Abstract
A pathway of succinate fermentation to acetate and butanoate (butyrate) in Clostridium kluyveri has been supported by the results of 13C nuclear magnetic resonance studies of the metabolic end products of growth and the detection of dehydrogenase activities involved in the conversion of succinate to 4-hydroxybutanoate (succinic semialdehyde dehydrogenase and 4-hydroxybutanoate dehydrogenase). C. kluyveri fermented [1,4-13C]succinate primarily to [1-13C]acetate, [2-13C]acetate, and [1,4-13C]butanoate. Any pathway proposed for this metabolism must account for the reduction of a carboxyl group to a methyl group. Succinic semialdehyde dehydrogenase activity was demonstrated after separation of the crude extracts of cells grown on succinate and ethanol (succinate cells) by anaerobic nondenaturing polyacrylamide gel electrophoresis. 4-Hydroxybutanoate dehydrogenase activity in crude extracts of succinate cells was detected and characterized. Neither activity was found in cells grown on acetate and ethanol (acetate cells). Analysis of cell extracts from acetate cells and succinate cells by sodium dodecyl sulfate-polyacrylamide gel electrophoreses showed that several proteins were present in succinate cell extracts that were not present in acetate cell extracts. In addition to these changes in protein composition, less ethanol dehydrogenase and hydrogenase activity was present in the crude extracts from succinate cells than in the crude extracts from acetate cells. These data support the hypothesis that C. kluyveri uses succinate as an electron acceptor for the reducing equivalents generated from the ATP-producing oxidation of ethanol.
Full text
PDF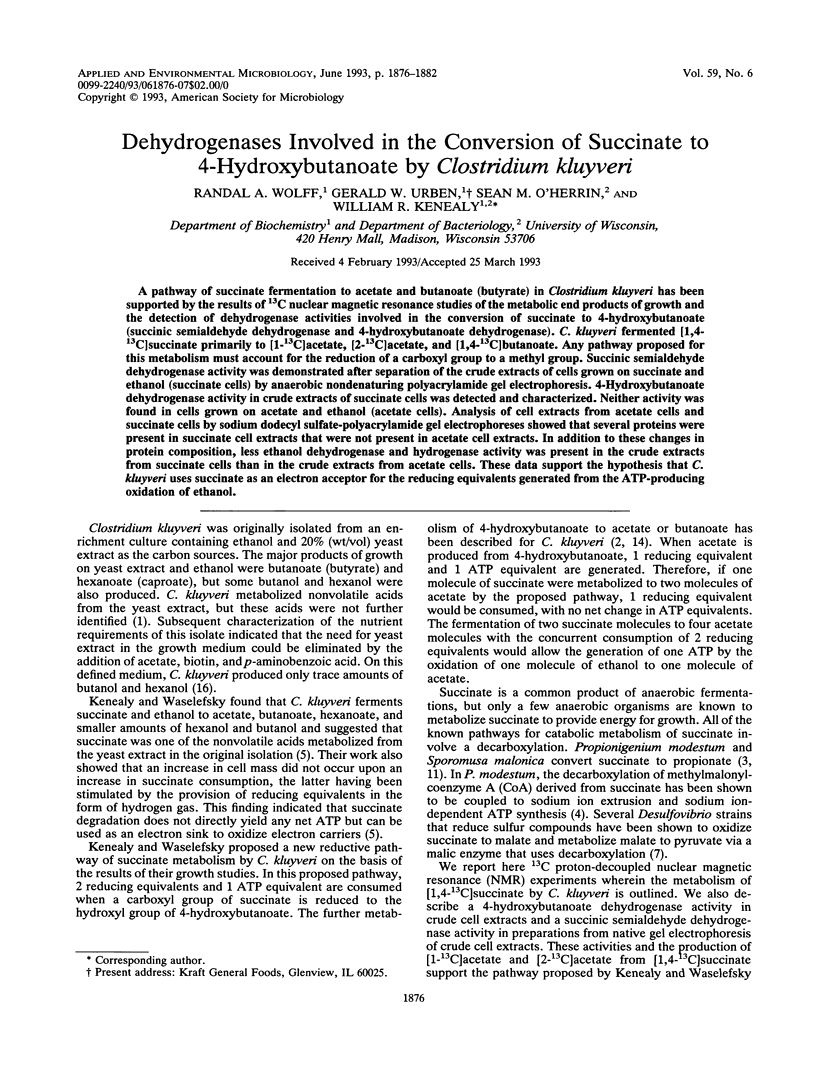
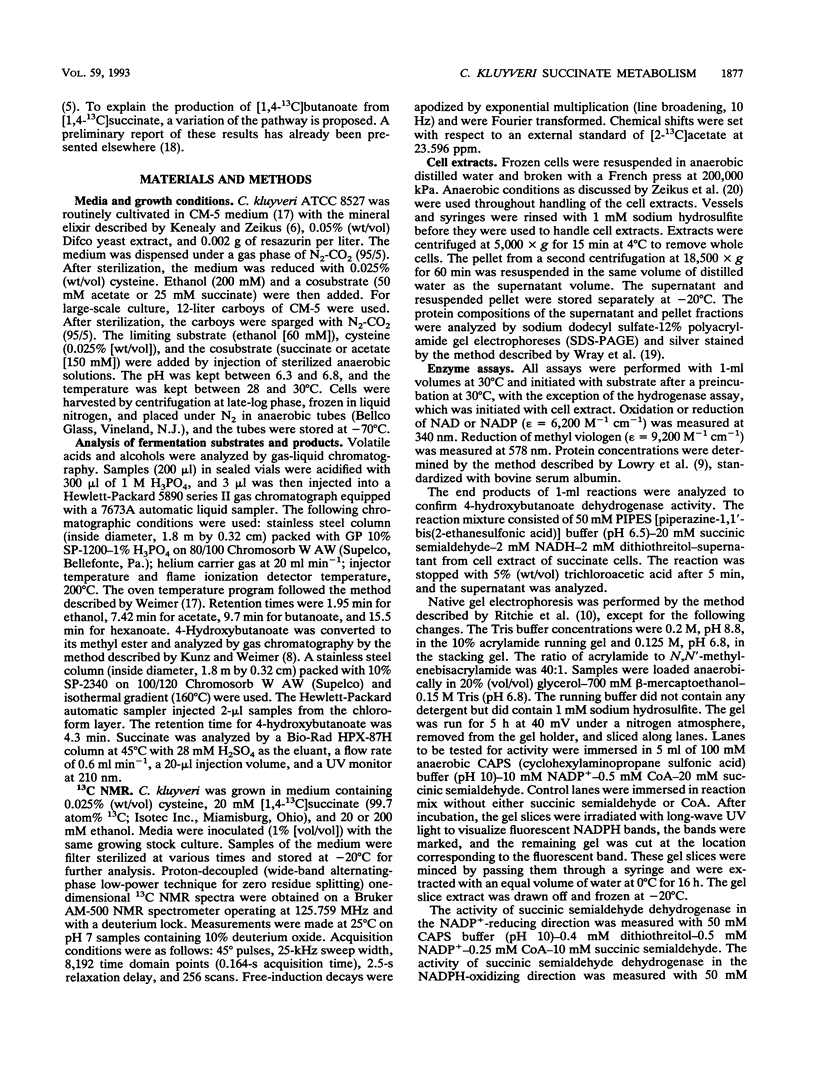
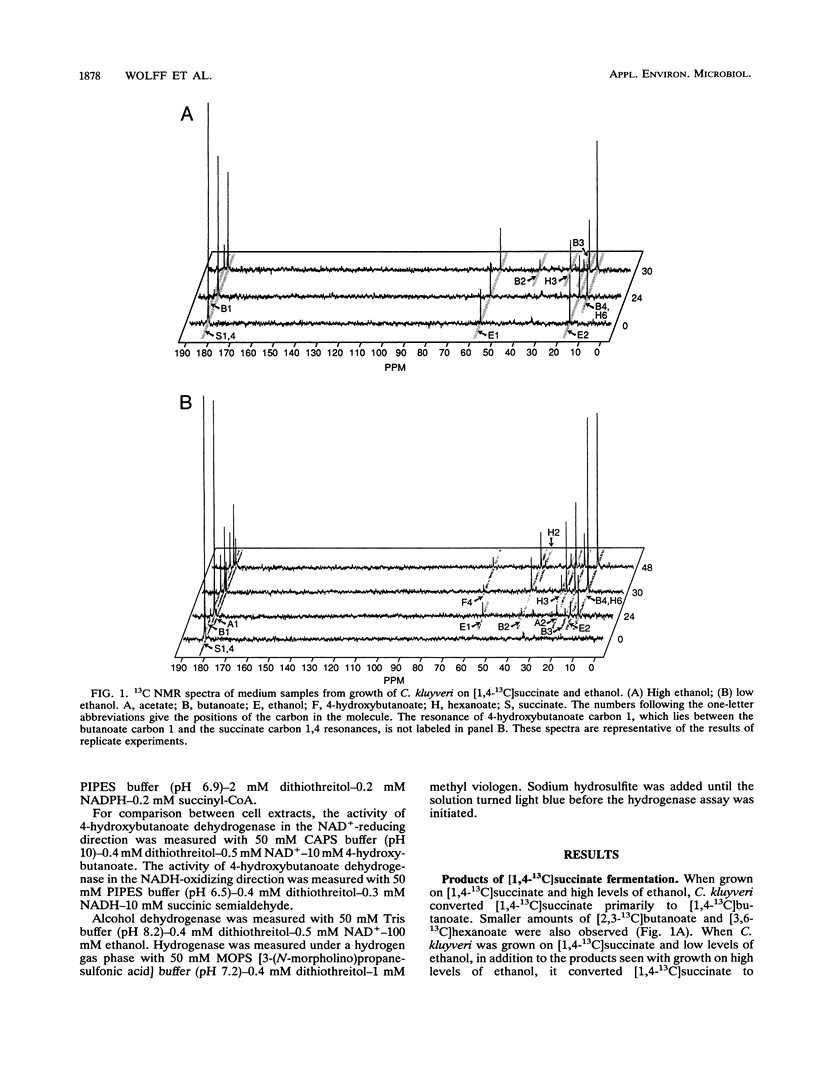
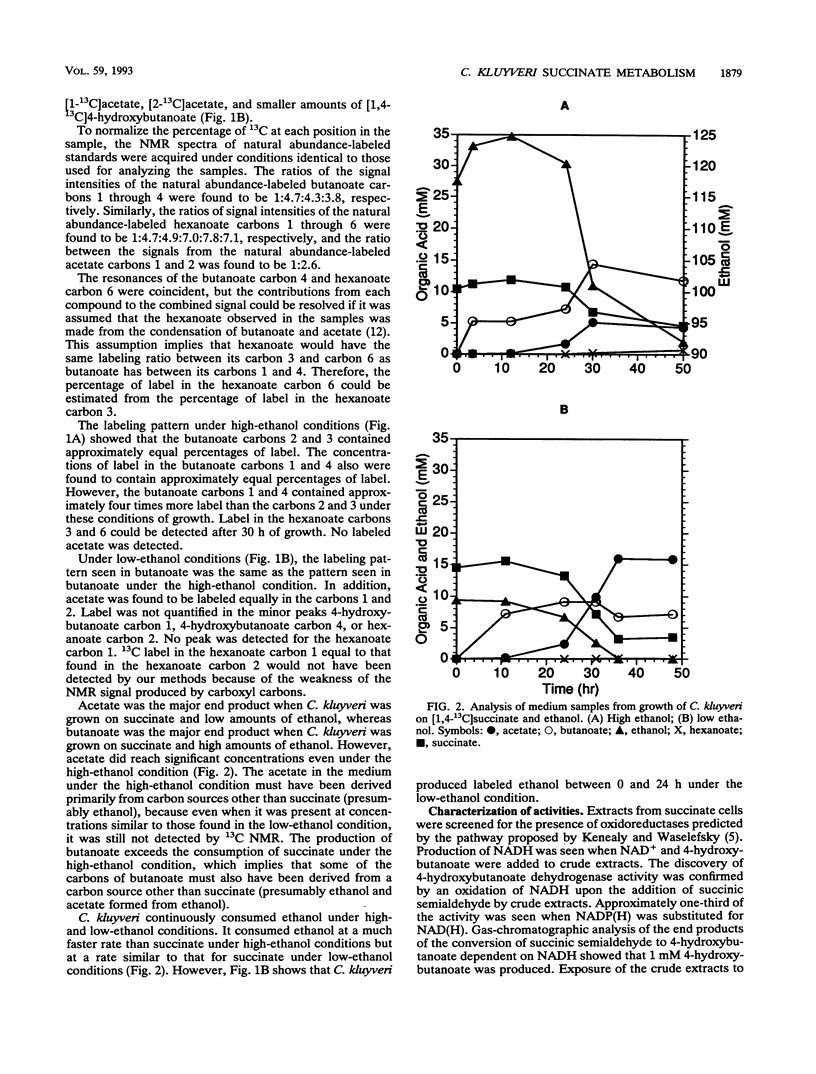
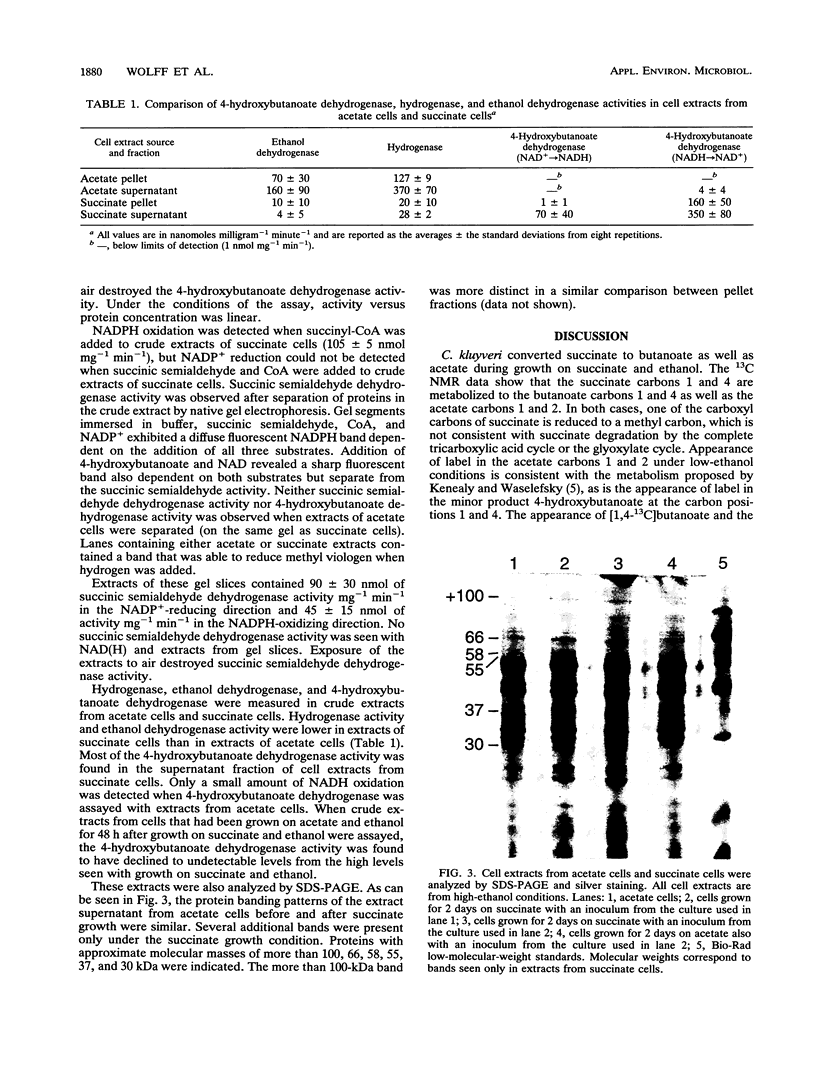
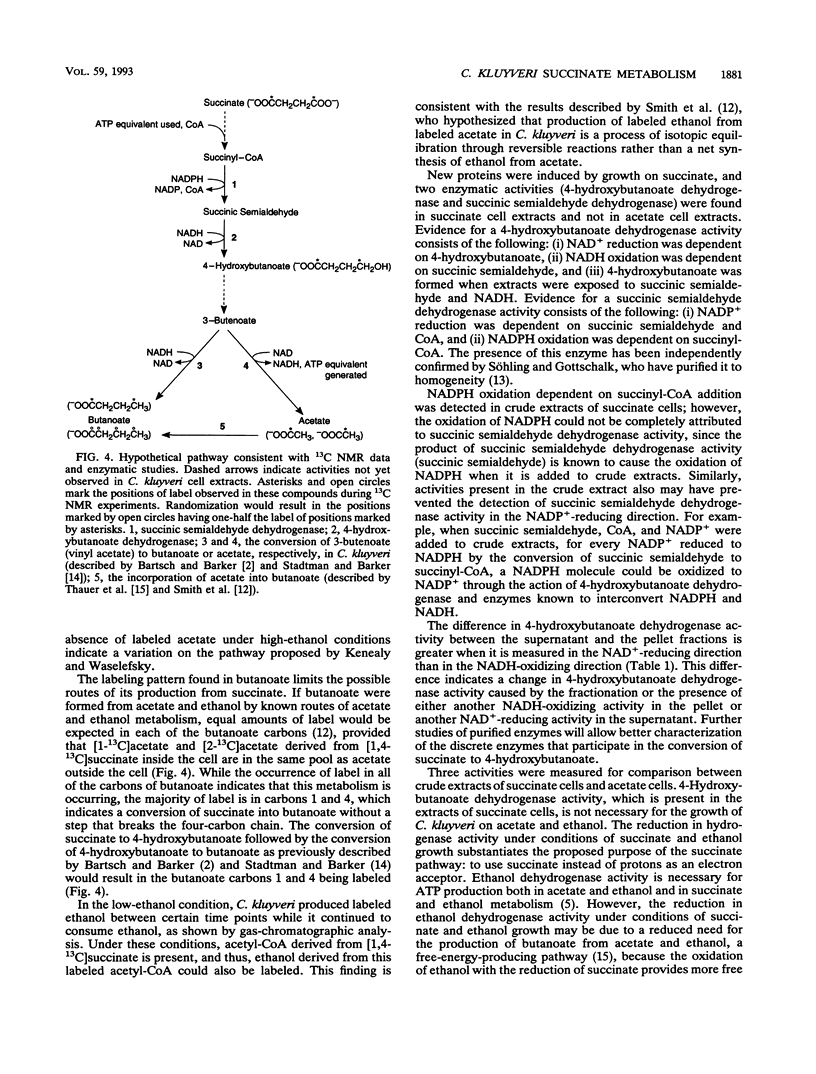
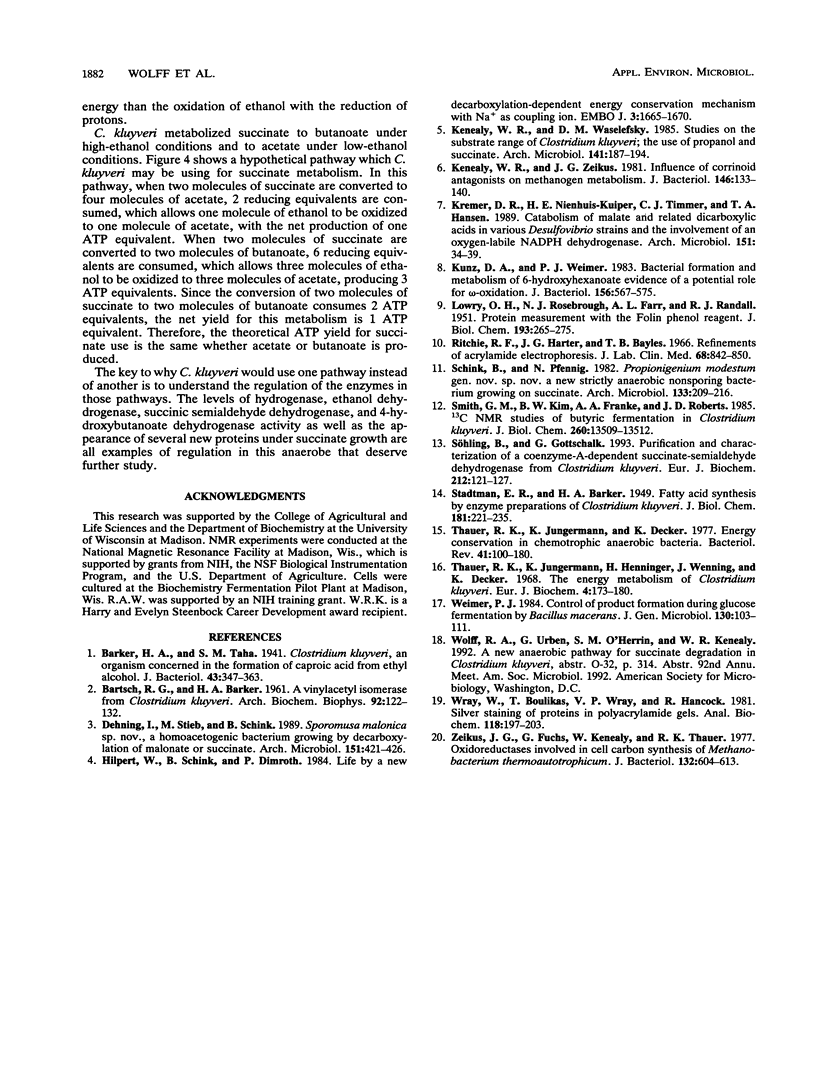
Images in this article
Selected References
These references are in PubMed. This may not be the complete list of references from this article.
- BARTSCH R. G., BARKER H. A. A vinylacetyl isomerase from Clostridium kluyveri. Arch Biochem Biophys. 1961 Jan;92:122–132. doi: 10.1016/0003-9861(61)90226-0. [DOI] [PubMed] [Google Scholar]
- Barker H. A., Taha S. M. Clostridium kluyverii, an Organism Concerned in the Formation of Caproic Acid from Ethyl Alcohol. J Bacteriol. 1942 Mar;43(3):347–363. doi: 10.1128/jb.43.3.347-363.1942. [DOI] [PMC free article] [PubMed] [Google Scholar]
- Hilpert W., Schink B., Dimroth P. Life by a new decarboxylation-dependent energy conservation mechanism with Na as coupling ion. EMBO J. 1984 Aug;3(8):1665–1670. doi: 10.1002/j.1460-2075.1984.tb02030.x. [DOI] [PMC free article] [PubMed] [Google Scholar]
- Kenealy W., Zeikus J. G. Influence of corrinoid antagonists on methanogen metabolism. J Bacteriol. 1981 Apr;146(1):133–140. doi: 10.1128/jb.146.1.133-140.1981. [DOI] [PMC free article] [PubMed] [Google Scholar]
- Kunz D. A., Weimer P. J. Bacterial formation and metabolism of 6-hydroxyhexanoate: evidence of a potential role for omega-oxidation. J Bacteriol. 1983 Nov;156(2):567–575. doi: 10.1128/jb.156.2.567-575.1983. [DOI] [PMC free article] [PubMed] [Google Scholar]
- LOWRY O. H., ROSEBROUGH N. J., FARR A. L., RANDALL R. J. Protein measurement with the Folin phenol reagent. J Biol Chem. 1951 Nov;193(1):265–275. [PubMed] [Google Scholar]
- Ritchie R. F., Harter J. G., Bayles T. B. Refinements of acrylamide electrophoresis. J Lab Clin Med. 1966 Nov;68(5):842–850. [PubMed] [Google Scholar]
- STADTMAN E. R., BARKER H. A. Fatty acid synthesis by enzyme preparations of Clostridium kluyveri; a consideration of postulated 4-carbon intermediates in butyrate synthesis. J Biol Chem. 1949 Nov;181(1):221–235. [PubMed] [Google Scholar]
- Smith G. M., Kim B. W., Franke A. A., Roberts J. D. 13C NMR studies of butyric fermentation in Clostridium kluyveri. J Biol Chem. 1985 Nov 5;260(25):13509–13512. [PubMed] [Google Scholar]
- Söhling B., Gottschalk G. Purification and characterization of a coenzyme-A-dependent succinate-semialdehyde dehydrogenase from Clostridium kluyveri. Eur J Biochem. 1993 Feb 15;212(1):121–127. doi: 10.1111/j.1432-1033.1993.tb17641.x. [DOI] [PubMed] [Google Scholar]
- Thauer R. K., Jungermann K., Decker K. Energy conservation in chemotrophic anaerobic bacteria. Bacteriol Rev. 1977 Mar;41(1):100–180. doi: 10.1128/br.41.1.100-180.1977. [DOI] [PMC free article] [PubMed] [Google Scholar]
- Thauer R. K., Jungermann K., Henninger H., Wenning J., Decker K. The energy metabolism of Clostridium kluyveri. Eur J Biochem. 1968 Apr 3;4(2):173–180. doi: 10.1111/j.1432-1033.1968.tb00189.x. [DOI] [PubMed] [Google Scholar]
- Wray W., Boulikas T., Wray V. P., Hancock R. Silver staining of proteins in polyacrylamide gels. Anal Biochem. 1981 Nov 15;118(1):197–203. doi: 10.1016/0003-2697(81)90179-2. [DOI] [PubMed] [Google Scholar]
- Zeikus J. G., Fuchs G., Kenealy W., Thauer R. K. Oxidoreductases involved in cell carbon synthesis of Methanobacterium thermoautotrophicum. J Bacteriol. 1977 Nov;132(2):604–613. doi: 10.1128/jb.132.2.604-613.1977. [DOI] [PMC free article] [PubMed] [Google Scholar]