Abstract
Recently it has become possible to measure fluorescence phase-shift and modulation data over a wide range of modulation frequencies. In this paper we describe the analysis of these data by the method of nonlinear least squares to determine the values of the lifetimes and fractional intensities for a mixture of exponentially decaying fluorophores. Analyzing simulated data allowed us to determine those experimental factors that are most critical for successfully resolving the emissions from mixtures of fluorophores. The most critical factors are the accuracy of the experimental data, the relative difference of the individual decay times, and the inclusion of data measured at multiple emission wavelengths. After measuring at eight widely spaced modulation frequencies, additional measurements yielded only a modest increase in resolution. In particular, the uncertainty in the parameters decreased approximately as the reciprocal of the square root of the number of modulation frequencies. Our simulations showed that with presently available precision and data for one emission bandpass, two decay times could be accurately determined if their ratio were greater than or equal to 1.4. Three exponential decays could also be resolved, but only if the range of the lifetimes were fivefold or greater. To reliably determine closely-spaced decay times, the data were measured at multiple emission wavelengths so that the fractional intensities of the components could be varied. Also, independent knowledge of any of the parameters substantially increased the accuracy with which the remaining parameters could be determined. In the subsequent paper we present experimental results that broadly confirm the predicted resolving potential of variable-frequency phase-modulation fluorometry.
Full text
PDF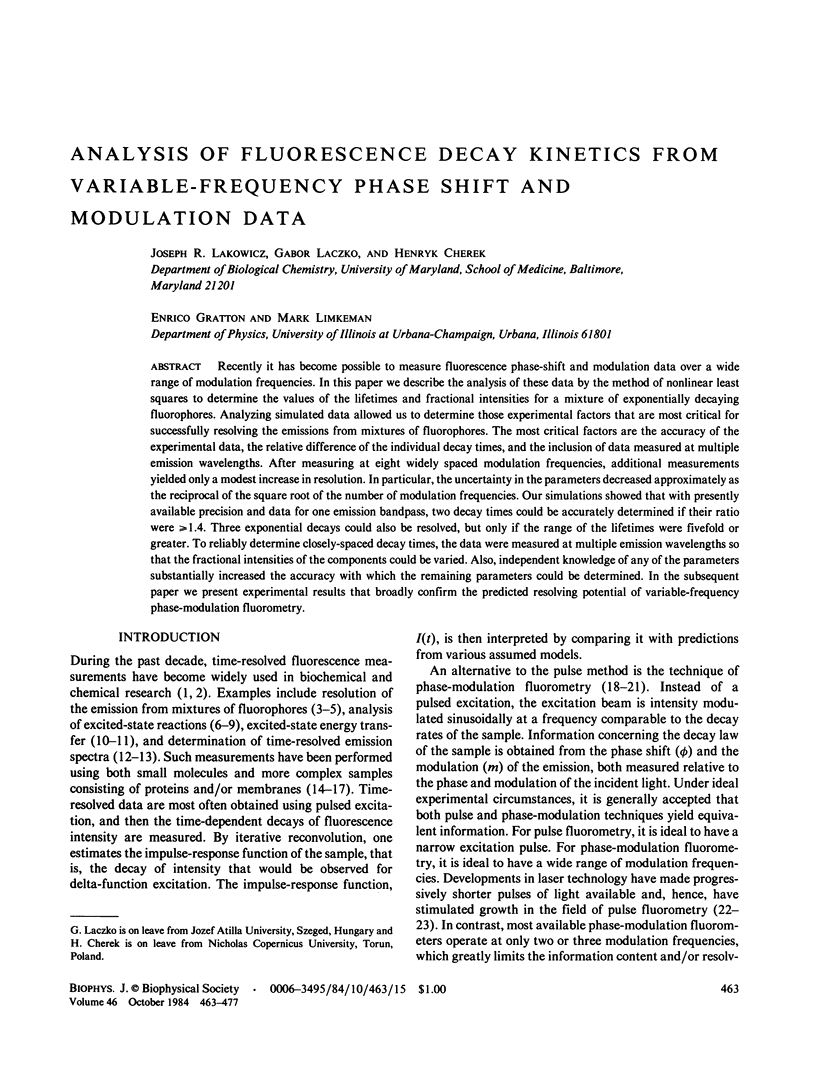
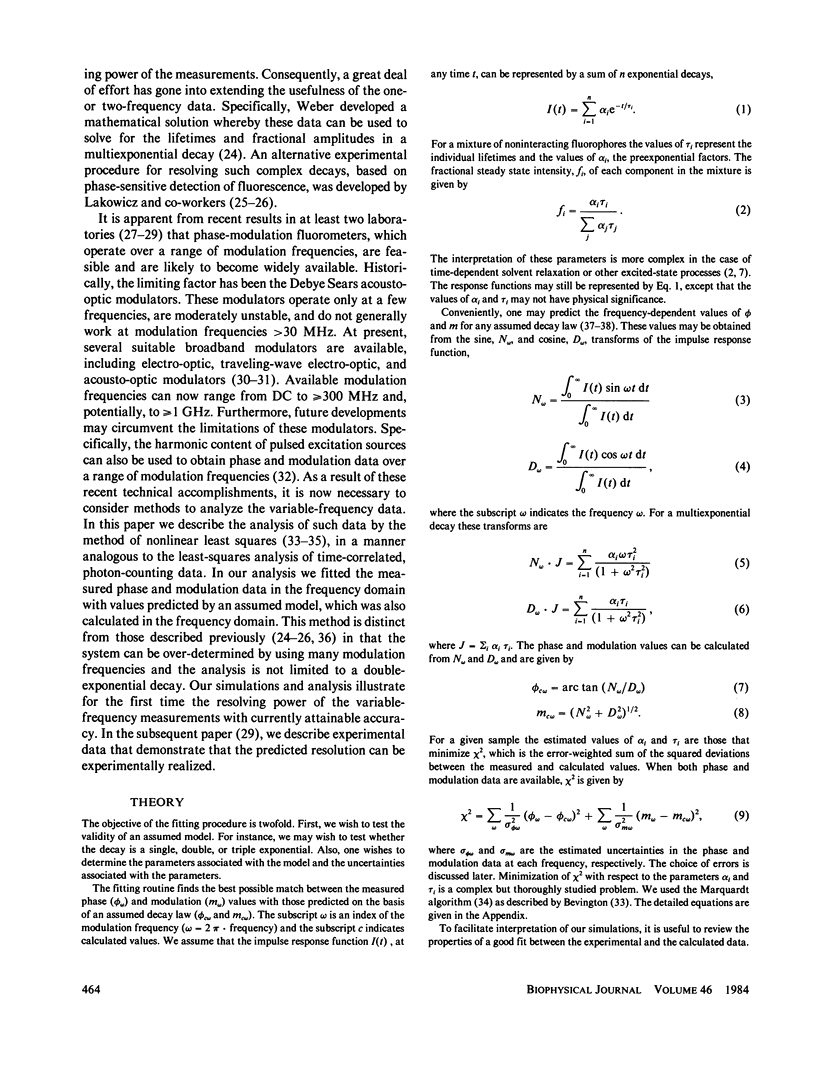
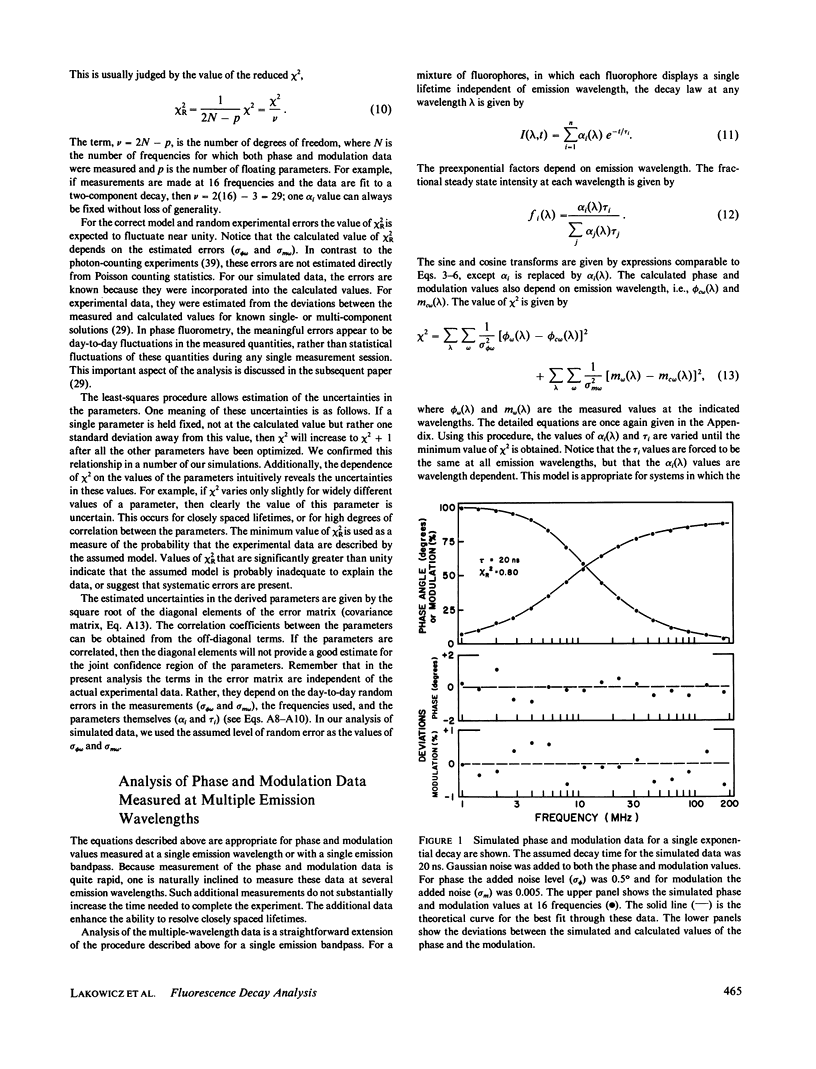
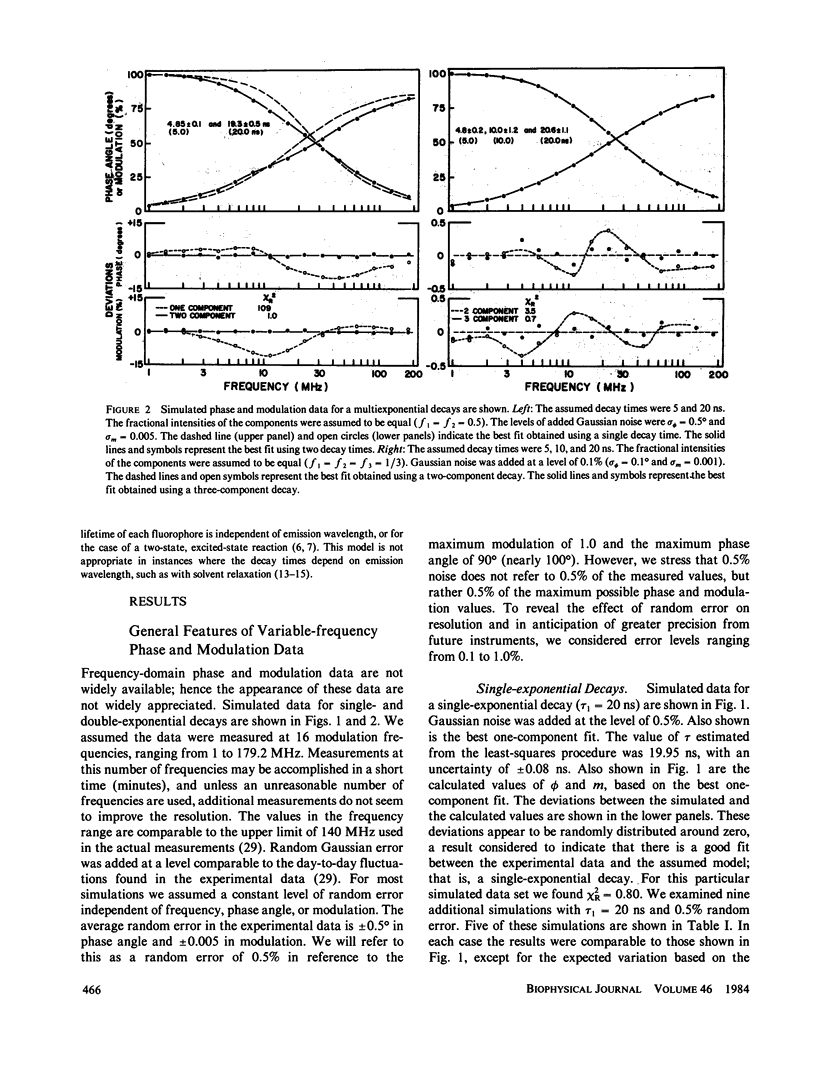
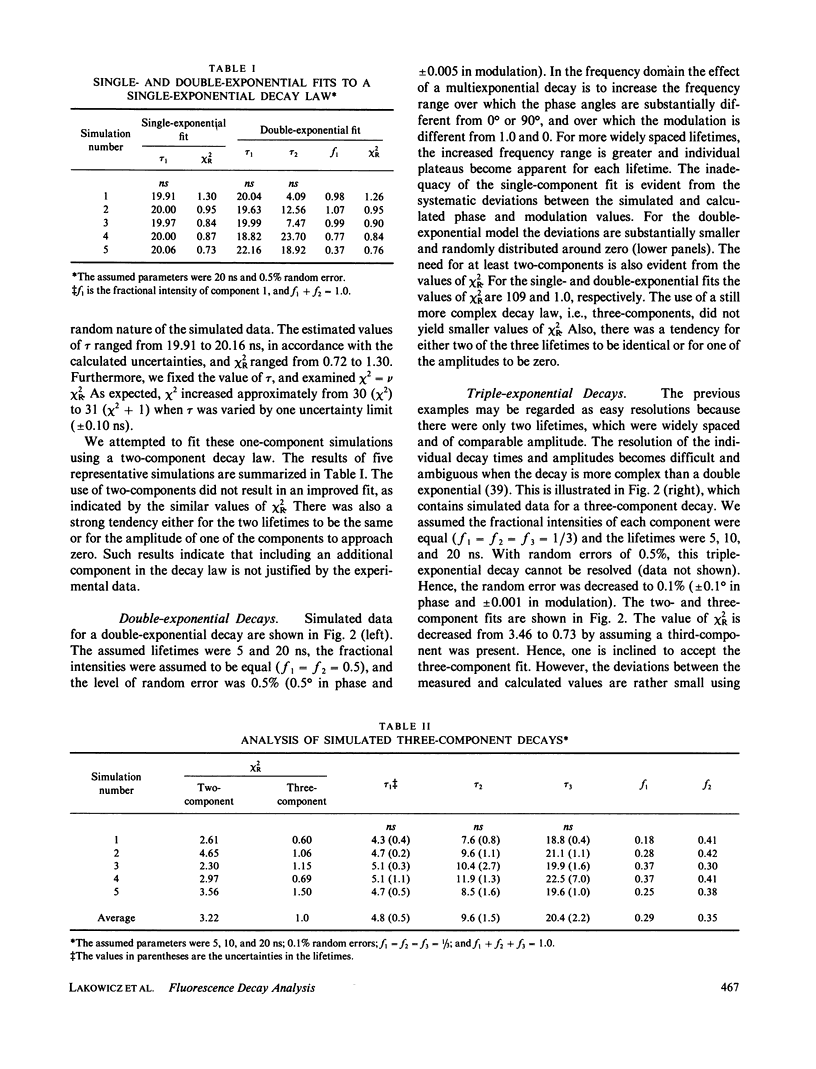
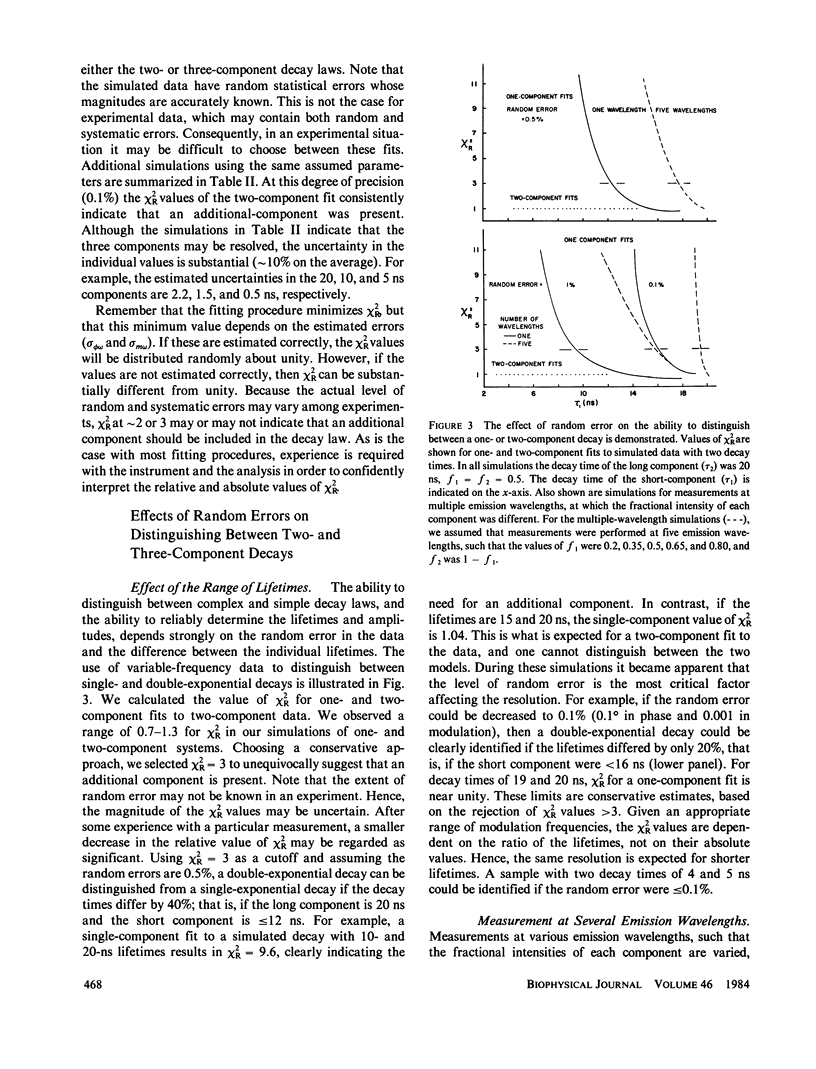
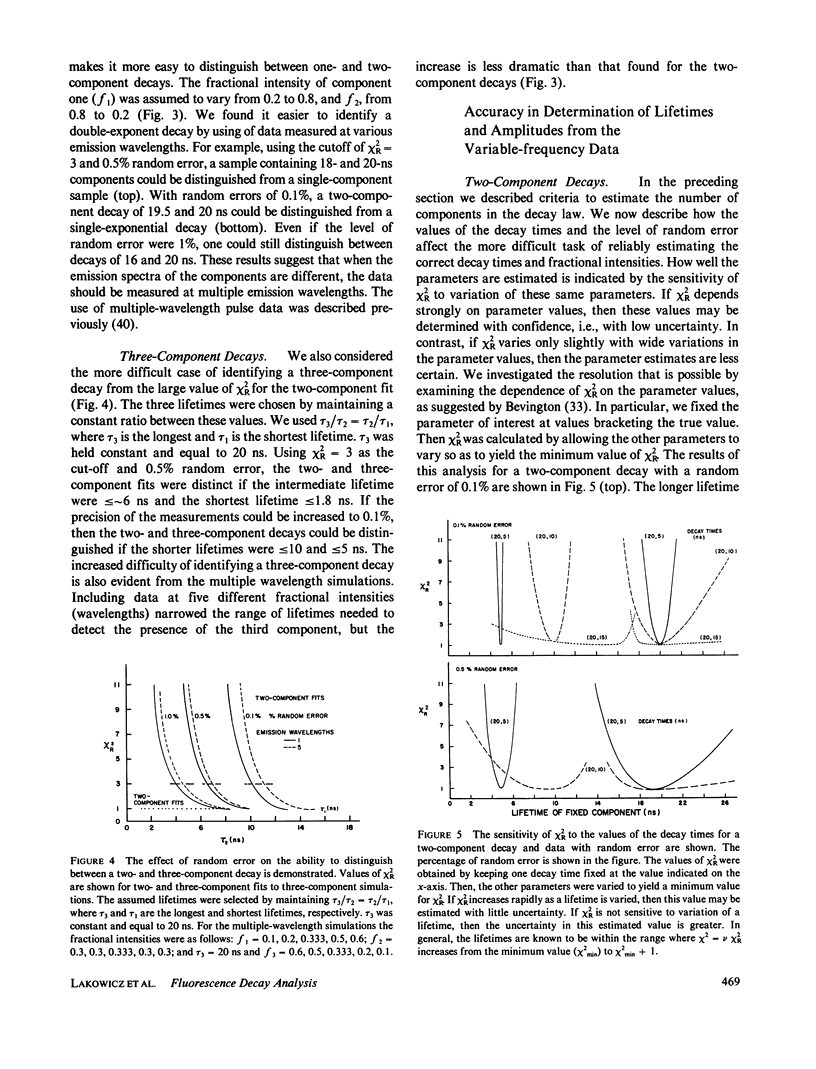
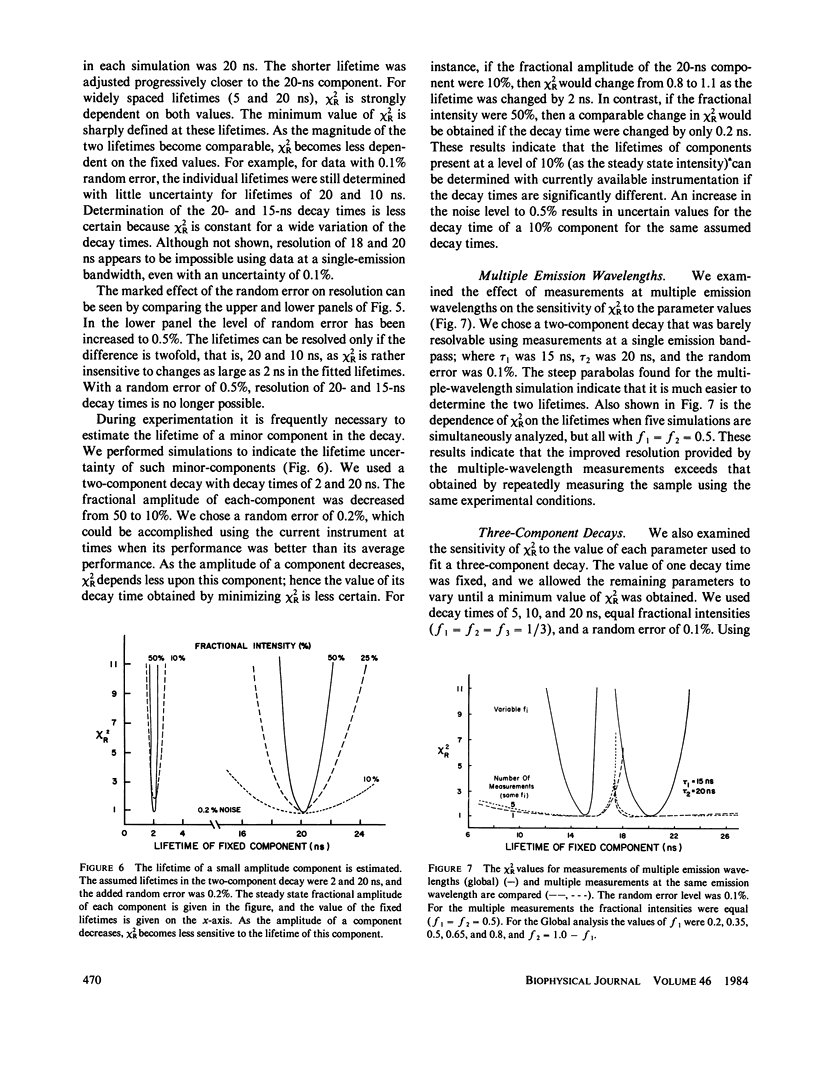
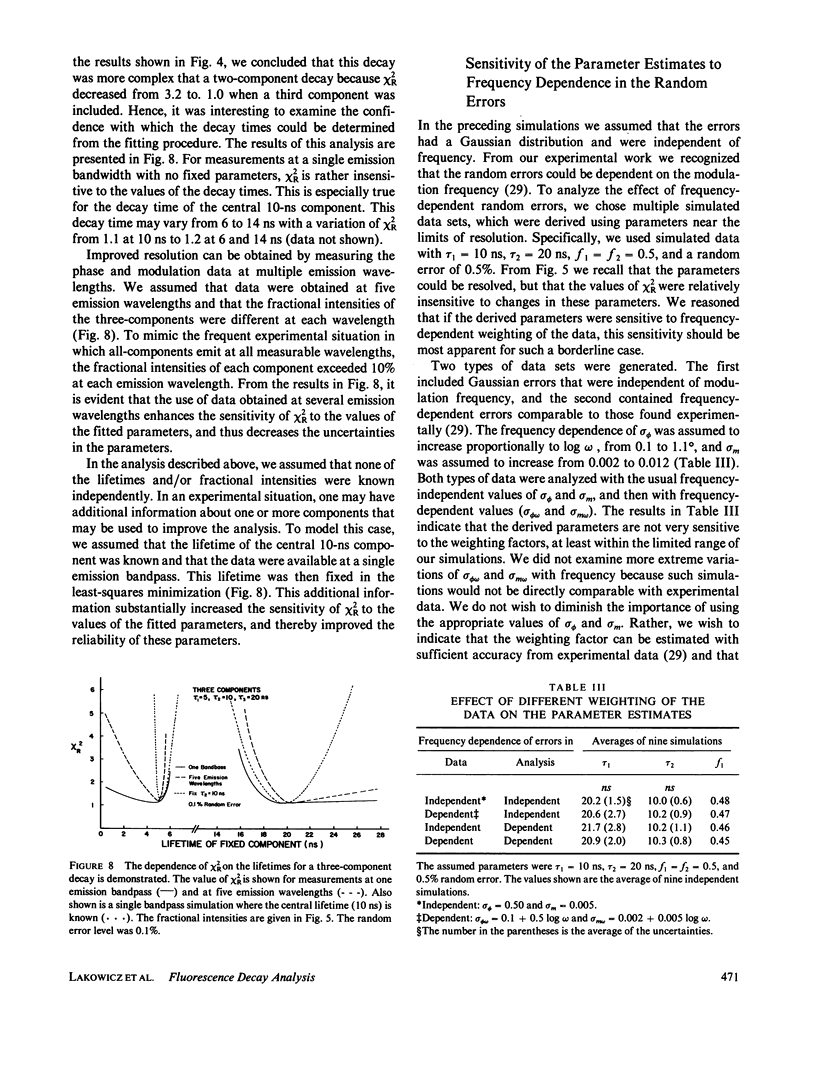
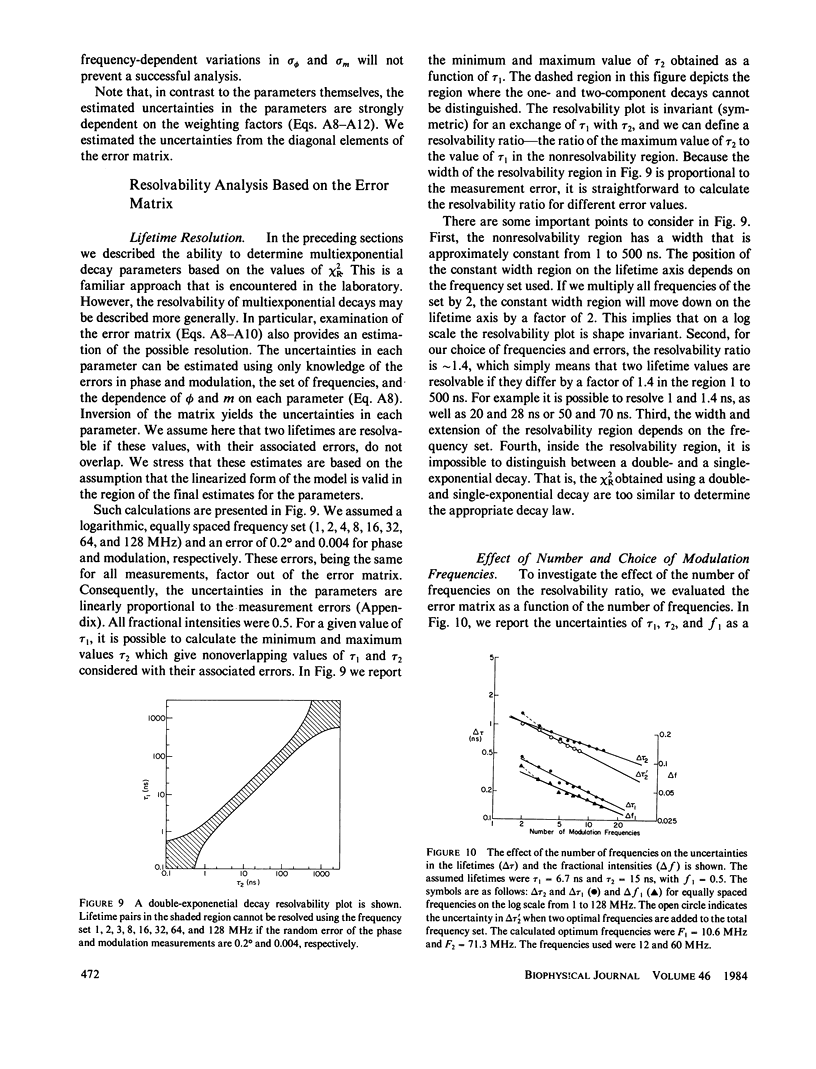
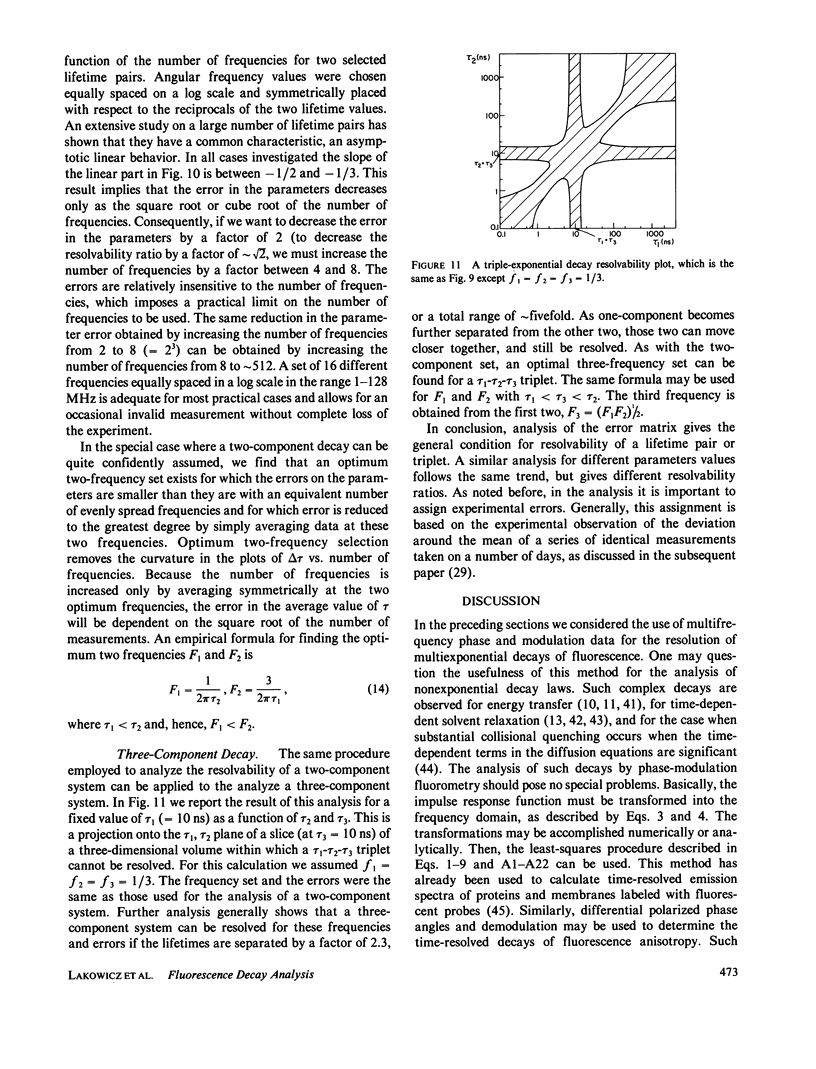
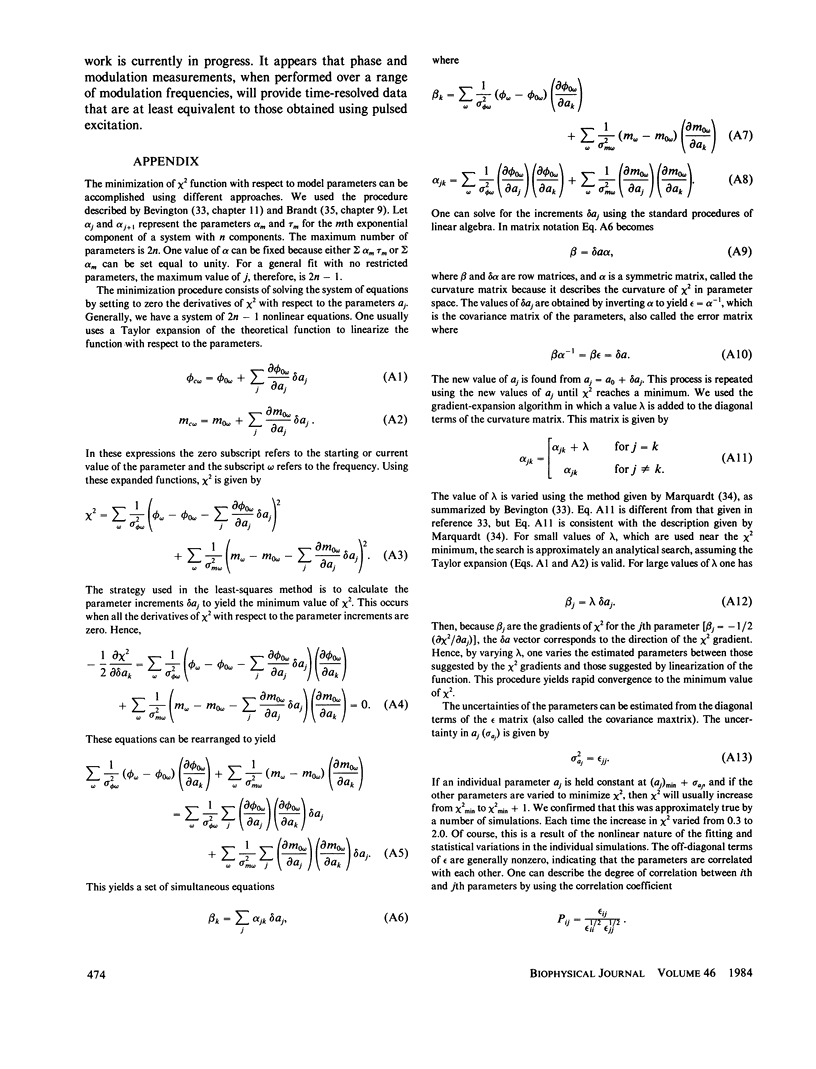
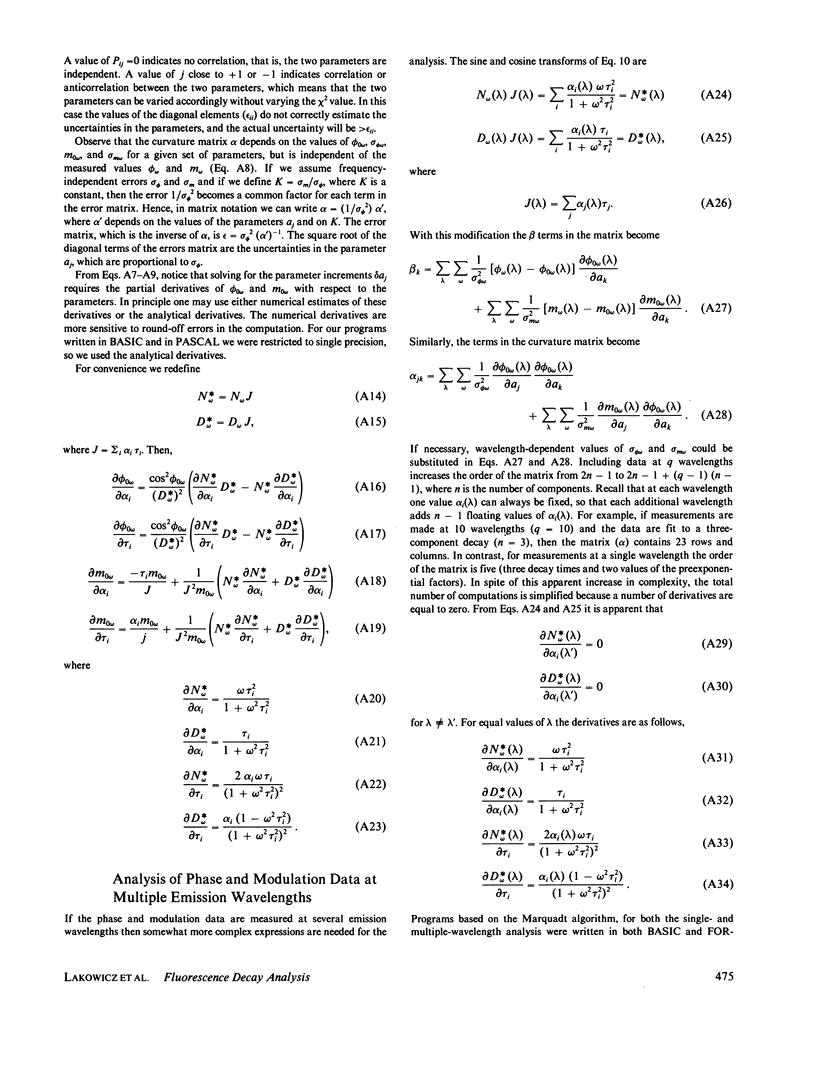
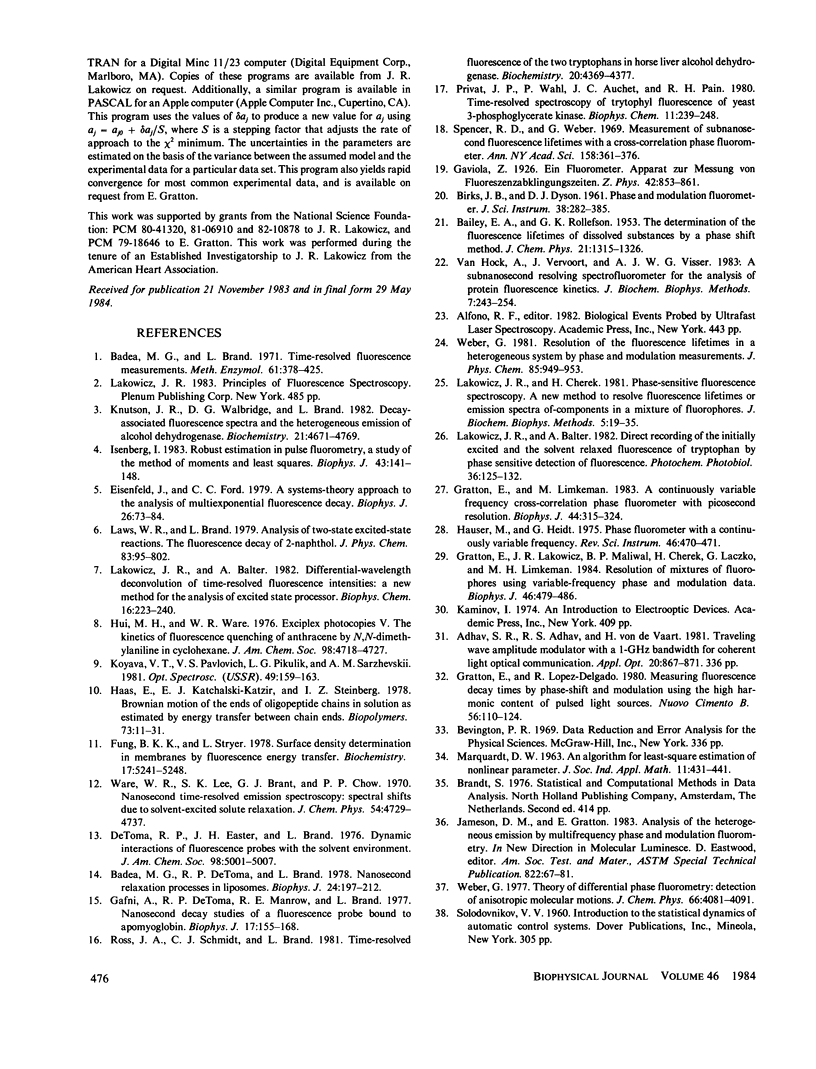
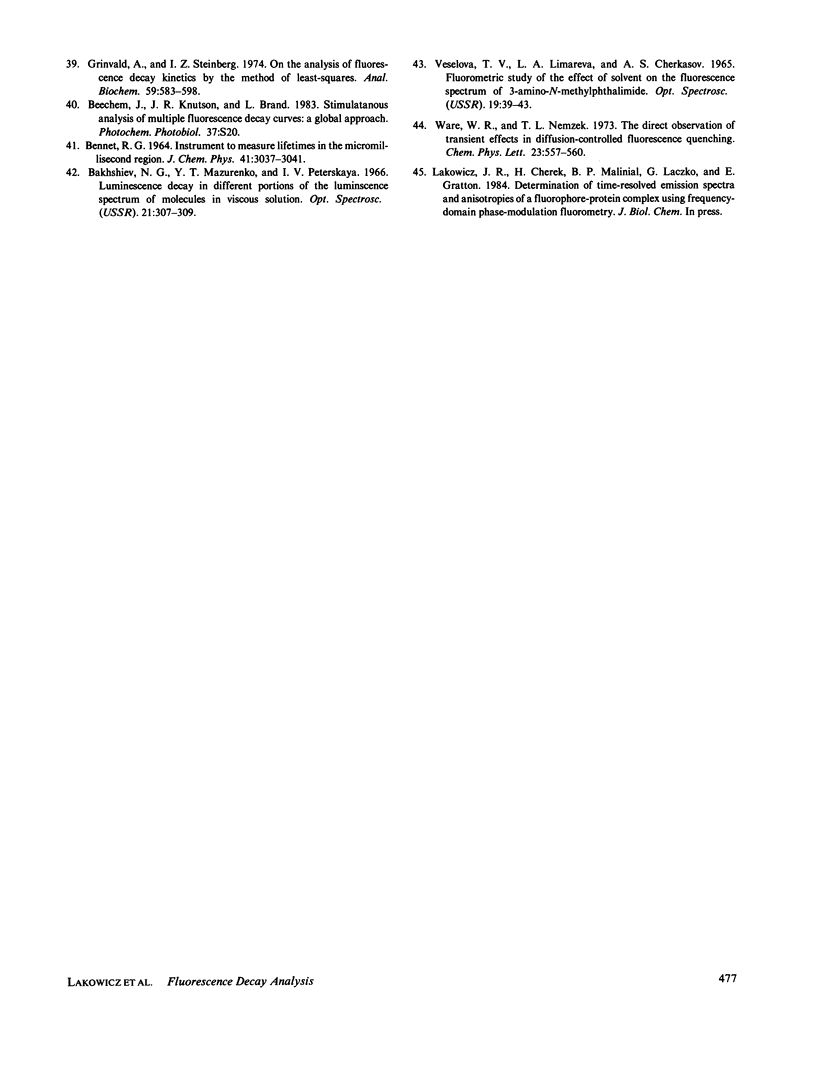
Selected References
These references are in PubMed. This may not be the complete list of references from this article.
- Badea M. G., Brand L. Time-resolved fluorescence measurements. Methods Enzymol. 1979;61:378–425. doi: 10.1016/0076-6879(79)61019-4. [DOI] [PubMed] [Google Scholar]
- Badea M. G., DeToma R. P., Brand L. Nanosecond relaxation processes in liposomes. Biophys J. 1978 Oct;24(1):197–212. doi: 10.1016/S0006-3495(78)85356-9. [DOI] [PMC free article] [PubMed] [Google Scholar]
- De Toma R. P., Easter J. H., Brand L. Dynamic interactions of fluorescence probes with the solvent environment. J Am Chem Soc. 1976 Aug 4;98(16):5001–5007. doi: 10.1021/ja00432a048. [DOI] [PubMed] [Google Scholar]
- Eisenfeld J., Ford C. C. A systems-theory approach to the analysis of multiexponential fluorescence decay. Biophys J. 1979 Apr;26(1):73–83. doi: 10.1016/S0006-3495(79)85236-4. [DOI] [PMC free article] [PubMed] [Google Scholar]
- Fung B. K., Stryer L. Surface density determination in membranes by fluorescence energy transfer. Biochemistry. 1978 Nov 28;17(24):5241–5248. doi: 10.1021/bi00617a025. [DOI] [PubMed] [Google Scholar]
- Gafni A., DeToma R. P., Manrow R. E., Brand L. Nanosecond decay studies of a fluorescence probe bound to apomyoglobin. Biophys J. 1977 Feb;17(2):155–168. doi: 10.1016/S0006-3495(77)85633-6. [DOI] [PMC free article] [PubMed] [Google Scholar]
- Gratton E., Limkeman M. A continuously variable frequency cross-correlation phase fluorometer with picosecond resolution. Biophys J. 1983 Dec;44(3):315–324. doi: 10.1016/S0006-3495(83)84305-7. [DOI] [PMC free article] [PubMed] [Google Scholar]
- Gratton E., Limkeman M., Lakowicz J. R., Maliwal B. P., Cherek H., Laczko G. Resolution of mixtures of fluorophores using variable-frequency phase and modulation data. Biophys J. 1984 Oct;46(4):479–486. doi: 10.1016/S0006-3495(84)84044-8. [DOI] [PMC free article] [PubMed] [Google Scholar]
- Grinvald A., Steinberg I. Z. On the analysis of fluorescence decay kinetics by the method of least-squares. Anal Biochem. 1974 Jun;59(2):583–598. doi: 10.1016/0003-2697(74)90312-1. [DOI] [PubMed] [Google Scholar]
- Isenberg I. Robust estimation in pulse fluorometry. A study of the method of moments and least squares. Biophys J. 1983 Aug;43(2):141–148. doi: 10.1016/S0006-3495(83)84334-3. [DOI] [PMC free article] [PubMed] [Google Scholar]
- Knutson J. R., Walbridge D. G., Brand L. Decay-associated fluorescence spectra and the heterogeneous emission of alcohol dehydrogenase. Biochemistry. 1982 Sep 14;21(19):4671–4679. doi: 10.1021/bi00262a024. [DOI] [PubMed] [Google Scholar]
- Lakowicz J. R., Balter A. Differential-wavelength deconvolution of time-resolved fluorescence intensities. A new method for the analysis of excited-state processes. Biophys Chem. 1982 Nov;16(3):223–240. doi: 10.1016/0301-4622(82)87005-1. [DOI] [PubMed] [Google Scholar]
- Lakowicz J. R., Balter A. Direct recording of the initially excited and the solvent relaxed fluorescence emission spectra of tryptophan by phase sensitive detection of fluorescence. Photochem Photobiol. 1982 Aug;36(2):125–132. doi: 10.1111/j.1751-1097.1982.tb04353.x. [DOI] [PubMed] [Google Scholar]
- Lakowicz J. R., Cherek H. Phase-sensitive fluorescence spectroscopy: a new method to resolve fluorescence lifetimes or emission spectra of components in a mixture of fluorophores. J Biochem Biophys Methods. 1981 Jul;5(1):19–35. doi: 10.1016/0165-022x(81)90030-0. [DOI] [PubMed] [Google Scholar]
- Privat J. P., Wahl P., Auchet J. C., Pain R. H. Time resolved spectroscopy of tryptophyl fluorescence of yeast 3-phosphoglycerate kinase. Biophys Chem. 1980 Apr;11(2):239–248. doi: 10.1016/0301-4622(80)80026-3. [DOI] [PubMed] [Google Scholar]
- Ross J. B., Schmidt C. J., Brand L. Time-resolved fluorescence of the two tryptophans in horse liver alcohol dehydrogenase. Biochemistry. 1981 Jul 21;20(15):4369–4377. doi: 10.1021/bi00518a021. [DOI] [PubMed] [Google Scholar]
- van Hoek A., Vervoort J., Visser A. J. A subnanosecond resolving spectrofluorimeter for the analysis of protein fluorescence kinetics. J Biochem Biophys Methods. 1983 May;7(3):243–254. doi: 10.1016/0165-022x(83)90033-7. [DOI] [PubMed] [Google Scholar]