Abstract
Sickle hemoglobin (Hb S) gelation displays kinetics consistent with a rate-limiting nucleation step. The approximate size of the critical nucleus can be inferred from the order of the reaction with respect to Hb S activity, but determination of the reaction order is complicated by the fact that Hb S activity is substantially different from Hb S concentration at the high protein concentrations required for gelation. Equilibrium and kinetic experiments on Hb S gelation were designed to evaluate the relative activity coefficient of Hb S as a function of concentration. These experiments used non-Hb S proteins to mimic, and thus evaluate, the effect on activity coefficients of increasing Hb S concentration. At Hb S concentrations near 20% the change in Hb S activity coefficient generates two-thirds of the apparent dependence of nucleation rate on Hb S concentration. When this effect is explicitly accounted for, the nucleation reaction is seen to be approximately 10th-order with respect to effective number concentration of Hb S. The closeness of the reaction order to the number of strands in models of Hb S fibers suggests a nucleus close to the size of one turn of the Hb S fiber. These experiments introduce a new approach to the study of Hb S gelation, the equal activity isotherm, used here also to show that Hb S.Hb A (normal adult hemoglobin) hybrids do incorporate into growing nuclei and stable microtubules but that A.S hybridization is neutral with respect to promotion of Hb S nucleation and the sol-gel equilibrium.
Full text
PDF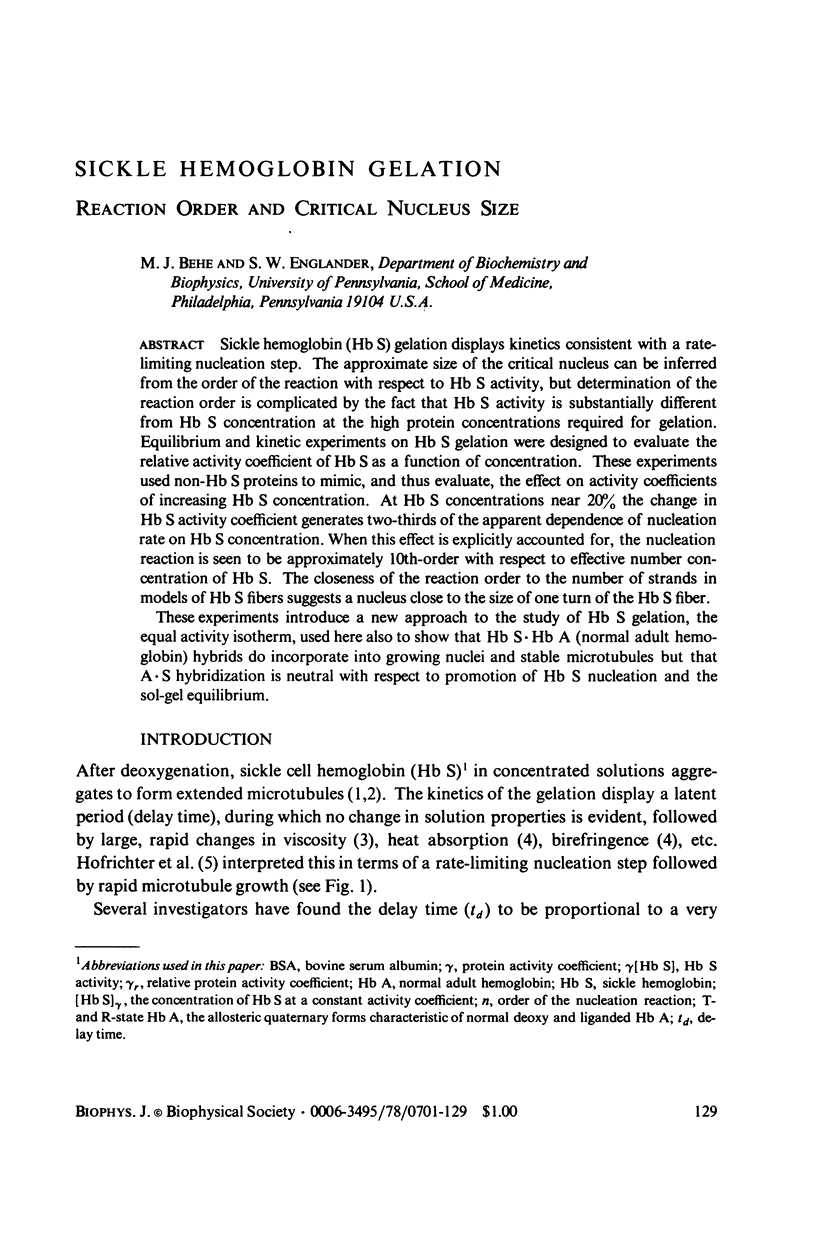
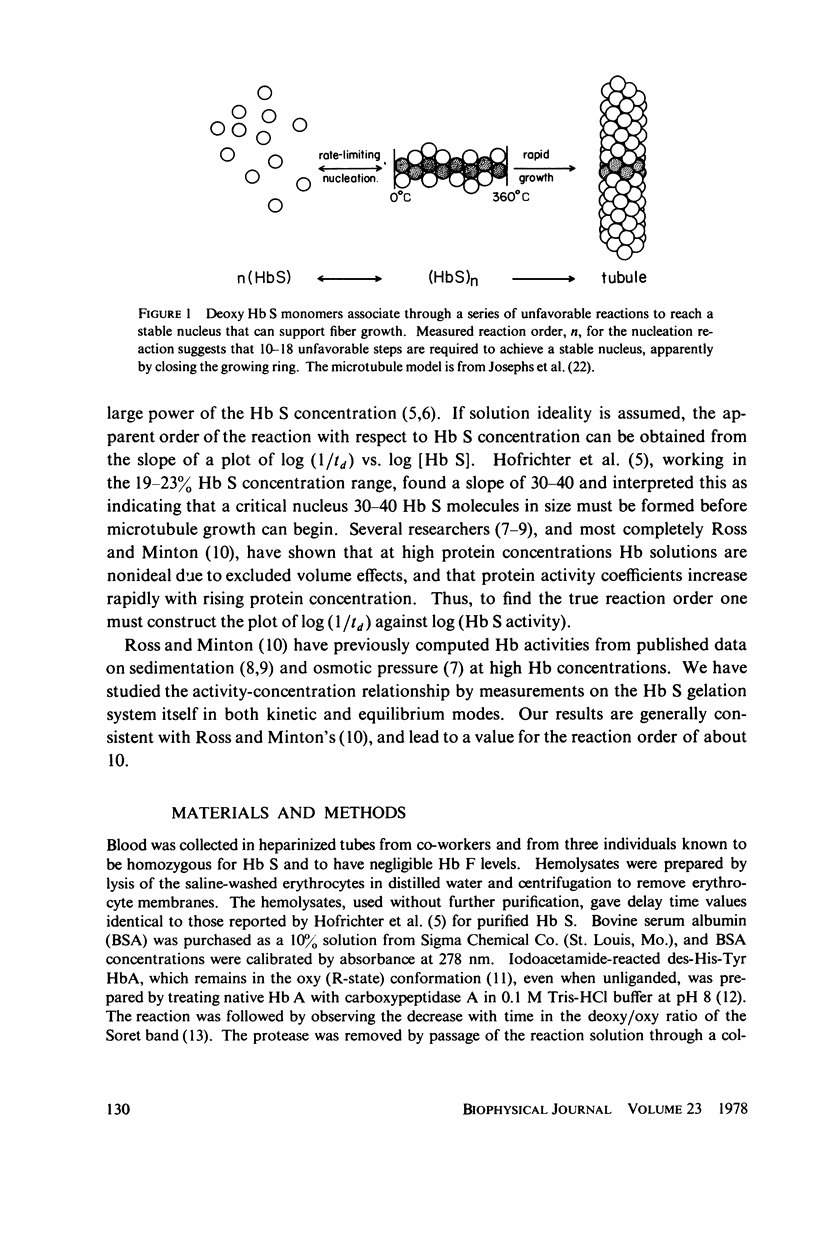
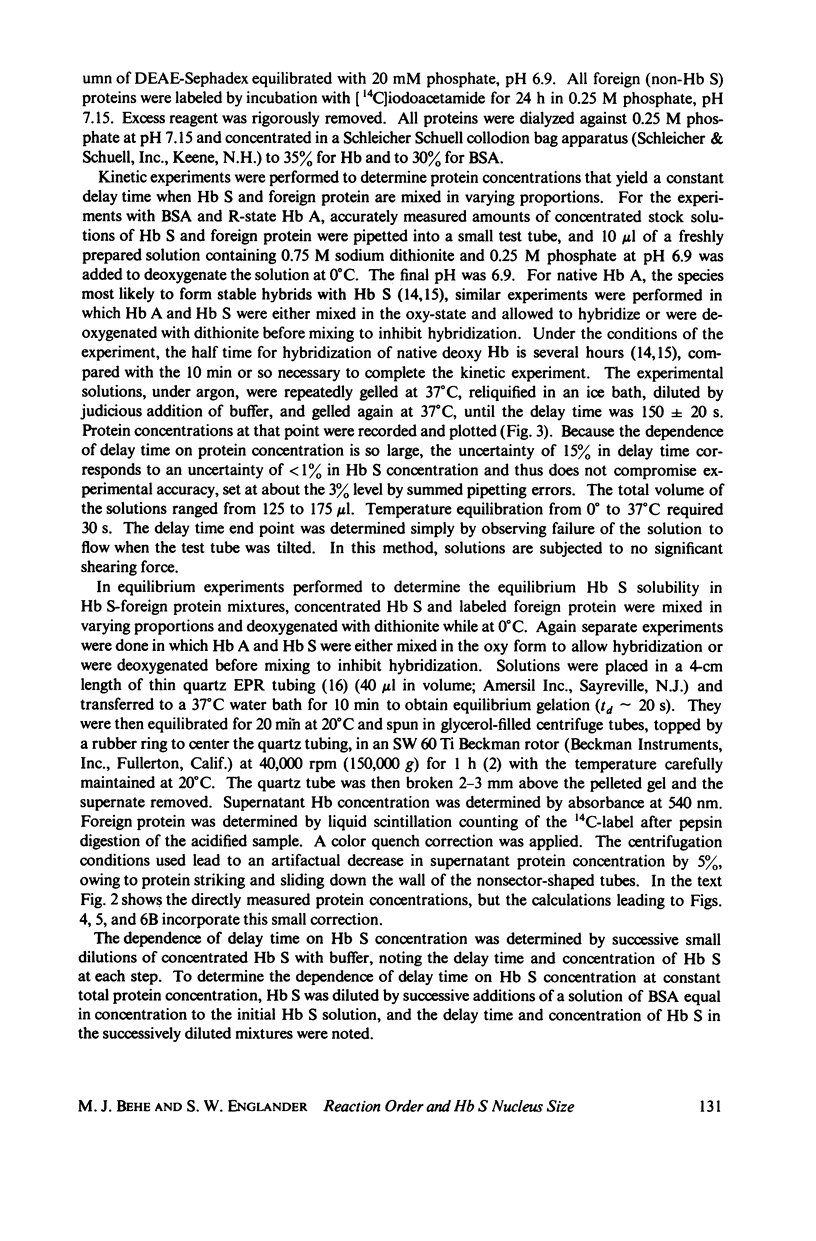
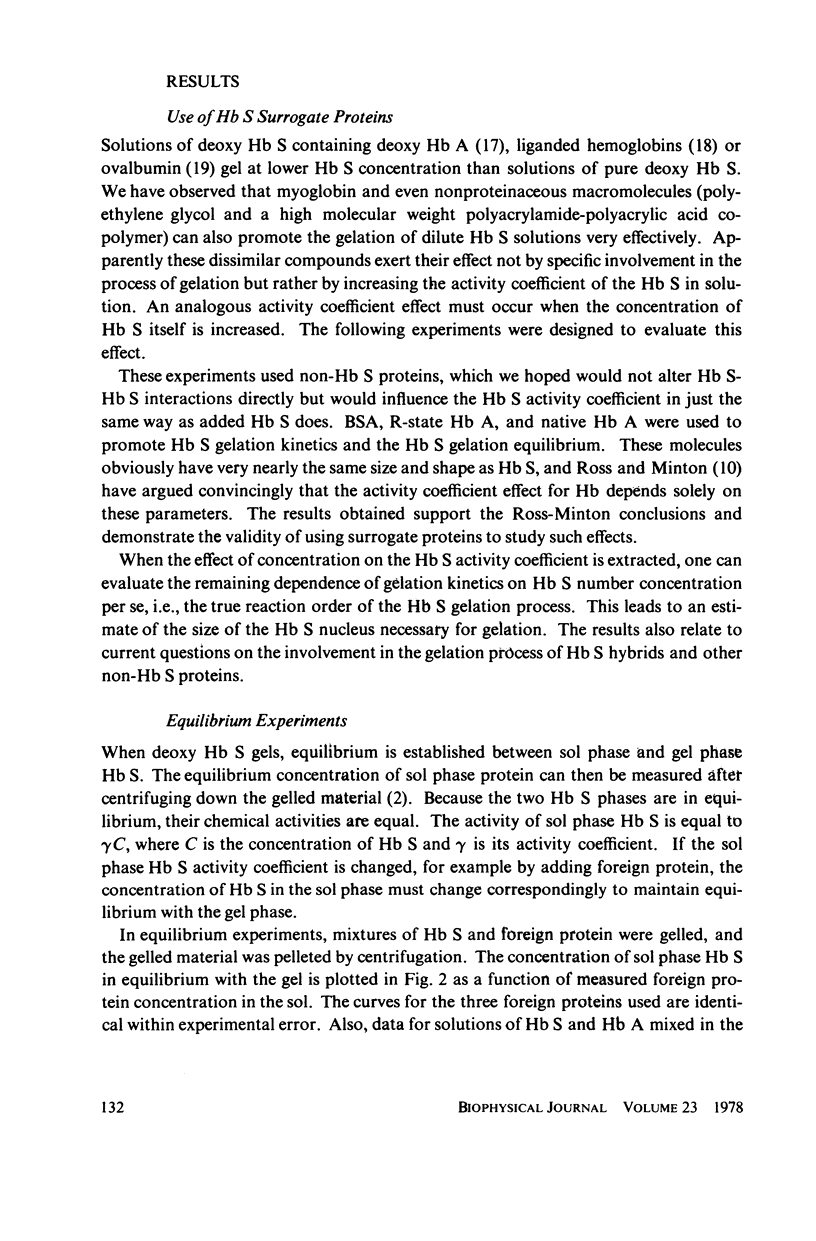
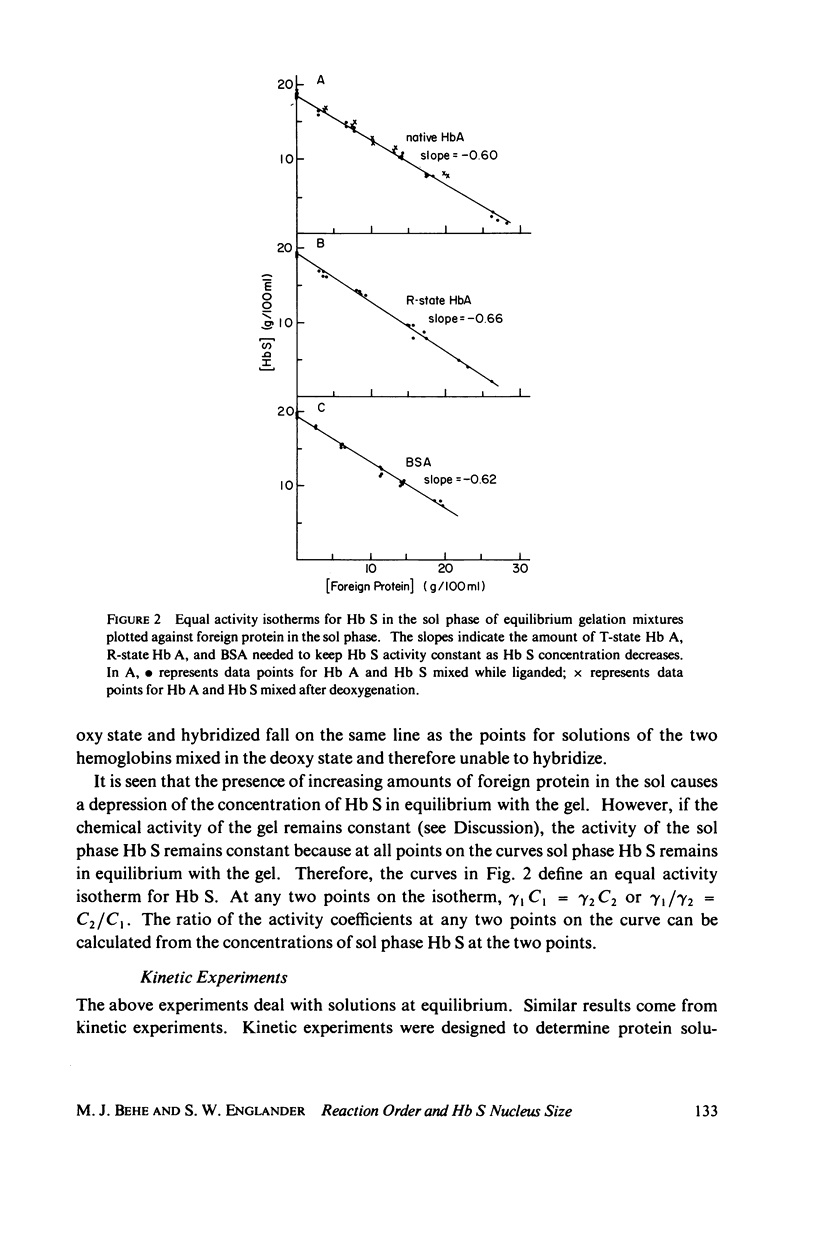
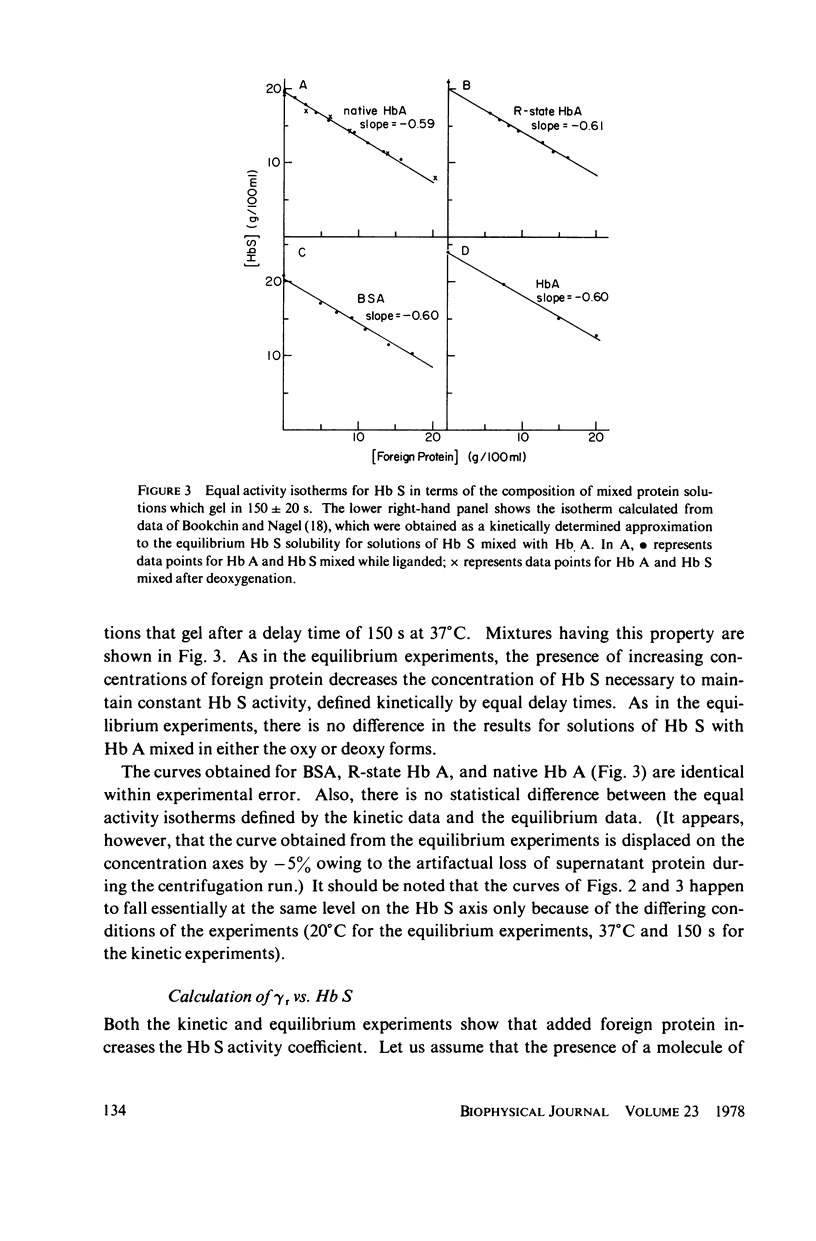
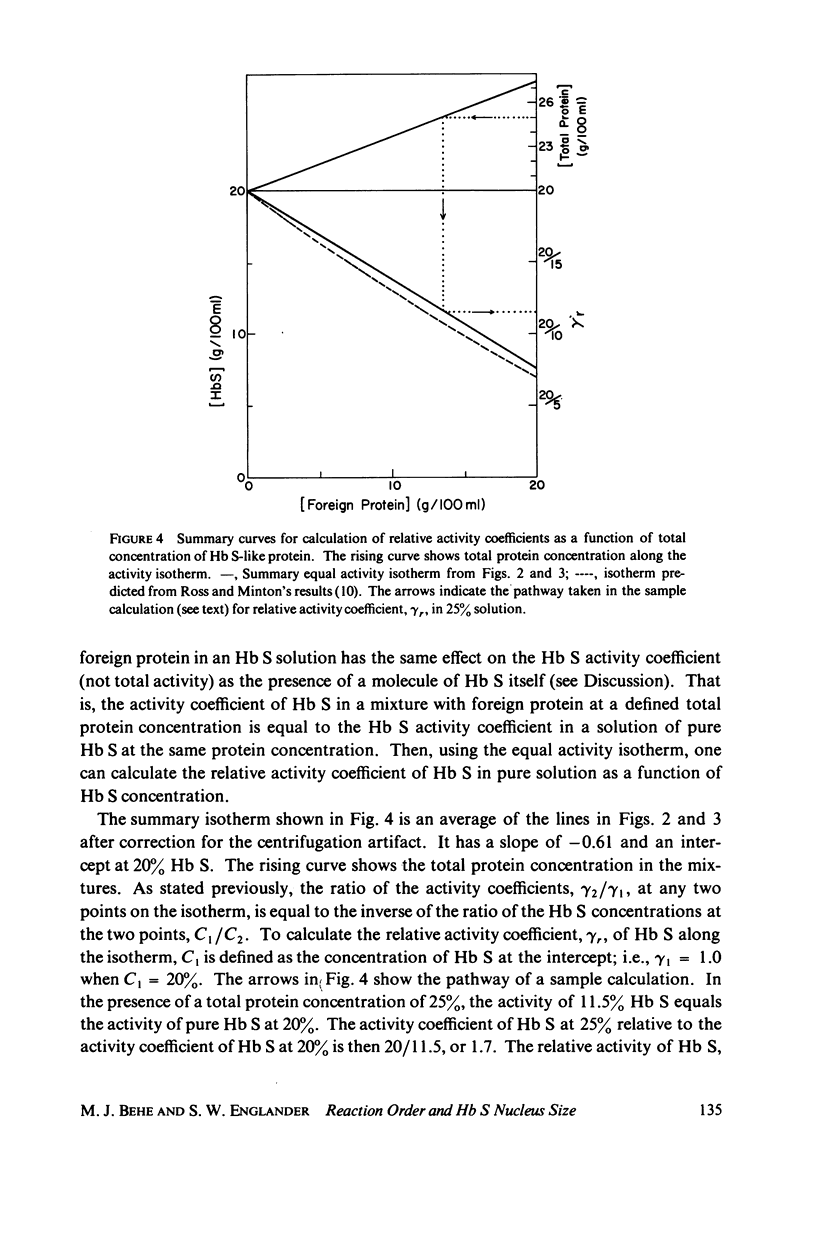
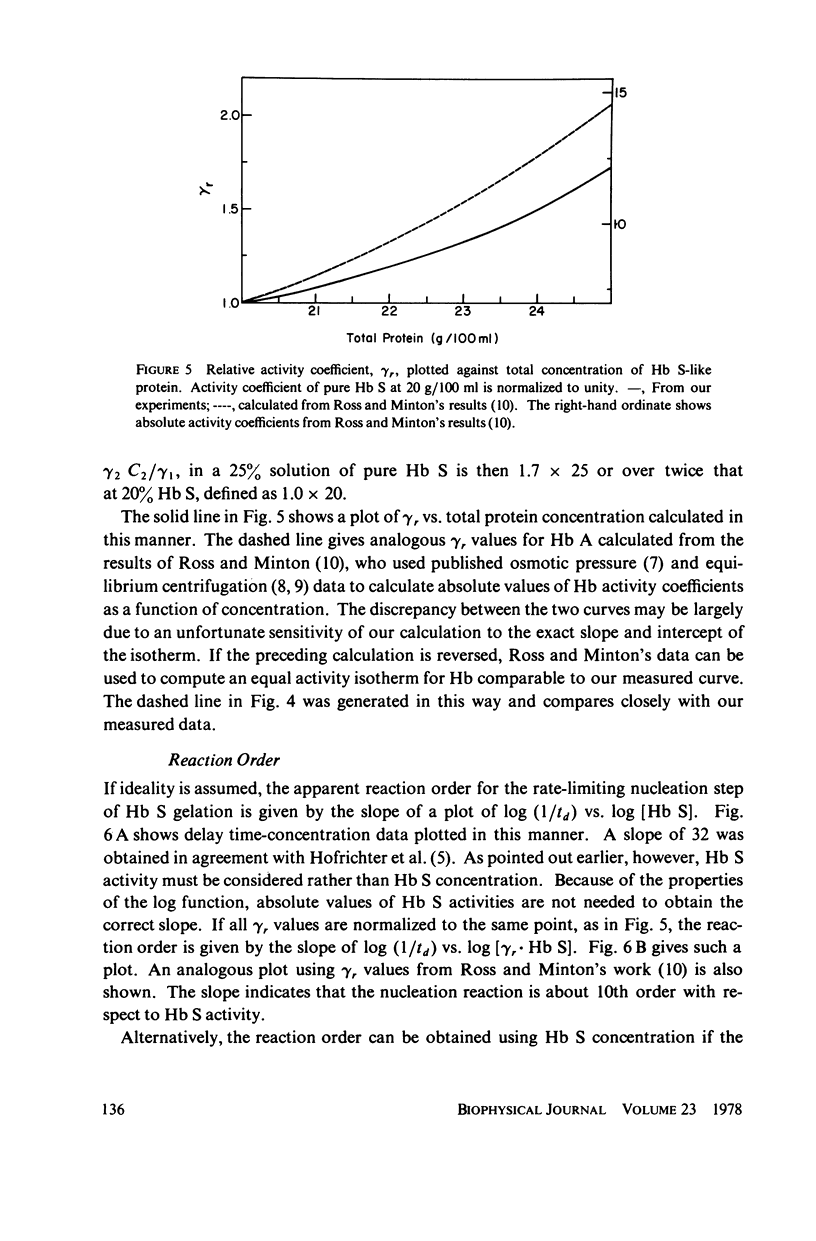
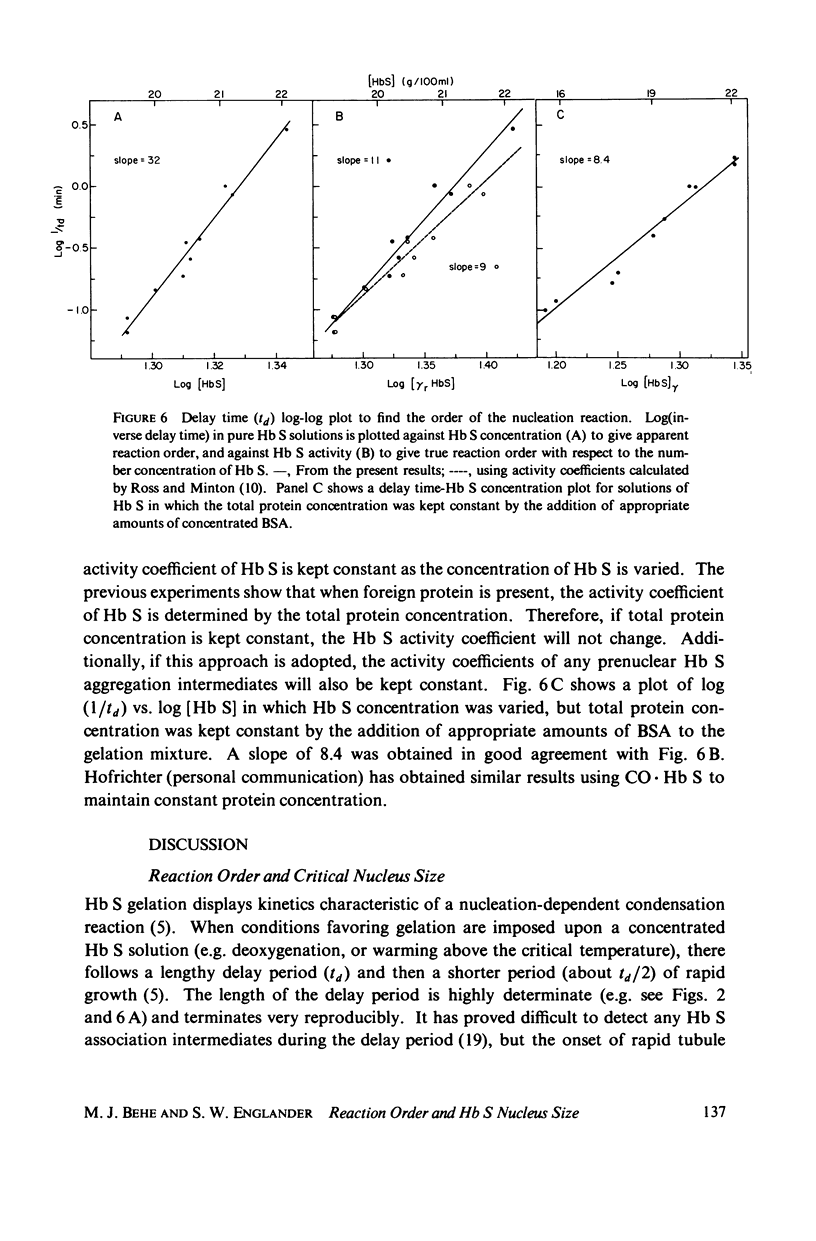
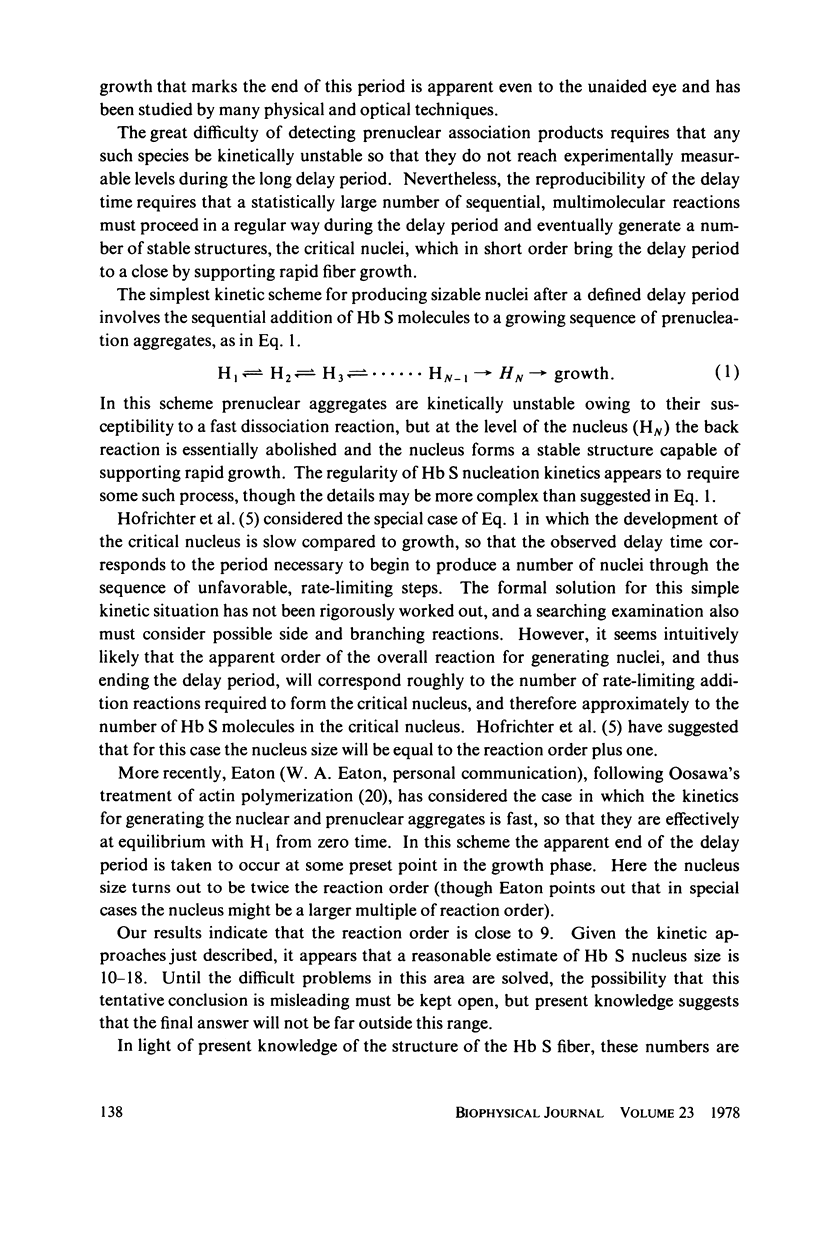
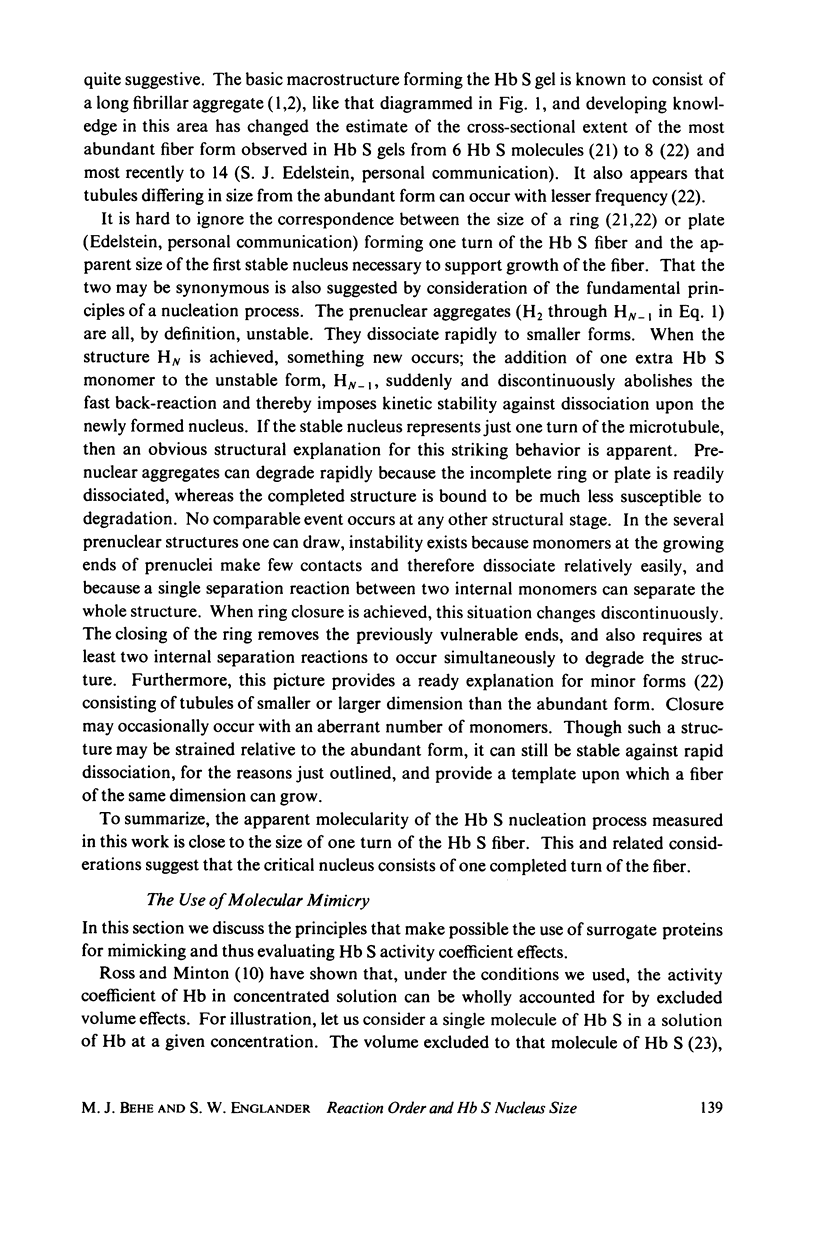
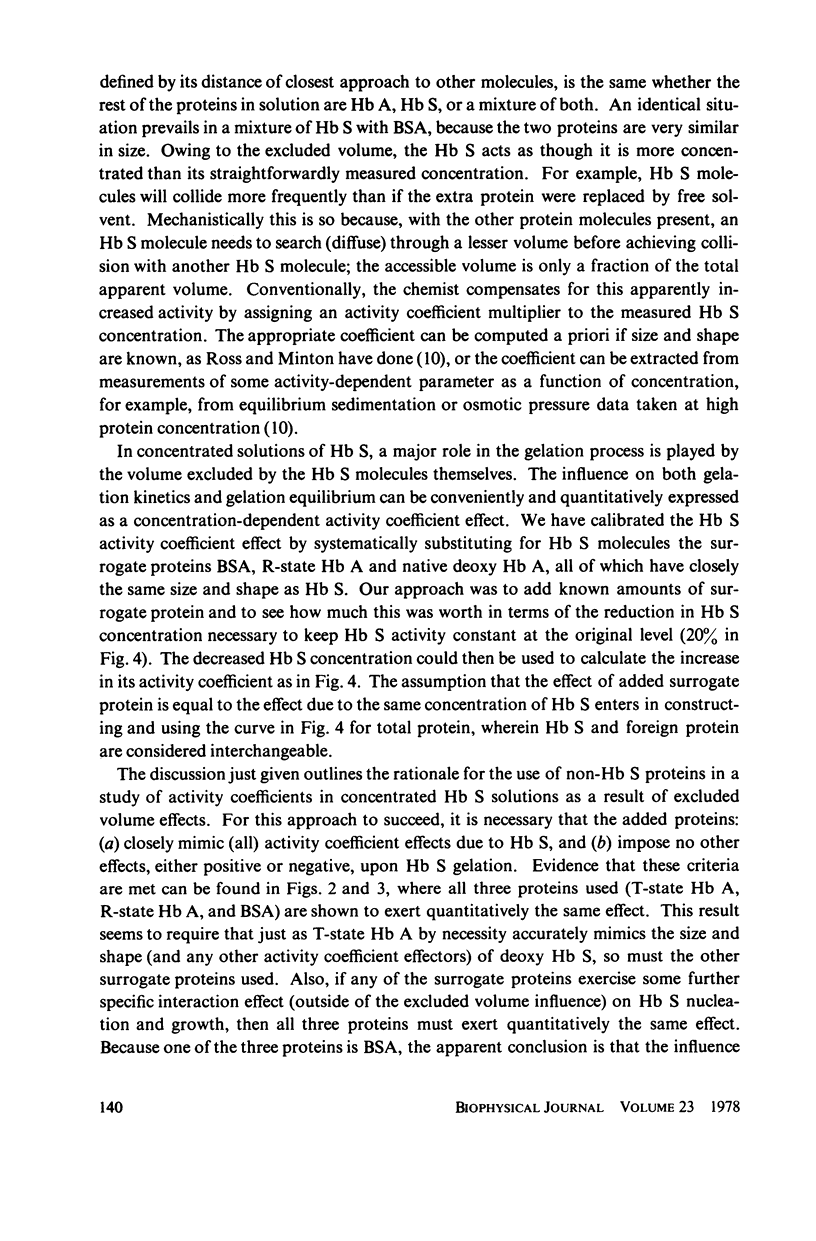
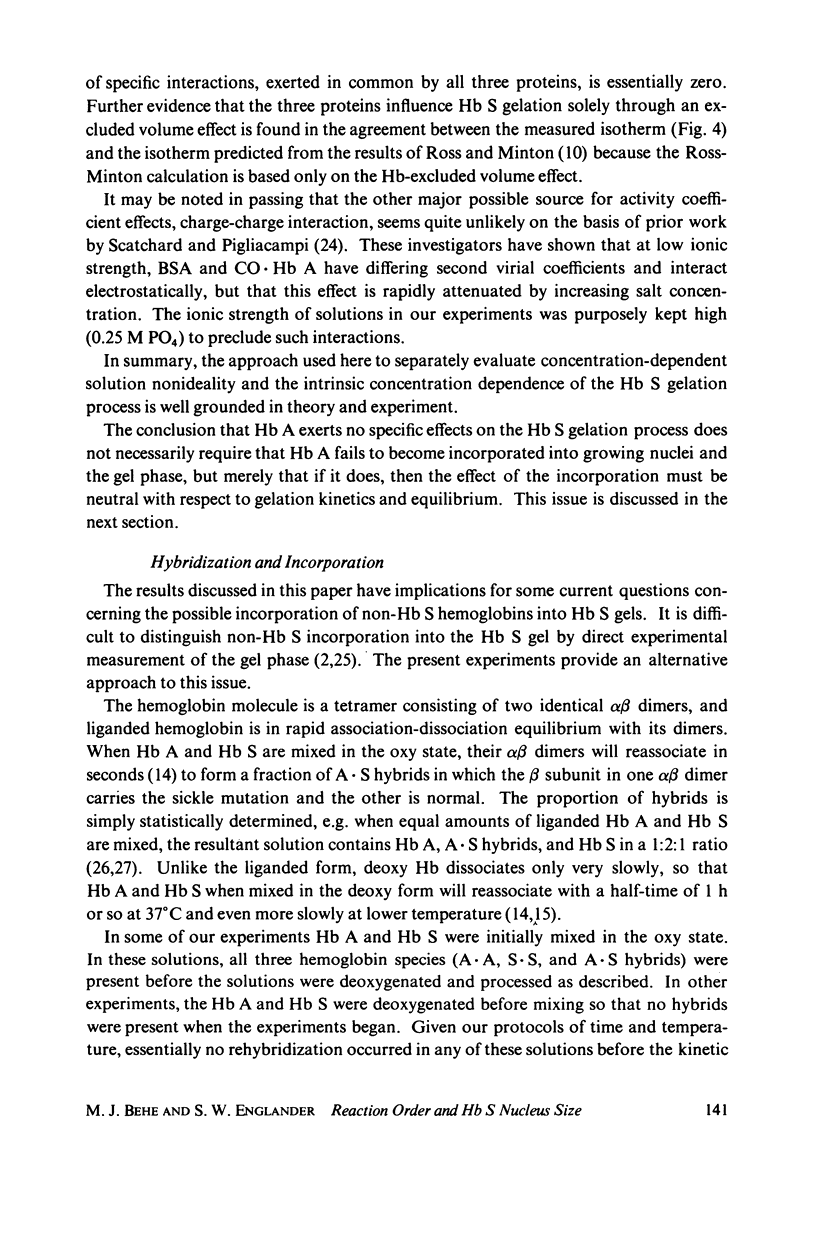
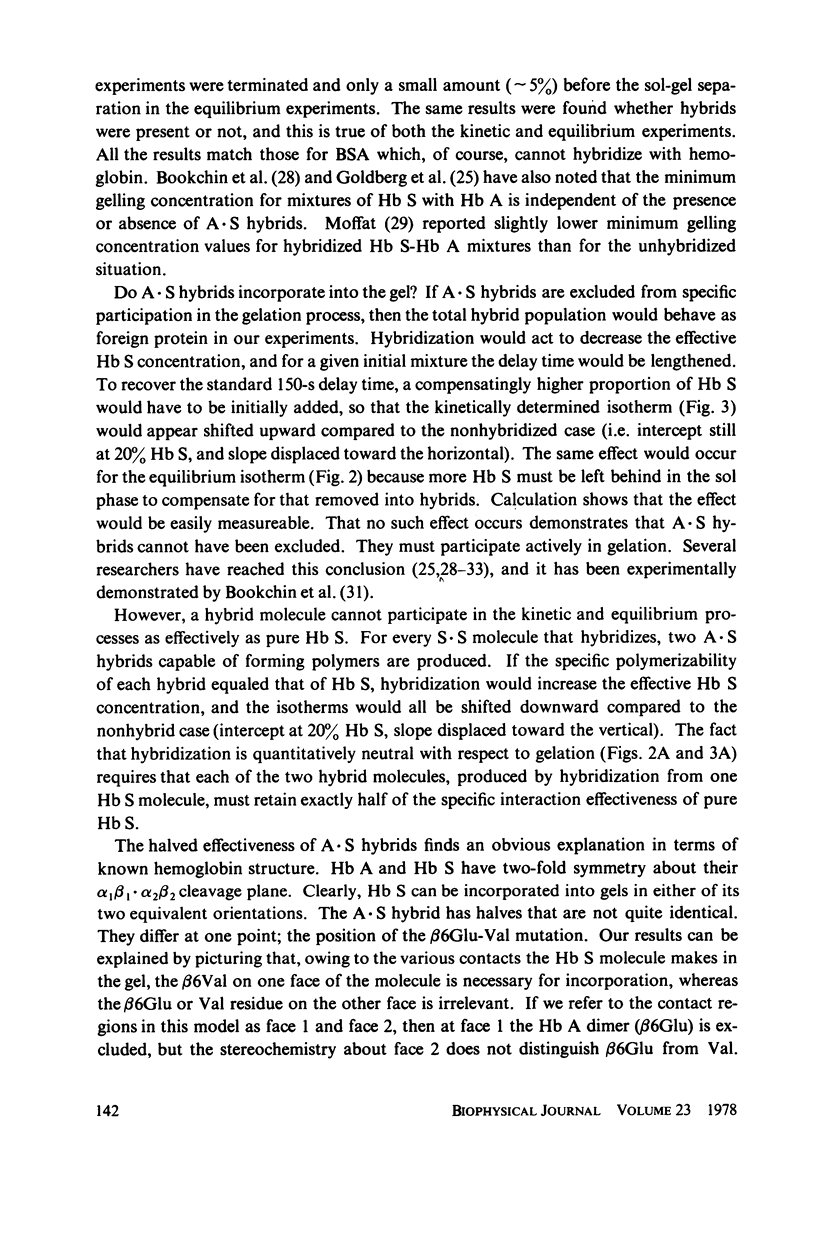
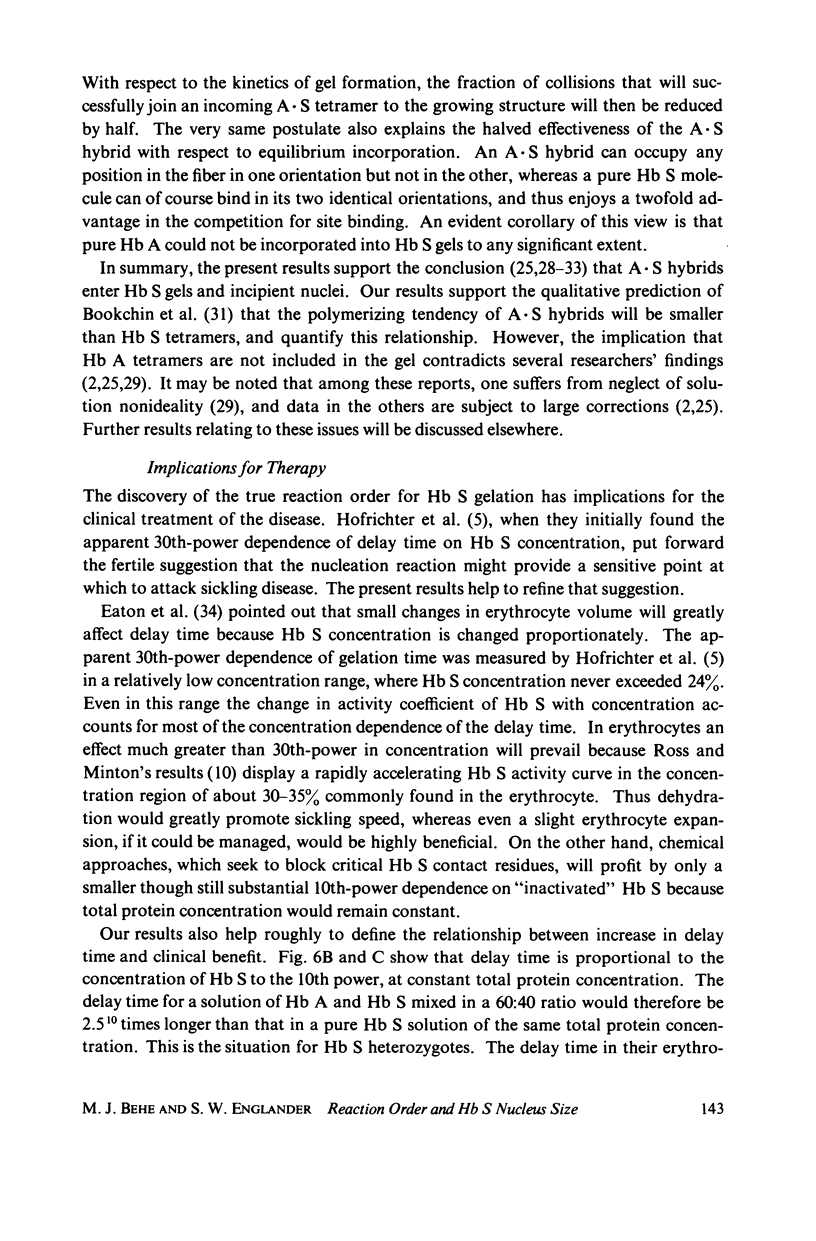
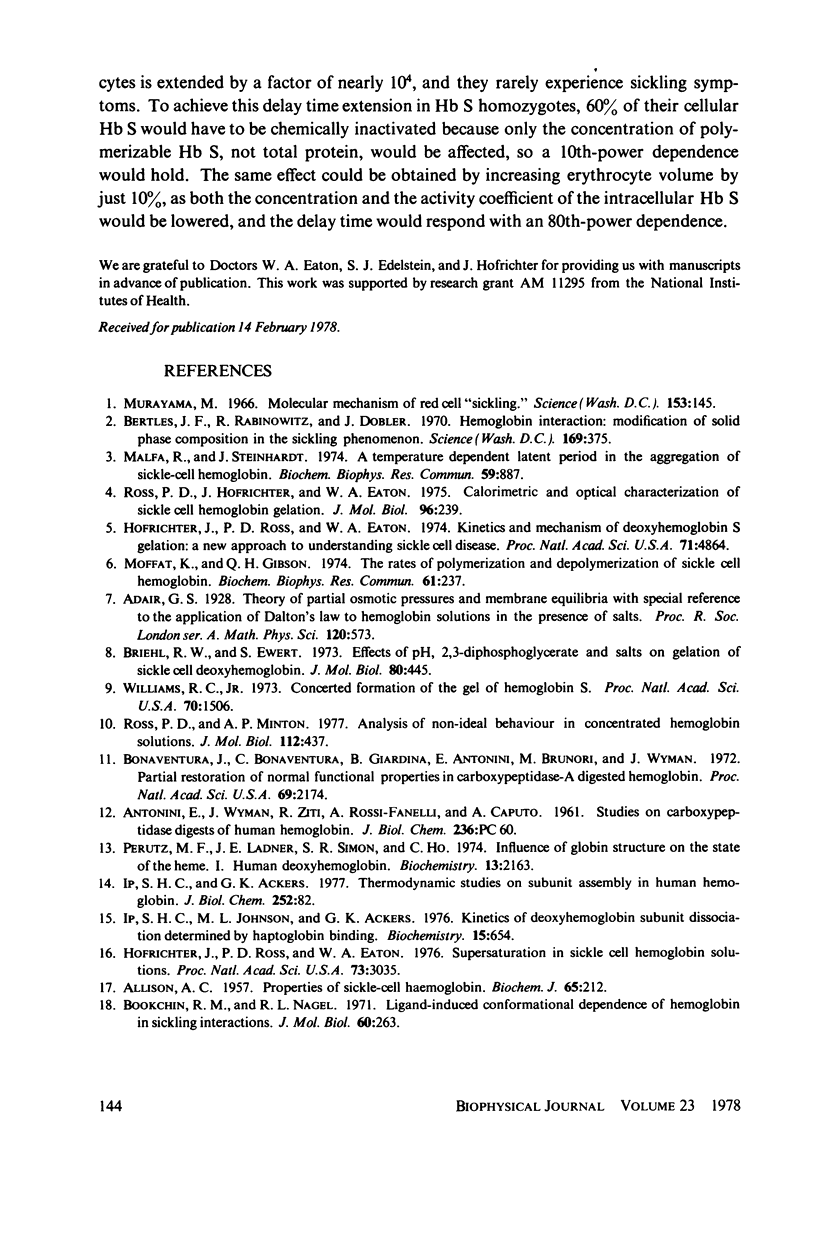
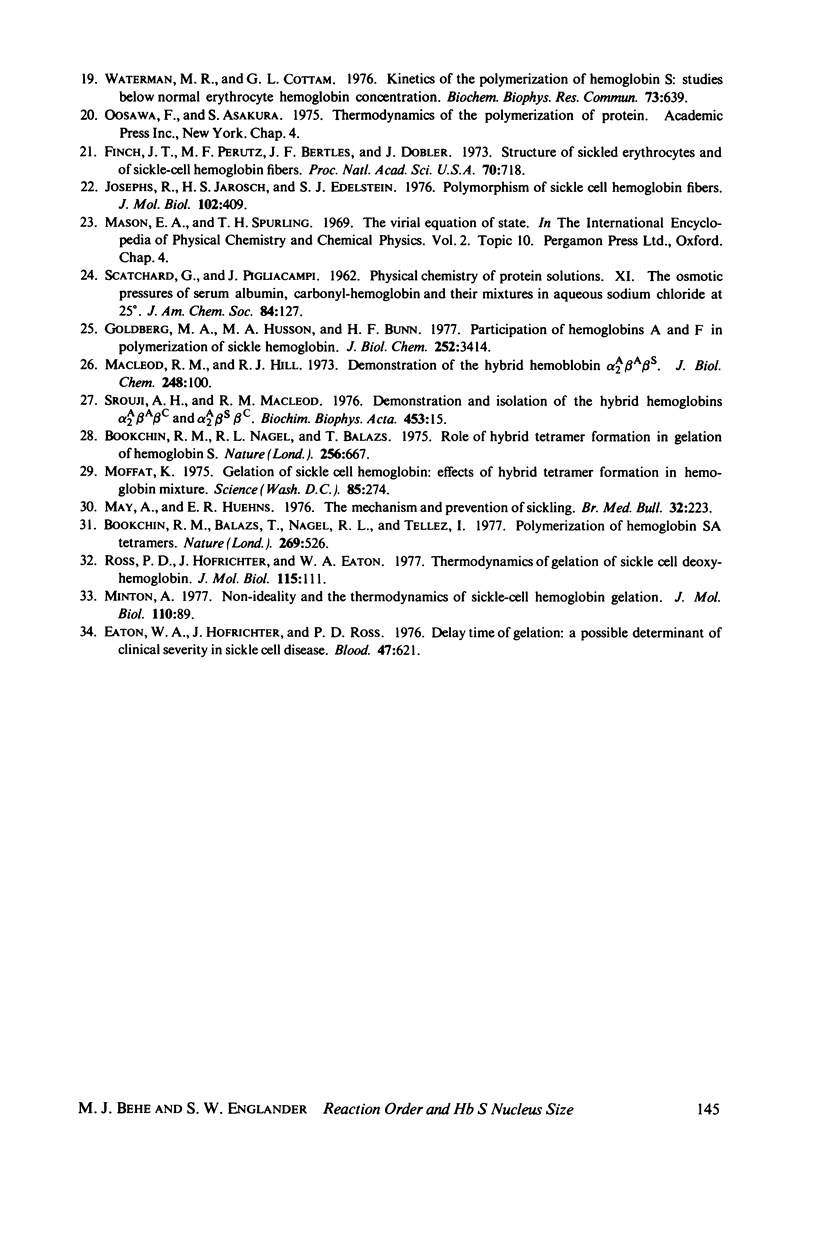
Selected References
These references are in PubMed. This may not be the complete list of references from this article.
- ALLISON A. C. Properties of sickle-cell haemoglobin. Biochem J. 1957 Feb;65(2):212–219. doi: 10.1042/bj0650212. [DOI] [PMC free article] [PubMed] [Google Scholar]
- Bertles J. F., Rabinowitz R., Döbler J. Hemoglobin interaction: modification of solid phase composition in the sickling phenomenon. Science. 1970 Jul 24;169(3943):375–377. doi: 10.1126/science.169.3943.375. [DOI] [PubMed] [Google Scholar]
- Bonaventura J., Bonaventura C., Giardina B., Antonini E., Brunori M., Wyman J. Partial restoration of normal functional properties in carboxypeptidase A-digested hemoglobin. Proc Natl Acad Sci U S A. 1972 Aug;69(8):2174–2178. doi: 10.1073/pnas.69.8.2174. [DOI] [PMC free article] [PubMed] [Google Scholar]
- Bookchin R. M., Balazs T., Nagel R. L., Tellez I. Polymerisation of haemoglobin SA hybrid tetramers. Nature. 1977 Oct 6;269(5628):526–527. doi: 10.1038/269526a0. [DOI] [PubMed] [Google Scholar]
- Bookchin R. M., Nagel R. L., Balazs T. Role of hybrid tetramer formation in gelation of haemoglobin S. Nature. 1975 Aug 21;256(5519):667–668. doi: 10.1038/256667a0. [DOI] [PubMed] [Google Scholar]
- Bookchin R. M., Nagel R. L. Ligand-induced conformational dependence of hemoglobin in sickling interactios. J Mol Biol. 1971 Sep 14;60(2):263–270. doi: 10.1016/0022-2836(71)90292-0. [DOI] [PubMed] [Google Scholar]
- Briehl R. W., Ewert S. Effects of pH, 2,3-diphosphoglycerate and salts on gelation of sickle cell deoxyhemoglobin. J Mol Biol. 1973 Nov 5;80(3):445–458. doi: 10.1016/0022-2836(73)90415-4. [DOI] [PubMed] [Google Scholar]
- Finch J. T., Perutz M. F., Bertles J. F., Döbler J. Structure of sickled erythrocytes and of sickle-cell hemoglobin fibers. Proc Natl Acad Sci U S A. 1973 Mar;70(3):718–722. doi: 10.1073/pnas.70.3.718. [DOI] [PMC free article] [PubMed] [Google Scholar]
- Goldberg M. A., Husson M. A., Bunn H. F. Participation of hemoglobins A and F in polymerization of sickle hemoglobin. J Biol Chem. 1977 May 25;252(10):3414–3421. [PubMed] [Google Scholar]
- Hofrichter J., Ross P. D., Eaton W. A. Kinetics and mechanism of deoxyhemoglobin S gelation: a new approach to understanding sickle cell disease. Proc Natl Acad Sci U S A. 1974 Dec;71(12):4864–4868. doi: 10.1073/pnas.71.12.4864. [DOI] [PMC free article] [PubMed] [Google Scholar]
- Hofrichter J., Ross P. D., Eaton W. A. Supersaturation in sickle cell hemoglobin solutions. Proc Natl Acad Sci U S A. 1976 Sep;73(9):3035–3039. doi: 10.1073/pnas.73.9.3035. [DOI] [PMC free article] [PubMed] [Google Scholar]
- Ip S. H., Ackers G. K. Thermodynamic studies on subunit assembly in human hemoglobin. Temperature dependence of the dimer-tetramer association constants for oxygenated and unliganded hemoglobins. J Biol Chem. 1977 Jan 10;252(1):82–87. [PubMed] [Google Scholar]
- Ip S. H., Johnson M. L., Ackers G. K. Kinetics of deoxyhemoglobin subunit dissociation determined by haptoglobin binding: estimation of the equilibrium constant from forward and reverse rates. Biochemistry. 1976 Feb 10;15(3):654–660. doi: 10.1021/bi00648a032. [DOI] [PubMed] [Google Scholar]
- Josephs R., Jarosch H. S., Edelstein S. J. Polymorphism of sickle cell hemoglobin fibers. J Mol Biol. 1976 Apr 15;102(3):409–426. doi: 10.1016/0022-2836(76)90324-7. [DOI] [PubMed] [Google Scholar]
- Macleod R. M., Hill R. J. Demonstration of the hybrid hemoglobin 2 A A S . J Biol Chem. 1973 Jan 10;248(1):100–103. [PubMed] [Google Scholar]
- Malfa R., Steinhardt J. A temperature-dependent latent-period in the aggregation of sickle-cell deoxyhemoglobin. Biochem Biophys Res Commun. 1974 Aug 5;59(3):887–893. doi: 10.1016/s0006-291x(74)80062-8. [DOI] [PubMed] [Google Scholar]
- May A., Huehns E. R. The mechanism and prevention of sickling. Br Med Bull. 1976 Sep;32(3):223–233. doi: 10.1093/oxfordjournals.bmb.a071367. [DOI] [PubMed] [Google Scholar]
- Minton A. P. Non-ideality and the thermodynamics of sickle-cell hemoglobin gelation. J Mol Biol. 1977 Feb 15;110(1):89–103. doi: 10.1016/s0022-2836(77)80100-9. [DOI] [PubMed] [Google Scholar]
- Moffat K., Gibson Q. H. The rates of polymerization and depolymerization of sickle cell hemoglobin. Biochem Biophys Res Commun. 1974 Nov 6;61(1):237–242. doi: 10.1016/0006-291x(74)90558-0. [DOI] [PubMed] [Google Scholar]
- Murayama M. Molecular mechanism of red cell "sickling". Science. 1966 Jul 8;153(3732):145–149. doi: 10.1126/science.153.3732.145. [DOI] [PubMed] [Google Scholar]
- Perutz M. F., Ladner J. E., Simon S. R., Ho C. Influence of globin structure on the state of the heme. I. Human deoxyhemoglobin. Biochemistry. 1974 May 7;13(10):2163–2173. doi: 10.1021/bi00707a026. [DOI] [PubMed] [Google Scholar]
- Ross P. D., Hofrichter J., Eaton W. A. Calorimetric and optical characterization of sickle cell hemoglobin gelation. J Mol Biol. 1975 Aug 5;96(2):239–253. doi: 10.1016/0022-2836(75)90345-9. [DOI] [PubMed] [Google Scholar]
- Ross P. D., Minton A. P. Analysis of non-ideal behavior in concentrated hemoglobin solutions. J Mol Biol. 1977 May 25;112(3):437–452. doi: 10.1016/s0022-2836(77)80191-5. [DOI] [PubMed] [Google Scholar]
- Srouji A. H., Macleod R. M. Demonstration and isolation of the hybrid hemoglobins alpha 2 A beta A beta C and alpha 2 A beta S beta C. Biochim Biophys Acta. 1976 Nov 26;453(1):15–25. doi: 10.1016/0005-2795(76)90246-4. [DOI] [PubMed] [Google Scholar]
- Waterman M. R., Cottam G. L. Kinetics of the polymerization of hemoglobin S: studies below normal erythrocyte hemoglobin concentration. Biochem Biophys Res Commun. 1976 Dec 6;73(3):639–645. doi: 10.1016/0006-291x(76)90858-5. [DOI] [PubMed] [Google Scholar]
- Williams R. C., Jr Concerted formation of the gel of hemoglobin S. Proc Natl Acad Sci U S A. 1973 May;70(5):1506–1508. doi: 10.1073/pnas.70.5.1506. [DOI] [PMC free article] [PubMed] [Google Scholar]