Abstract
The high resolution proton magnetic resonance spectrum of the sciatic nerve of the frog was studied in both the polarized and depolarized states. Paramagnetic salts were introduced into the system in order to separate the signals from the intra- and extracellular environments. It was determined that about 65% of the proton signal from the nerve trunk was accounted for by the intracellular environment in the polarized nerve trunk and that this percentage decreased to about 34% in the depolarized case.
The temperature dependence of the line widths of the intracellular proton signal was studied. The enthalpy and entropy of activation for proton exchange between the intra- and extracellular environments were found to be 11.1 kcal/mole and -17.1 cal/deg-mole respectively. The pseudo-first-order rate constant for proton exchange between the intra- and extracellular environments was determined at 20°C and shown to agree with the measured permeability coefficients of similar cells.
Data are presented which indicate that the pseudo-first order rate constant for proton exchange between the two environments decreases upon depolarization of the nerve trunk and that the proton spin-spin relaxation time of the protons of intracellular water decreases significantly with depolarization.
These results indicate a possibly quite important role of water in neural phenomena.
Full text
PDF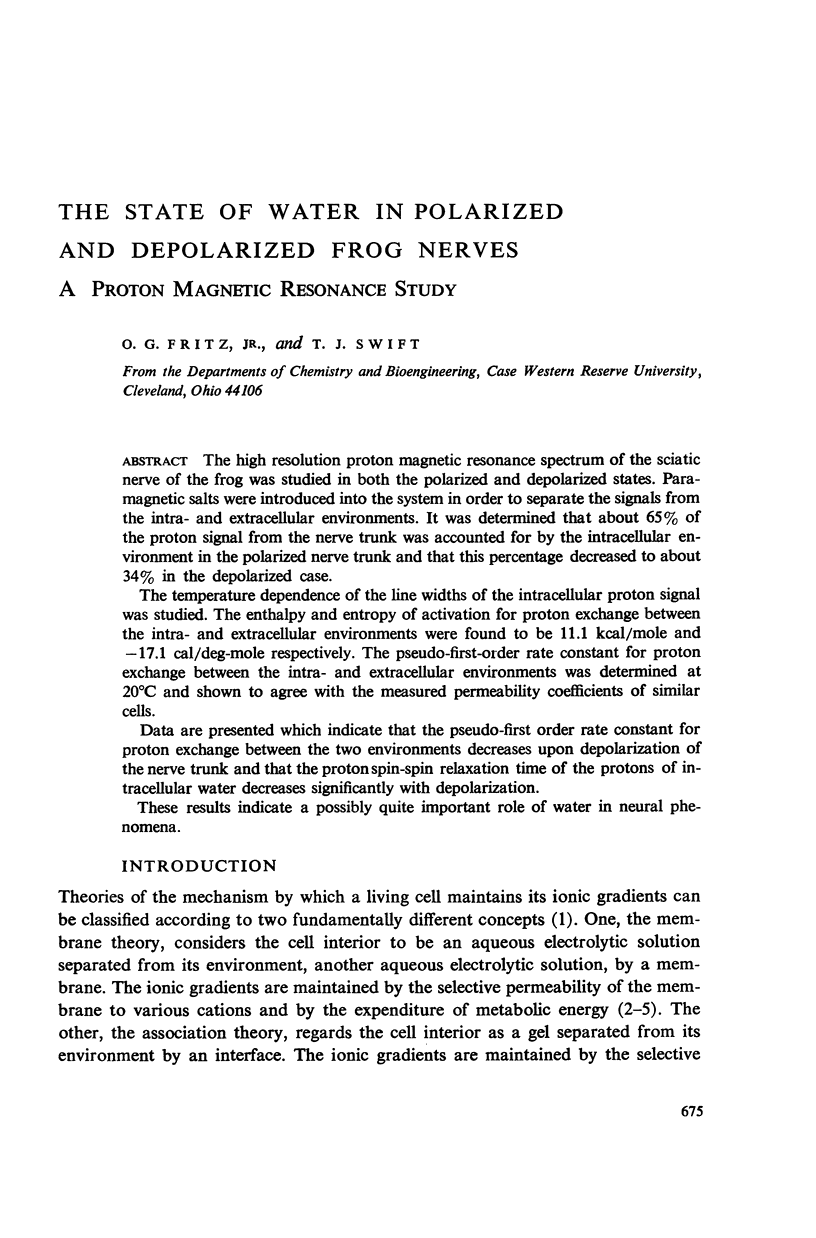
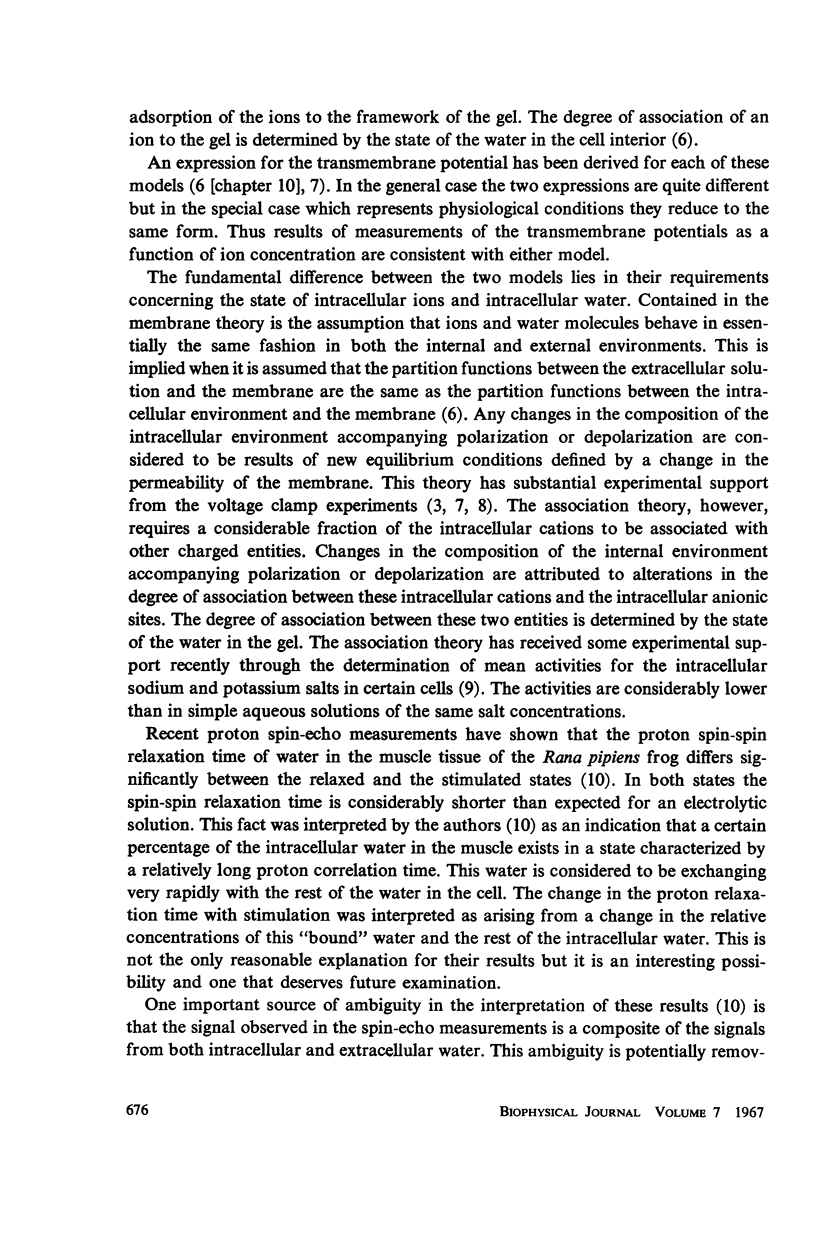
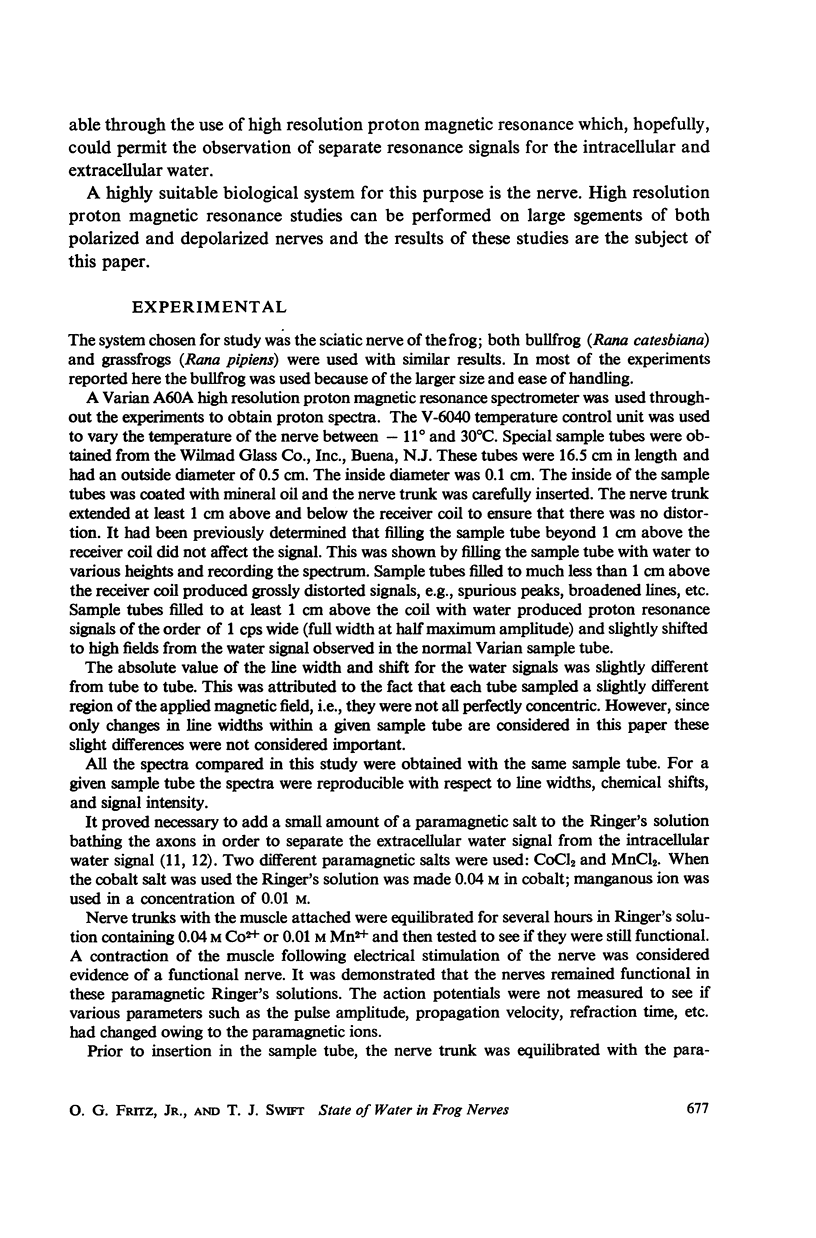
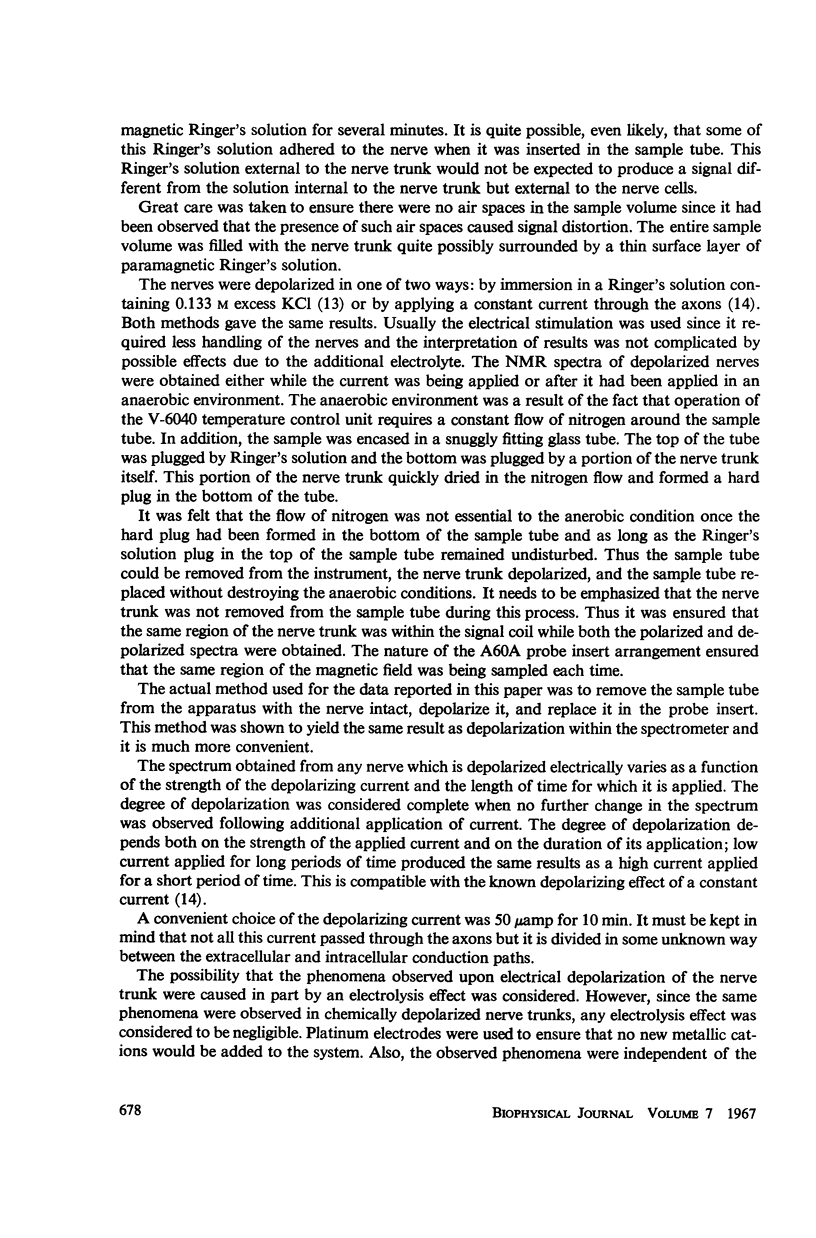
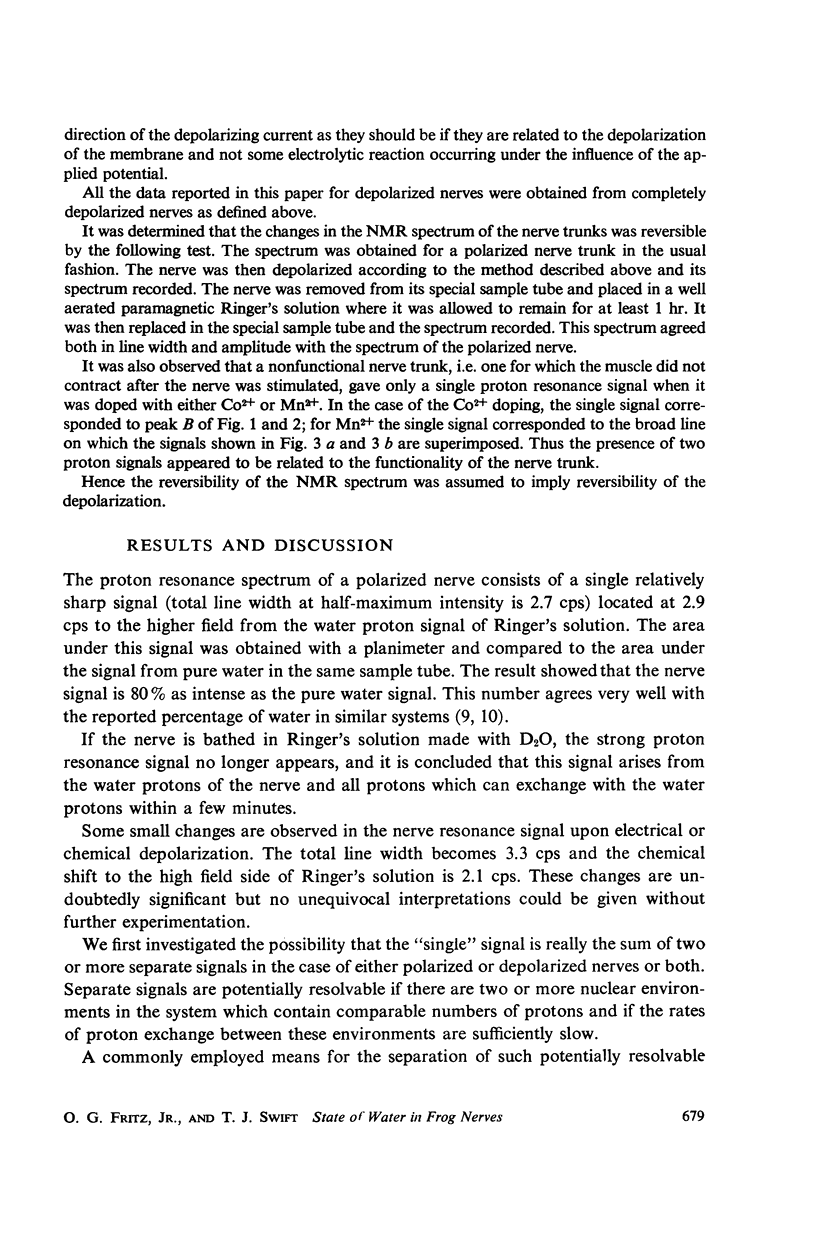
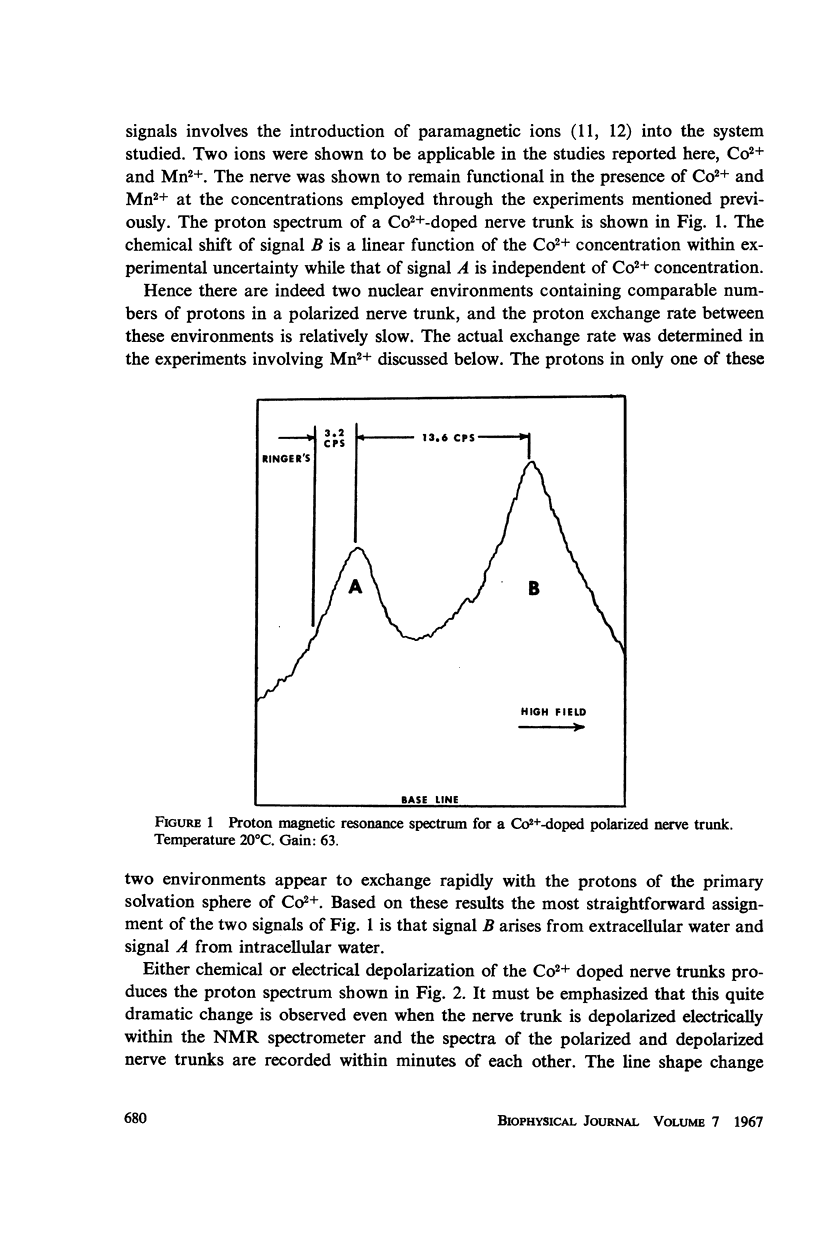
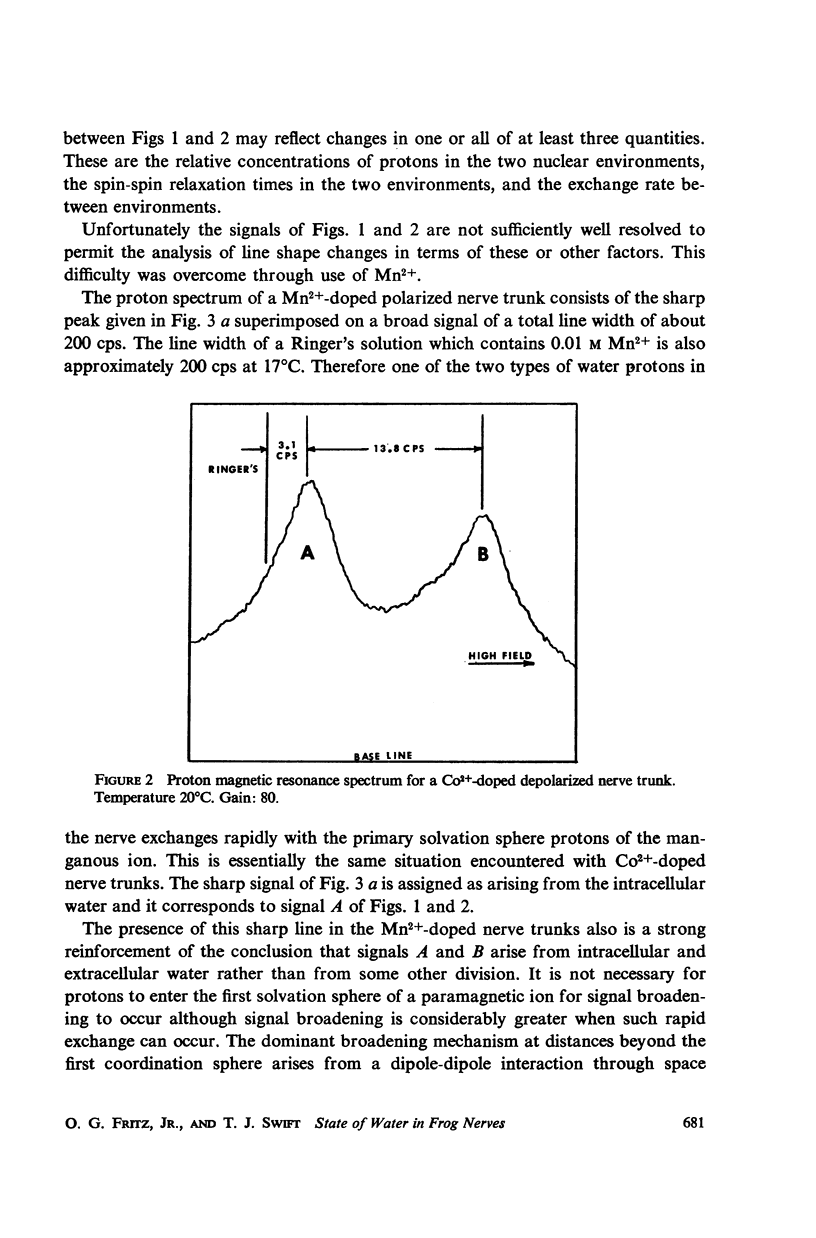
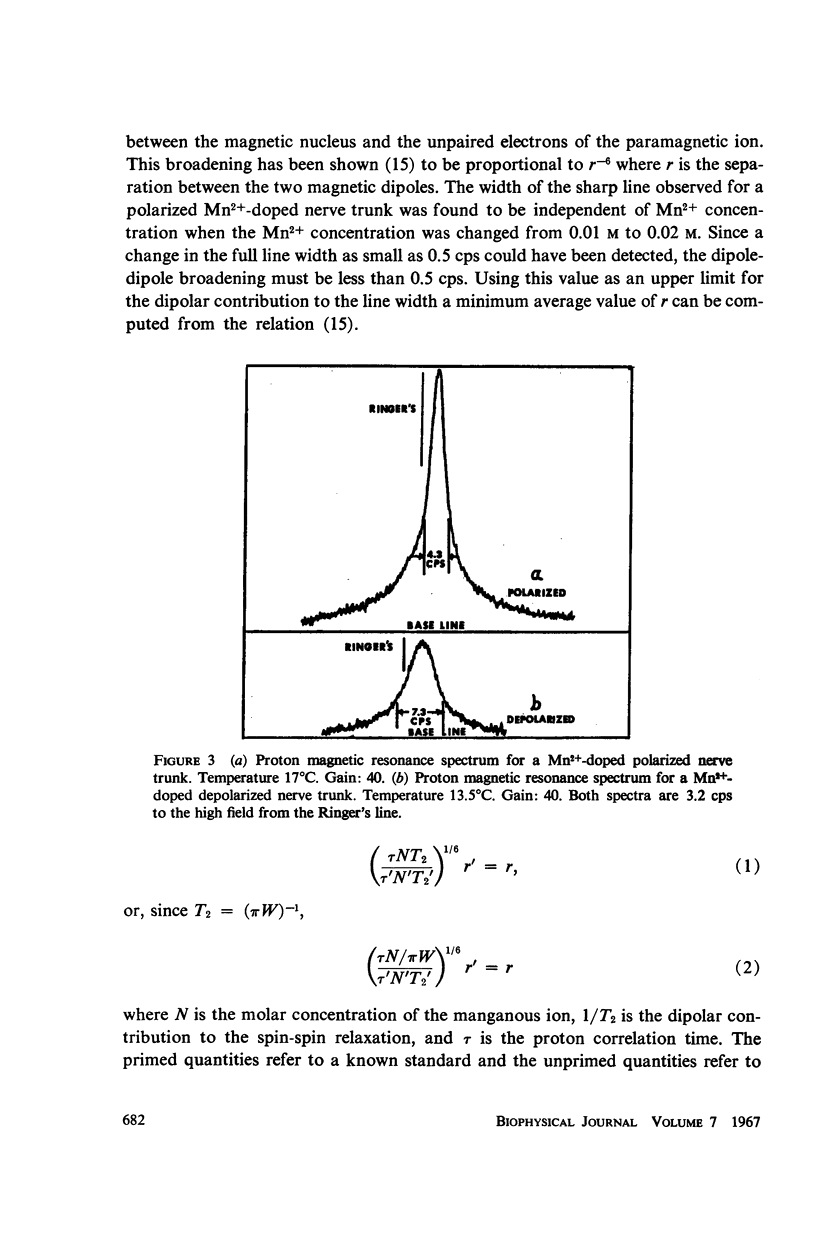
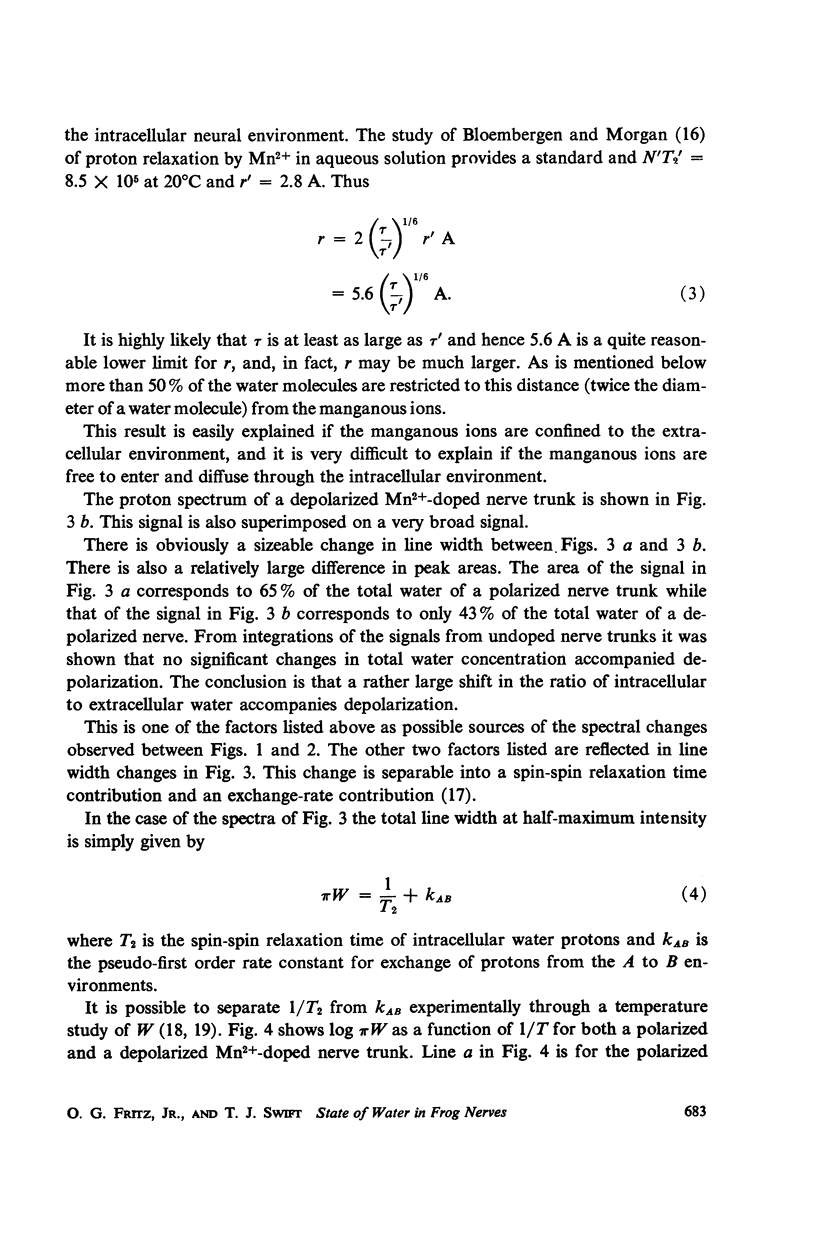
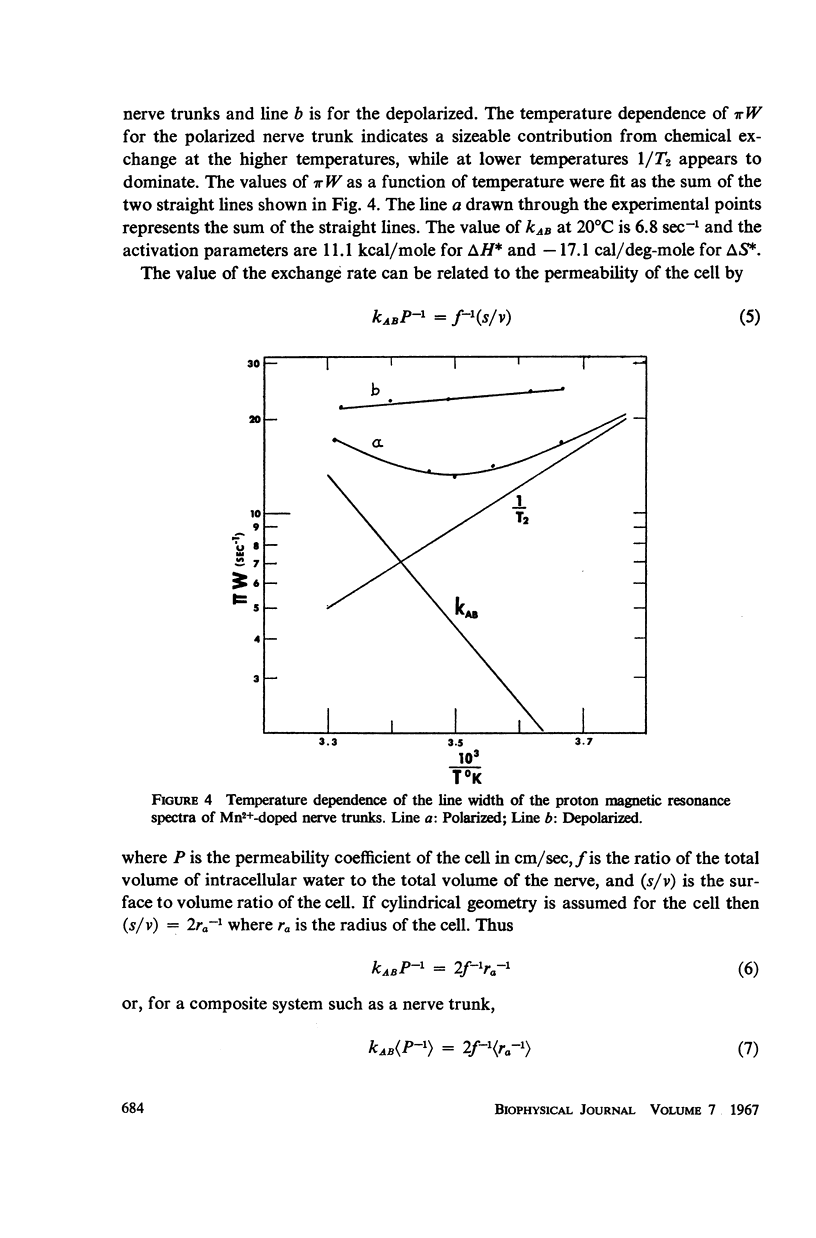
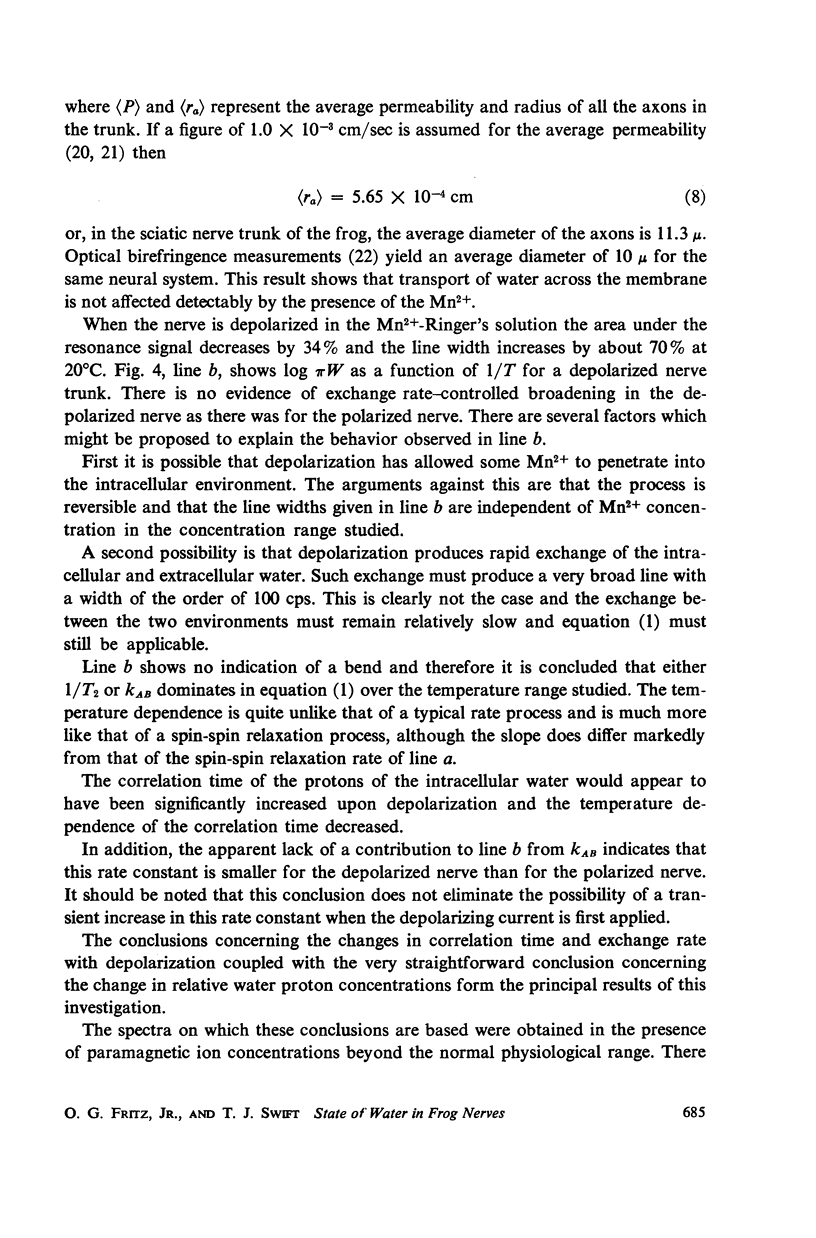
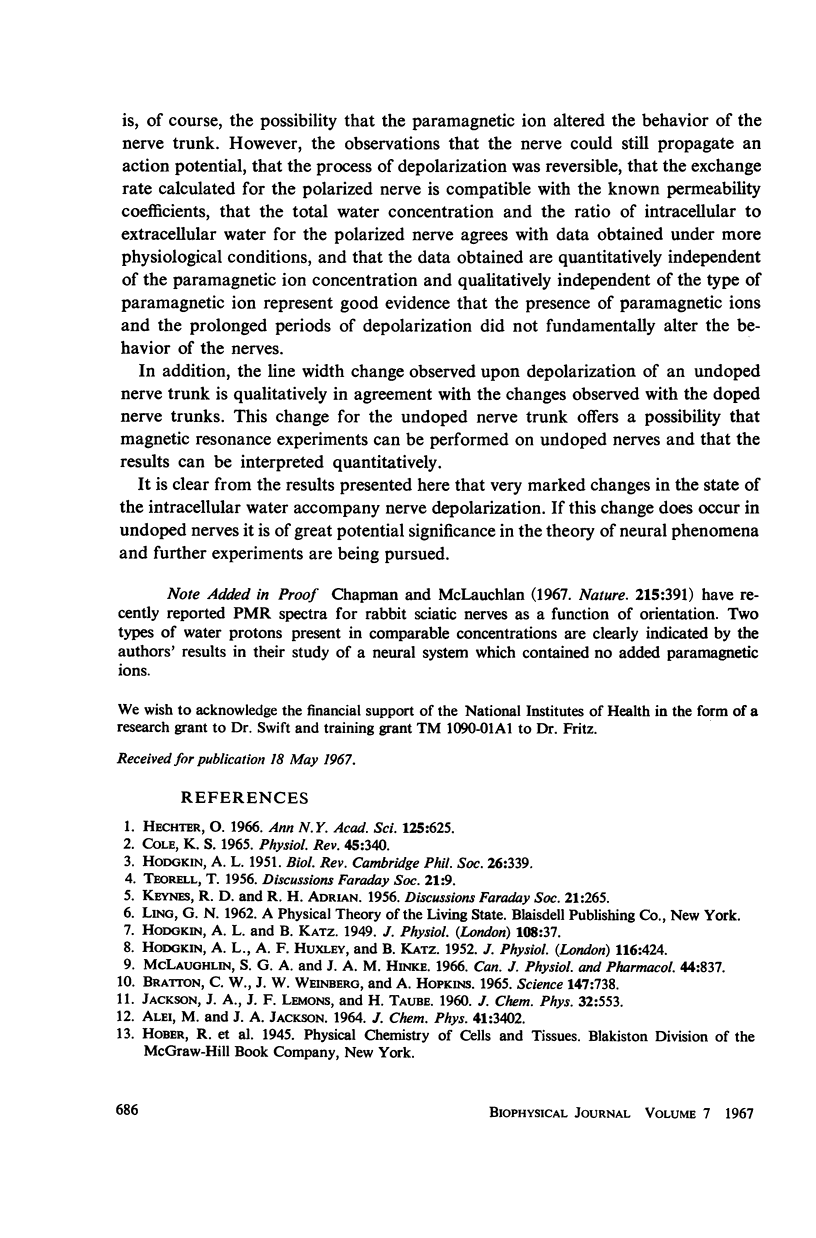
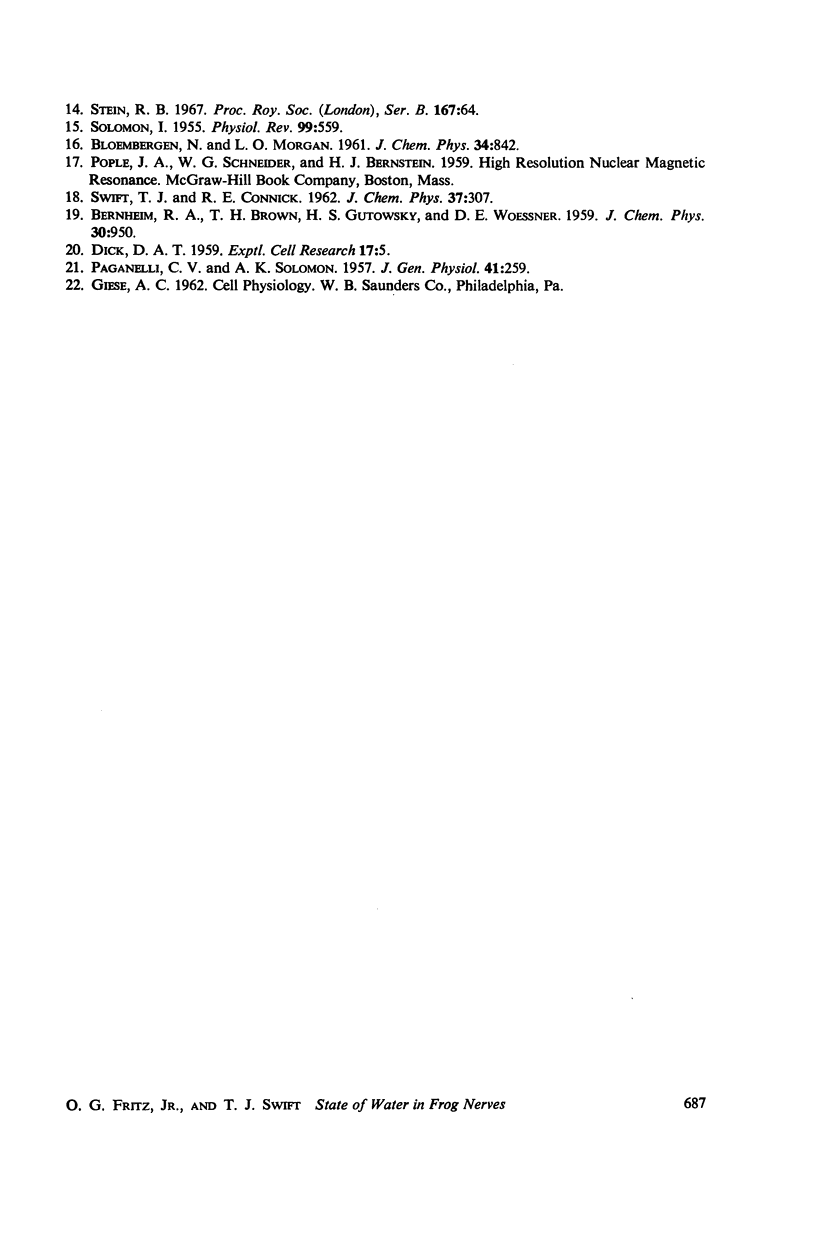
Selected References
These references are in PubMed. This may not be the complete list of references from this article.
- BRATTON C. B., HOPKINS A. L., WEINBERG J. W. NUCLEAR MAGNETIC RESONANCE STUDIES OF LIVING MUSCLE. Science. 1965 Feb 12;147(3659):738–739. doi: 10.1126/science.147.3659.738. [DOI] [PubMed] [Google Scholar]
- COLE K. S. ELECTRODIFFUSION MODELS FOR THE MEMBRANE OF SQUID GIANT AXON. Physiol Rev. 1965 Apr;45:340–379. doi: 10.1152/physrev.1965.45.2.340. [DOI] [PubMed] [Google Scholar]
- Chapman G., McLauchlan K. A. Oriented water in the sciatic nerve of rabbit. Nature. 1967 Jul 22;215(5099):391–392. doi: 10.1038/215391a0. [DOI] [PubMed] [Google Scholar]
- DICK D. A. The rate of diffusion of water in the protoplasm of living cells. Exp Cell Res. 1959 Apr;17(1):5–12. doi: 10.1016/0014-4827(59)90146-6. [DOI] [PubMed] [Google Scholar]
- HODGKIN A. L., HUXLEY A. F., KATZ B. Measurement of current-voltage relations in the membrane of the giant axon of Loligo. J Physiol. 1952 Apr;116(4):424–448. doi: 10.1113/jphysiol.1952.sp004716. [DOI] [PMC free article] [PubMed] [Google Scholar]
- HODGKIN A. L., KATZ B. The effect of sodium ions on the electrical activity of giant axon of the squid. J Physiol. 1949 Mar 1;108(1):37–77. doi: 10.1113/jphysiol.1949.sp004310. [DOI] [PMC free article] [PubMed] [Google Scholar]
- Hechter O. Intracellular water structure and mechanisms of cellular transport. Ann N Y Acad Sci. 1965 Oct 13;125(2):625–646. doi: 10.1111/j.1749-6632.1965.tb45418.x. [DOI] [PubMed] [Google Scholar]
- McLaughlin S. G., Hinke J. A. Sodium and water binding in single striated muscle fibers of the giant barnacle. Can J Physiol Pharmacol. 1966 Sep;44(5):837–848. doi: 10.1139/y66-102. [DOI] [PubMed] [Google Scholar]
- PAGANELLI C. V., SOLOMON A. K. The rate of exchange of tritiated water across the human red cell membrane. J Gen Physiol. 1957 Nov 20;41(2):259–277. doi: 10.1085/jgp.41.2.259. [DOI] [PMC free article] [PubMed] [Google Scholar]