Abstract
The relationship between epithelial fluid transport, standing osmotic gradients, and standing hydrostatic pressure gradients has been investigated using a perturbation expansion of the governing equations. The assumptions used in the expansion are: (a) the volume of lateral intercellular space per unit volume of epithelium is small; (b) the membrane osmotic permeability is much larger than the solute permeability. We find that the rate of fluid reabsorption is set by the rate of active solute transport across lateral membranes. The fluid that crosses the lateral membranes and enters the intercellular cleft is driven longitudinally by small gradients in hydrostatic pressure. The small hydrostatic pressure in the intercellular space is capable of causing significant transmembrane fluid movement, however, the transmembrane effect is countered by the presence of a small standing osmotic gradient. Longitudinal hydrostatic and osmotic gradients balance such that their combined effect on transmembrane fluid flow is zero, whereas longitudinal flow is driven by the hydrostatic gradient. Because of this balance, standing gradients within intercellular clefts are effectively uncoupled from the rate of fluid reabsorption, which is driven by small, localized osmotic gradients within the cells. Water enters the cells across apical membranes and leaves across the lateral intercellular membranes. Fluid that enters the intercellular clefts can, in principle, exit either the basal end or be secreted from the apical end through tight junctions. Fluid flow through tight junctions is shown to depend on a dimensionless parameter, which scales the resistance to solute flow of the entire cleft relative to that of the junction. Estimates of the value of this parameter suggest that an electrically leaky epithelium may be effectively a tight epithelium in regard to fluid flow.
Full text
PDF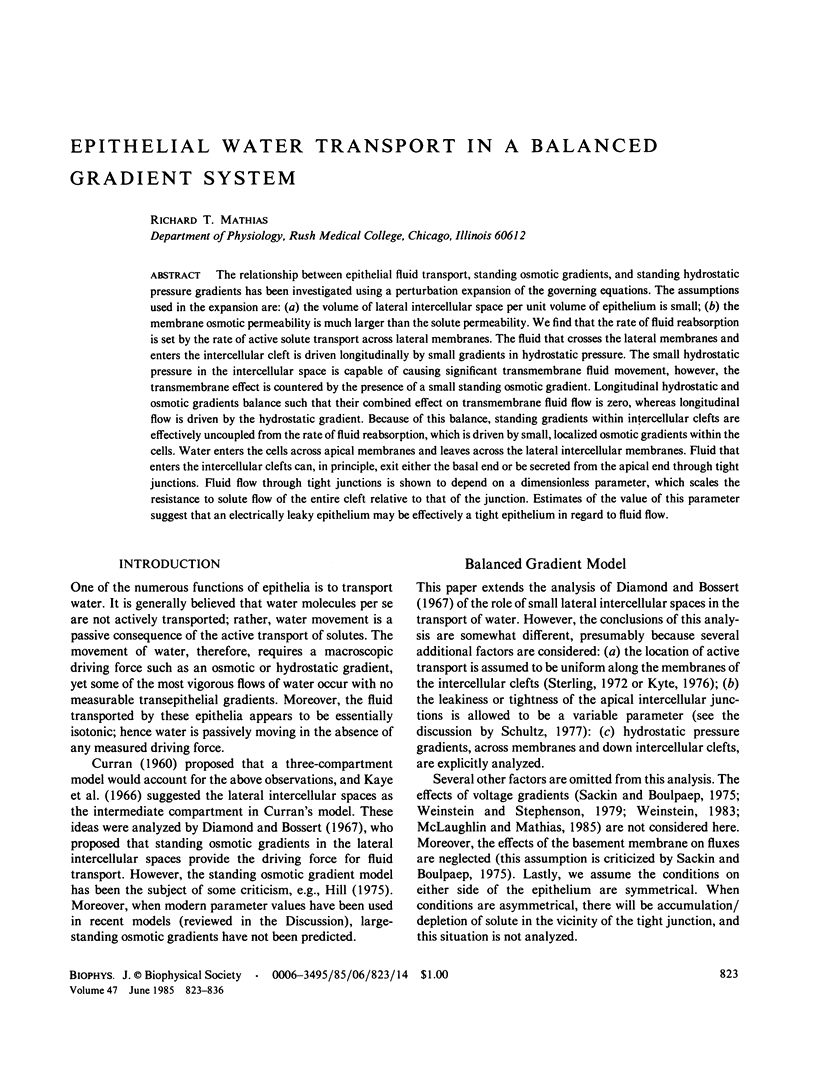
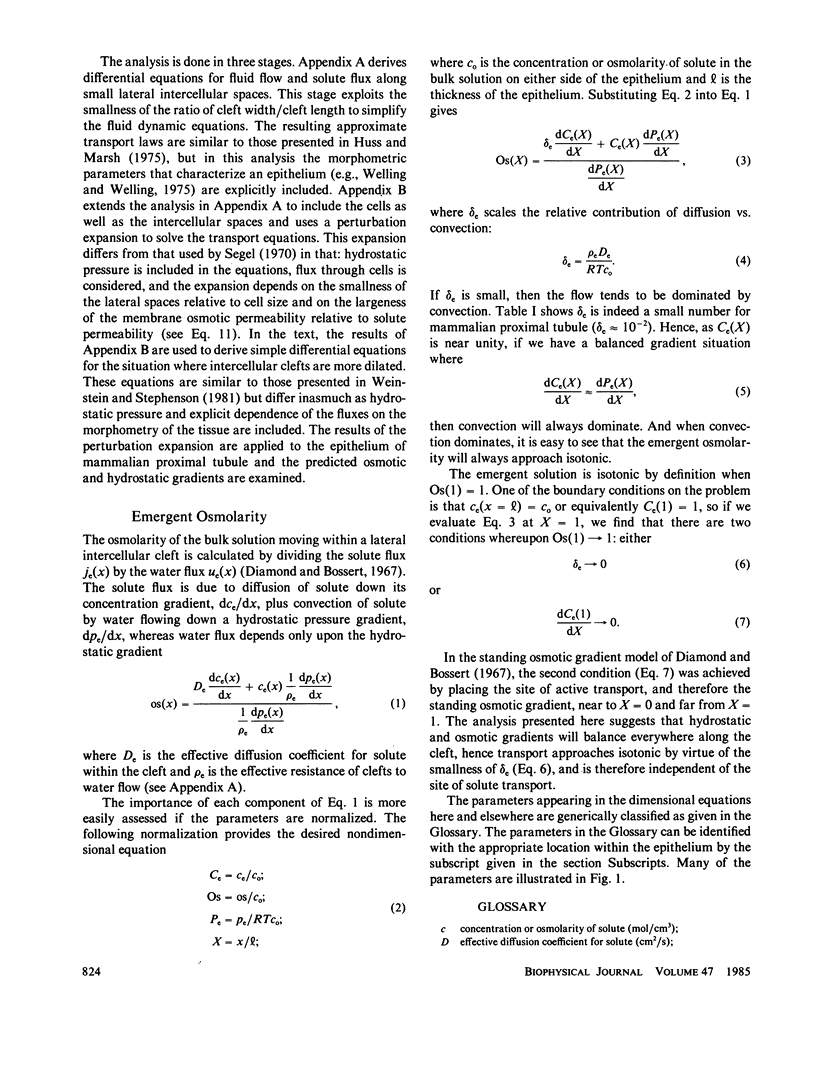
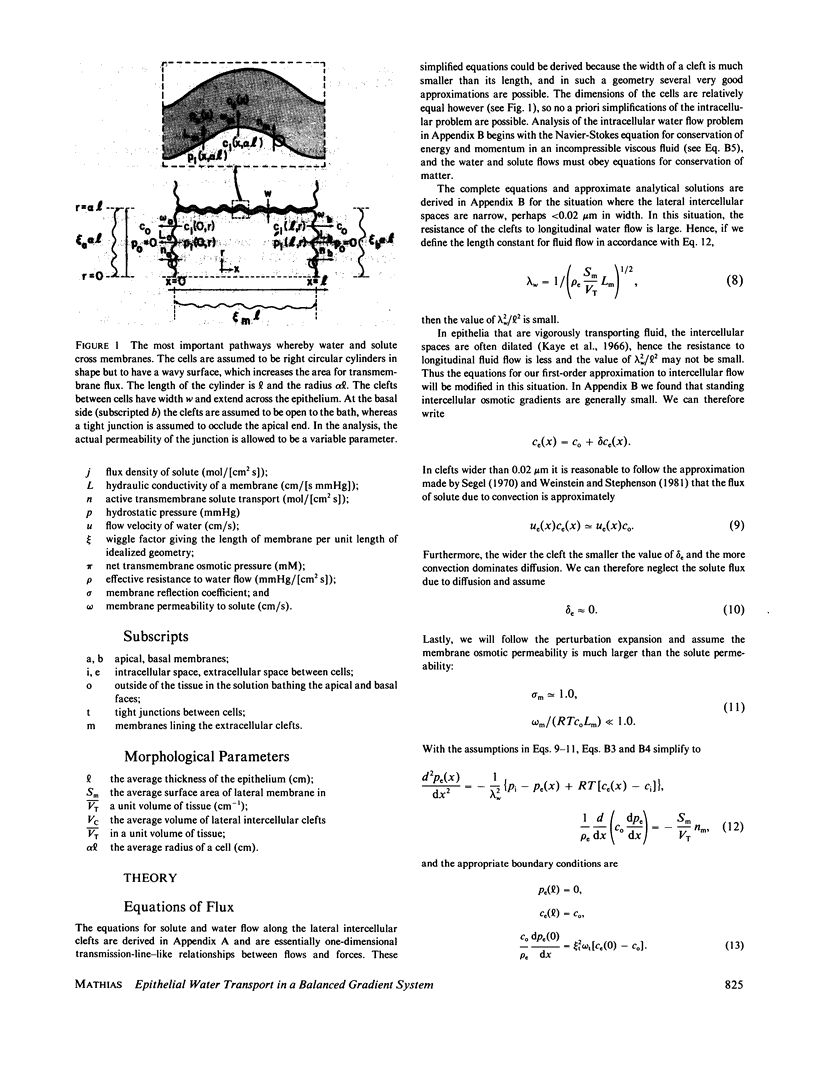
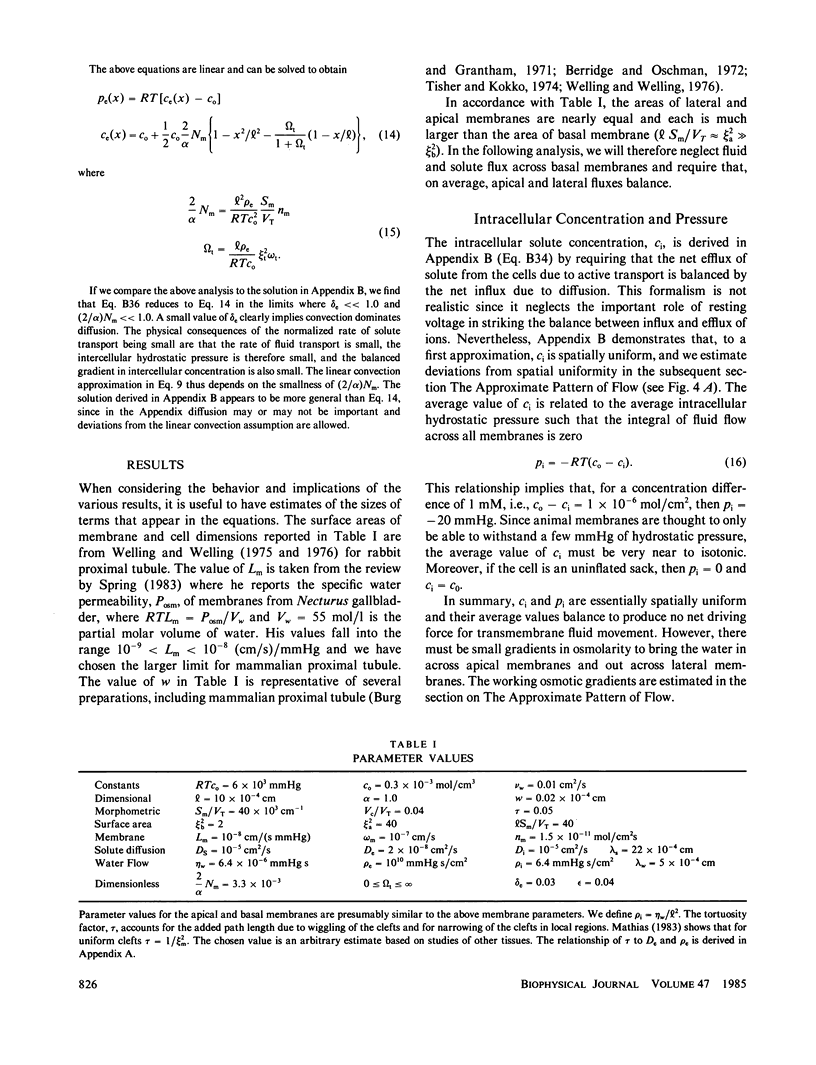
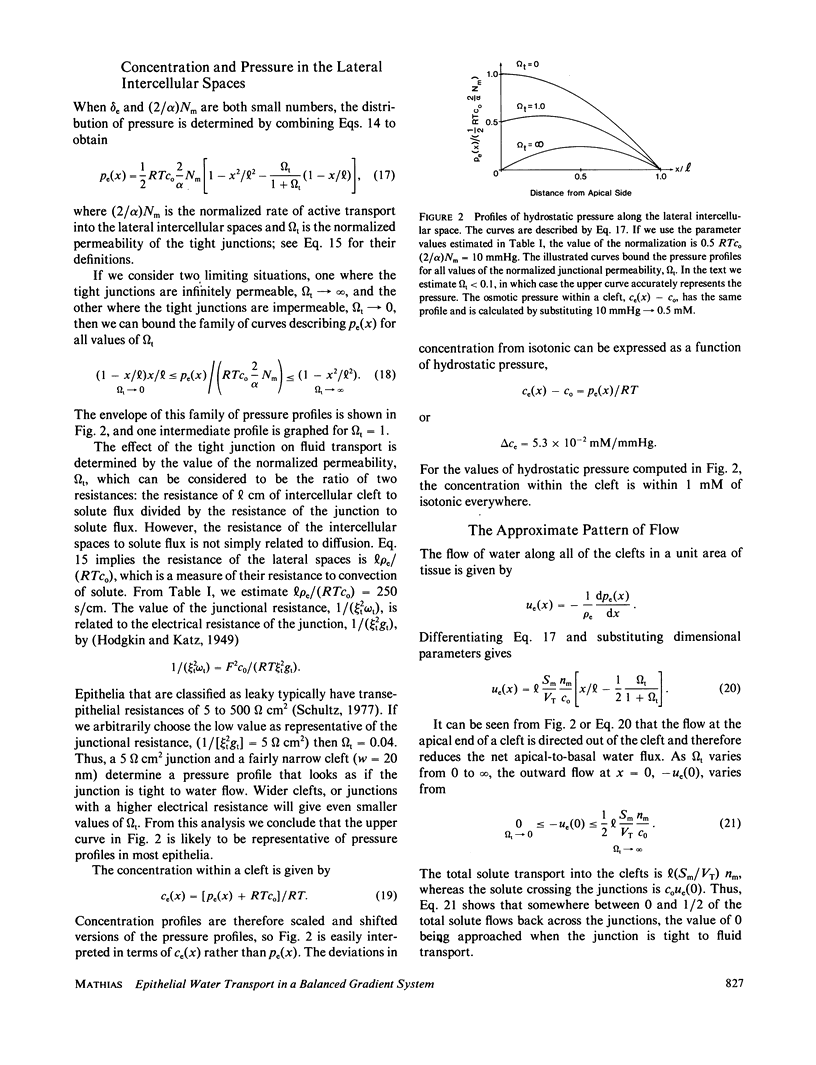
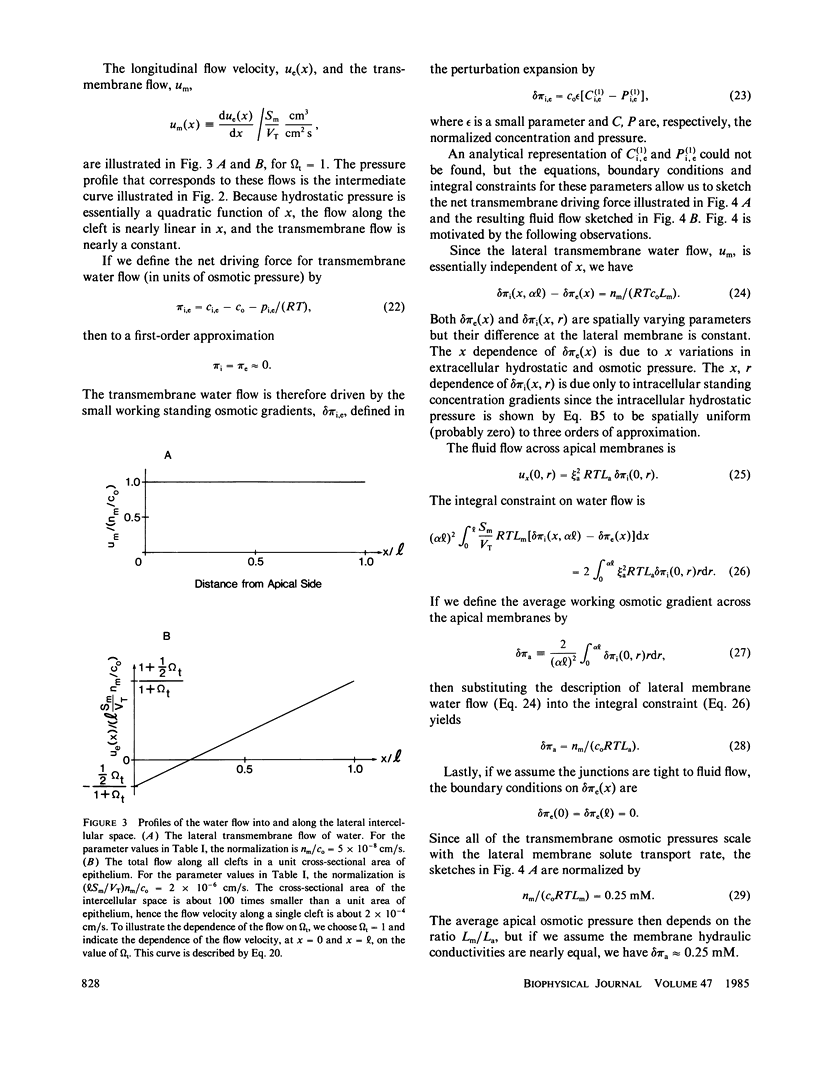
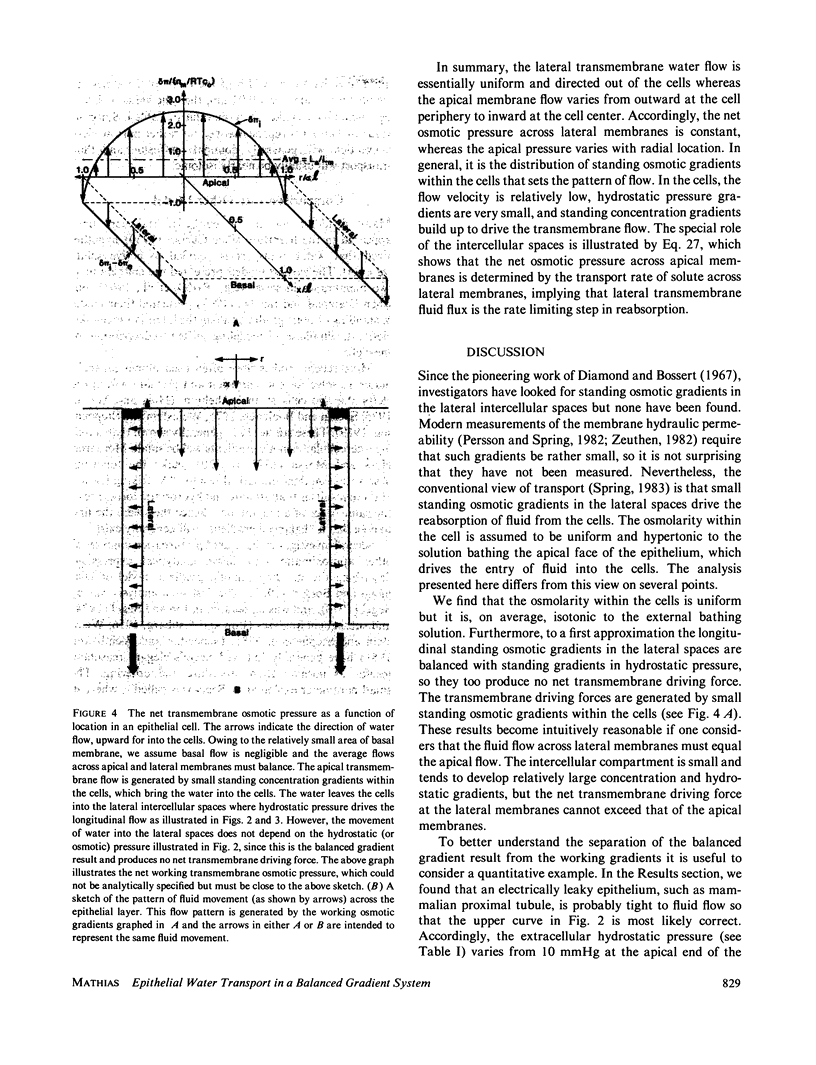
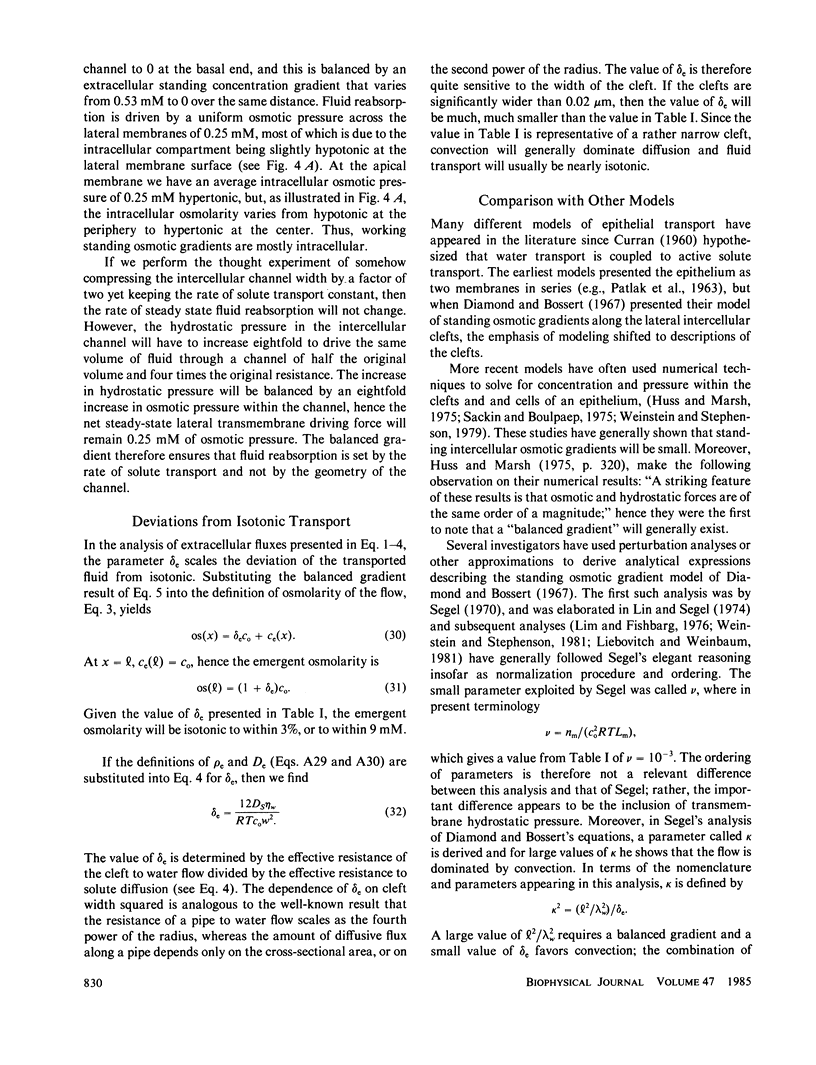
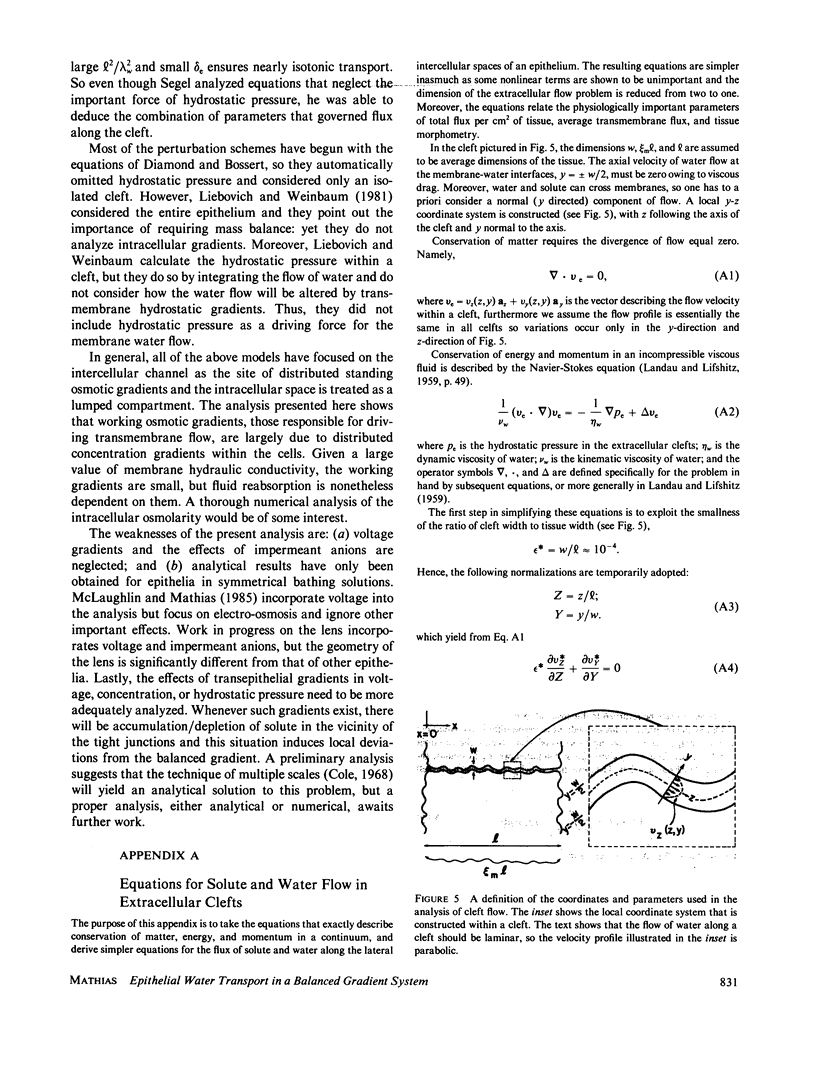
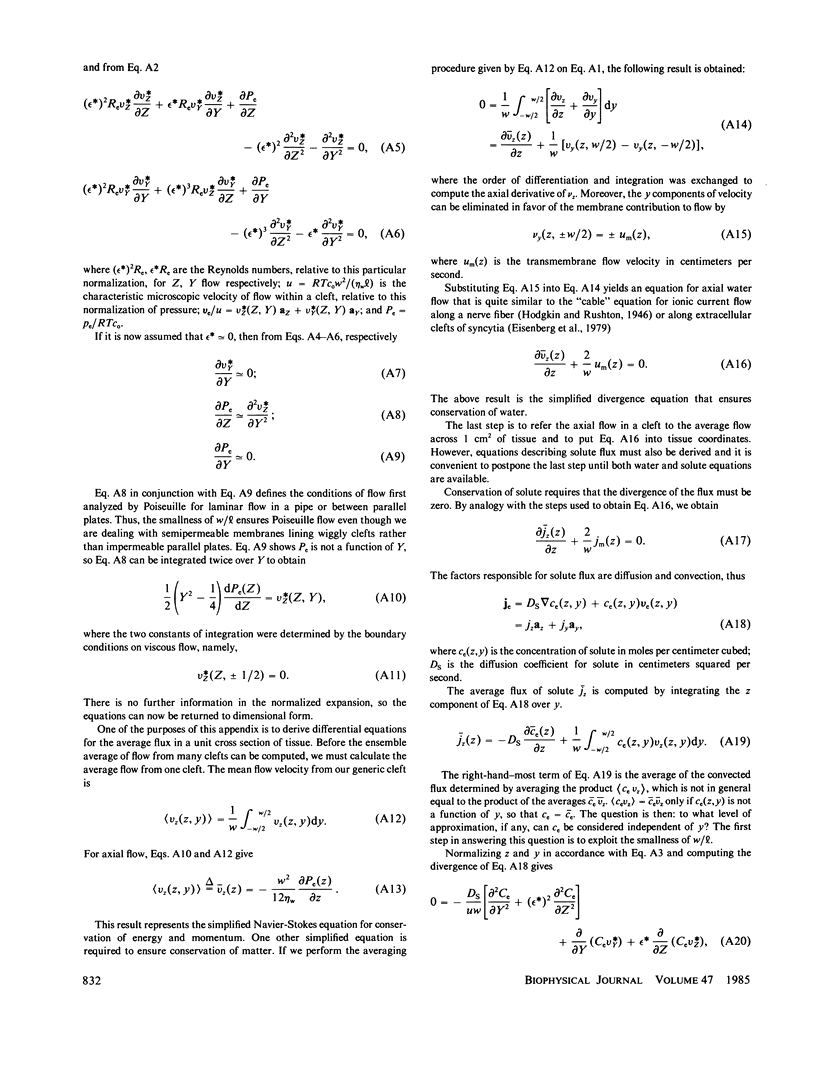
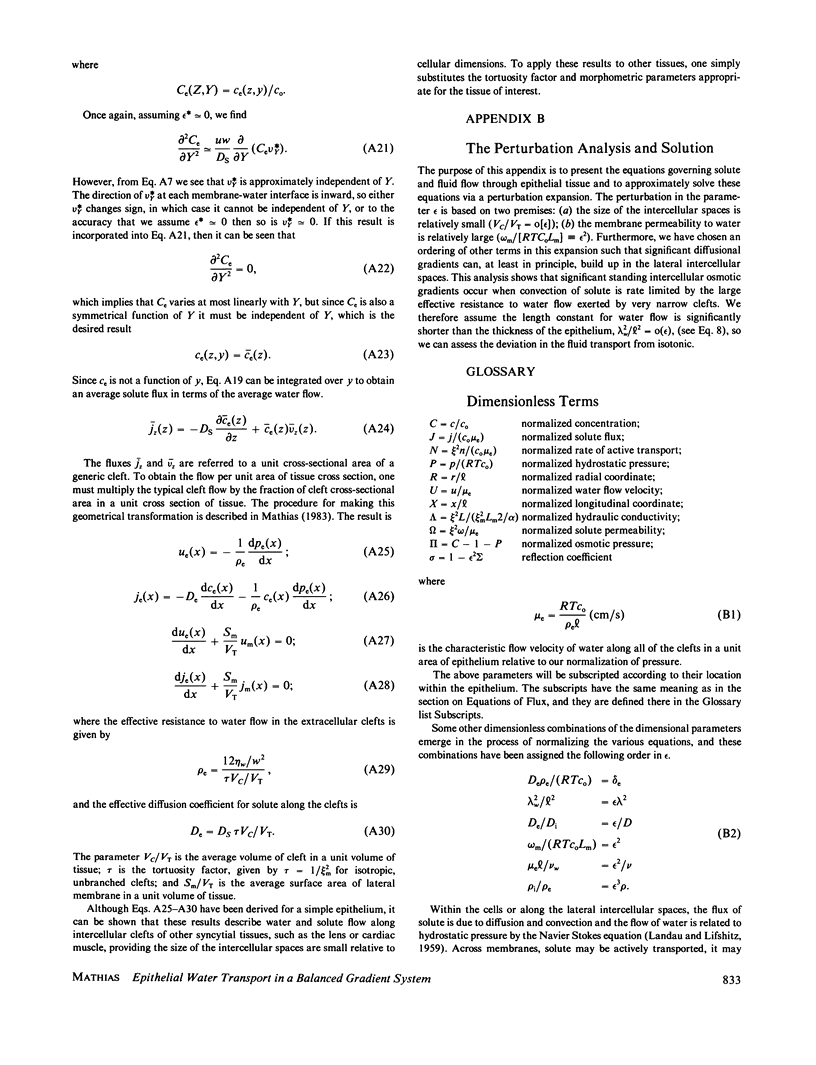
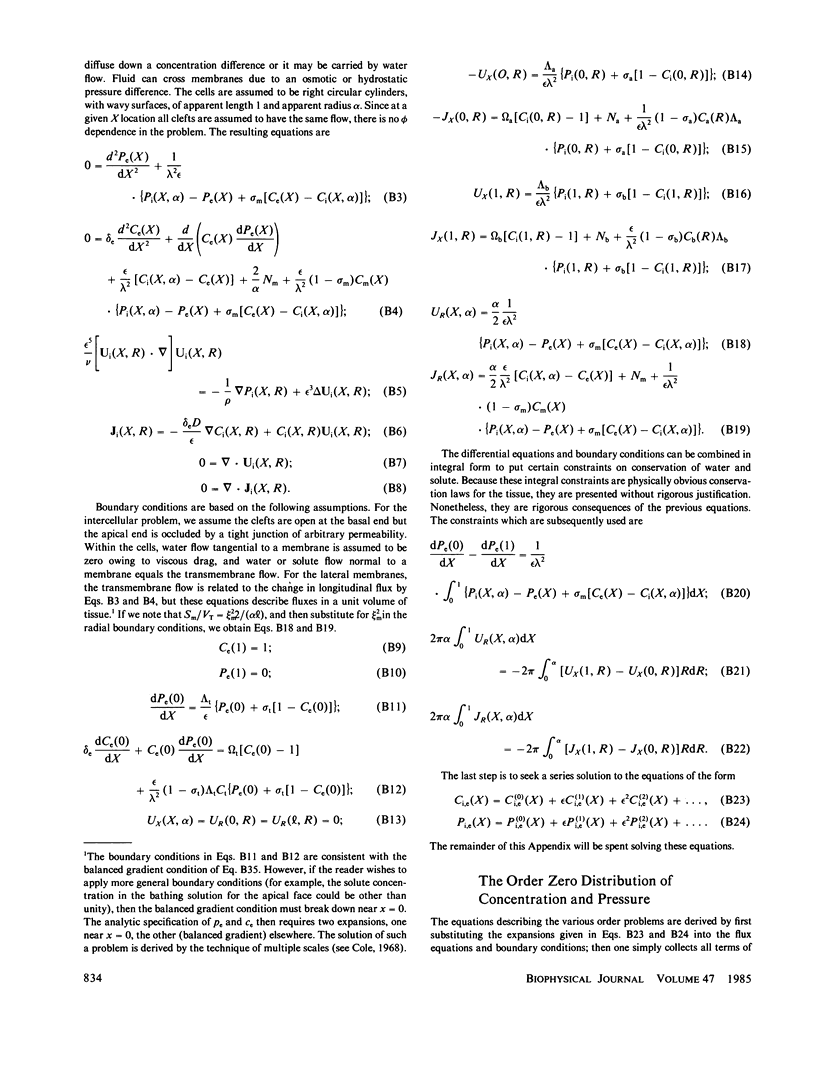
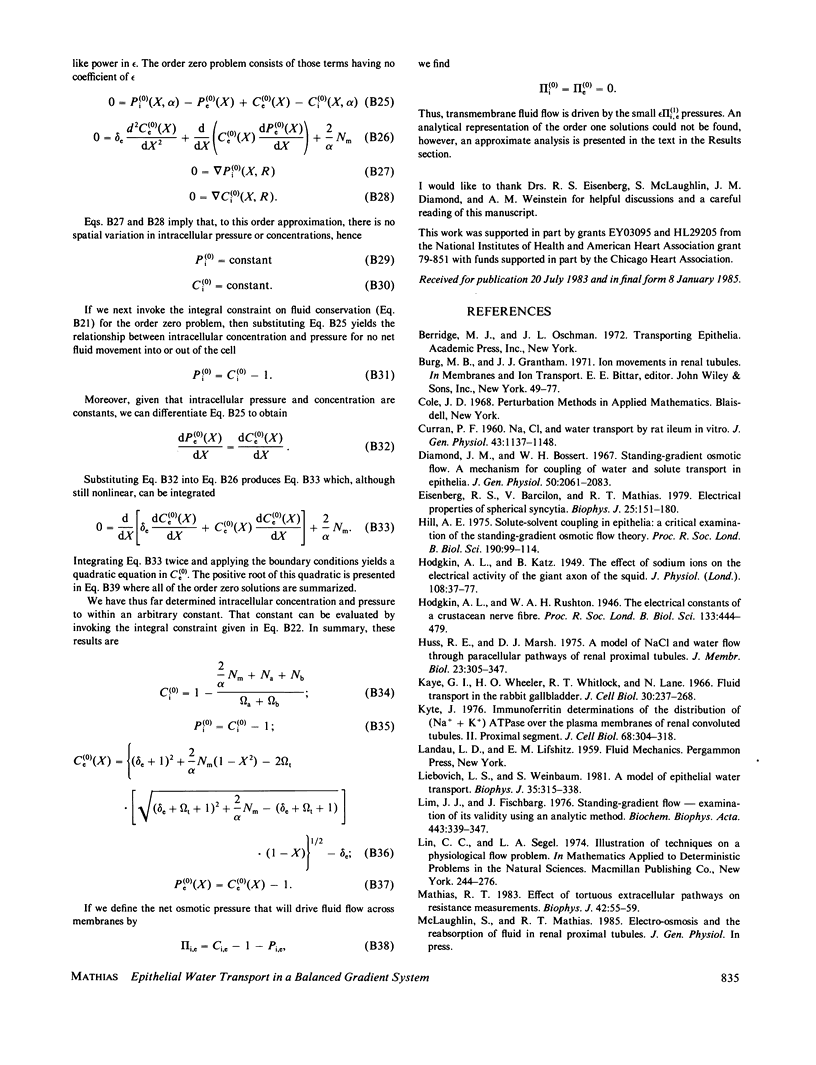
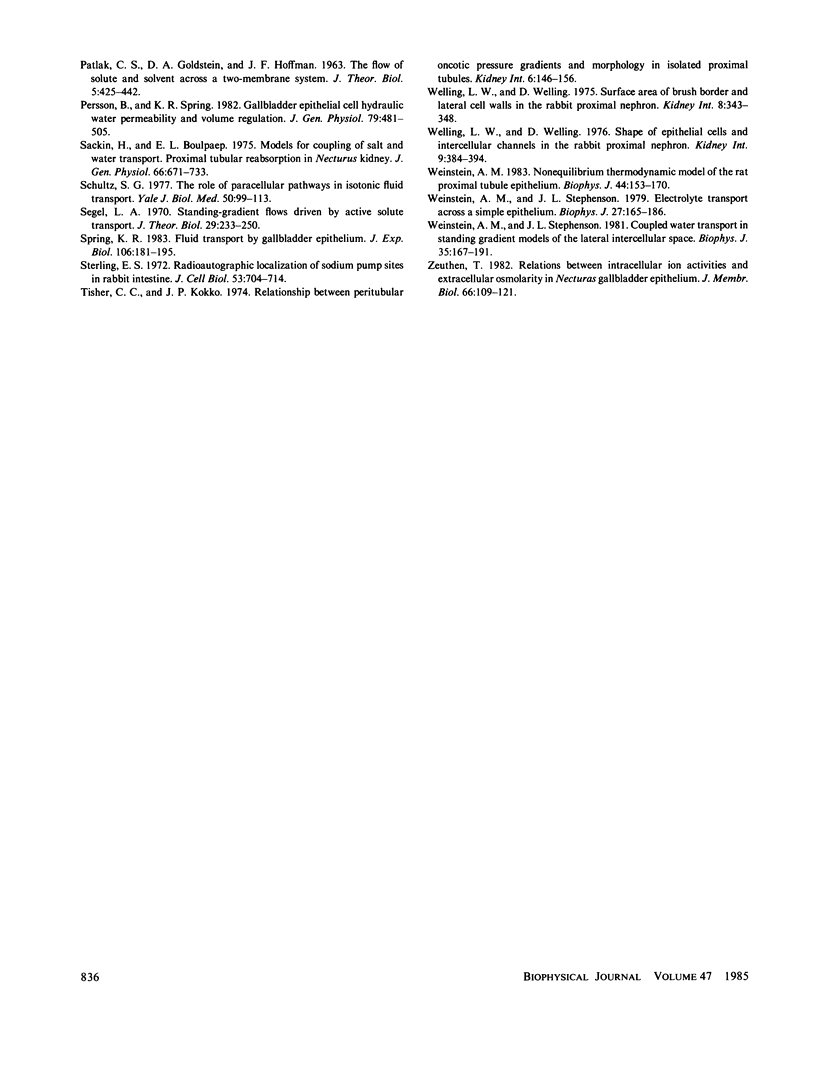
Selected References
These references are in PubMed. This may not be the complete list of references from this article.
- CURRAN P. F. Na, Cl, and water transport by rat ileum in vitro. J Gen Physiol. 1960 Jul;43:1137–1148. doi: 10.1085/jgp.43.6.1137. [DOI] [PMC free article] [PubMed] [Google Scholar]
- Diamond J. M., Bossert W. H. Standing-gradient osmotic flow. A mechanism for coupling of water and solute transport in epithelia. J Gen Physiol. 1967 Sep;50(8):2061–2083. doi: 10.1085/jgp.50.8.2061. [DOI] [PMC free article] [PubMed] [Google Scholar]
- Eisenberg R. S., Barcilon V., Mathias R. T. Electrical properties of spherical syncytia. Biophys J. 1979 Jan;25(1):151–180. doi: 10.1016/S0006-3495(79)85283-2. [DOI] [PMC free article] [PubMed] [Google Scholar]
- HODGKIN A. L., KATZ B. The effect of sodium ions on the electrical activity of giant axon of the squid. J Physiol. 1949 Mar 1;108(1):37–77. doi: 10.1113/jphysiol.1949.sp004310. [DOI] [PMC free article] [PubMed] [Google Scholar]
- Hill A. E. Solute-solvent coupling in epithelia: a critical examination of the standing-gradient osmotic flow theory. Proc R Soc Lond B Biol Sci. 1975 Jun 20;190(1098):99–114. doi: 10.1098/rspb.1975.0081. [DOI] [PubMed] [Google Scholar]
- Huss R. E., Marsh D. J. A model of NaCl and water flow through paracellular pathways of renal proximal tubules. J Membr Biol. 1975;23(3-4):305–347. doi: 10.1007/BF01870256. [DOI] [PubMed] [Google Scholar]
- Kaye G. I., Wheeler H. O., Whitlock R. T., Lane N. Fluid transport in the rabbit gallbladder. A combined physiological and electron microscopic study. J Cell Biol. 1966 Aug;30(2):237–268. doi: 10.1083/jcb.30.2.237. [DOI] [PMC free article] [PubMed] [Google Scholar]
- Kyte J. Immunoferritin determination of the distribution of (Na+ + K+) ATPase over the plasma membranes of renal convoluted tubules. II. Proximal segment. J Cell Biol. 1976 Feb;68(2):304–318. doi: 10.1083/jcb.68.2.304. [DOI] [PMC free article] [PubMed] [Google Scholar]
- Liebovitch L. S., Weinbaum S. A model of epithelial water transport. The corneal endothelium. Biophys J. 1981 Aug;35(2):315–338. doi: 10.1016/S0006-3495(81)84792-3. [DOI] [PMC free article] [PubMed] [Google Scholar]
- Lim J. J., Fischbarg J. Standing-gradient osmotic flow. Examination of its validity using an analytical method. Biochim Biophys Acta. 1976 Sep 7;443(3):339–347. doi: 10.1016/0005-2736(76)90034-1. [DOI] [PubMed] [Google Scholar]
- Mathias R. T. Effect of tortuous extracellular pathways on resistance measurements. Biophys J. 1983 Apr;42(1):55–59. doi: 10.1016/S0006-3495(83)84368-9. [DOI] [PMC free article] [PubMed] [Google Scholar]
- Patlak C. S., Goldstein D. A., Hoffman J. F. The flow of solute and solvent across a two-membrane system. J Theor Biol. 1963 Nov;5(3):426–442. doi: 10.1016/0022-5193(63)90088-2. [DOI] [PubMed] [Google Scholar]
- Persson B. E., Spring K. R. Gallbladder epithelial cell hydraulic water permeability and volume regulation. J Gen Physiol. 1982 Mar;79(3):481–505. doi: 10.1085/jgp.79.3.481. [DOI] [PMC free article] [PubMed] [Google Scholar]
- Sackin H., Boulpaep E. L. Models for coupling of salt and water transport; Proximal tubular reabsorption in Necturus kidney. J Gen Physiol. 1975 Dec;66(6):671–733. doi: 10.1085/jgp.66.6.671. [DOI] [PMC free article] [PubMed] [Google Scholar]
- Schultz S. G. The role of paracellular pathways in isotonic fluid transport. Yale J Biol Med. 1977 Mar-Apr;50(2):99–113. [PMC free article] [PubMed] [Google Scholar]
- Segel L. A. Standing-gradient flows driven by active solute transport. J Theor Biol. 1970 Nov;29(2):233–250. doi: 10.1016/0022-5193(70)90020-2. [DOI] [PubMed] [Google Scholar]
- Spring K. R. Fluid transport by gallbladder epithelium. J Exp Biol. 1983 Sep;106:181–194. doi: 10.1242/jeb.106.1.181. [DOI] [PubMed] [Google Scholar]
- Stirling C. E. Radioautographic localization of sodium pump sites in rabbit intestine. J Cell Biol. 1972 Jun;53(3):704–714. doi: 10.1083/jcb.53.3.704. [DOI] [PMC free article] [PubMed] [Google Scholar]
- Tisher C. C., Kokko J. P. Relationship between peritubular oncotic pressure gradients and morphology in isolated proximal tubules. Kidney Int. 1974 Sep;6(3):146–156. doi: 10.1038/ki.1974.93. [DOI] [PubMed] [Google Scholar]
- Weinstein A. M. Nonequilibrium thermodynamic model of the rat proximal tubule epithelium. Biophys J. 1983 Nov;44(2):153–170. doi: 10.1016/S0006-3495(83)84287-8. [DOI] [PMC free article] [PubMed] [Google Scholar]
- Weinstein A. M., Stephenson J. L. Coupled water transport in standing gradient models of the lateral intercellular space. Biophys J. 1981 Jul;35(1):167–191. doi: 10.1016/S0006-3495(81)84781-9. [DOI] [PMC free article] [PubMed] [Google Scholar]
- Weinstein A. M., Stephenson J. L. Electrolyte transport across a simple epithelium. Steady-state and transient analysis. Biophys J. 1979 Aug;27(2):165–186. doi: 10.1016/S0006-3495(79)85209-1. [DOI] [PMC free article] [PubMed] [Google Scholar]
- Welling L. W., Welling D. J. Shape of epithelial cells and intercellular channels in the rabbit proximal nephron. Kidney Int. 1976 May;9(5):385–394. doi: 10.1038/ki.1976.48. [DOI] [PubMed] [Google Scholar]
- Welling L. W., Welling D. J. Surface areas of brush border and lateral cell walls in the rabbit proximal nephron. Kidney Int. 1975 Dec;8(6):343–348. doi: 10.1038/ki.1975.125. [DOI] [PubMed] [Google Scholar]
- Zeuthen T. Relations between intracellular ion activities and extracellular osmolarity in Necturus gallbladder epithelium. J Membr Biol. 1982;66(2):109–121. doi: 10.1007/BF01868487. [DOI] [PubMed] [Google Scholar]