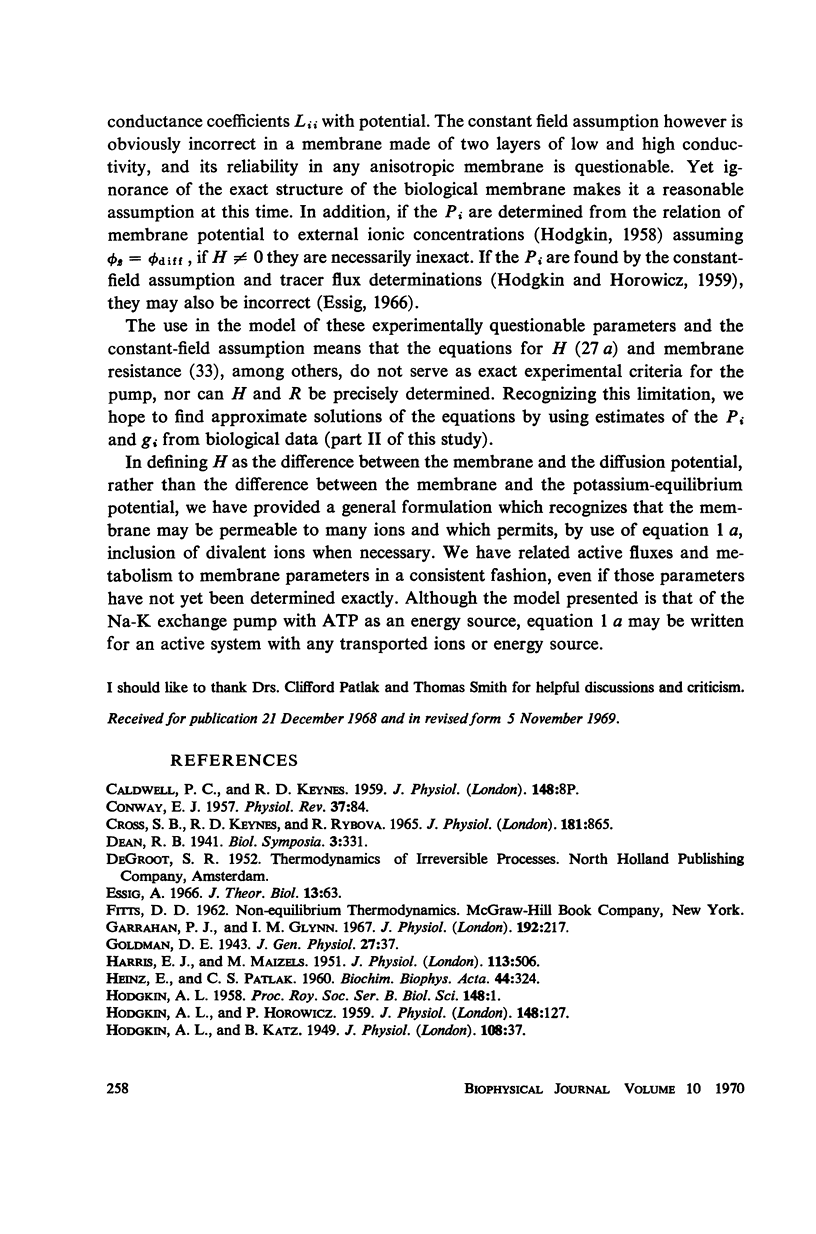
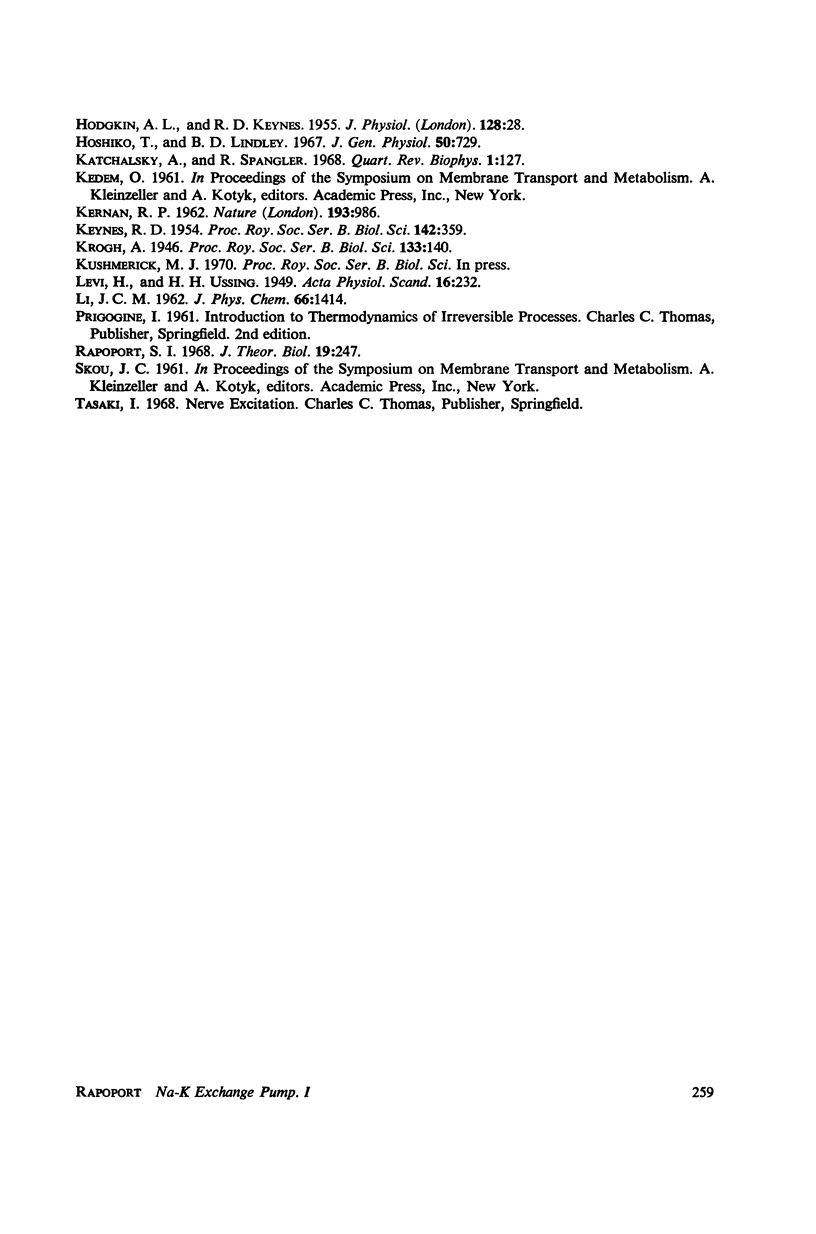
Images in this article
Selected References
These references are in PubMed. This may not be the complete list of references from this article.
- Cross S. B., Keynes R. D., Rybová R. The coupling of sodium efflux and potassium influx in frog muscle. J Physiol. 1965 Dec;181(4):865–880. doi: 10.1113/jphysiol.1965.sp007802. [DOI] [PMC free article] [PubMed] [Google Scholar]
- Garrahan P. J., Glynn I. M. The stoicheiometry of the sodium pump. J Physiol. 1967 Sep;192(1):217–235. doi: 10.1113/jphysiol.1967.sp008297. [DOI] [PMC free article] [PubMed] [Google Scholar]
- Goldman D. E. POTENTIAL, IMPEDANCE, AND RECTIFICATION IN MEMBRANES. J Gen Physiol. 1943 Sep 20;27(1):37–60. doi: 10.1085/jgp.27.1.37. [DOI] [PMC free article] [PubMed] [Google Scholar]
- HEINZ E., PATLAK C. S. Energy expenditure by active transport mechanisms. Biochim Biophys Acta. 1960 Nov 4;44:324–334. doi: 10.1016/0006-3002(60)91568-7. [DOI] [PubMed] [Google Scholar]
- HODGKIN A. L., HOROWICZ P. The influence of potassium and chloride ions on the membrane potential of single muscle fibres. J Physiol. 1959 Oct;148:127–160. doi: 10.1113/jphysiol.1959.sp006278. [DOI] [PMC free article] [PubMed] [Google Scholar]
- Hoshiko T., Lindley B. D. Phenomenological description of active transport of salt and water. J Gen Physiol. 1967 Jan;50(3):729–758. doi: 10.1085/jgp.50.3.729. [DOI] [PMC free article] [PubMed] [Google Scholar]
- KERNAN R. P. Membrane potential changes during sodium transport in frog sartorius muscle. Nature. 1962 Mar 10;193:986–987. doi: 10.1038/193986a0. [DOI] [PubMed] [Google Scholar]
- KEYNES R. D. The ionic fluxes in frog muscle. Proc R Soc Lond B Biol Sci. 1954 May 27;142(908):359–382. doi: 10.1098/rspb.1954.0030. [DOI] [PubMed] [Google Scholar]
- Katchalsky A., Spangler R. Dynamics of membrane processes. Q Rev Biophys. 1968 Jun;1(2):127–175. doi: 10.1017/s0033583500000524. [DOI] [PubMed] [Google Scholar]