Abstract
Ca2+ serves a nearly universal intracellular messenger function in cell activation, but excess Ca2+ is also a cellular toxin. The possibility of Ca2+ intoxication is minimized by an elaborate autoregulatory system in which changes in Ca2+ influx rate across the plasma membrane are rapidly compensated for by parallel changes in Ca2+ efflux rate. By this mean, cellular Ca2+ homestasis is maintained so that minimal changes in total cell calcium and cytosolic Ca2+ concentration occur during sustained Ca2(+)-mediated responses. Rather than a sustained increase in cytosolic Ca2+ concentration, it is the localized cycling of Ca2+ across the plasma membrane that is the critically important Ca2+ messenger during the sustained phase of cellular responses mediated via surface receptors linked to the hydrolysis of phosphatidylinositol 4,5-bisphosphate (PIP2). PIP2 hydrolysis gives rise to inositol(1,4,5)trisphosphate (IP3) and diacylglycerol (DAG). The IP3 acts to release Ca2+ from an intracellular pool, thereby causing a transient rise in cytosolic Ca2+ concentration. This transient Ca2+ signal activates calmodulin-dependent protein kinases transiently, and hence, causes the transient phosphorylation of a subset of cellular proteins that mediate the initial phase of the response. The DAG brings about the association of protein kinase C (PKC) with the plasma membrane where a receptor-mediated increase in Ca2+ cycling across the membrane regulates PKC activity. The sustained phosphorylation of a second subset of proteins by PKC mediates the sustained phase of the response. Hence, Ca2+ serves as a messenger during both phases of the cellular response, but its cellular sites of action, its mechanisms of generation, and its molecular targets differ during the initial and sustained phases of the response.(ABSTRACT TRUNCATED AT 250 WORDS)
Full text
PDF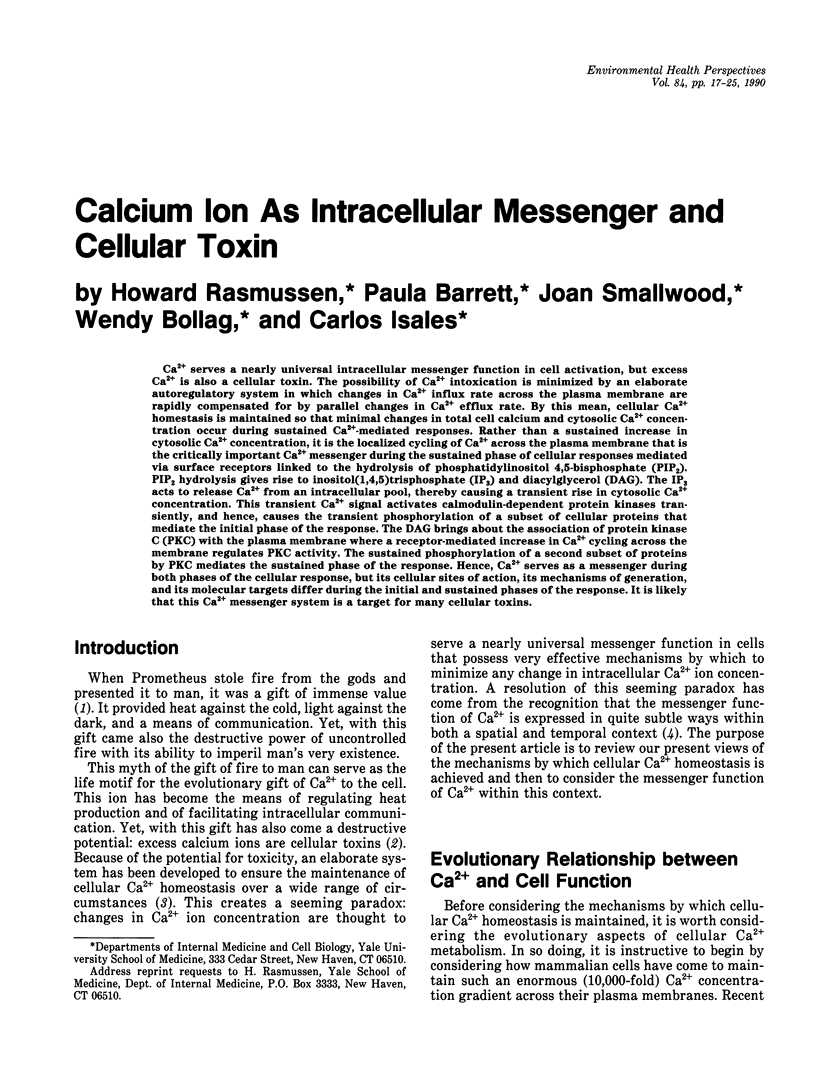
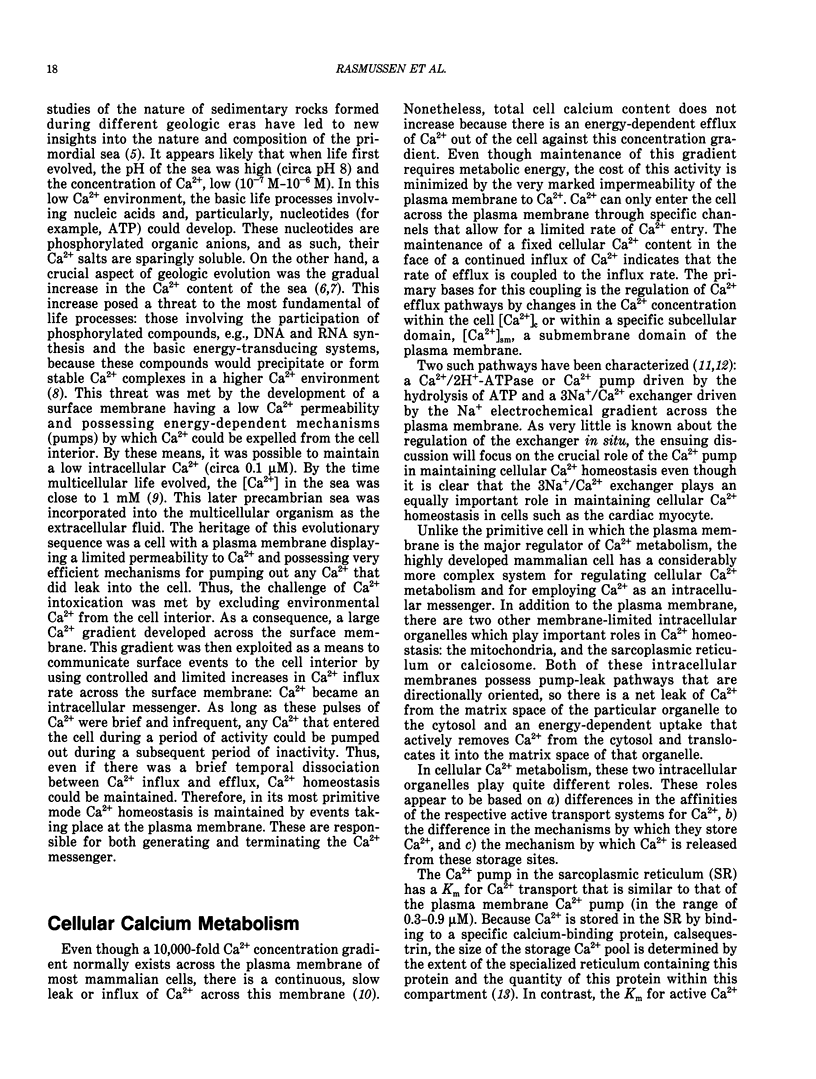
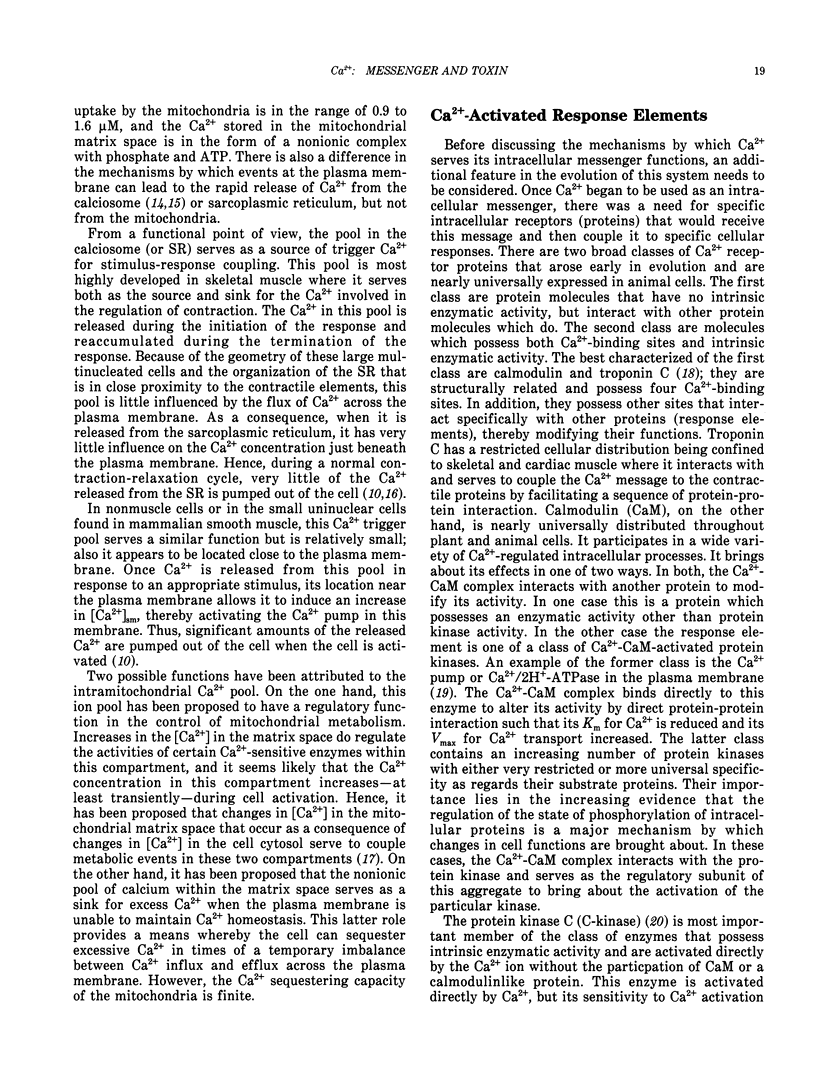
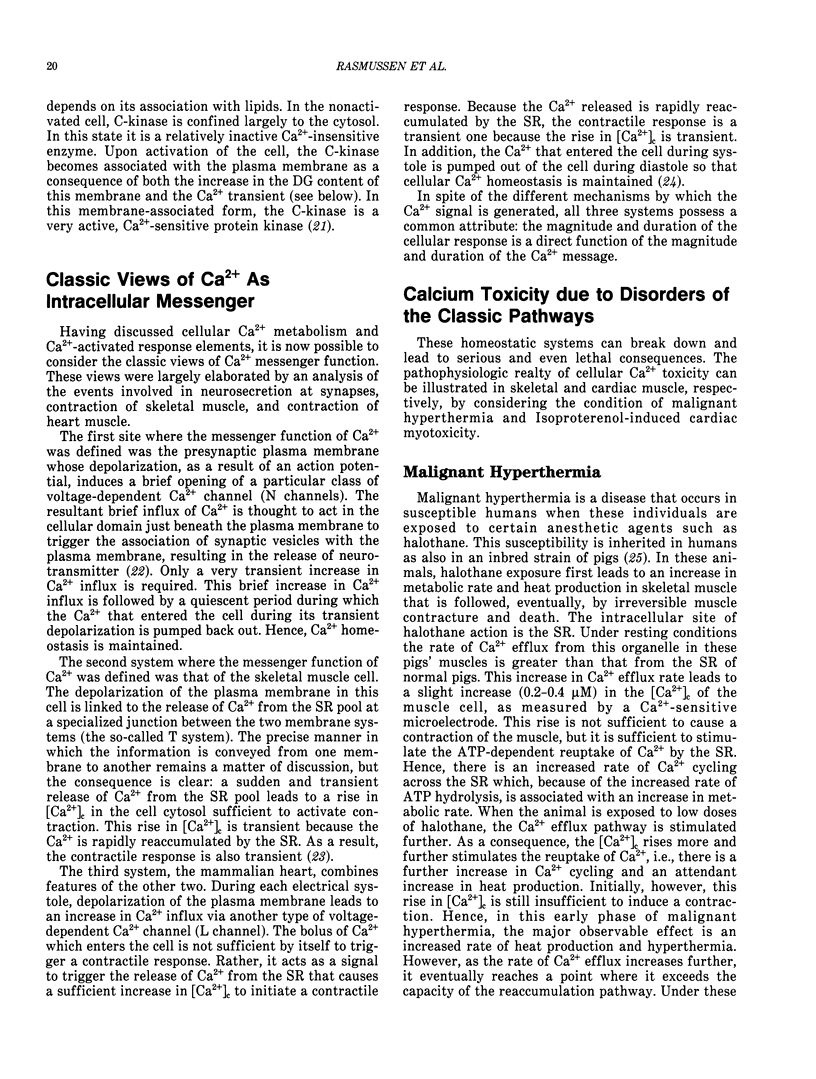
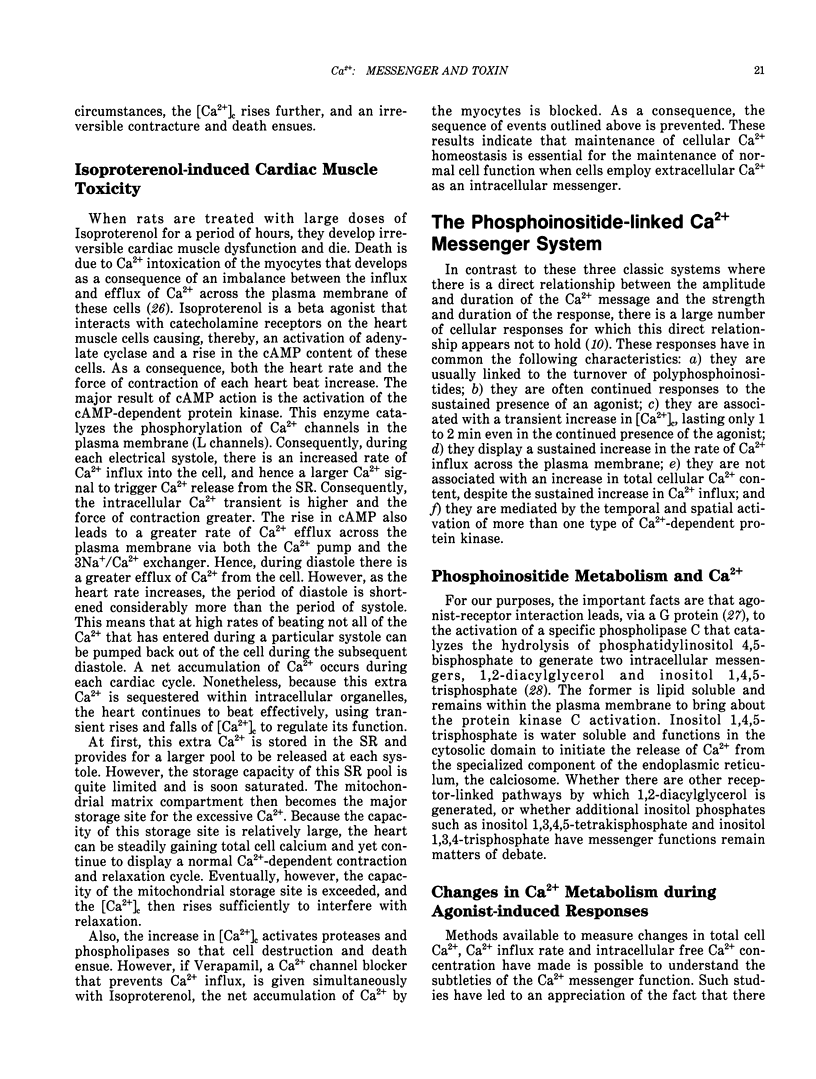
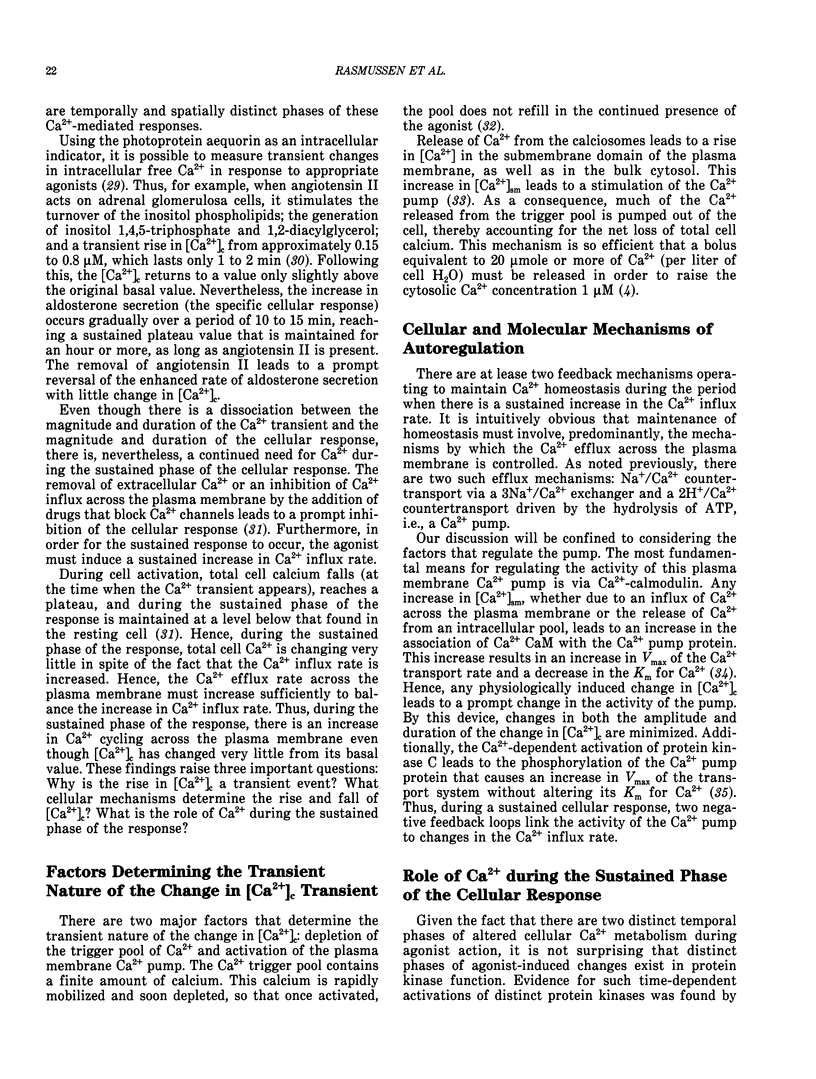
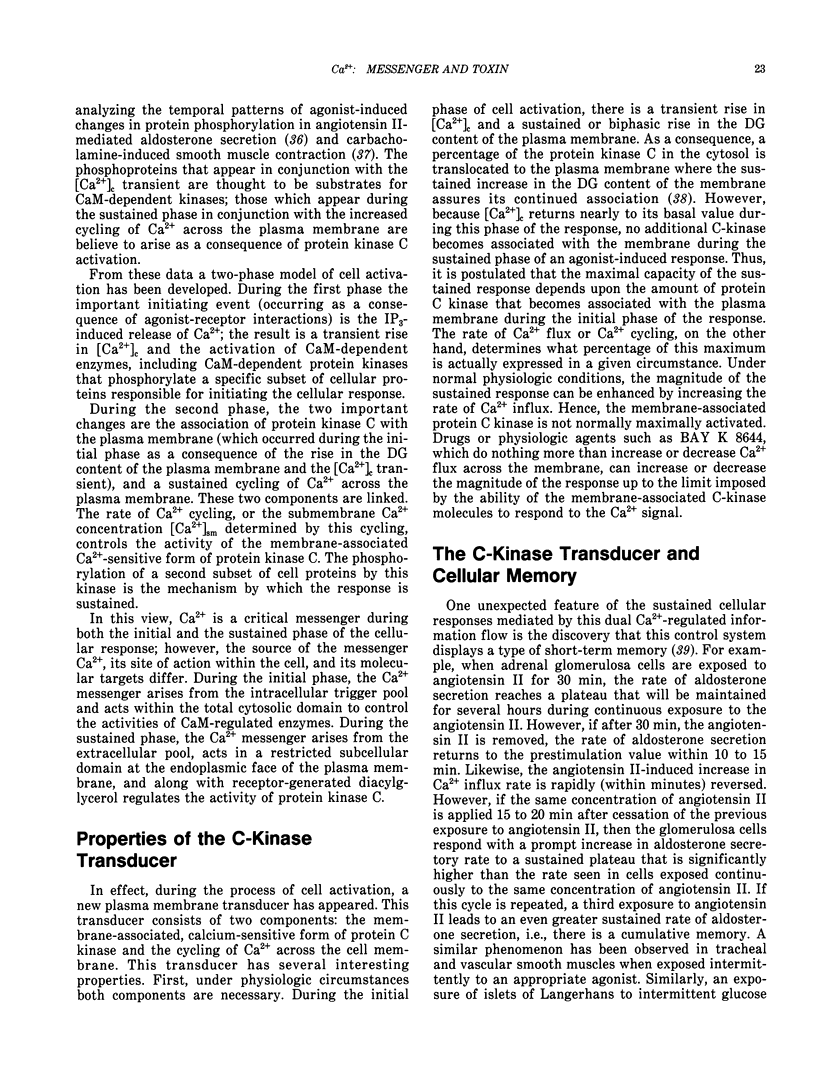
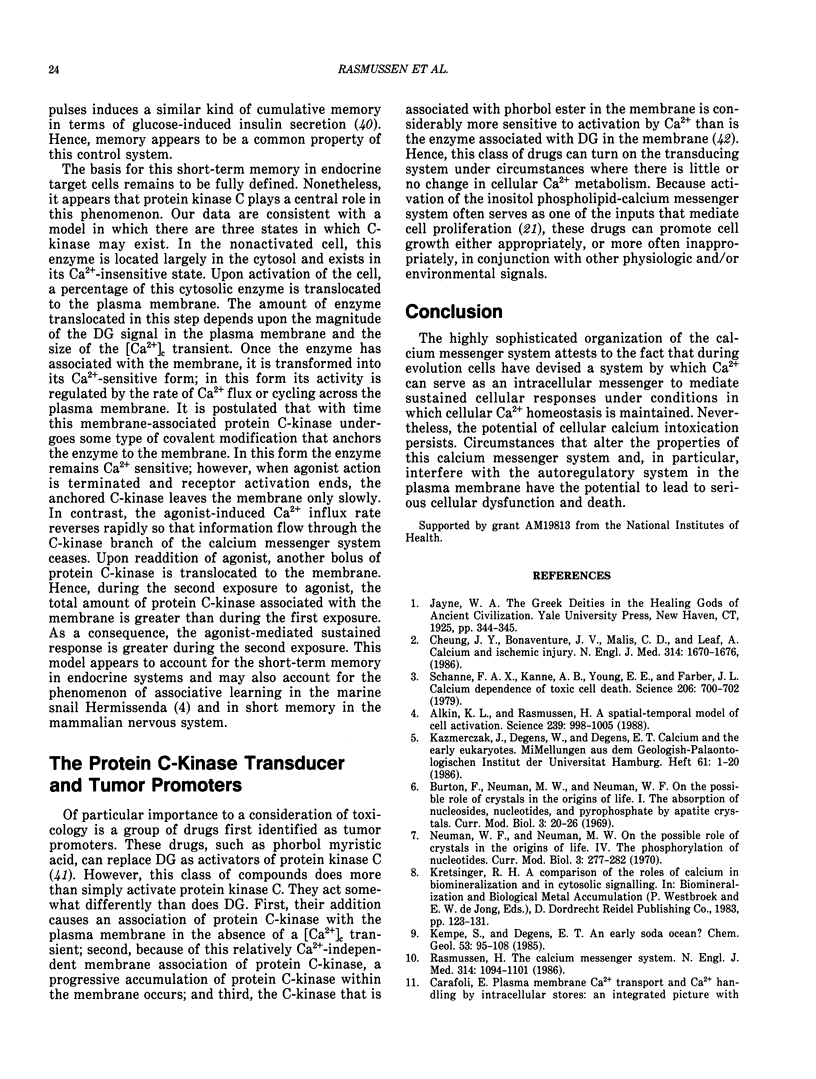
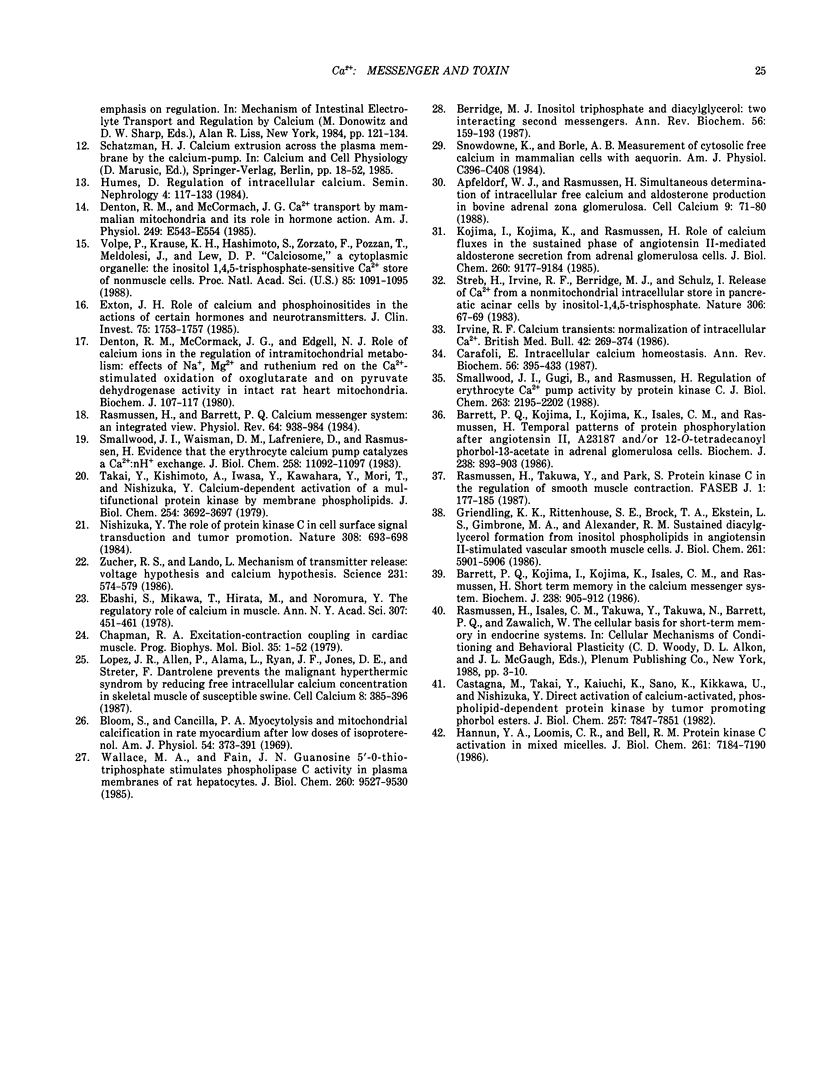
Selected References
These references are in PubMed. This may not be the complete list of references from this article.
- Alkon D. L., Rasmussen H. A spatial-temporal model of cell activation. Science. 1988 Feb 26;239(4843):998–1005. doi: 10.1126/science.2830669. [DOI] [PubMed] [Google Scholar]
- Apfeldorf W. J., Rasmussen H. Simultaneous determination of intracellular free calcium and aldosterone production in bovine adrenal zona glomerulosa. Cell Calcium. 1988 Apr;9(2):71–80. doi: 10.1016/0143-4160(88)90026-7. [DOI] [PubMed] [Google Scholar]
- Barrett P. Q., Kojima I., Kojima K., Zawalich K., Isales C. M., Rasmussen H. Short term memory in the calcium messenger system. Evidence for a sustained activation of protein kinase C in adrenal glomerulosa cells. Biochem J. 1986 Sep 15;238(3):905–912. doi: 10.1042/bj2380905. [DOI] [PMC free article] [PubMed] [Google Scholar]
- Barrett P. Q., Kojima I., Kojima K., Zawalich K., Isales C. M., Rasmussen H. Temporal patterns of protein phosphorylation after angiotensin II, A23187 and/or 12-O-tetradecanoylphorbol 13-acetate in adrenal glomerulosa cells. Biochem J. 1986 Sep 15;238(3):893–903. doi: 10.1042/bj2380893. [DOI] [PMC free article] [PubMed] [Google Scholar]
- Berridge M. J. Inositol trisphosphate and diacylglycerol: two interacting second messengers. Annu Rev Biochem. 1987;56:159–193. doi: 10.1146/annurev.bi.56.070187.001111. [DOI] [PubMed] [Google Scholar]
- Bloom S., Cancilla P. A. Myocytolysis and mitochondrial calcification in rat myocardium after low doses of isoproterenol. Am J Pathol. 1969 Mar;54(3):373–391. [PMC free article] [PubMed] [Google Scholar]
- Burton F. G., Neuman M. W., Neuman W. F. On the possible role of crystals in the origins of life. I. The adsorption of nucleosides, nucleotides and pyrophosphate by apatite crystals. Curr Mod Biol. 1969 Oct;3(1):20–26. doi: 10.1016/0303-2647(69)90005-7. [DOI] [PubMed] [Google Scholar]
- Carafoli E. Intracellular calcium homeostasis. Annu Rev Biochem. 1987;56:395–433. doi: 10.1146/annurev.bi.56.070187.002143. [DOI] [PubMed] [Google Scholar]
- Castagna M., Takai Y., Kaibuchi K., Sano K., Kikkawa U., Nishizuka Y. Direct activation of calcium-activated, phospholipid-dependent protein kinase by tumor-promoting phorbol esters. J Biol Chem. 1982 Jul 10;257(13):7847–7851. [PubMed] [Google Scholar]
- Chapman R. A. Excitation-contraction coupling in cardiac muscle. Prog Biophys Mol Biol. 1979;35(1):1–52. doi: 10.1016/0079-6107(80)90002-4. [DOI] [PubMed] [Google Scholar]
- Cheung J. Y., Bonventre J. V., Malis C. D., Leaf A. Calcium and ischemic injury. N Engl J Med. 1986 Jun 26;314(26):1670–1676. doi: 10.1056/NEJM198606263142604. [DOI] [PubMed] [Google Scholar]
- Denton R. M., McCormack J. G. Ca2+ transport by mammalian mitochondria and its role in hormone action. Am J Physiol. 1985 Dec;249(6 Pt 1):E543–E554. doi: 10.1152/ajpendo.1985.249.6.E543. [DOI] [PubMed] [Google Scholar]
- Ebashi S., Mikawa T., Hirata M., Nonomura Y. The regulatory role of calcium in muscle. Ann N Y Acad Sci. 1978 Apr 28;307:451–461. doi: 10.1111/j.1749-6632.1978.tb41975.x. [DOI] [PubMed] [Google Scholar]
- Exton J. H. Role of calcium and phosphoinositides in the actions of certain hormones and neurotransmitters. J Clin Invest. 1985 Jun;75(6):1753–1757. doi: 10.1172/JCI111886. [DOI] [PMC free article] [PubMed] [Google Scholar]
- Griendling K. K., Rittenhouse S. E., Brock T. A., Ekstein L. S., Gimbrone M. A., Jr, Alexander R. W. Sustained diacylglycerol formation from inositol phospholipids in angiotensin II-stimulated vascular smooth muscle cells. J Biol Chem. 1986 May 5;261(13):5901–5906. [PubMed] [Google Scholar]
- Hannun Y. A., Loomis C. R., Bell R. M. Protein kinase C activation in mixed micelles. Mechanistic implications of phospholipid, diacylglycerol, and calcium interdependencies. J Biol Chem. 1986 Jun 5;261(16):7184–7190. [PubMed] [Google Scholar]
- Irvine R. F. Calcium transients: mobilization of intracellular Ca2+. Br Med Bull. 1986 Oct;42(4):369–374. doi: 10.1093/oxfordjournals.bmb.a072154. [DOI] [PubMed] [Google Scholar]
- Kojima I., Kojima K., Rasmussen H. Role of calcium fluxes in the sustained phase of angiotensin II-mediated aldosterone secretion from adrenal glomerulosa cells. J Biol Chem. 1985 Aug 5;260(16):9177–9184. [PubMed] [Google Scholar]
- Lopez J. R., Allen P., Alamo L., Ryan J. F., Jones D. E., Sreter F. Dantrolene prevents the malignant hyperthermic syndrome by reducing free intracellular calcium concentration in skeletal muscle of susceptible swine. Cell Calcium. 1987 Oct;8(5):385–396. doi: 10.1016/0143-4160(87)90013-3. [DOI] [PubMed] [Google Scholar]
- Neuman M. W., Neuman W. F., Lane K. On the possible role of crystals in the origins of life. IV. The phosphorylation of nucleotides. Curr Mod Biol. 1970 Jul;3(3):277–283. doi: 10.1016/0303-2647(70)90010-9. [DOI] [PubMed] [Google Scholar]
- Nishizuka Y. The role of protein kinase C in cell surface signal transduction and tumour promotion. Nature. 1984 Apr 19;308(5961):693–698. doi: 10.1038/308693a0. [DOI] [PubMed] [Google Scholar]
- Rasmussen H., Barrett P. Q. Calcium messenger system: an integrated view. Physiol Rev. 1984 Jul;64(3):938–984. doi: 10.1152/physrev.1984.64.3.938. [DOI] [PubMed] [Google Scholar]
- Rasmussen H., Takuwa Y., Park S. Protein kinase C in the regulation of smooth muscle contraction. FASEB J. 1987 Sep;1(3):177–185. [PubMed] [Google Scholar]
- Rasmussen H. The calcium messenger system (1). N Engl J Med. 1986 Apr 24;314(17):1094–1101. doi: 10.1056/NEJM198604243141707. [DOI] [PubMed] [Google Scholar]
- Schanne F. A., Kane A. B., Young E. E., Farber J. L. Calcium dependence of toxic cell death: a final common pathway. Science. 1979 Nov 9;206(4419):700–702. doi: 10.1126/science.386513. [DOI] [PubMed] [Google Scholar]
- Smallwood J. I., Gügi B., Rasmussen H. Regulation of erythrocyte Ca2+ pump activity by protein kinase C. J Biol Chem. 1988 Feb 15;263(5):2195–2202. [PubMed] [Google Scholar]
- Smallwood J. I., Waisman D. M., Lafreniere D., Rasmussen H. Evidence that the erythrocyte calcium pump catalyzes a Ca2+:nH+ exchange. J Biol Chem. 1983 Sep 25;258(18):11092–11097. [PubMed] [Google Scholar]
- Streb H., Irvine R. F., Berridge M. J., Schulz I. Release of Ca2+ from a nonmitochondrial intracellular store in pancreatic acinar cells by inositol-1,4,5-trisphosphate. Nature. 1983 Nov 3;306(5938):67–69. doi: 10.1038/306067a0. [DOI] [PubMed] [Google Scholar]
- Takai Y., Kishimoto A., Iwasa Y., Kawahara Y., Mori T., Nishizuka Y. Calcium-dependent activation of a multifunctional protein kinase by membrane phospholipids. J Biol Chem. 1979 May 25;254(10):3692–3695. [PubMed] [Google Scholar]
- Volpe P., Krause K. H., Hashimoto S., Zorzato F., Pozzan T., Meldolesi J., Lew D. P. "Calciosome," a cytoplasmic organelle: the inositol 1,4,5-trisphosphate-sensitive Ca2+ store of nonmuscle cells? Proc Natl Acad Sci U S A. 1988 Feb;85(4):1091–1095. doi: 10.1073/pnas.85.4.1091. [DOI] [PMC free article] [PubMed] [Google Scholar]
- Wallace M. A., Fain J. N. Guanosine 5'-O-thiotriphosphate stimulates phospholipase C activity in plasma membranes of rat hepatocytes. J Biol Chem. 1985 Aug 15;260(17):9527–9530. [PubMed] [Google Scholar]
- Zucker R. S., Landò L. Mechanism of transmitter release: voltage hypothesis and calcium hypothesis. Science. 1986 Feb 7;231(4738):574–579. doi: 10.1126/science.2868525. [DOI] [PubMed] [Google Scholar]