Abstract
Radial stiffness in various conditions of mechanically skinned fibers of semitendinosus muscle of Rana catesbeiana was determined by compressing the fiber with polyvinylpyrrolidone (PVP K-30, Mr = 40,000) in incubating solution. The change in width (D) of fibers with increasing and decreasing PVP concentrations was highly reproducible at a range 0-6% PVP. Radial stiffness of relaxed fibers was almost independent of the sarcomere length. On the other hand, radial stiffness of rigor fibers showed a linear relation against the sarcomere length. These results indicate that cross-bridge attachment would be a major factor in the increase of the radial stiffness. Radial stiffness of relaxed and rigor fibers was (2.14 +/- 0.52) X 10(4) N/m2 (mean +/- SD) and (8.76 +/- 2.04) X 10(4) N/m2, respectively, at the relative fiber width (D/D0) of 0.92, where D0 denotes the fiber width in the rigor solution at 0% PVP. Radial stiffness of a fiber in a rigor solution containing pyrophosphate (PPi) was between those of relaxed and rigor fibers, i.e., (4.76 +/- 0.86) X 10(4) N/m2 at D/Do of 0.92. In PPi and rigor solutions, radial stiffness reversibly increased to around 150 and 130%, respectively, in the presence of 10(-6) M Ca2+. To explain these results, especially the Ca2+-induced change in the radial stiffness, some factor in addition to the number of attached cross-bridges has to be taken into account. The variation of radial stiffness under various conditions will be discussed in relation to the possible manner of cross-bridge attachment.
Full text
PDF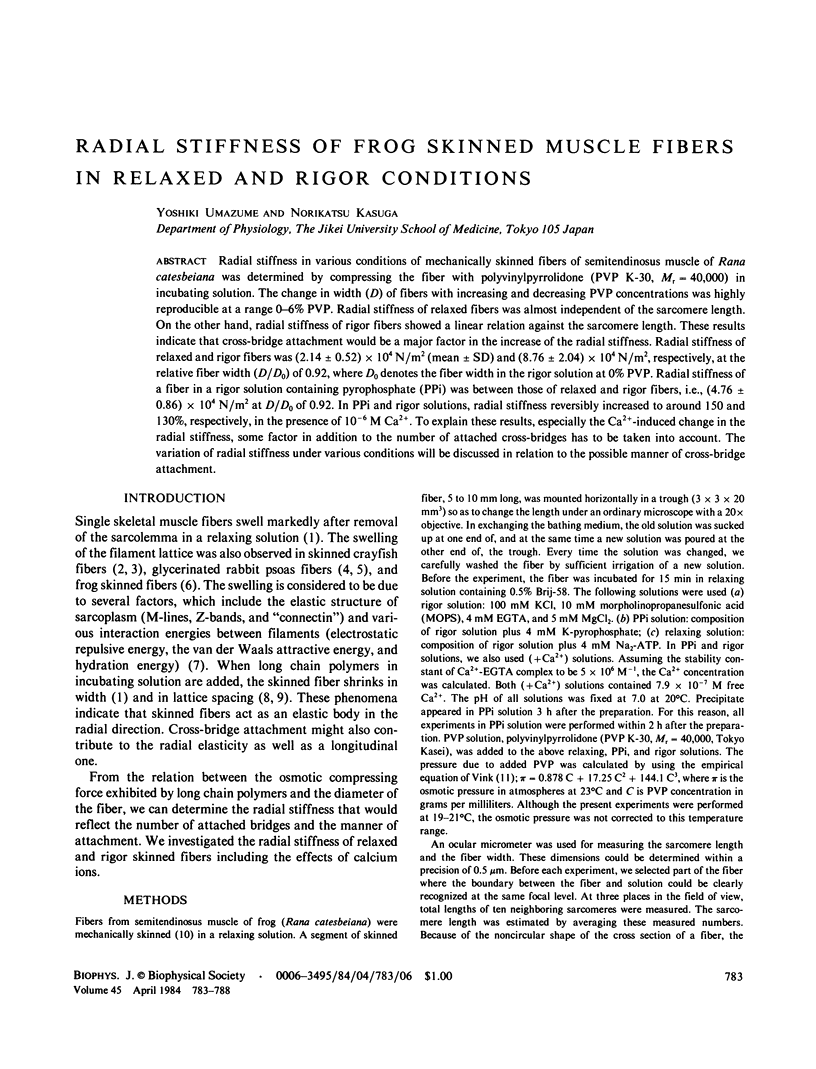
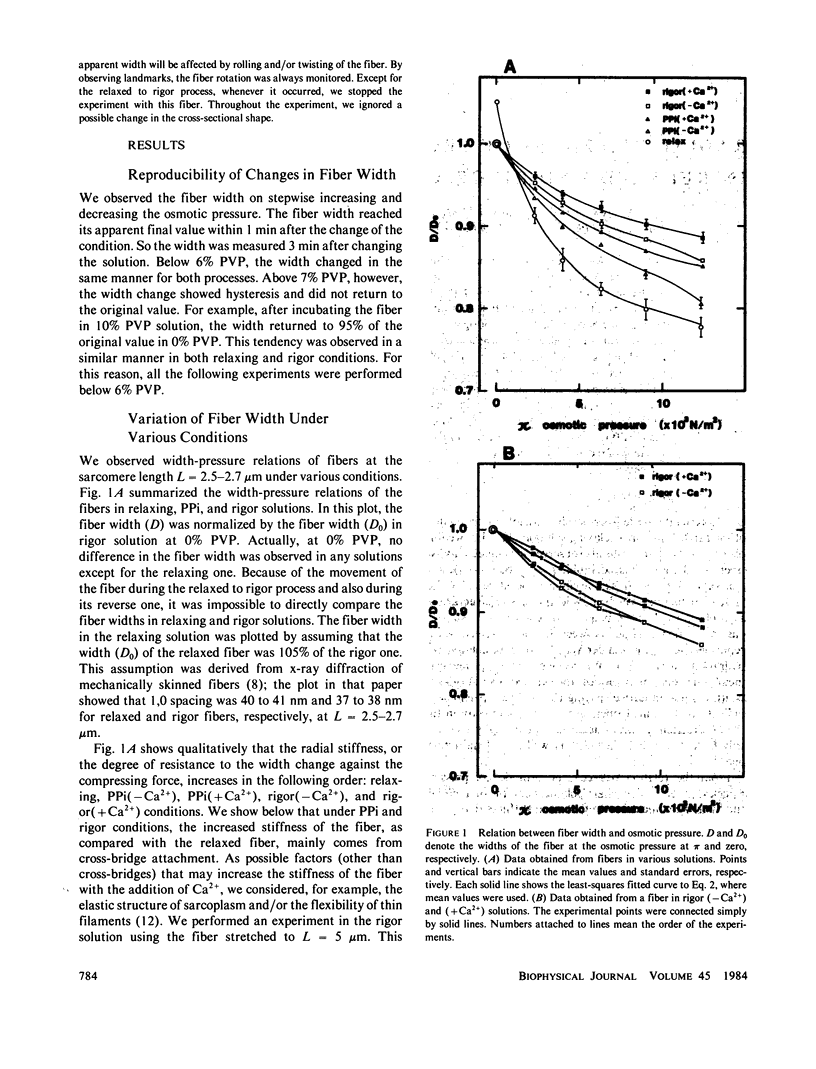
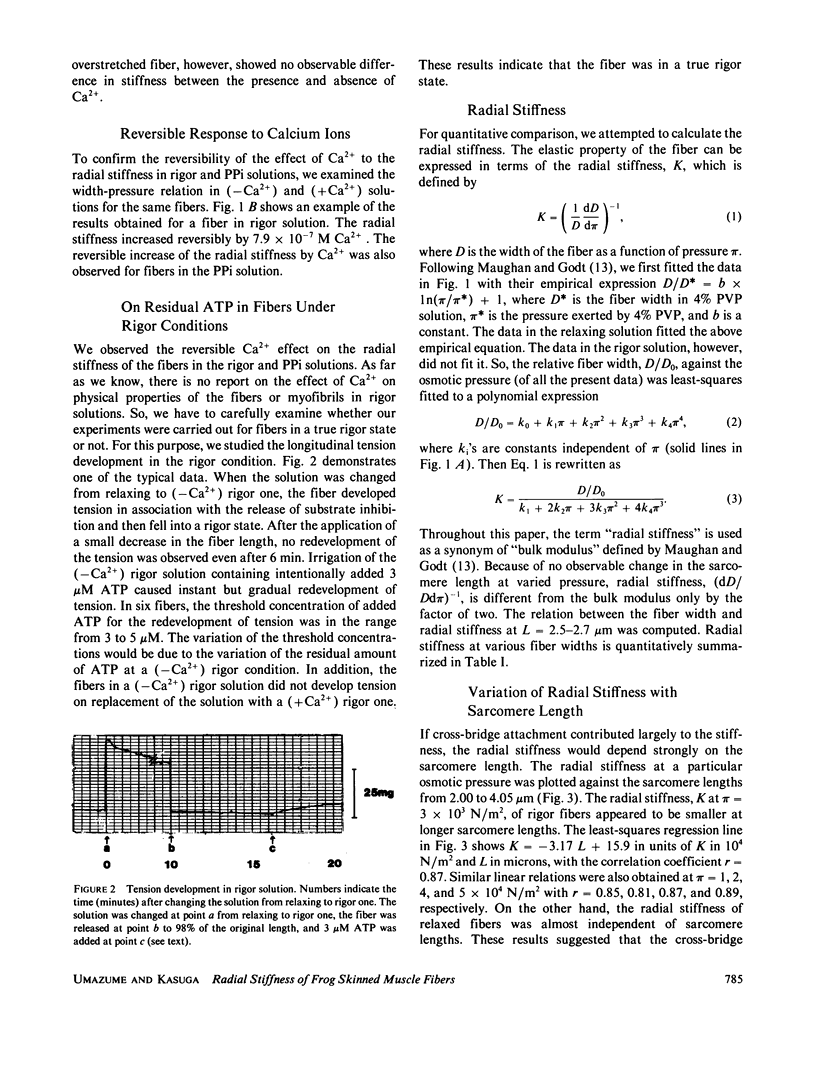
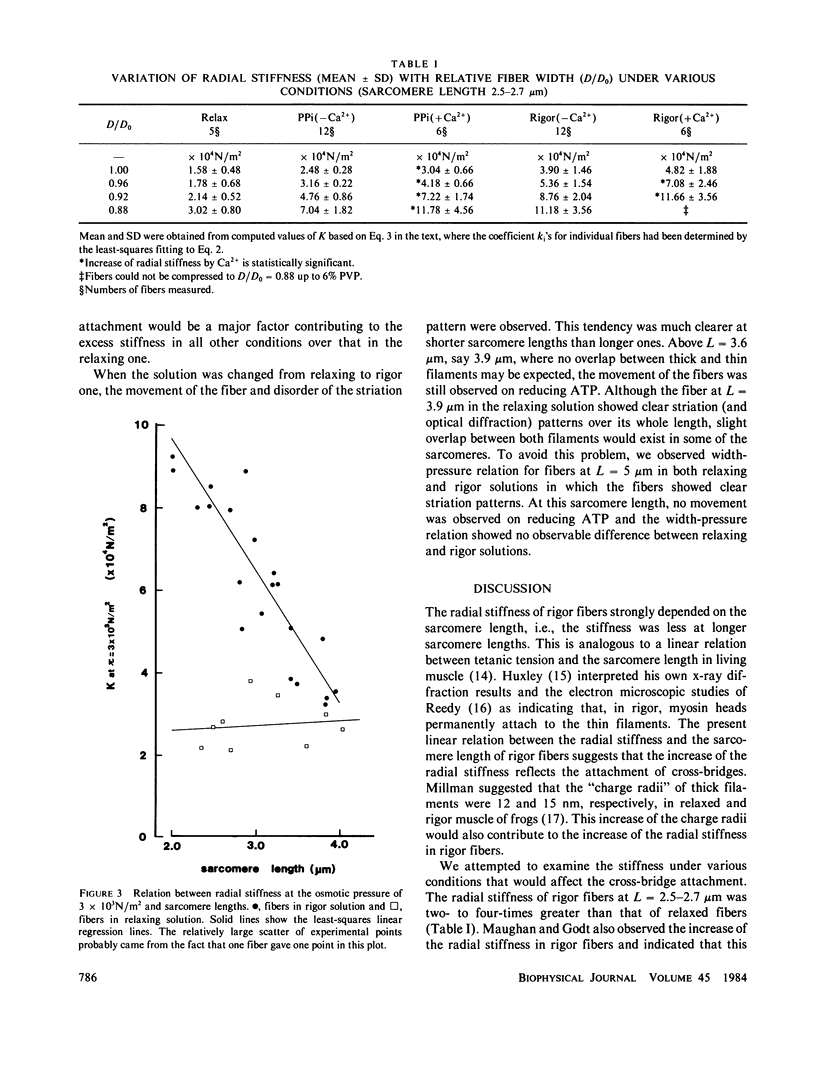
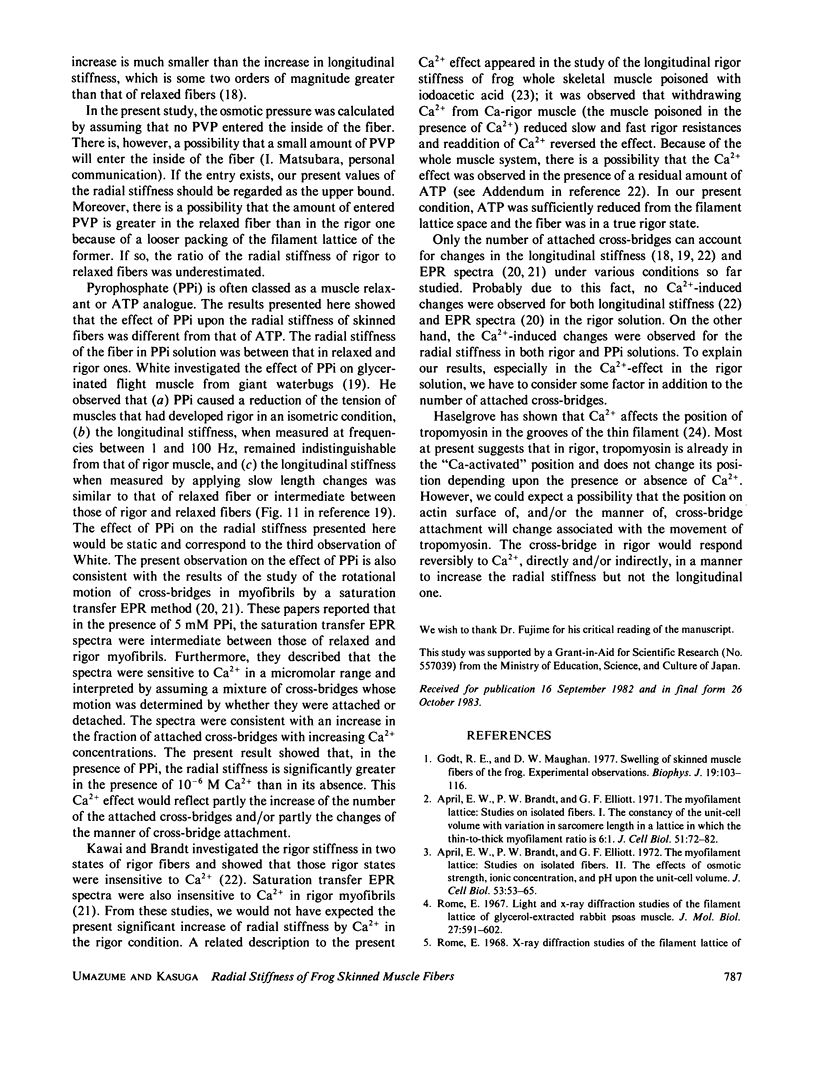
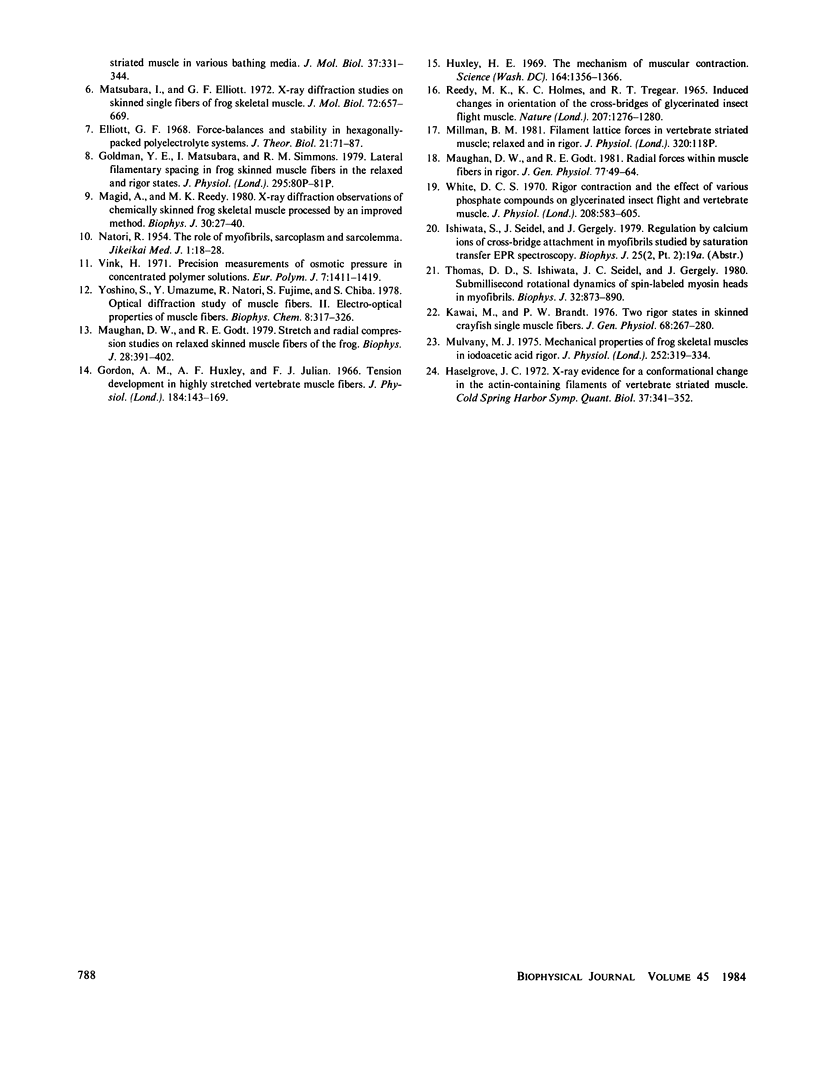
Selected References
These references are in PubMed. This may not be the complete list of references from this article.
- April E. W., Brandt P. W., Elliott G. F. The myofilament lattice: studies on isolated fibers. I. The constancy of the unit-cell volume with variation in sarcomere length in a lattice in which the thin-to-thick myofilament ratio is 6:1. J Cell Biol. 1971 Oct;51(1):72–82. doi: 10.1083/jcb.51.1.72. [DOI] [PMC free article] [PubMed] [Google Scholar]
- April E. W., Brandt P. W., Elliott G. F. The myofilament lattice: studies on isolated fibers. II. The effects of osmotic strength, ionic concentration, and pH upon the unit-cell volume. J Cell Biol. 1972 Apr;53(1):53–65. doi: 10.1083/jcb.53.1.53. [DOI] [PMC free article] [PubMed] [Google Scholar]
- Elliott G. F. Force-balances and stability in hexagonally-packed polyelectrolyte systems. J Theor Biol. 1968 Oct;21(1):71–87. doi: 10.1016/0022-5193(68)90060-x. [DOI] [PubMed] [Google Scholar]
- Godt R. E., Maughan D. W. Swelling of skinned muscle fibers of the frog. Experimental observations. Biophys J. 1977 Aug;19(2):103–116. doi: 10.1016/S0006-3495(77)85573-2. [DOI] [PMC free article] [PubMed] [Google Scholar]
- Gordon A. M., Huxley A. F., Julian F. J. Tension development in highly stretched vertebrate muscle fibres. J Physiol. 1966 May;184(1):143–169. doi: 10.1113/jphysiol.1966.sp007908. [DOI] [PMC free article] [PubMed] [Google Scholar]
- Huxley H. E. The mechanism of muscular contraction. Science. 1969 Jun 20;164(3886):1356–1365. doi: 10.1126/science.164.3886.1356. [DOI] [PubMed] [Google Scholar]
- Kawai M., Brandt P. W. Two rigor states in skinned crayfish single muscle fibers. J Gen Physiol. 1976 Sep;68(3):267–280. doi: 10.1085/jgp.68.3.267. [DOI] [PMC free article] [PubMed] [Google Scholar]
- Magid A., Reedy M. K. X-ray diffraction observations of chemically skinned frog skeletal muscle processed by an improved method. Biophys J. 1980 Apr;30(1):27–40. doi: 10.1016/S0006-3495(80)85074-0. [DOI] [PMC free article] [PubMed] [Google Scholar]
- Matsubara I., Elliott G. F. X-ray diffraction studies on skinned single fibres of frog skeletal muscle. J Mol Biol. 1972 Dec 30;72(3):657–669. doi: 10.1016/0022-2836(72)90183-0. [DOI] [PubMed] [Google Scholar]
- Maughan D. W., Godt R. E. Radial forces within muscle fibers in rigor. J Gen Physiol. 1981 Jan;77(1):49–64. doi: 10.1085/jgp.77.1.49. [DOI] [PMC free article] [PubMed] [Google Scholar]
- Maughan D. W., Godt R. E. Stretch and radial compression studies on relaxed skinned muscle fibers of the frog. Biophys J. 1979 Dec;28(3):391–402. doi: 10.1016/S0006-3495(79)85188-7. [DOI] [PMC free article] [PubMed] [Google Scholar]
- Mulvany M. J. Mechanical properties of frog skeletal muscles in iodoacetic acid rigor. J Physiol. 1975 Nov;252(2):319–334. doi: 10.1113/jphysiol.1975.sp011146. [DOI] [PMC free article] [PubMed] [Google Scholar]
- Reedy M. K., Holmes K. C., Tregear R. T. Induced changes in orientation of the cross-bridges of glycerinated insect flight muscle. Nature. 1965 Sep 18;207(5003):1276–1280. doi: 10.1038/2071276a0. [DOI] [PubMed] [Google Scholar]
- Rome E. Light and X-ray diffraction studies of the filament lattice of glycerol-extracted rabbit psoas muscle. J Mol Biol. 1967 Aug 14;27(3):591–602. doi: 10.1016/0022-2836(67)90061-7. [DOI] [PubMed] [Google Scholar]
- Rome E. X-ray diffraction studies of the filament lattice of striated muscle in various bathing media. J Mol Biol. 1968 Oct 28;37(2):331–344. doi: 10.1016/0022-2836(68)90272-6. [DOI] [PubMed] [Google Scholar]
- Thomas D. D., Ishiwata S., Seidel J. C., Gergely J. Submillisecond rotational dynamics of spin-labeled myosin heads in myofibrils. Biophys J. 1980 Dec;32(3):873–889. doi: 10.1016/S0006-3495(80)85023-5. [DOI] [PMC free article] [PubMed] [Google Scholar]
- White D. C. Rigor contraction and the effect of various phosphate compounds on glycerinated insect flight and vertebrate muscle. J Physiol. 1970 Jul;208(3):583–605. doi: 10.1113/jphysiol.1970.sp009138. [DOI] [PMC free article] [PubMed] [Google Scholar]
- Yoshino S., Umazume Y., Natori R., Fujime S., Chiba S. Optical diffraction study of muscle fibers. II. Electro-optical properties of muscle fibers. Biophys Chem. 1978 Sep;8(4):317–326. doi: 10.1016/0301-4622(78)80014-3. [DOI] [PubMed] [Google Scholar]