Abstract
Initial energy utilization in the twitch is visualized as the result of the activity of two distinct processes. The first is the calcium-pumping activity of the sarcoplasmic reticulum, which has a constant energy requirement under normal conditions. The second is the chemomechanical transduction process consisting of a variable number of quantal contractile events, each with a fixed enthalpy equal to the molecular enthalpy of adenosine triphosphate (ATP) hydrolysis in vivo. This enthalpy appears either as heat or as contractile element work. Total enthalpy varies according to the number of quantal contractile events that occur in the twitch cycle. The basis of the variation is suggested to be velocity-dependent activity of the actomyosin ATPase, allowing more quantal events to occur in a contraction cycle when shortening occurs. The classical designation “activation heat” is held to be appropriate for the first process. The partition of the enthalpy of the second process that is currently in vogue is held to be misleading and a new formulation is suggested in which the properties of the quantal contractile event are reflected in general terms. The formulation of the proposed transduction model represents a conceptual return to the viscoelastic theory, but at a quantal level. The model can explain the results of the preceding paper and is adaptable to different muscles without having to postulate fundamental differences in energy utilization.
Full text
PDF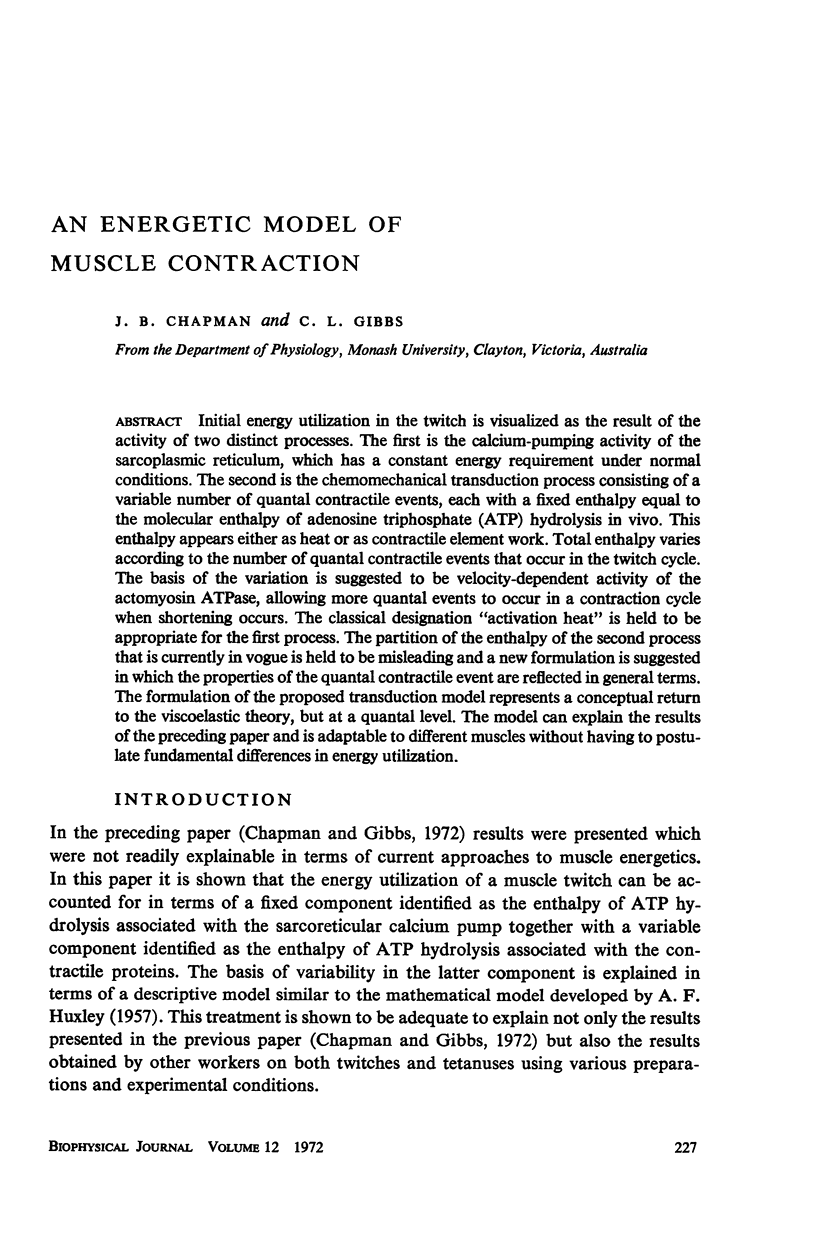
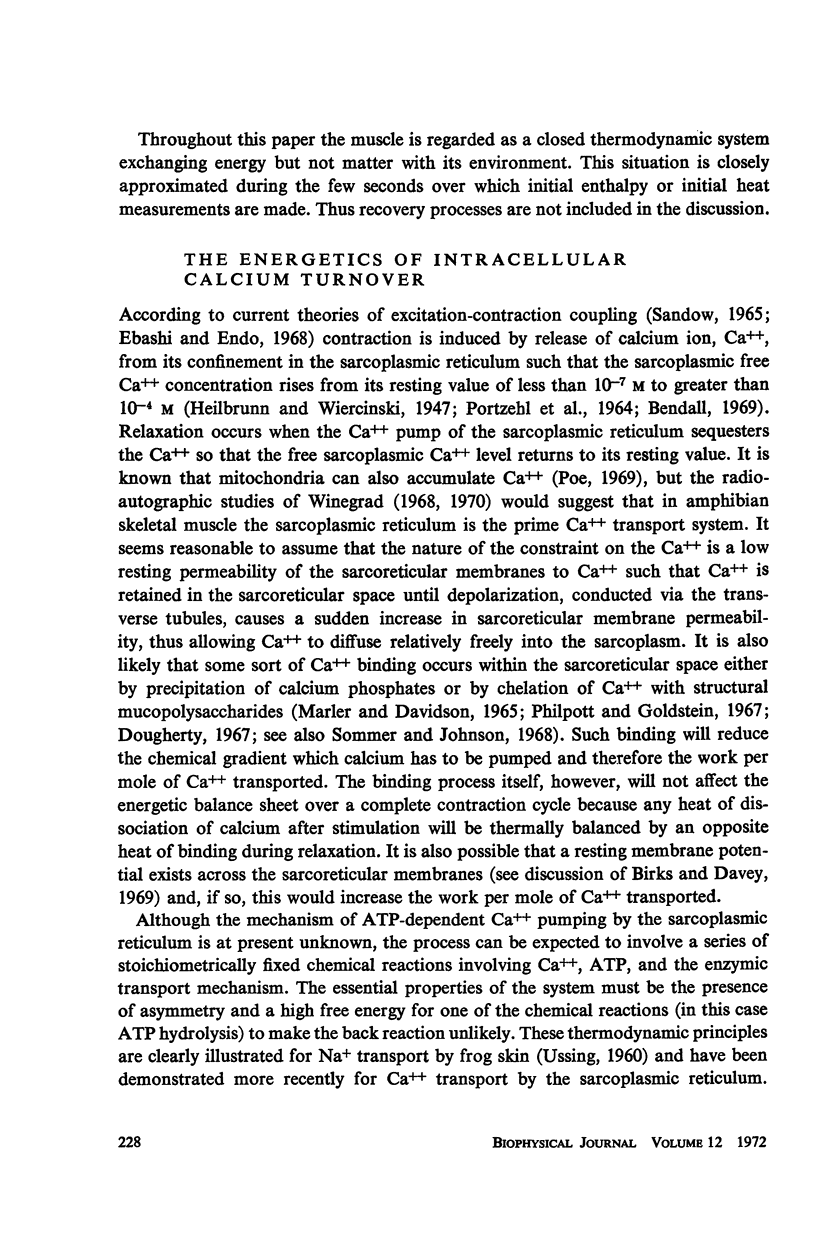
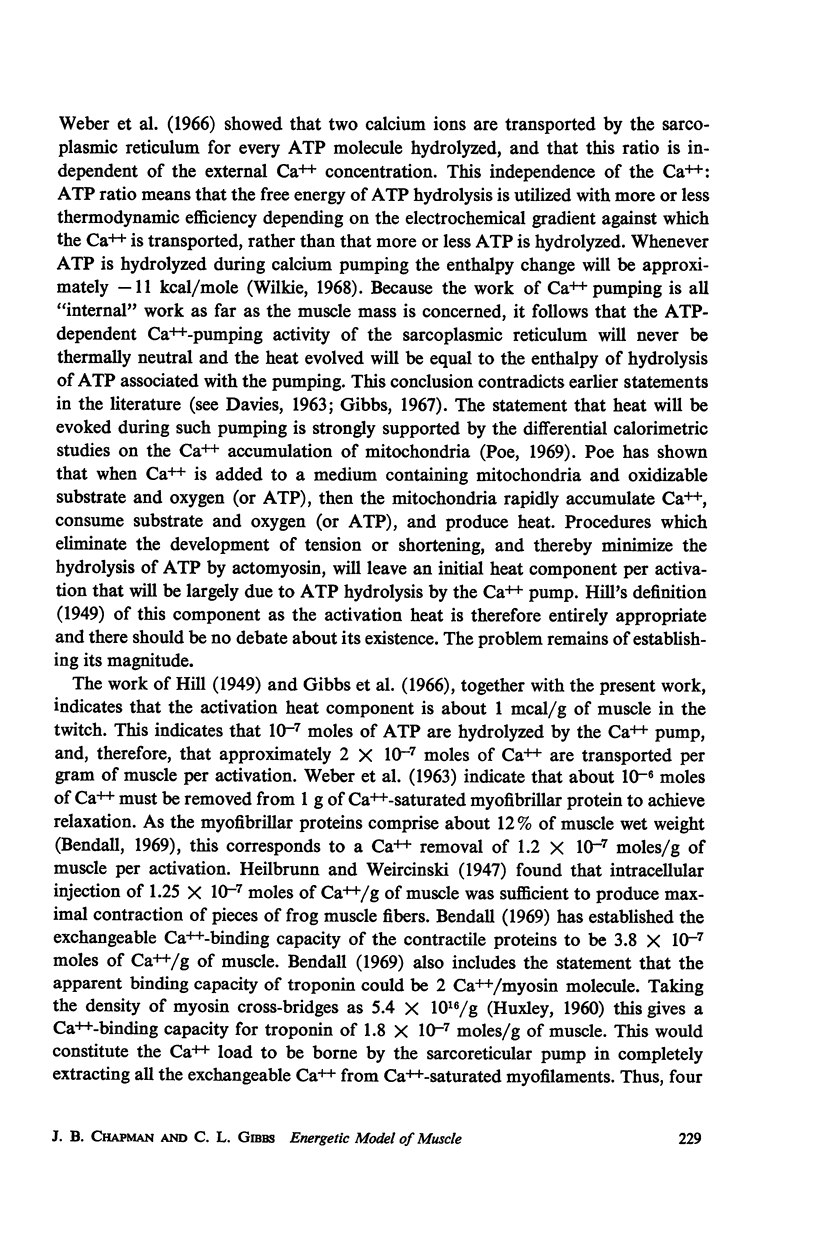
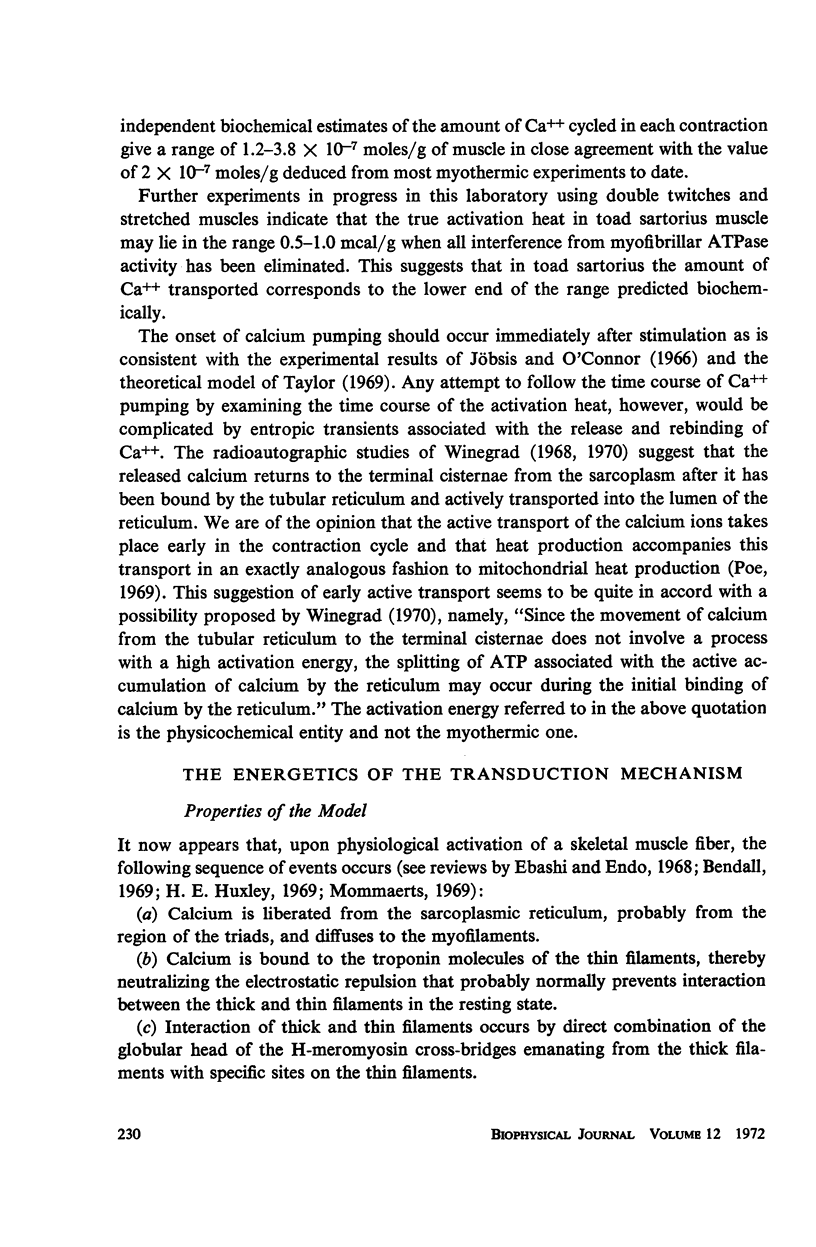
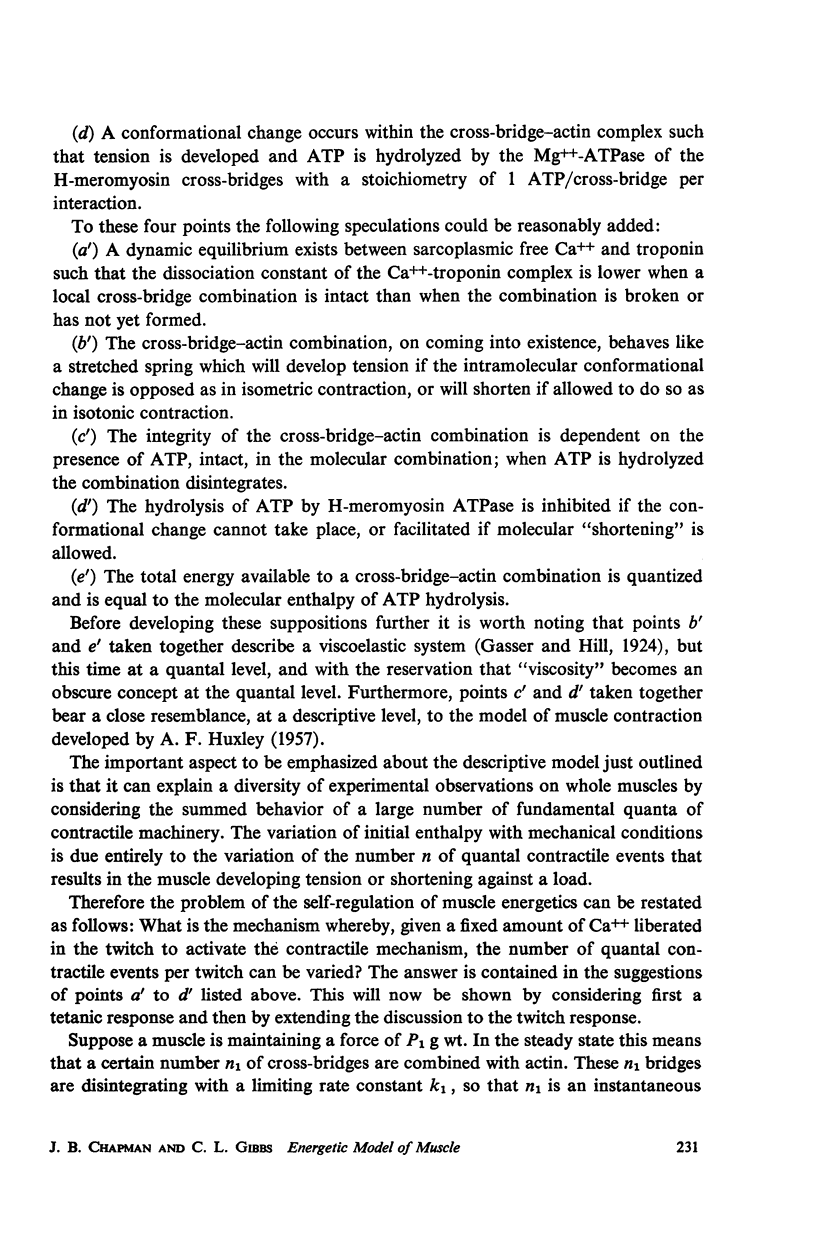
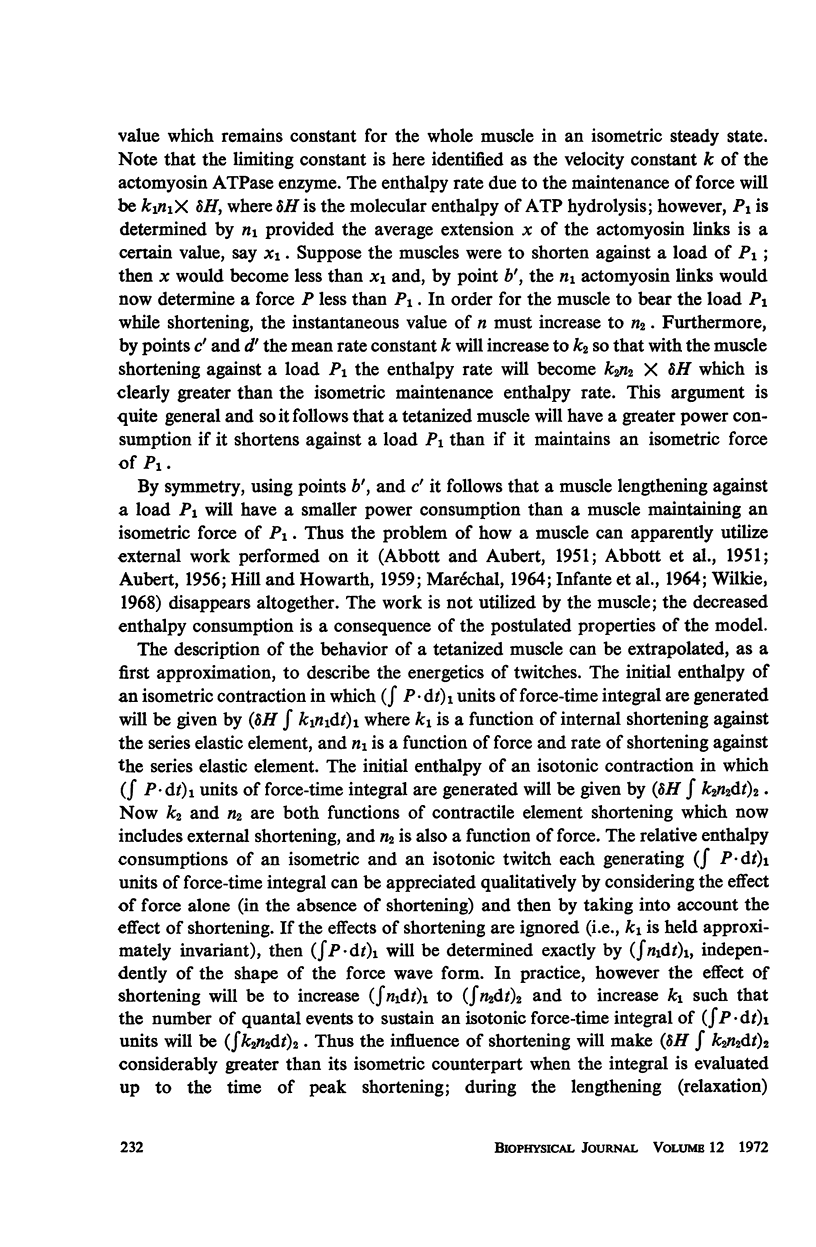
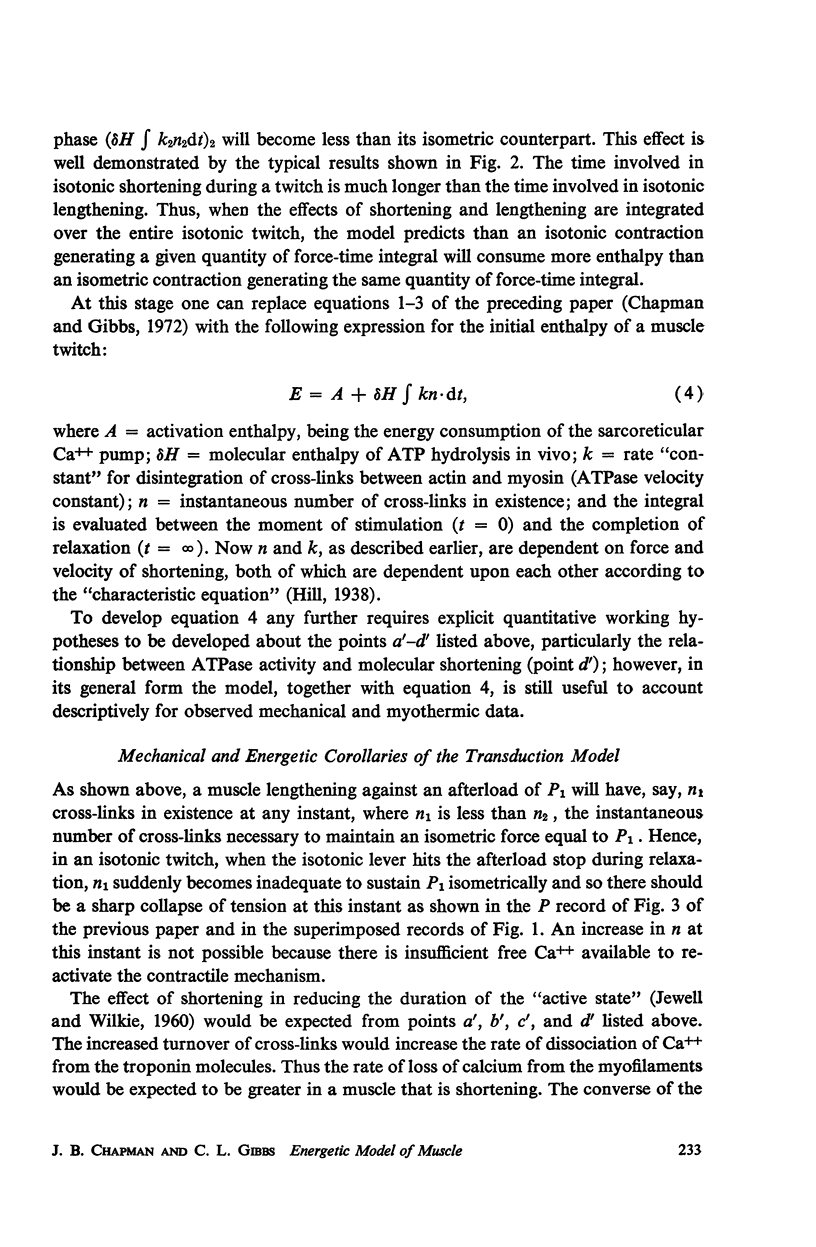
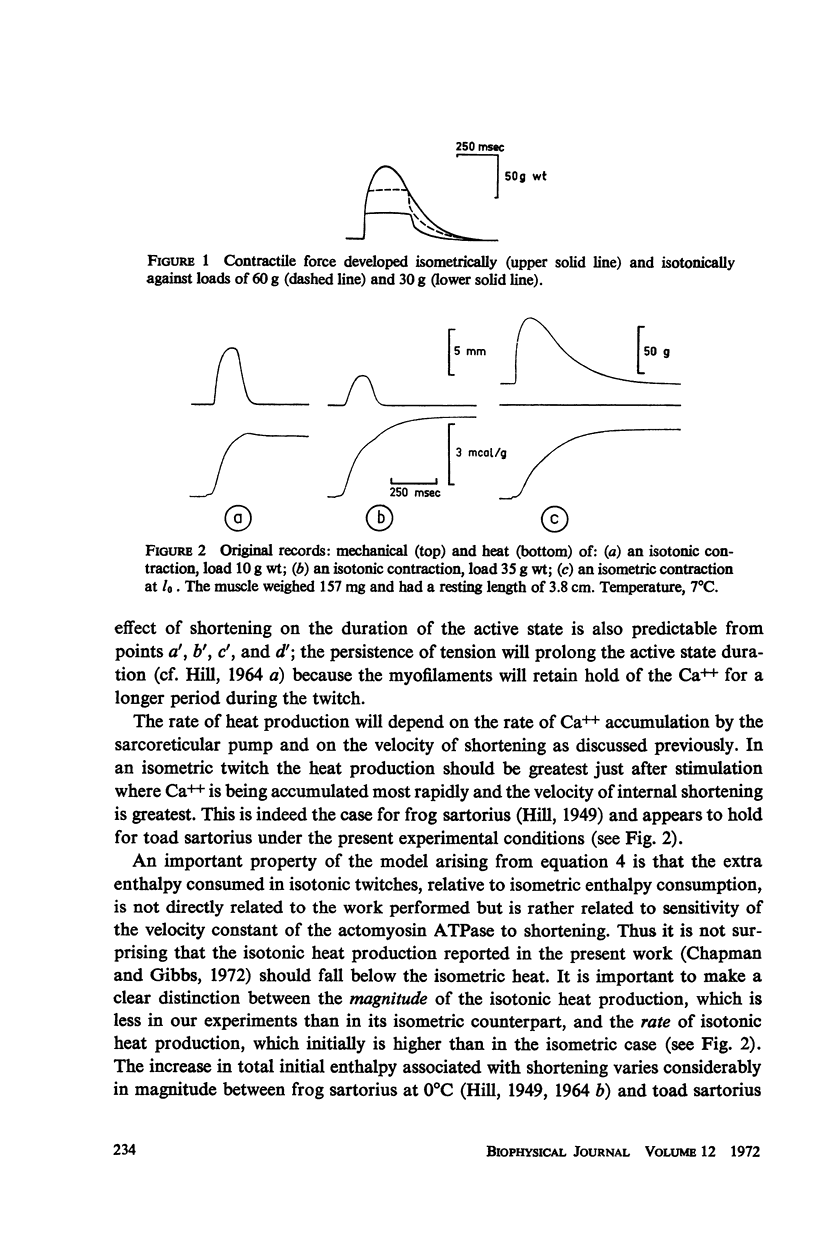
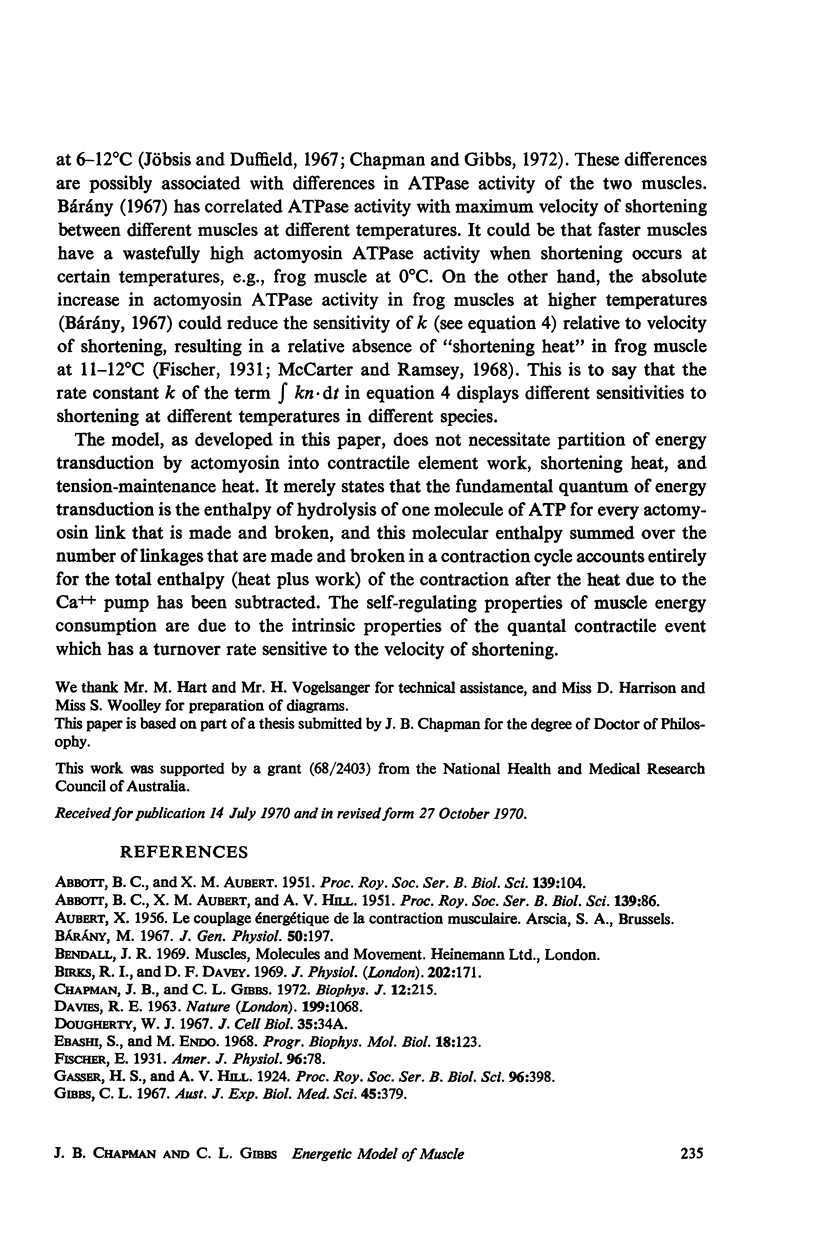
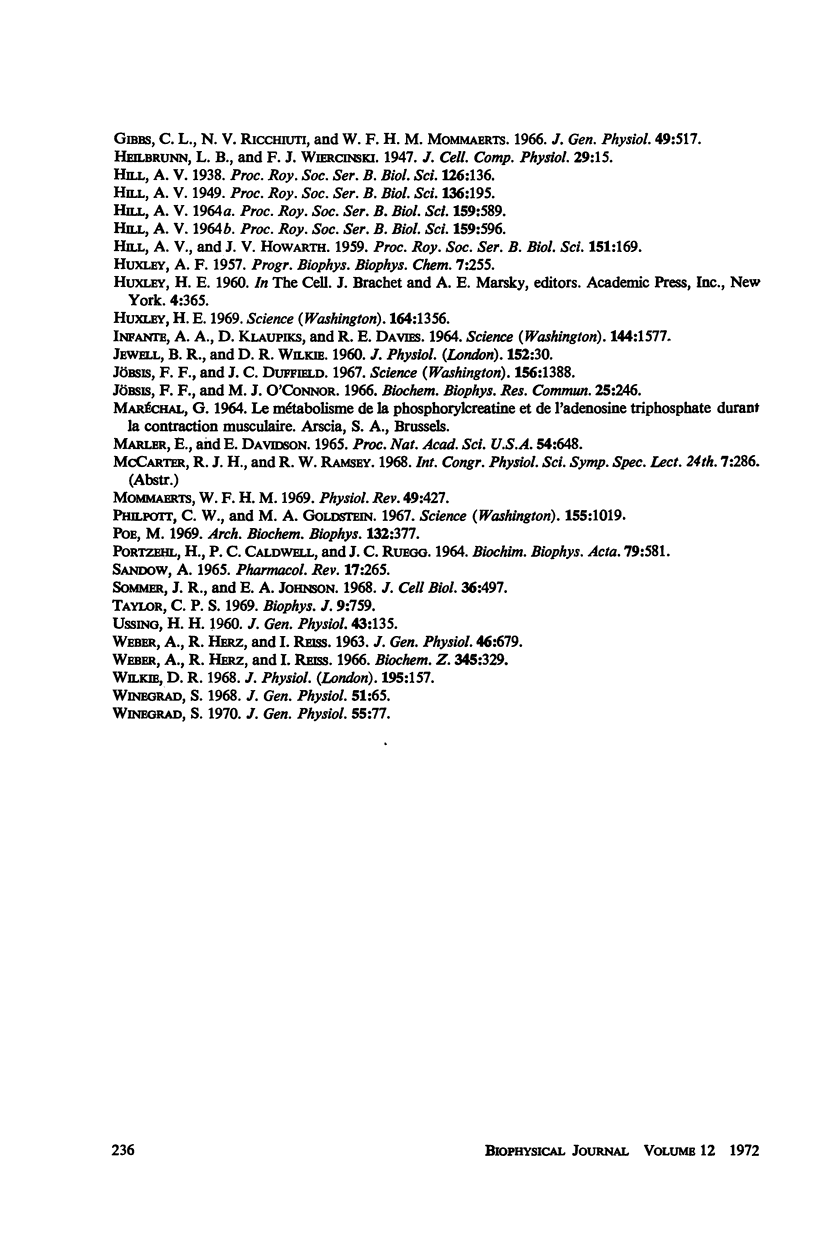
Selected References
These references are in PubMed. This may not be the complete list of references from this article.
- ABBOTT B. C., AUBERT X. M., HILL A. V. The absorption of work by a muscle stretched during a single twitch or a short tetanus. Proc R Soc Lond B Biol Sci. 1951 Dec 31;139(894):86–104. doi: 10.1098/rspb.1951.0048. [DOI] [PubMed] [Google Scholar]
- Birks R. I., Davey D. F. Osmotic responses demonstrating the extracellular character of the sarcoplasmic reticulum. J Physiol. 1969 May;202(1):171–188. doi: 10.1113/jphysiol.1969.sp008802. [DOI] [PMC free article] [PubMed] [Google Scholar]
- Bárány M. ATPase activity of myosin correlated with speed of muscle shortening. J Gen Physiol. 1967 Jul;50(6 Suppl):197–218. doi: 10.1085/jgp.50.6.197. [DOI] [PMC free article] [PubMed] [Google Scholar]
- Chapman J. B., Gibbs C. L. Energetics of isometric and isotonic twitches in toad sartorius. Biophys J. 1972 Mar;12(3):215–226. doi: 10.1016/S0006-3495(72)86081-8. [DOI] [PMC free article] [PubMed] [Google Scholar]
- Ebashi S., Endo M. Calcium ion and muscle contraction. Prog Biophys Mol Biol. 1968;18:123–183. doi: 10.1016/0079-6107(68)90023-0. [DOI] [PubMed] [Google Scholar]
- Gibbs C. L., Ricchiuti N. V., Mommaerts W. F. Activation heat in frog sartorius muscle. J Gen Physiol. 1966 Jan;49(3):517–535. doi: 10.1085/jgp.49.3.517. [DOI] [PMC free article] [PubMed] [Google Scholar]
- HILL A. V. THE VARIATION OF TOTAL HEAL PRODUCTION IN A TWITCH WITH VELOCITY OF SHORTENING. Proc R Soc Lond B Biol Sci. 1964 Mar 17;159:596–605. doi: 10.1098/rspb.1964.0022. [DOI] [PubMed] [Google Scholar]
- Huxley H. E. The mechanism of muscular contraction. Science. 1969 Jun 20;164(3886):1356–1365. doi: 10.1126/science.164.3886.1356. [DOI] [PubMed] [Google Scholar]
- INFANTE A. A., KLAUPIKS D., DAVIES R. E. ADENOSINE TRIPHOSPHATE: CHANGES IN MUSCLES DOING NEGATIVE WORK. Science. 1964 Jun 26;144(3626):1577–1578. doi: 10.1126/science.144.3626.1577. [DOI] [PubMed] [Google Scholar]
- Jöbsis F. F., O'Connor M. J. Calcium release and reabsorption in the sartorius muscle of the toad. Biochem Biophys Res Commun. 1966 Oct 20;25(2):246–252. doi: 10.1016/0006-291x(66)90588-2. [DOI] [PubMed] [Google Scholar]
- Marler E., Davidson E. A. Structure of a polysaccharide protein complex. Proc Natl Acad Sci U S A. 1965 Aug;54(2):648–656. doi: 10.1073/pnas.54.2.648. [DOI] [PMC free article] [PubMed] [Google Scholar]
- PORTZEHL H., CALDWELL P. C., RUEEGG J. C. THE DEPENDENCE OF CONTRACTION AND RELAXATION OF MUSCLE FIBRES FROM THE CRAB MAIA SQUINADO ON THE INTERNAL CONCENTRATION OF FREE CALCIUM IONS. Biochim Biophys Acta. 1964 May 25;79:581–591. doi: 10.1016/0926-6577(64)90224-4. [DOI] [PubMed] [Google Scholar]
- Poe M. Kinetic studies of temperature changes and oxygen uptake in a differential calorimeter: energy balance during calcium accumulation by mitochondria. Arch Biochem Biophys. 1969 Jul;132(2):377–387. doi: 10.1016/0003-9861(69)90379-8. [DOI] [PubMed] [Google Scholar]
- Sandow A. Excitation-contraction coupling in skeletal muscle. Pharmacol Rev. 1965 Sep;17(3):265–320. [PubMed] [Google Scholar]
- Taylor C. P. Isometric muscle contraction and the active state: an analog computer study. Biophys J. 1969 Jun;9(6):759–780. doi: 10.1016/s0006-3495(69)86416-7. [DOI] [PMC free article] [PubMed] [Google Scholar]
- USSING H. H. The frog skin potential. J Gen Physiol. 1960 May;43:135–147. doi: 10.1085/jgp.43.5.135. [DOI] [PMC free article] [PubMed] [Google Scholar]
- WEBER A., HERZ R., REISS I. On the mechanism of the relaxing effect of fragmented sarcoplasmic reticulum. J Gen Physiol. 1963 Mar;46:679–702. doi: 10.1085/jgp.46.4.679. [DOI] [PMC free article] [PubMed] [Google Scholar]