Abstract
1. Potential changes in isolated rat superior cervical ganglia following addition and removal of depolarizing agents were recorded using a moving-fluid extracellular electrode system.
2. Ganglionic negativity produced by carbachol was followed by a pronounced ganglionic positivity on washing. This after-positivity was attributed to hyperpolarization of the ganglion cells since it was unaffected by crushing the postganglionic trunk.
3. The after-hyperpolarization was selectively depressed by (a) cooling (Q10 2·3), (b) metabolic inhibitors (cyanide, azide, 2,4-dinitrophenol), (c) reducing [K+]o or substituting Cs+ for K+, (d) ouabain, and (e) substituting Li+ for Na+. This suggested a close dependence on active Na+ transport.
4. When K+ was restored to K+-free solution, or the preparation was warmed rapidly, or when metabolic inhibitors were washed away, the hyperpolarization was rapidly regenerated. The effect of restoring K+ indicated that the hyperpolarization was generated directly by the Na+ pump.
5. The hyperpolarization was not altered by replacing Cl- with isethionate, indicating that the voltage change produced by the Na+ current was not modified by passive Cl- movements.
6. Hexamethonium added to the washout fluid augmented the after-hyperpolarization, suggesting that there was a high (cationic) leak current due to continued receptor-activation on washing with normal Krebs solution.
7. The hyperpolarization was reduced by omission of Ca2+ and restored by addition of Mg2+. This was considered to result from changes in passive membrane permeability.
8. The time-course of post-carbachol hyperpolarization accorded with a Na+ extrusion process whose rate was directly proportional to [Na+]i with a rate constant of 0·38±0·02 min-1 at 23-27° C.
9. With increasing concentrations of carbachol, the amplitude of the hyperpolarization increased in proportion to the preceding depolarization, but the rate constant of the hyperpolarization was unchanged.
Full text
PDF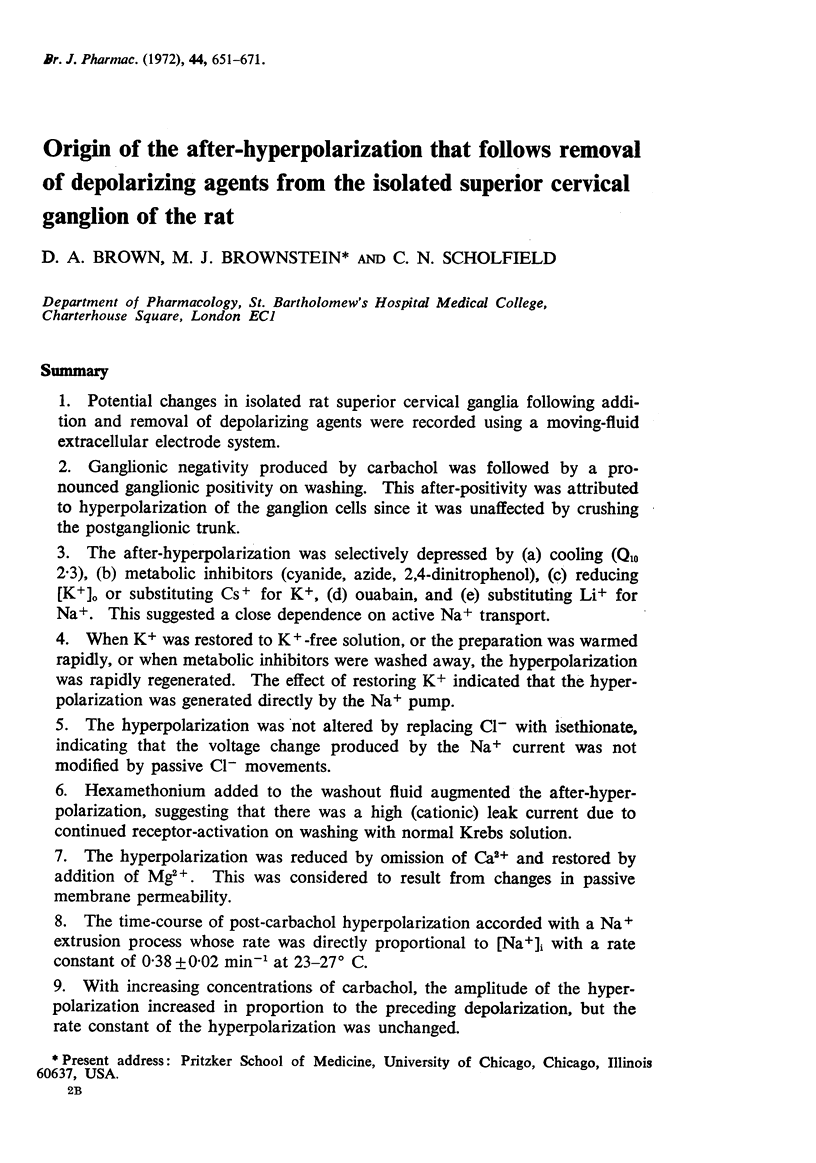
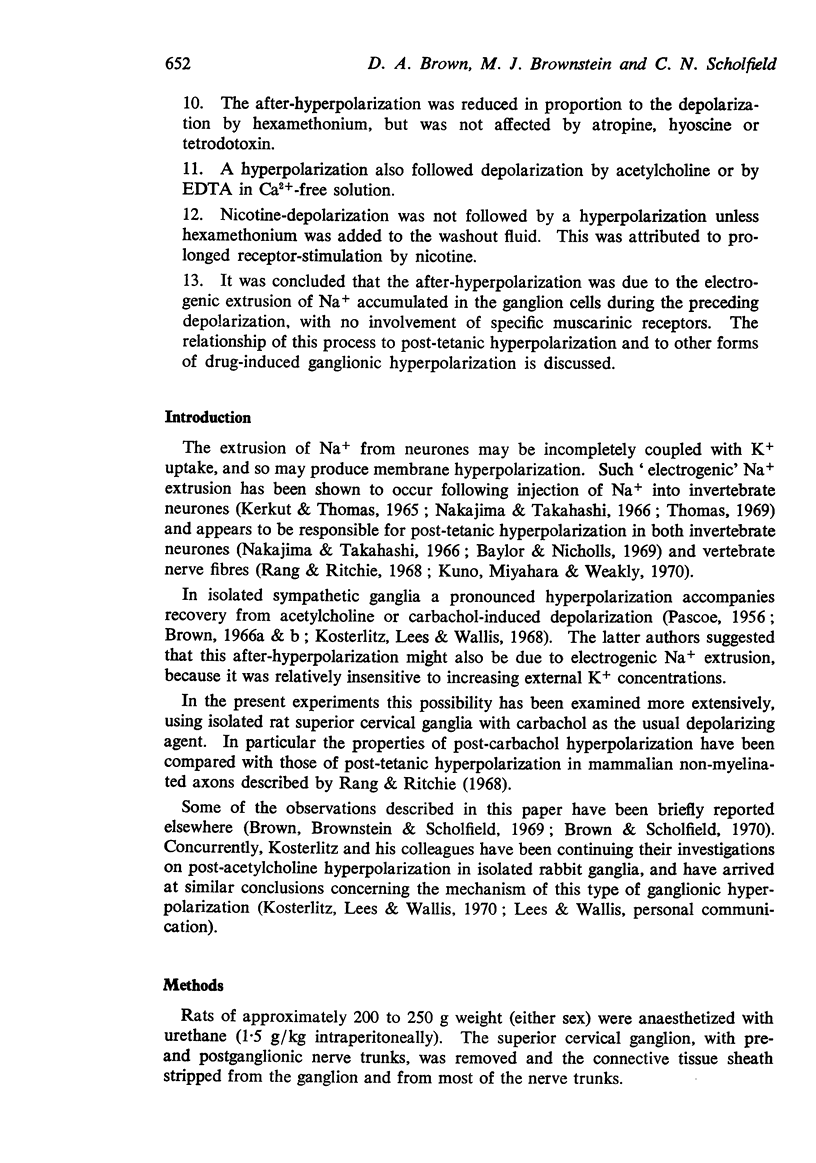
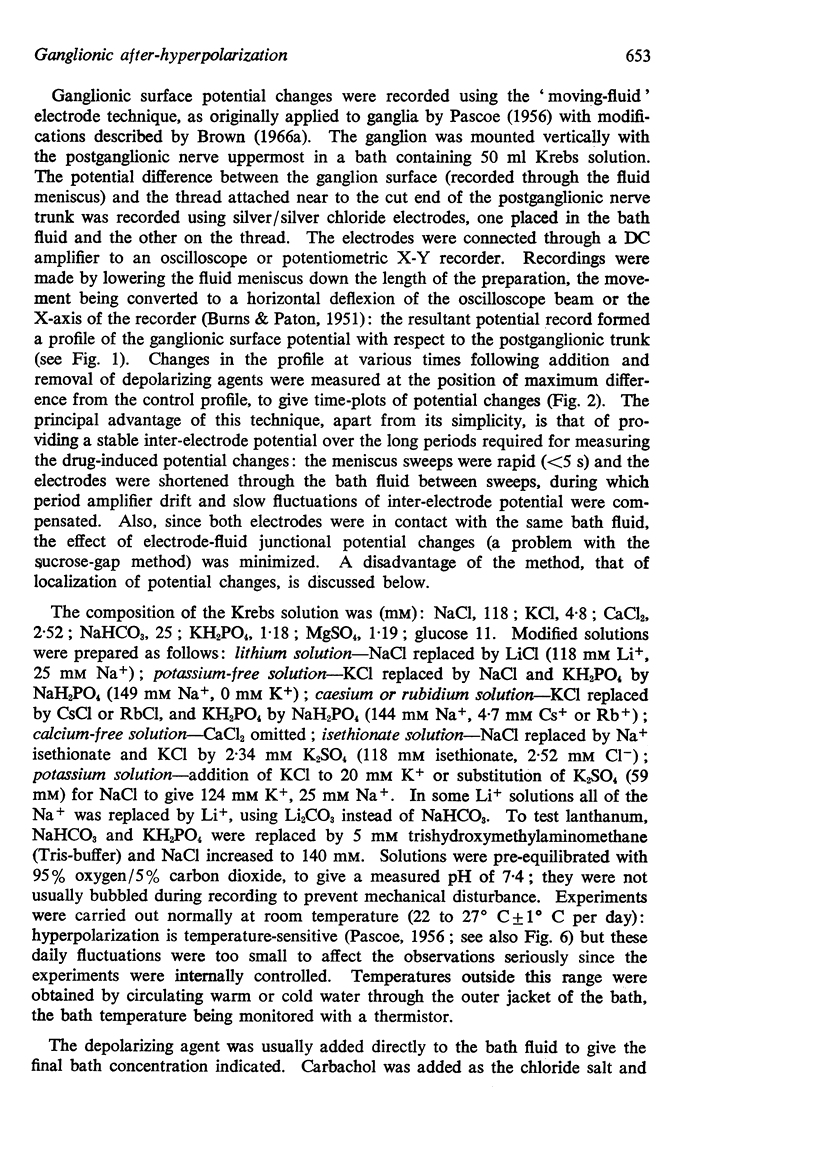
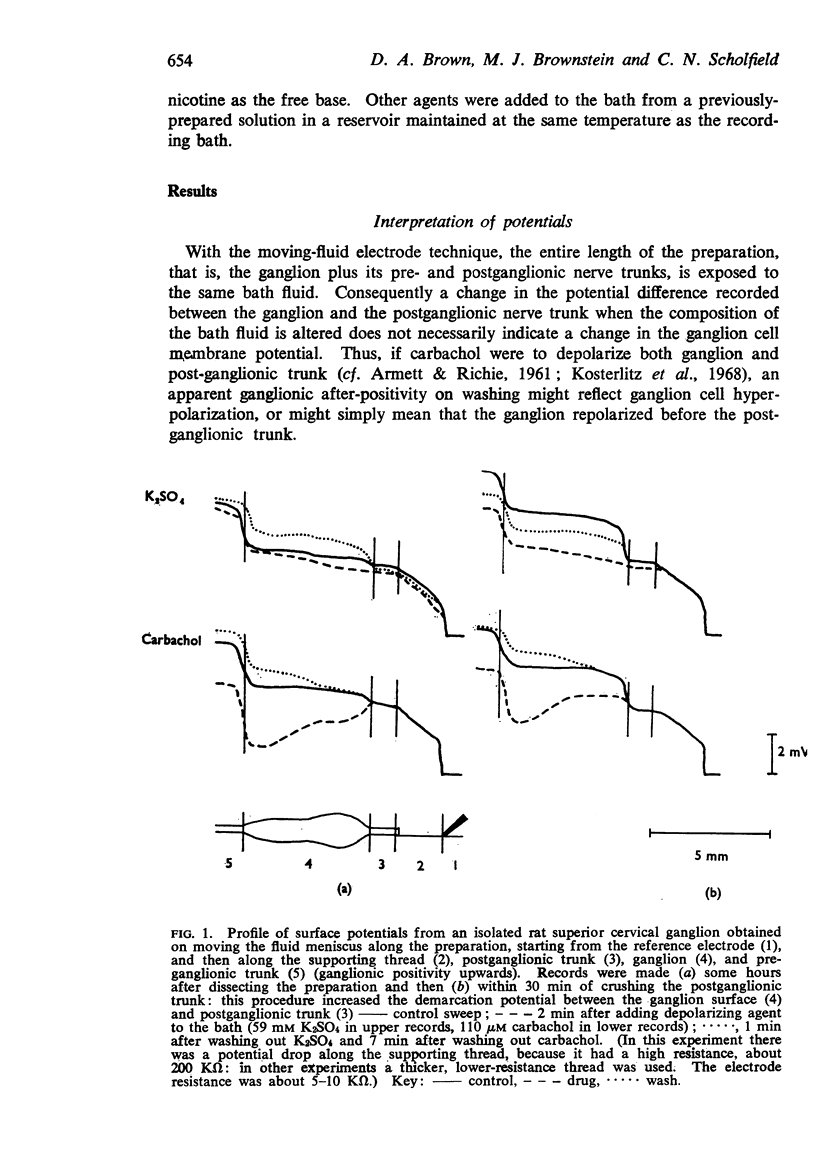
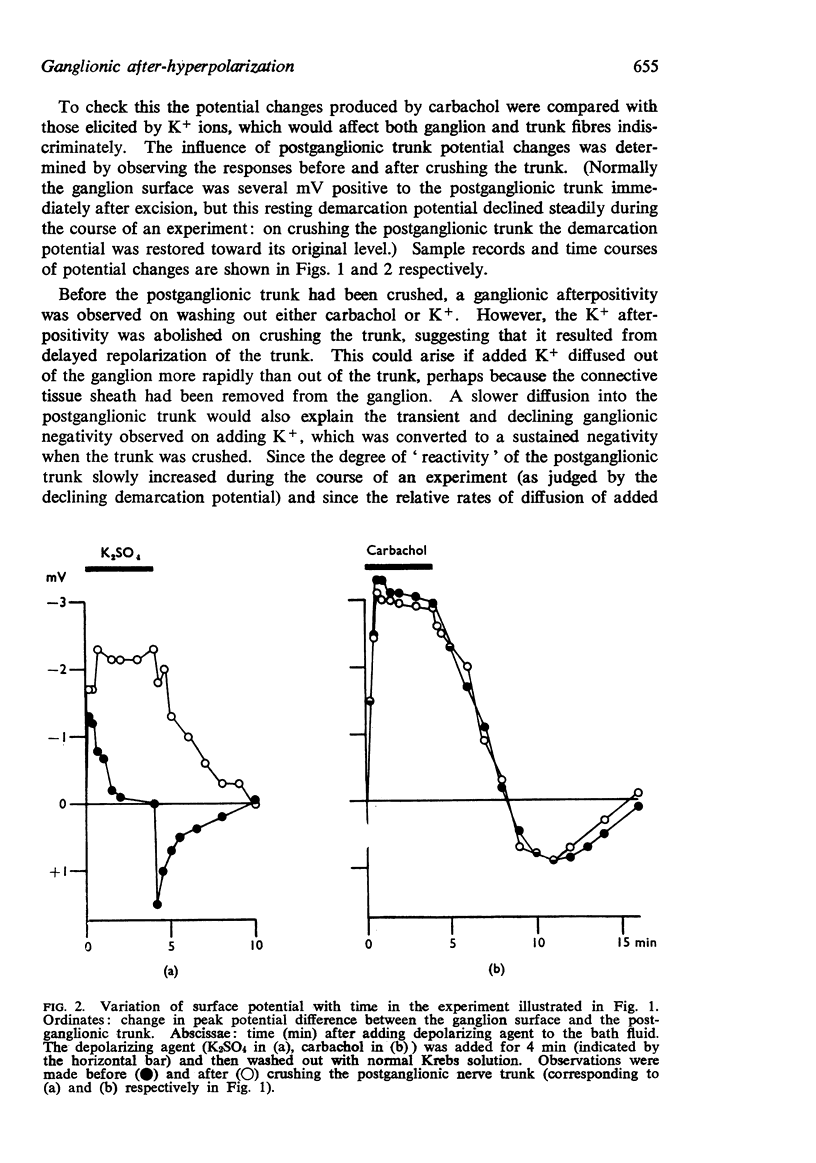
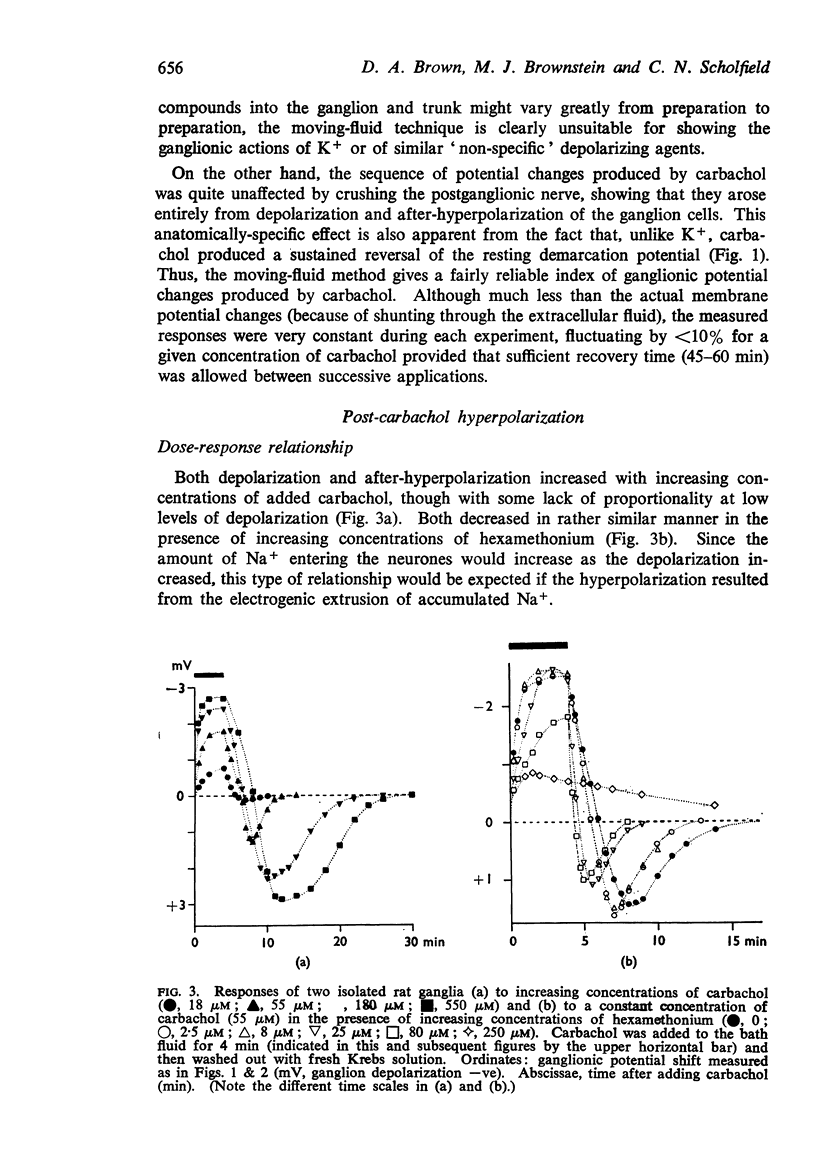
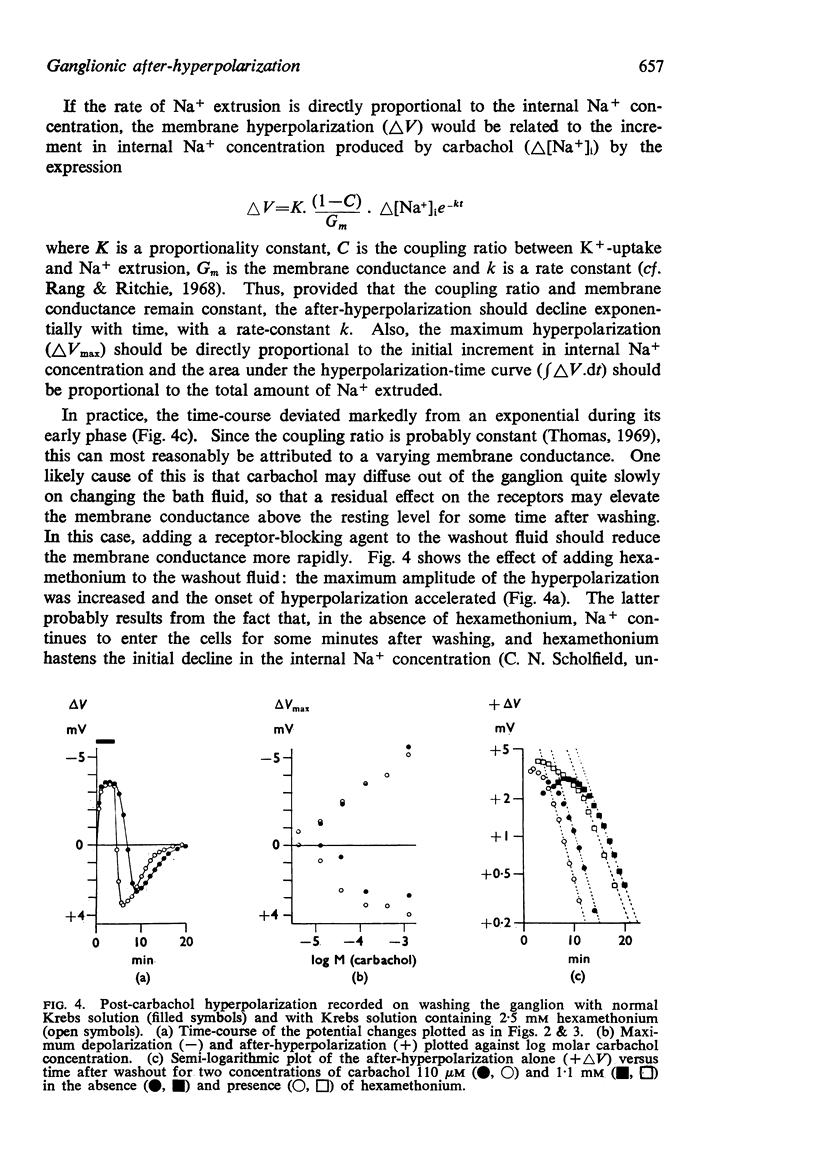
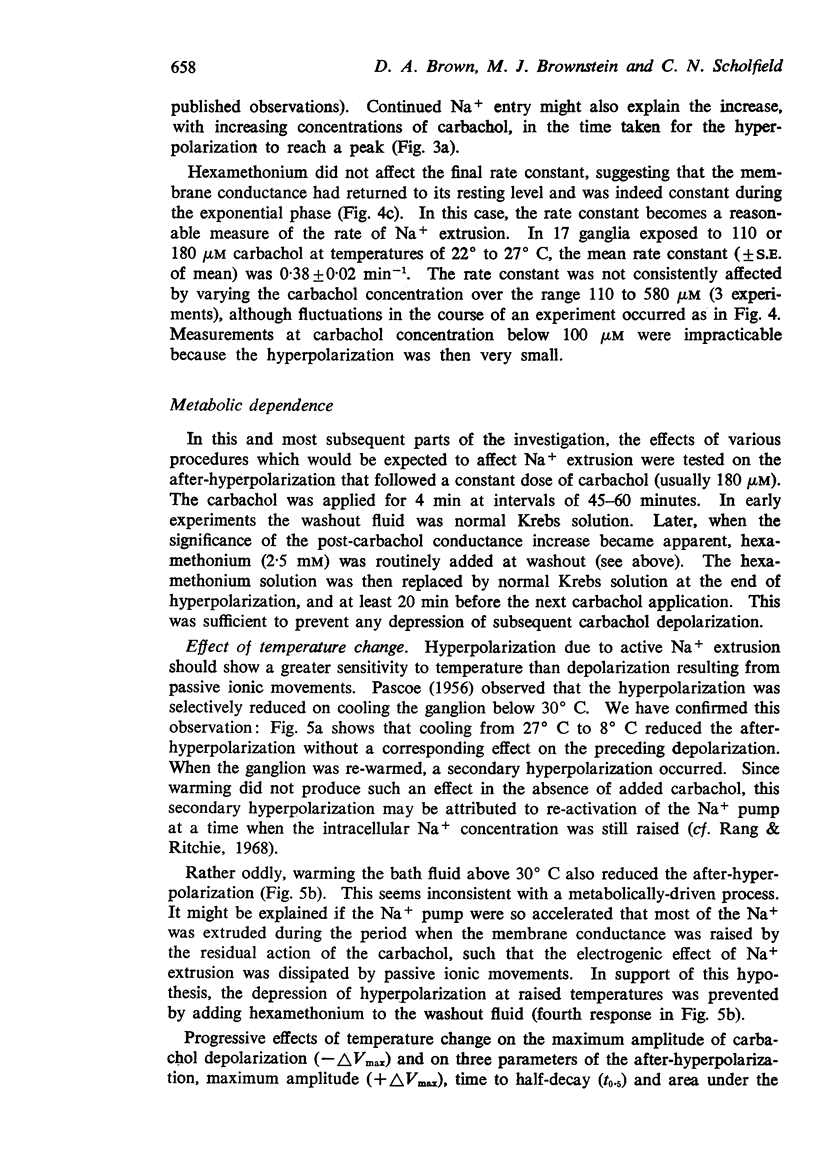
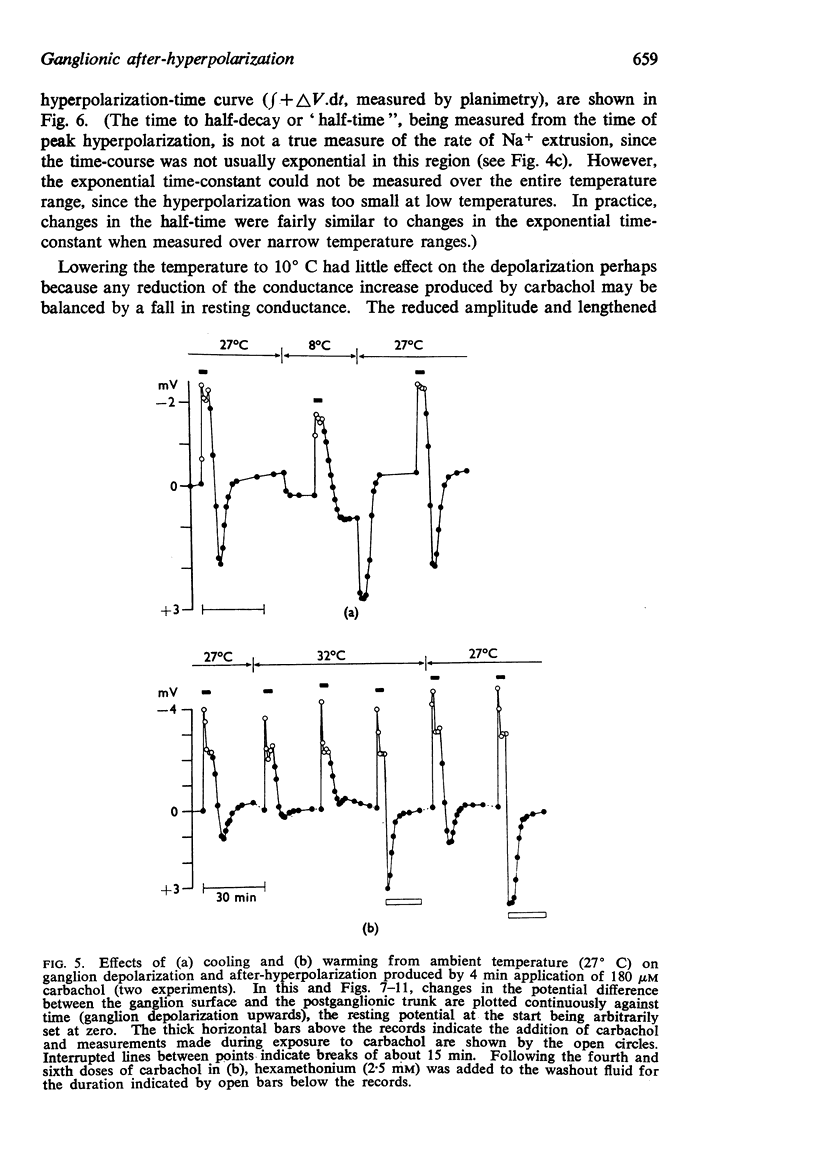
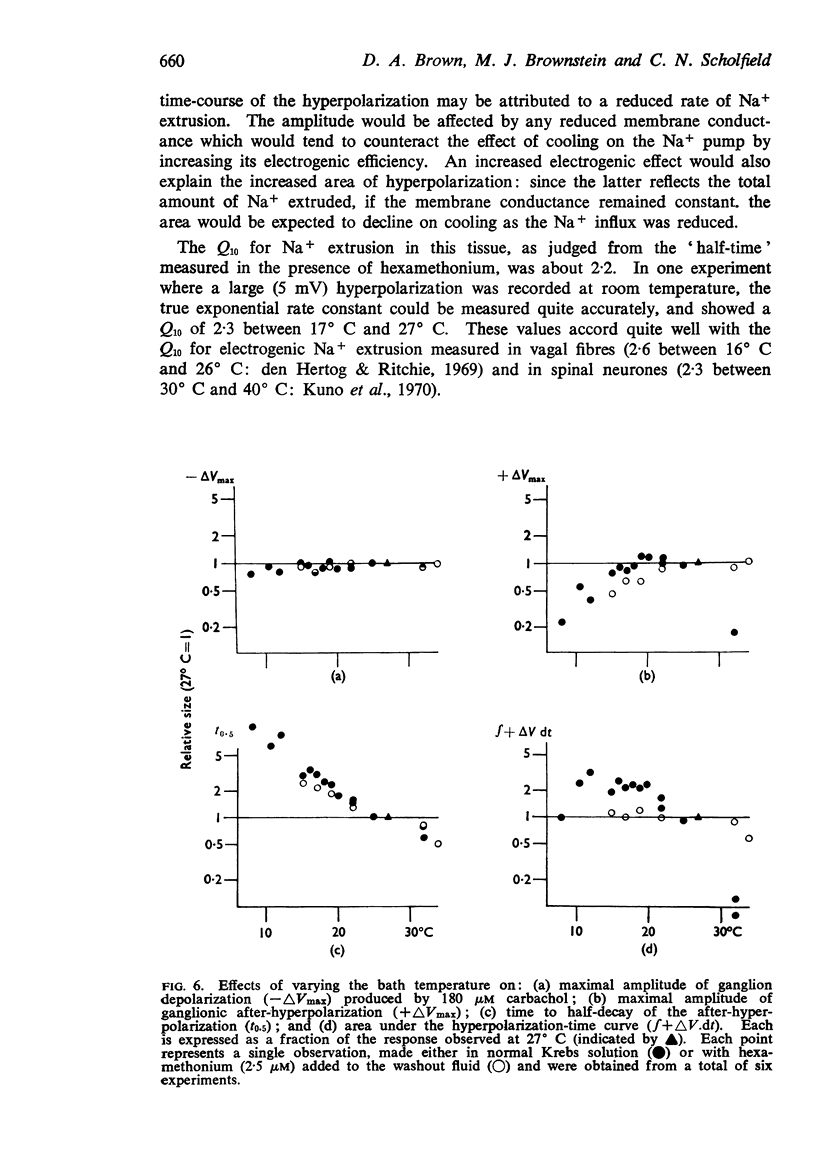
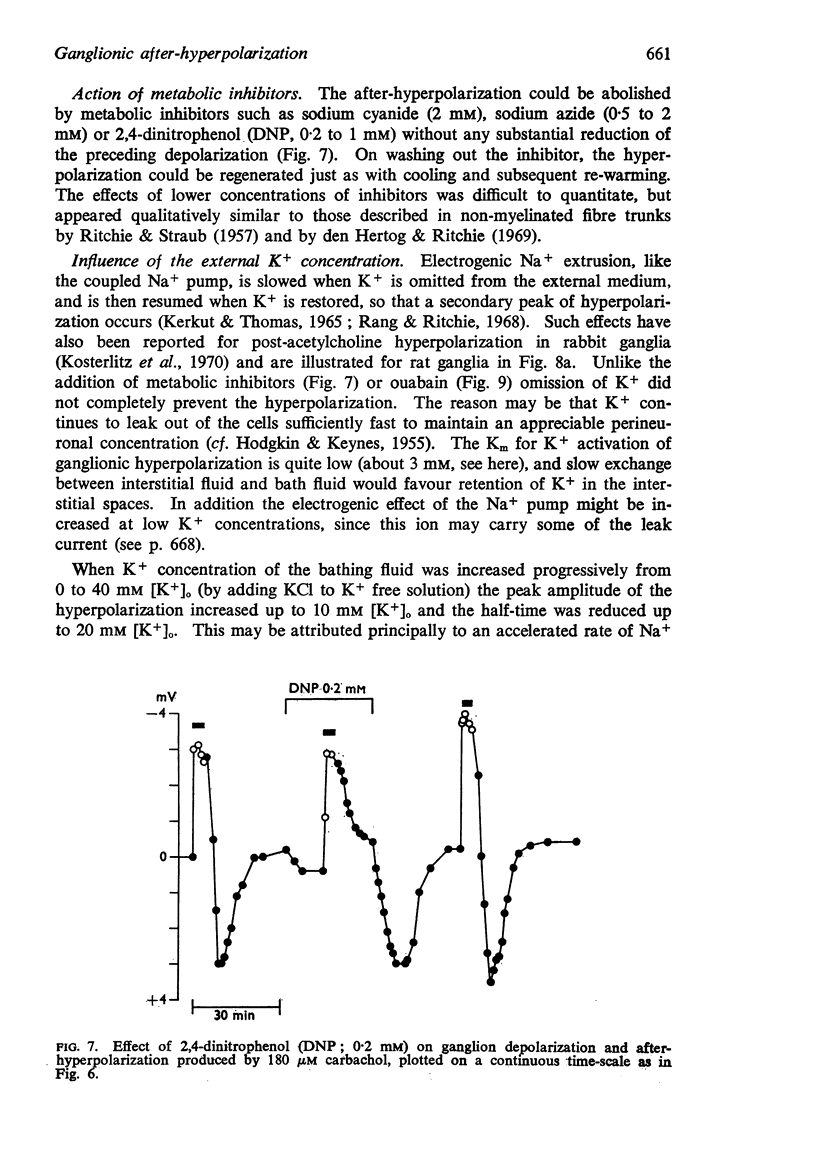
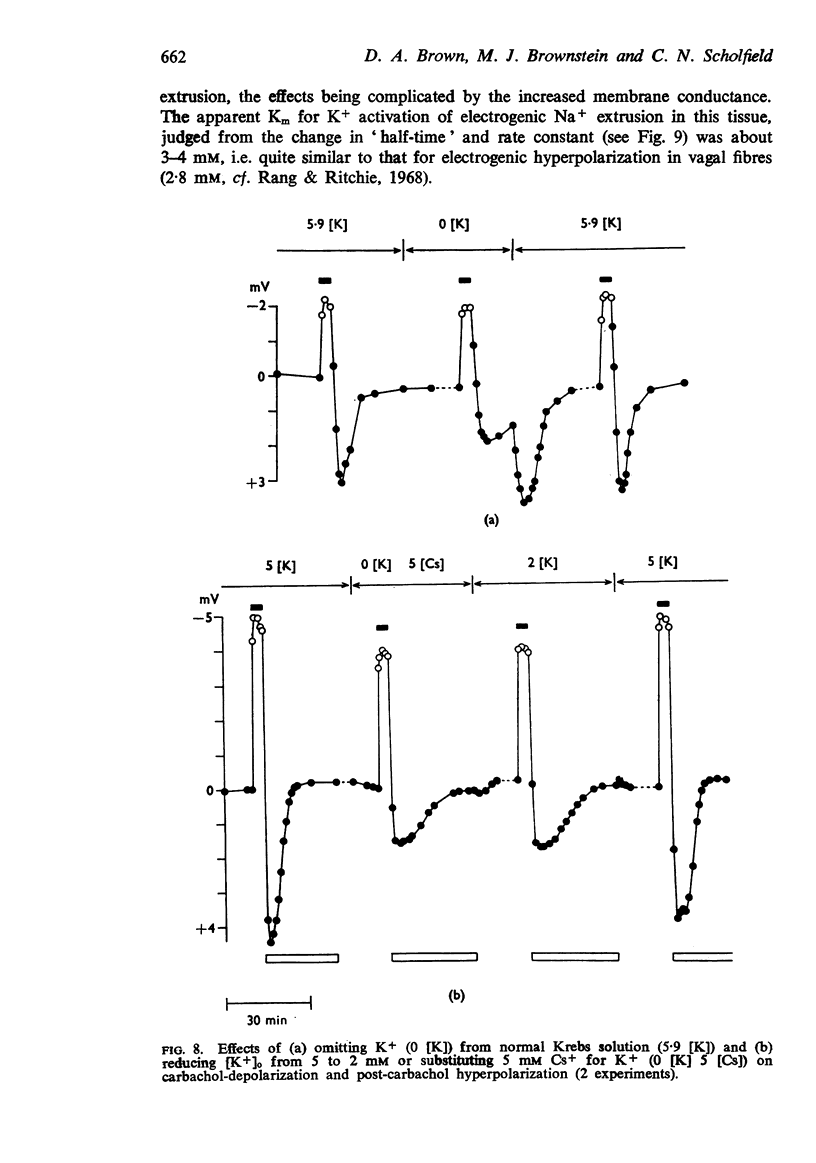
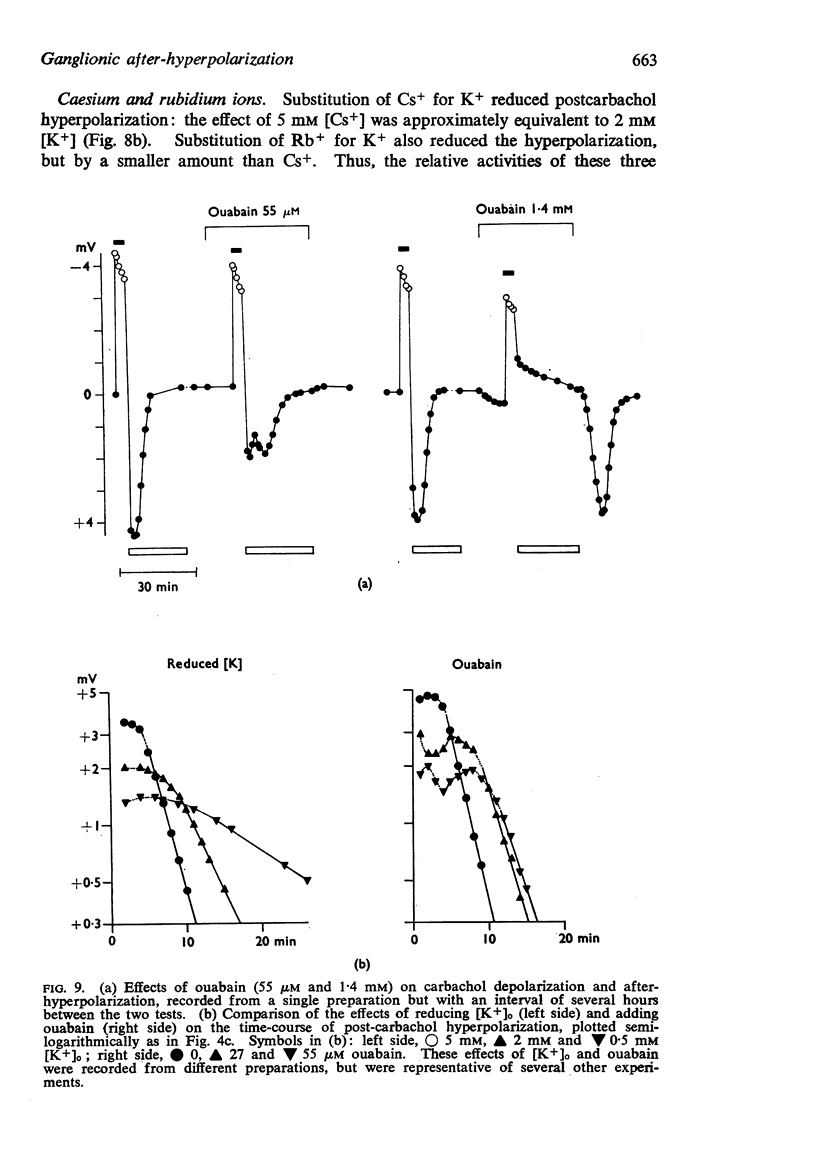
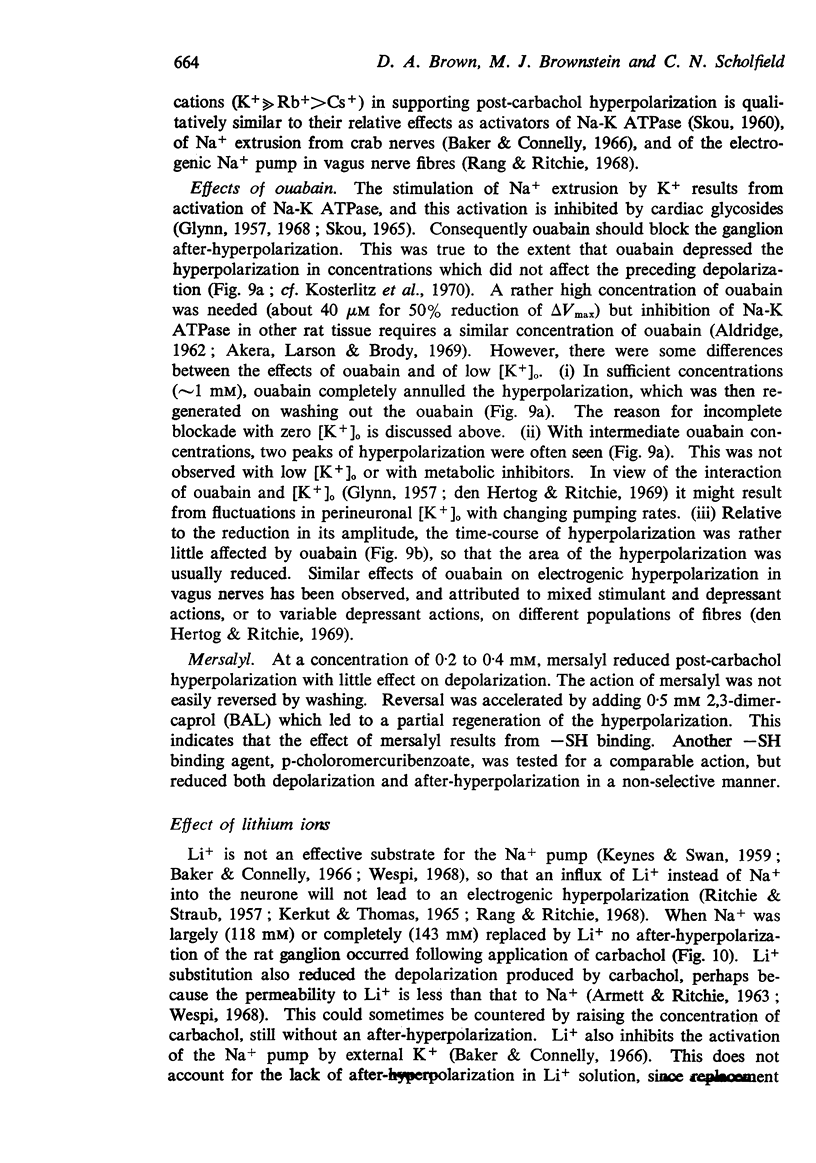
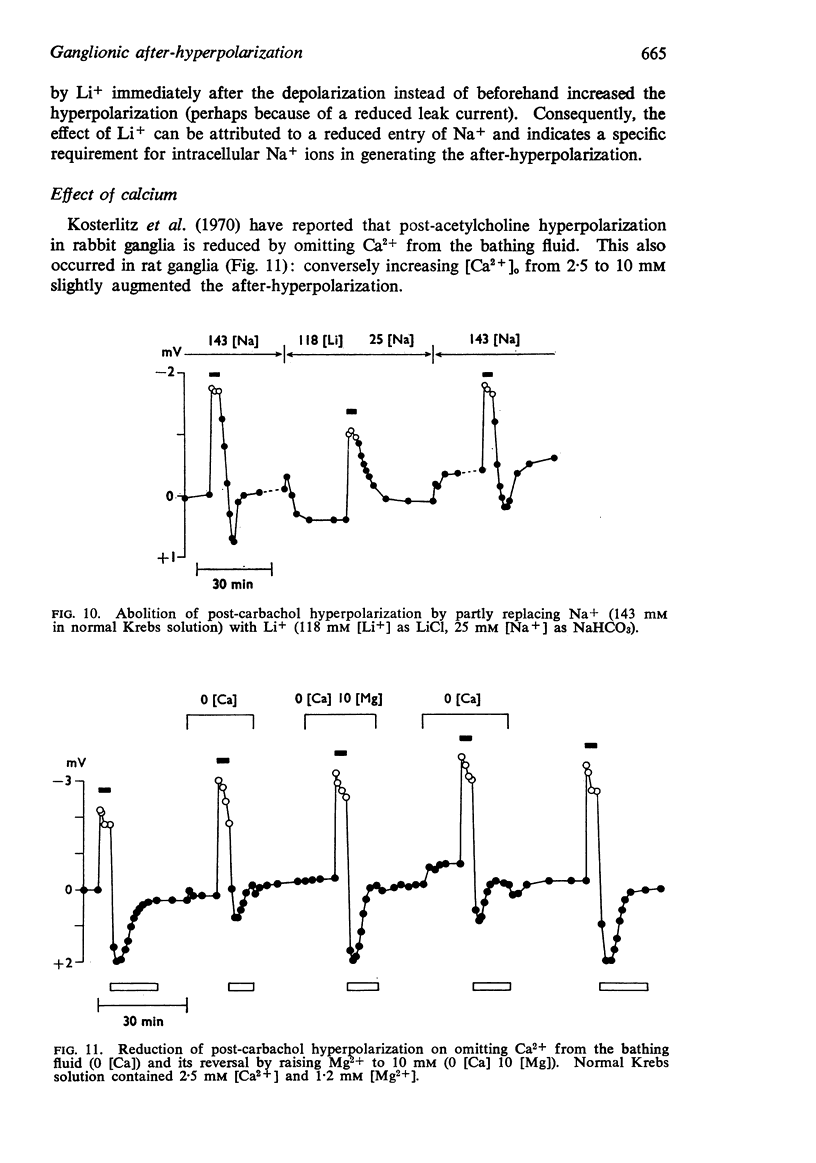
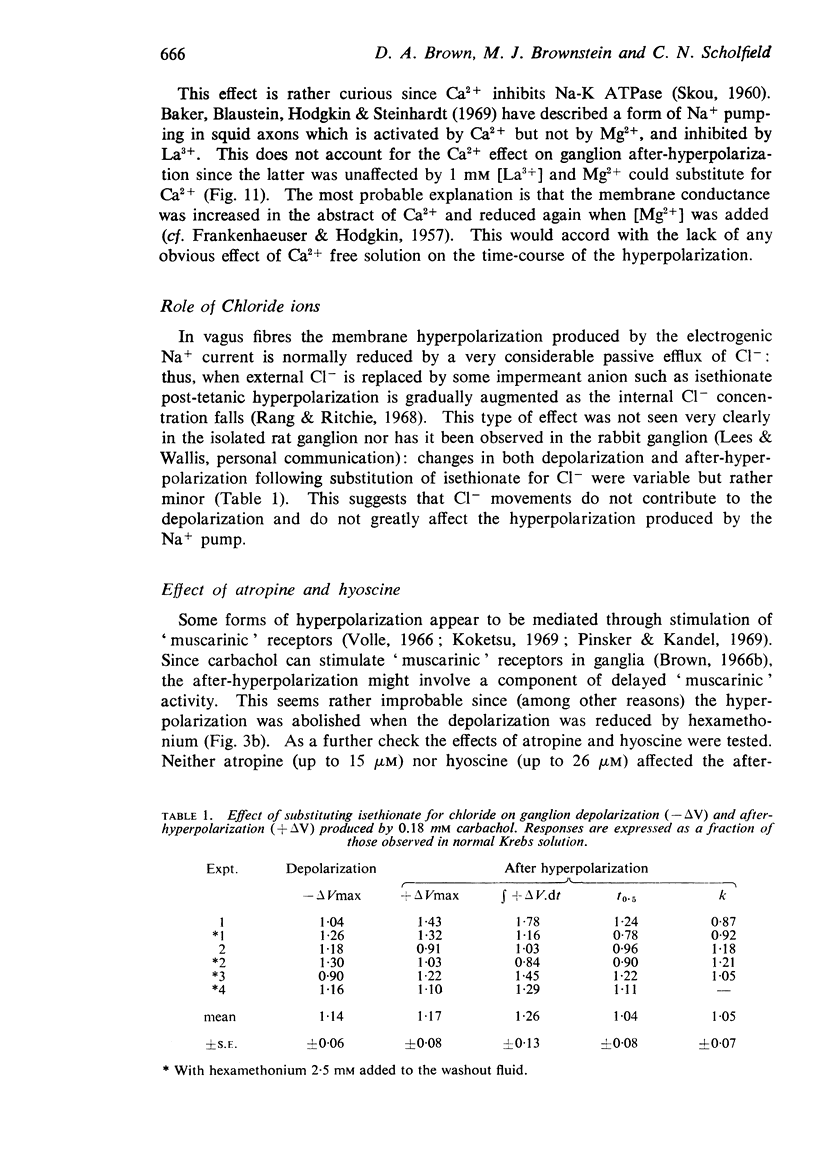
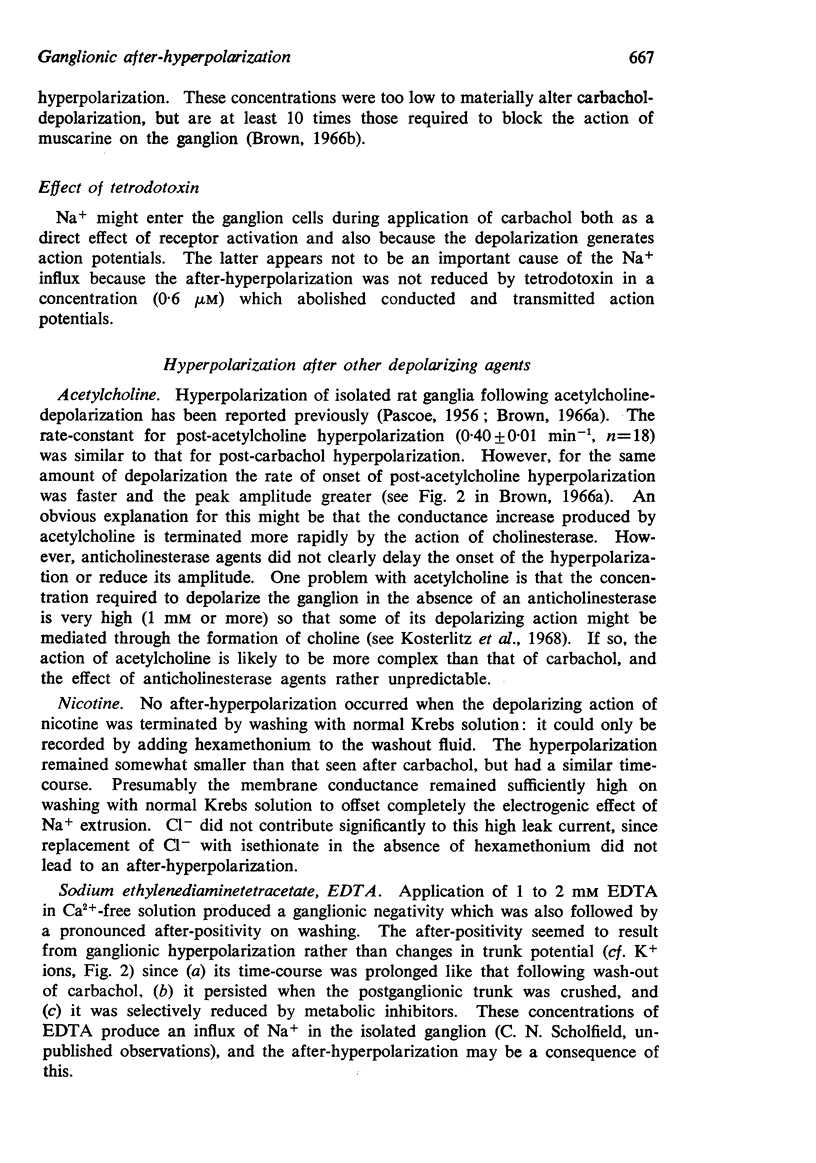
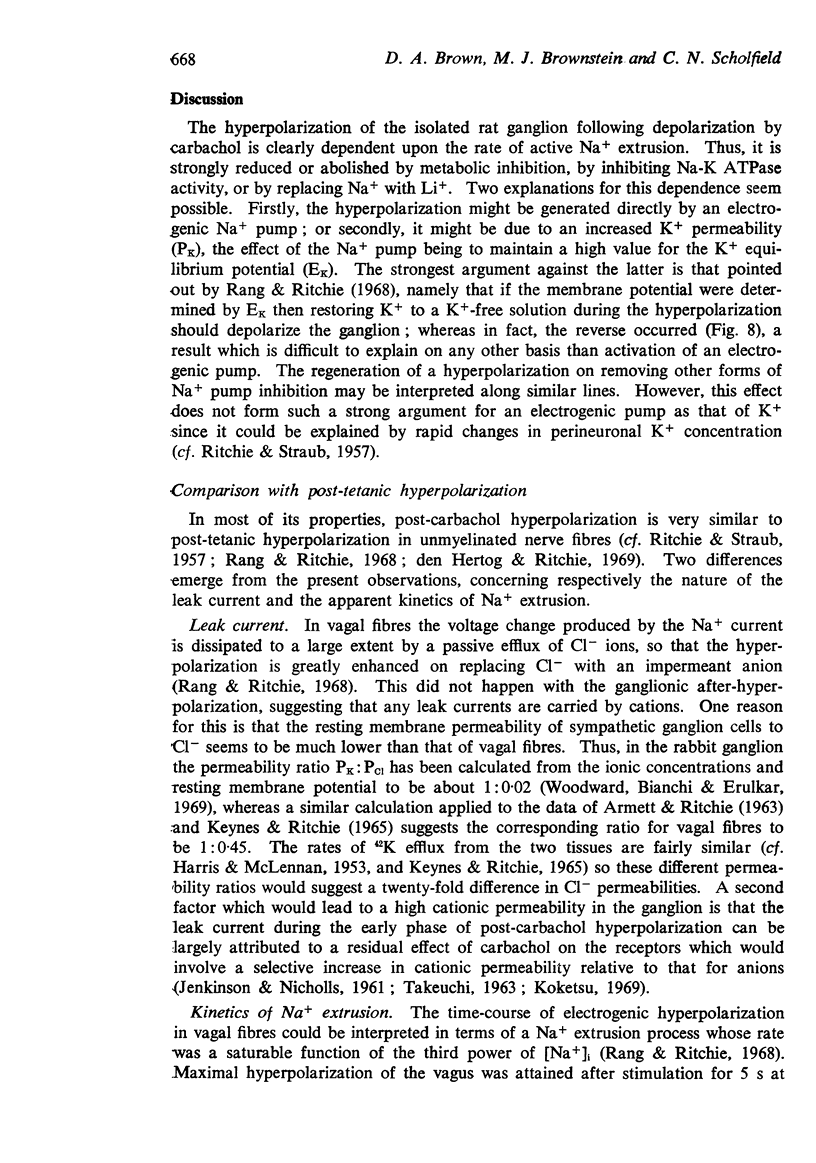
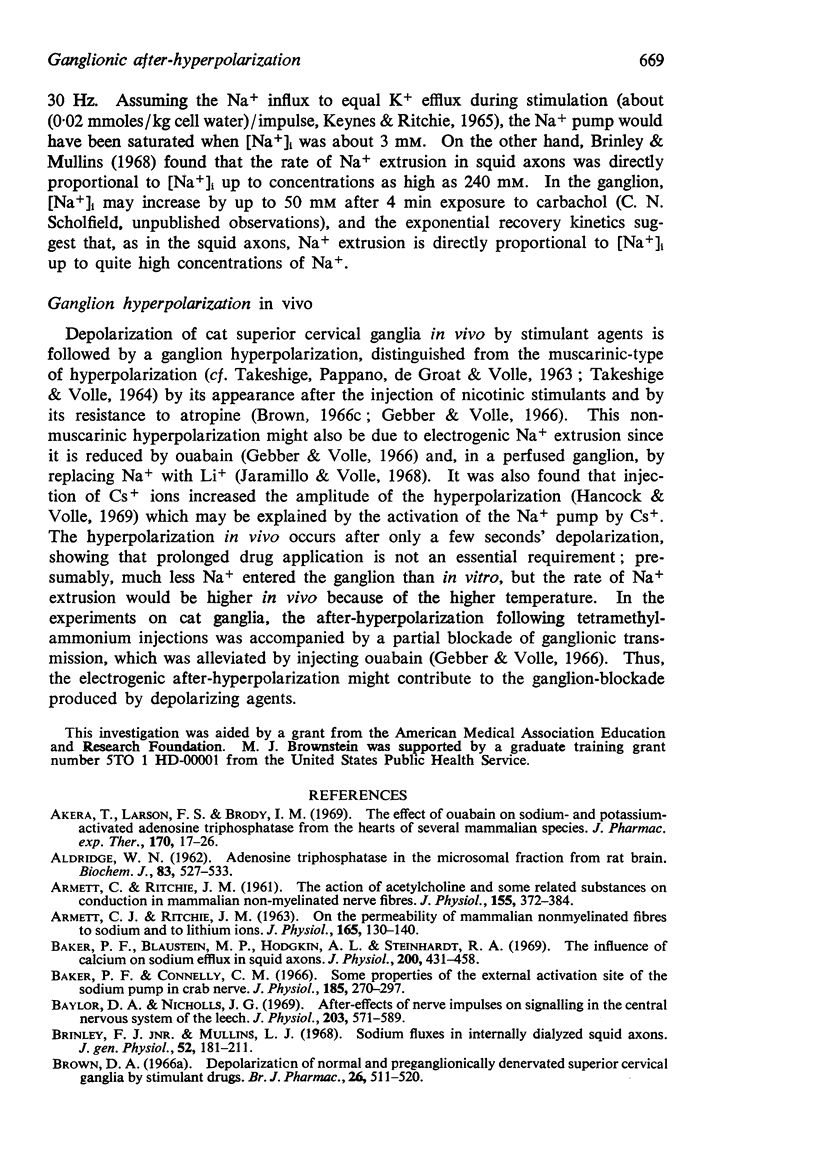
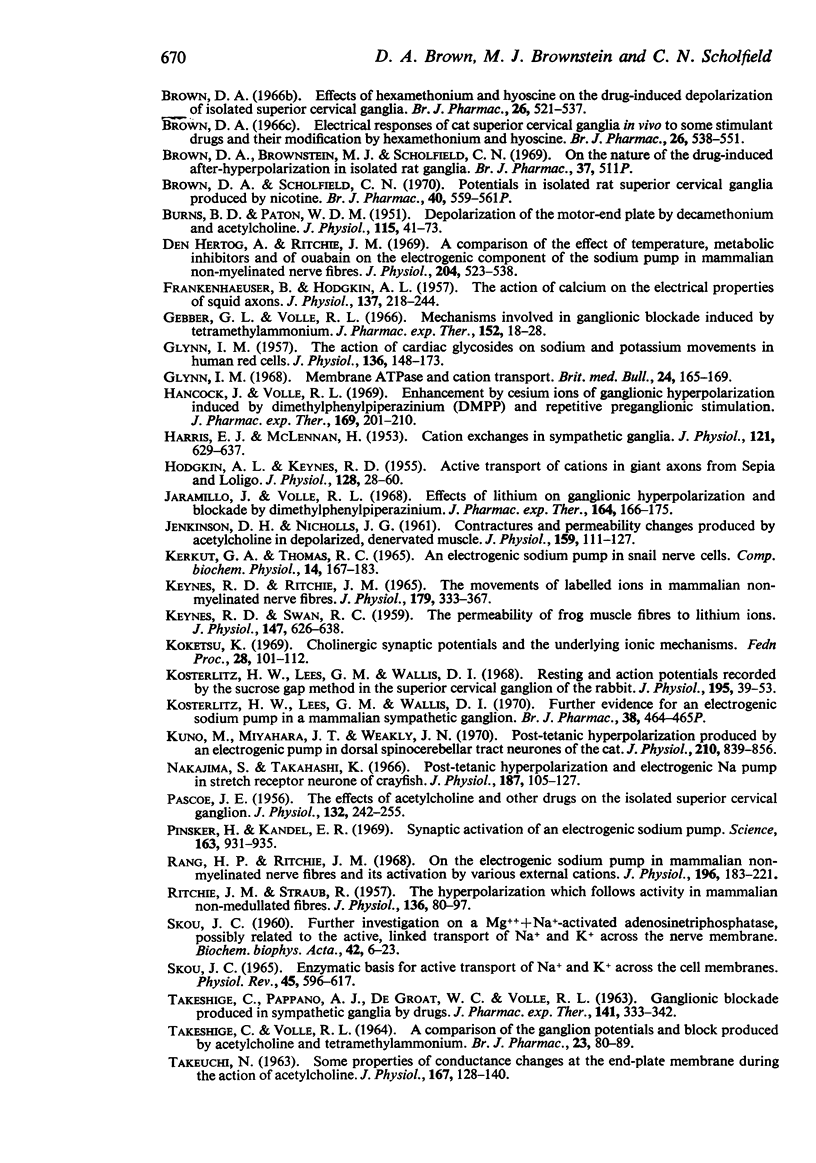
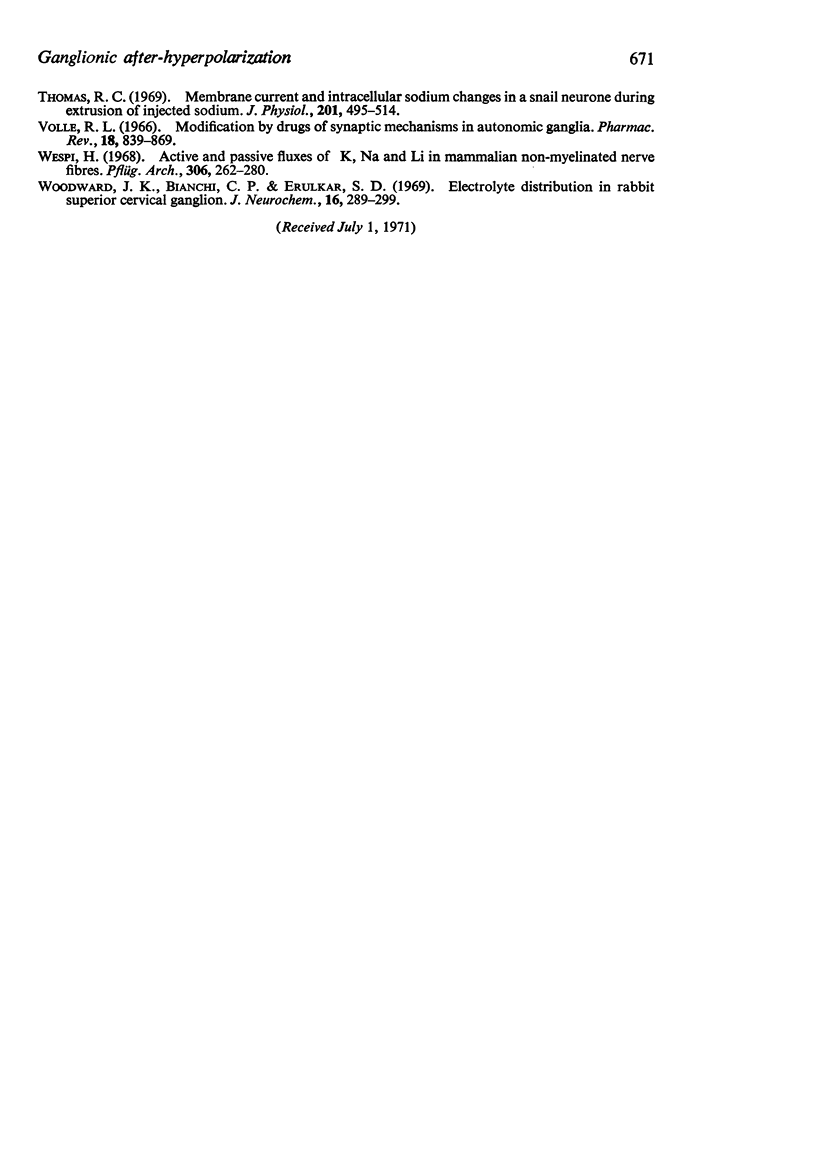
Selected References
These references are in PubMed. This may not be the complete list of references from this article.
- ALDRIDGE W. N. Adenosine triphosphatase in the microsomal fraction from rat brain. Biochem J. 1962 Jun;83:527–533. doi: 10.1042/bj0830527. [DOI] [PMC free article] [PubMed] [Google Scholar]
- ARMETT C. J., RITCHIE J. M. The action of acetylcholine and some related substances on conduction in mammalian non-myelinated nerve fibres. J Physiol. 1961 Feb;155:372–384. doi: 10.1113/jphysiol.1961.sp006634. [DOI] [PMC free article] [PubMed] [Google Scholar]
- Akera T., Larsen F. S., Brody T. M. The effect of ouabain on sodium- and potassium-activated adenosine triphosphatase from the hearts of several mammalian species. J Pharmacol Exp Ther. 1969 Nov;170(1):17–26. [PubMed] [Google Scholar]
- Armett C. J., Ritchie J. M. On the permeability of mammalian non-myelinated fibres to sodium and to lithium ions. J Physiol. 1963 Jan;165(1):130–140. doi: 10.1113/jphysiol.1963.sp007047. [DOI] [PMC free article] [PubMed] [Google Scholar]
- BURNS B. D., PATON W. D. M. Depolarization of the motor end-plate by decamethonium and acetylcholine. J Physiol. 1951 Sep;115(1):41–73. doi: 10.1113/jphysiol.1951.sp004652. [DOI] [PMC free article] [PubMed] [Google Scholar]
- Baker P. F., Blaustein M. P., Hodgkin A. L., Steinhardt R. A. The influence of calcium on sodium efflux in squid axons. J Physiol. 1969 Feb;200(2):431–458. doi: 10.1113/jphysiol.1969.sp008702. [DOI] [PMC free article] [PubMed] [Google Scholar]
- Baker P. F., Connelly C. M. Some properties of the external activation site of the sodium pump in crab nerve. J Physiol. 1966 Jul;185(2):270–297. doi: 10.1113/jphysiol.1966.sp007987. [DOI] [PMC free article] [PubMed] [Google Scholar]
- Baylor D. A., Nicholls J. G. After-effects of nerve impulses on signalling in the central nervous system of the leech. J Physiol. 1969 Aug;203(3):571–589. doi: 10.1113/jphysiol.1969.sp008880. [DOI] [PMC free article] [PubMed] [Google Scholar]
- Brinley F. J., Jr, Mullins L. J. Sodium fluxes in internally dialyzed squid axons. J Gen Physiol. 1968 Aug;52(2):181–211. doi: 10.1085/jgp.52.2.181. [DOI] [PMC free article] [PubMed] [Google Scholar]
- Brown D. A. Depolarization of normal and preganglionically denervated superior cervical ganglia by stimulant drugs. Br J Pharmacol Chemother. 1966 Mar;26(3):511–520. doi: 10.1111/j.1476-5381.1966.tb01833.x. [DOI] [PMC free article] [PubMed] [Google Scholar]
- Brown D. A. Effects of hexamethonium and hyoscine on the drug-induced depolarization of isolated superior cervical ganglia. Br J Pharmacol Chemother. 1966 Mar;26(3):521–537. doi: 10.1111/j.1476-5381.1966.tb01834.x. [DOI] [PMC free article] [PubMed] [Google Scholar]
- Brown D. A. Electrical responses of cat superior cervical ganglia in vivo to some stimulant drugs and their modification by hexamethonium and hyoscine. Br J Pharmacol Chemother. 1966 Mar;26(3):538–551. doi: 10.1111/j.1476-5381.1966.tb01835.x. [DOI] [PMC free article] [PubMed] [Google Scholar]
- Brown D. A., Scholfield C. N. Potentials in isolated rat superior cervical ganglia produced by nicotine. Br J Pharmacol. 1970 Nov;40(3):559P–561P. [PMC free article] [PubMed] [Google Scholar]
- FRANKENHAEUSER B., HODGKIN A. L. The action of calcium on the electrical properties of squid axons. J Physiol. 1957 Jul 11;137(2):218–244. doi: 10.1113/jphysiol.1957.sp005808. [DOI] [PMC free article] [PubMed] [Google Scholar]
- GLYNN I. M. The action of cardiac glycosides on sodium and potassium movements in human red cells. J Physiol. 1957 Apr 3;136(1):148–173. doi: 10.1113/jphysiol.1957.sp005749. [DOI] [PMC free article] [PubMed] [Google Scholar]
- Gebber G. L., Volle R. L. Mechanisms involved in ganglionic blockade induced by tetramethylammonium. J Pharmacol Exp Ther. 1966 Apr;152(1):18–28. [PubMed] [Google Scholar]
- Glynn I. M. Membrane adenosine triphosphatase and cation transport. Br Med Bull. 1968 May;24(2):165–169. doi: 10.1093/oxfordjournals.bmb.a070620. [DOI] [PubMed] [Google Scholar]
- HARRIS E. J., McLENNAN H. Cation exchanges in sympathetic ganglia. J Physiol. 1953 Sep;121(3):629–637. doi: 10.1113/jphysiol.1953.sp004970. [DOI] [PMC free article] [PubMed] [Google Scholar]
- HODGKIN A. L., KEYNES R. D. Active transport of cations in giant axons from Sepia and Loligo. J Physiol. 1955 Apr 28;128(1):28–60. doi: 10.1113/jphysiol.1955.sp005290. [DOI] [PMC free article] [PubMed] [Google Scholar]
- Hancock J. C., Volle R. L. Enhancement by cesium ions of ganglionic hyperpolarization induced by dimethylphenylpiperazinium (DMPP) and repetitive preganglionic stimulation. J Pharmacol Exp Ther. 1969 Oct;169(2):201–210. [PubMed] [Google Scholar]
- JENKINSON D. H., NICHOLLS J. G. Contractures and permeability changes produced by acetylcholine in depolarized denervated muscle. J Physiol. 1961 Nov;159:111–127. doi: 10.1113/jphysiol.1961.sp006796. [DOI] [PMC free article] [PubMed] [Google Scholar]
- Jaramillo J., Volle R. L. Effects of lithium on ganglionic hyperpolarization and blockade by dimethylphenylipiperazinium. J Pharmacol Exp Ther. 1968 Nov;164(1):166–175. [PubMed] [Google Scholar]
- KERKUT G. A., THOMAS R. C. AN ELECTROGENIC SODIUM PUMP IN SNAIL NERVE CELLS. Comp Biochem Physiol. 1965 Jan;14:167–183. doi: 10.1016/0010-406x(65)90017-4. [DOI] [PubMed] [Google Scholar]
- KEYNES R. D., SWAN R. C. The permeability of frog muscle fibres to lithium ions. J Physiol. 1959 Oct;147:626–638. doi: 10.1113/jphysiol.1959.sp006265. [DOI] [PMC free article] [PubMed] [Google Scholar]
- Keynes R. D., Ritchie J. M. The movements of labelled ions in mammalian non-myelinated nerve fibres. J Physiol. 1965 Jul;179(2):333–367. doi: 10.1113/jphysiol.1965.sp007666. [DOI] [PMC free article] [PubMed] [Google Scholar]
- Koketsu K. Cholinergic synaptic potentials and the underlying ionic mechasims. Fed Proc. 1969 Jan-Feb;28(1):101–112. [PubMed] [Google Scholar]
- Kosterlitz H. W., Lees G. M., Wallis D. I. Further evidence for an electrogenic sodium pump in a mammalian sympathetic ganglion. Br J Pharmacol. 1970 Feb;38(2):464P–465P. [PMC free article] [PubMed] [Google Scholar]
- Kosterlitz H. W., Lees G. M., Wallis D. I. Resting and action potentials recorded by the sucrose-gap method in the superior cervical ganglion of the rabbit. J Physiol. 1968 Mar;195(1):39–53. doi: 10.1113/jphysiol.1968.sp008445. [DOI] [PMC free article] [PubMed] [Google Scholar]
- Kuno M., Miyahara J. T., Weakly J. N. Post-tetanic hyperpolarization produced by an electrogenic pump in dorsal spinocerebellar tract neurones of the cat. J Physiol. 1970 Nov;210(4):839–855. doi: 10.1113/jphysiol.1970.sp009245. [DOI] [PMC free article] [PubMed] [Google Scholar]
- Nakajima S., Takahashi K. Post-tetanic hyperpolarization and electrogenic Na pump in stretch receptor neurone of crayfish. J Physiol. 1966 Nov;187(1):105–127. doi: 10.1113/jphysiol.1966.sp008078. [DOI] [PMC free article] [PubMed] [Google Scholar]
- PASCOE J. E. The effects of acetylcholine and other drugs on the isolated superior cervical ganglion. J Physiol. 1956 Apr 27;132(1):242–255. doi: 10.1113/jphysiol.1956.sp005519. [DOI] [PMC free article] [PubMed] [Google Scholar]
- Pinsker H., Kandel E. R. Synaptic activation of an electrogenic sodium pump. Science. 1969 Feb 28;163(3870):931–935. doi: 10.1126/science.163.3870.931. [DOI] [PubMed] [Google Scholar]
- RITCHIE J. M., STRAUB R. W. The hyperpolarization which follows activity in mammalian non-medullated fibres. J Physiol. 1957 Apr 3;136(1):80–97. doi: 10.1113/jphysiol.1957.sp005744. [DOI] [PMC free article] [PubMed] [Google Scholar]
- Rang H. P., Ritchie J. M. On the electrogenic sodium pump in mammalian non-myelinated nerve fibres and its activation by various external cations. J Physiol. 1968 May;196(1):183–221. doi: 10.1113/jphysiol.1968.sp008502. [DOI] [PMC free article] [PubMed] [Google Scholar]
- SKOU J. C. ENZYMATIC BASIS FOR ACTIVE TRANSPORT OF NA+ AND K+ ACROSS CELL MEMBRANE. Physiol Rev. 1965 Jul;45:596–617. doi: 10.1152/physrev.1965.45.3.596. [DOI] [PubMed] [Google Scholar]
- TAKESHIGE C., PAPPANO A. J., DEGROAT W. C., VOLLE R. L. GANGLIONIC BLOCKADE PRODUCED IN SYMPATHETIC GANGLIA BY CHOLINOMIMETIC DRUGS. J Pharmacol Exp Ther. 1963 Sep;141:333–342. [PubMed] [Google Scholar]
- TAKESHIGE C., VOLLE R. L. A COMPARISON OF THE GANGLION POTENTIALS AND BLOCK PRODUCED BY ACETYLCHOLINE AND TETRAMETHYLAMMONIUM. Br J Pharmacol Chemother. 1964 Aug;23:80–89. doi: 10.1111/j.1476-5381.1964.tb01568.x. [DOI] [PMC free article] [PubMed] [Google Scholar]
- TAKEUCHI N. Some properties of conductance changes at the end-plate membrane during the action of acetylcholine. J Physiol. 1963 Jun;167:128–140. doi: 10.1113/jphysiol.1963.sp007136. [DOI] [PMC free article] [PubMed] [Google Scholar]
- Thomas R. C. Membrane current and intracellular sodium changes in a snail neurone during extrusion of injected sodium. J Physiol. 1969 Apr;201(2):495–514. doi: 10.1113/jphysiol.1969.sp008769. [DOI] [PMC free article] [PubMed] [Google Scholar]
- Wespi H. H. Active transport and passive fluxes of K, Na, and Li in mammalian non-myelinated nerve fibres. Pflugers Arch. 1969;306(3):262–280. doi: 10.1007/BF00592437. [DOI] [PubMed] [Google Scholar]
- Woodward J. K., Bianchi C. P., Erulkar S. D. Electrolyte distribution in rabbit superior cervical ganglion. J Neurochem. 1969 Mar;16(3):289–299. doi: 10.1111/j.1471-4159.1969.tb10367.x. [DOI] [PubMed] [Google Scholar]
- den Hertog A., Ritchie J. M. A comparison of the effect of temperature, metabolic inhibitors and of ouabain on the electrogenic componen of the sodium pump in mammalian non-myelinated nerve fibres. J Physiol. 1969 Oct;204(3):523–538. doi: 10.1113/jphysiol.1969.sp008929. [DOI] [PMC free article] [PubMed] [Google Scholar]