Abstract
Two strains of Desulfovibrio desulfuricans, one known to synthesize monomethylmercury from ionic mercury, were grown to determine methylmercury toxicity and for comparison with an anaerobic strain of Clostridium pasteurianum, a H2 producer, and with the broad-spectrum mercury-resistant Pseudomonas putida strain FB-1, capable of degrading 1 μg of methylmercury to methane and elemental mercury in 2 h. The CH3HgCl resistance of D. desulfuricans strains was 10 times that of P. putida FB-1 and 100 times that of C. pasteurianum. The methylmercury resistance of D. desulfuricans was related to the disappearance of methylmercury from cultures by transformation to dimethylmercury, metacinnabar, methane, and traces of ionic mercury. During a 15-day experiment the kinetics of the two volatile compounds dimethylmercury [(CH3)2Hg] and methane were monitored in the liquid by a specific new technique with purge-and-trap gas chromatography in line with Fourier transform infrared spectroscopy and in the headspace by gas chromatography with flame ionization detection. Insoluble metacinnabar (cubic HgS) of biological origin was detected by X-ray diffractometry in the gray precipitate from the insoluble residue of the pellet of a 1-liter culture spiked with 100 mg of CH3HgCl. This was compared with a 1-liter culture of D. desulfuricans LS spiked with 100 mg of HgCl2. In a further experiment, it was demonstrated that insoluble, decomposable, white dimethylmercury sulfide [(CH3Hg)2S] formed instantly in the reaction of methylmercury with hydrogen sulfide. This organomercurial was extracted with chloroform and identified by gas chromatography in line with mass spectrometry. The D. desulfuricans strains were resistant to high concentrations of methylmercury because they produced insoluble dimethylmercury sulfide, which slowly decomposed under anaerobic conditions to metacinnabar and volatilized to dimethylmercury and methane between pHs 6.2 and 6.5 for high (4.5-g · liter-1) or low (0.09-g · liter-1) sulfate contents. Methane was produced from CH3HgCl at a lower rate than by the broad-spectrum Hg-resistant P. putida strain FB-1.
Full text
PDF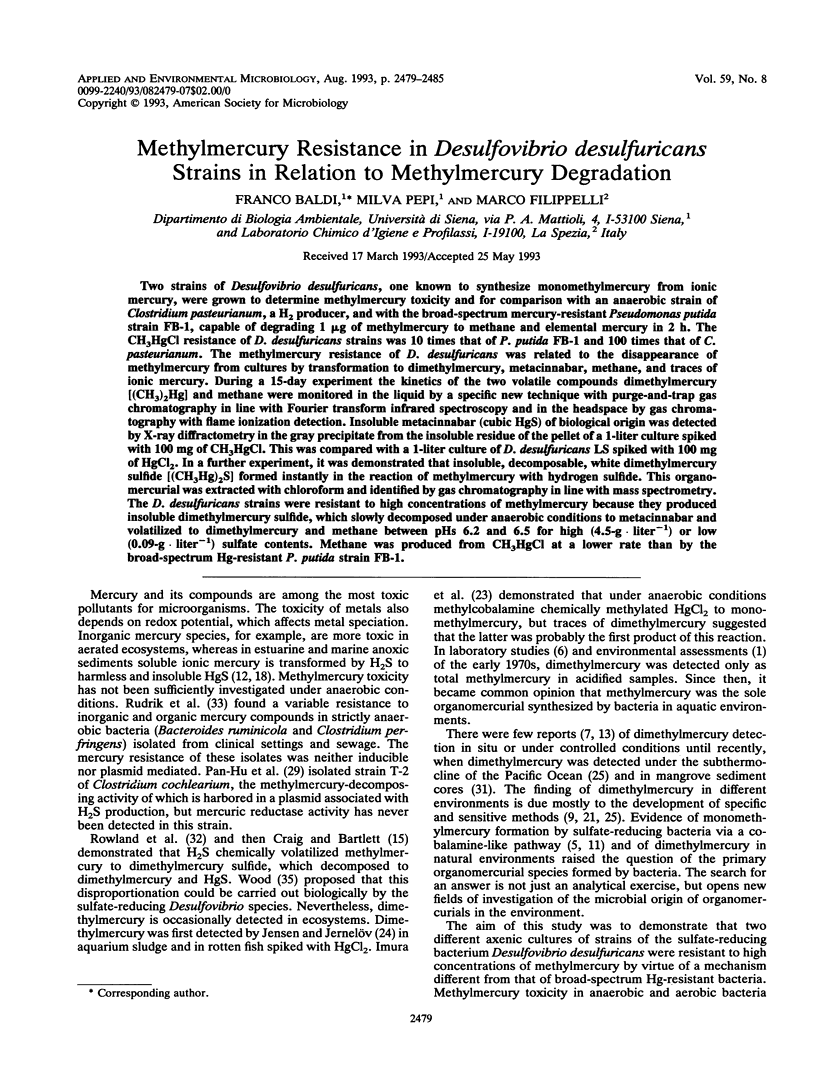
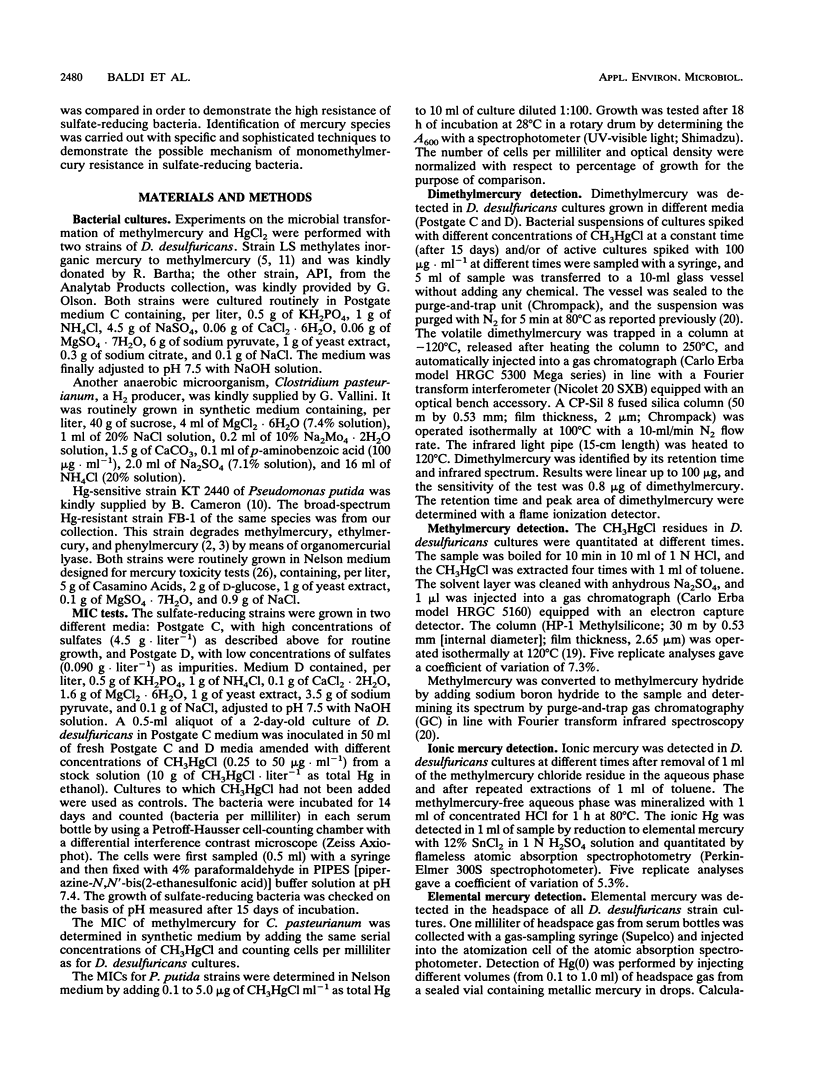
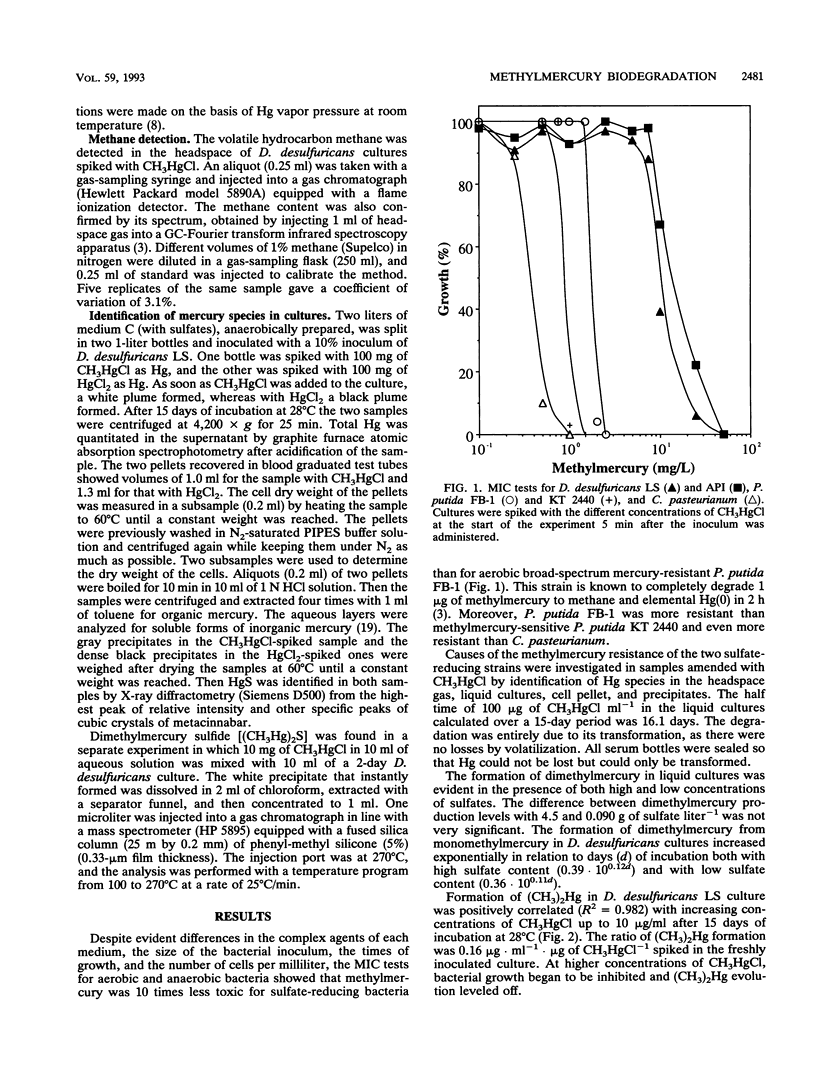
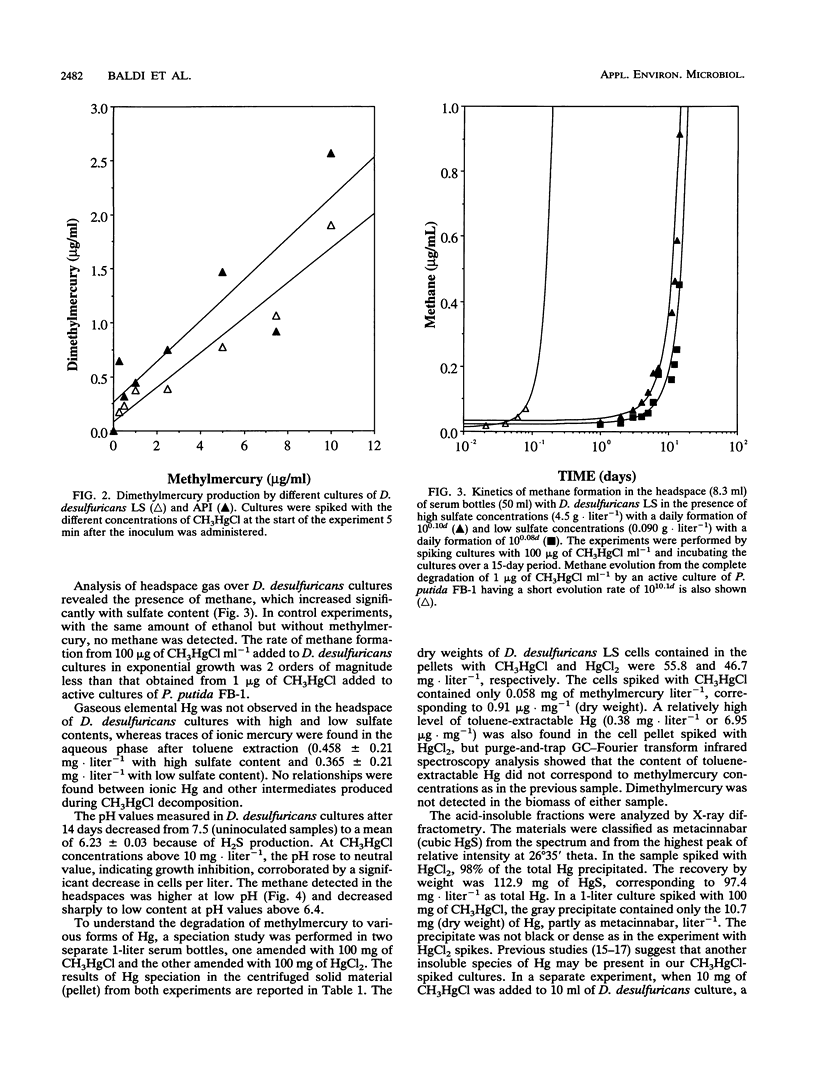
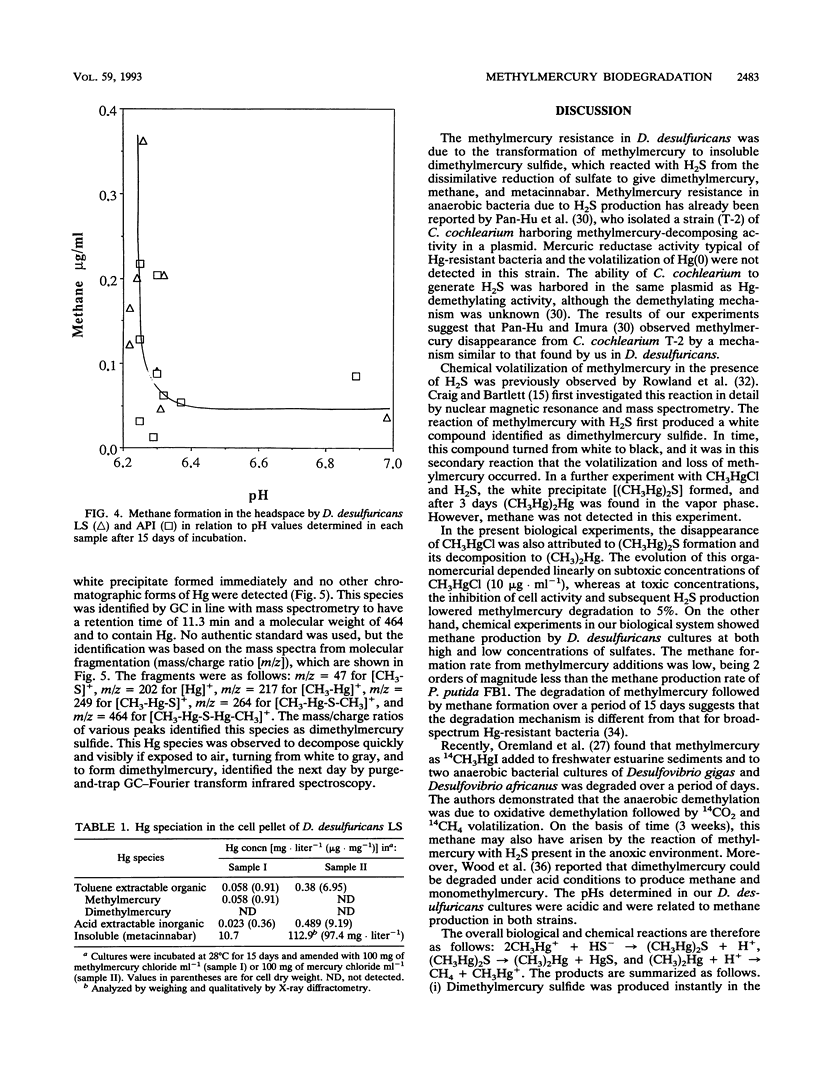
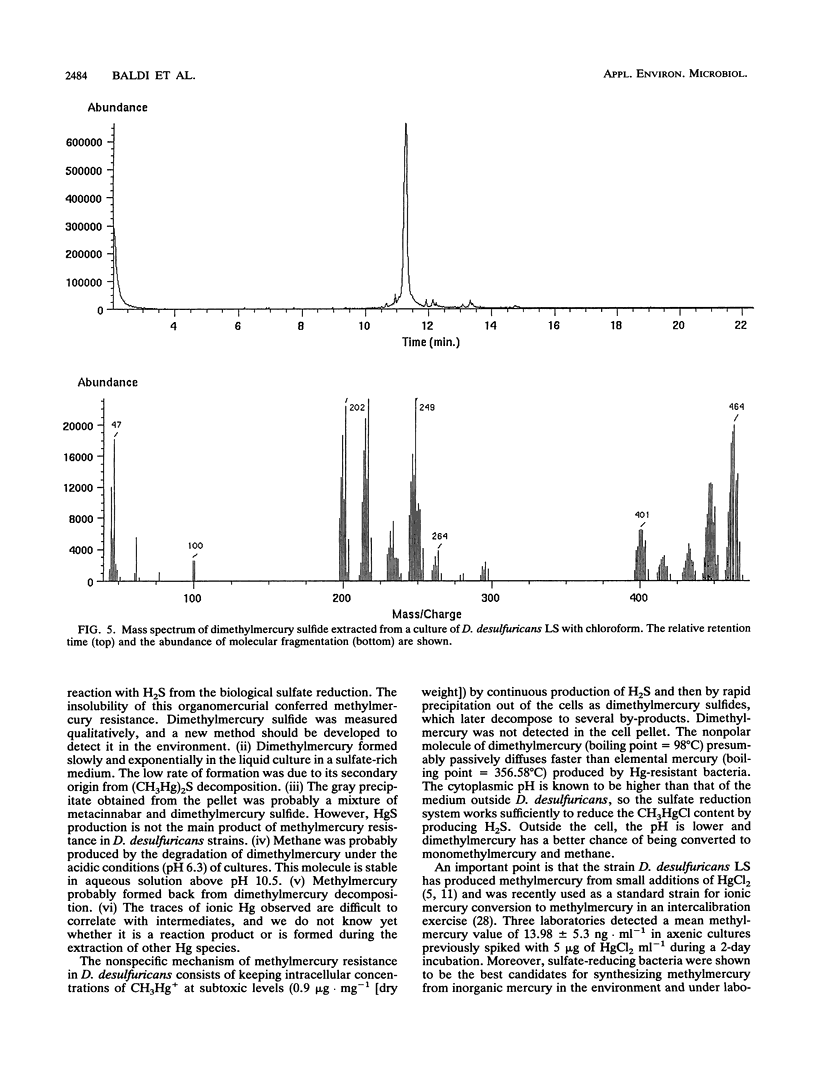
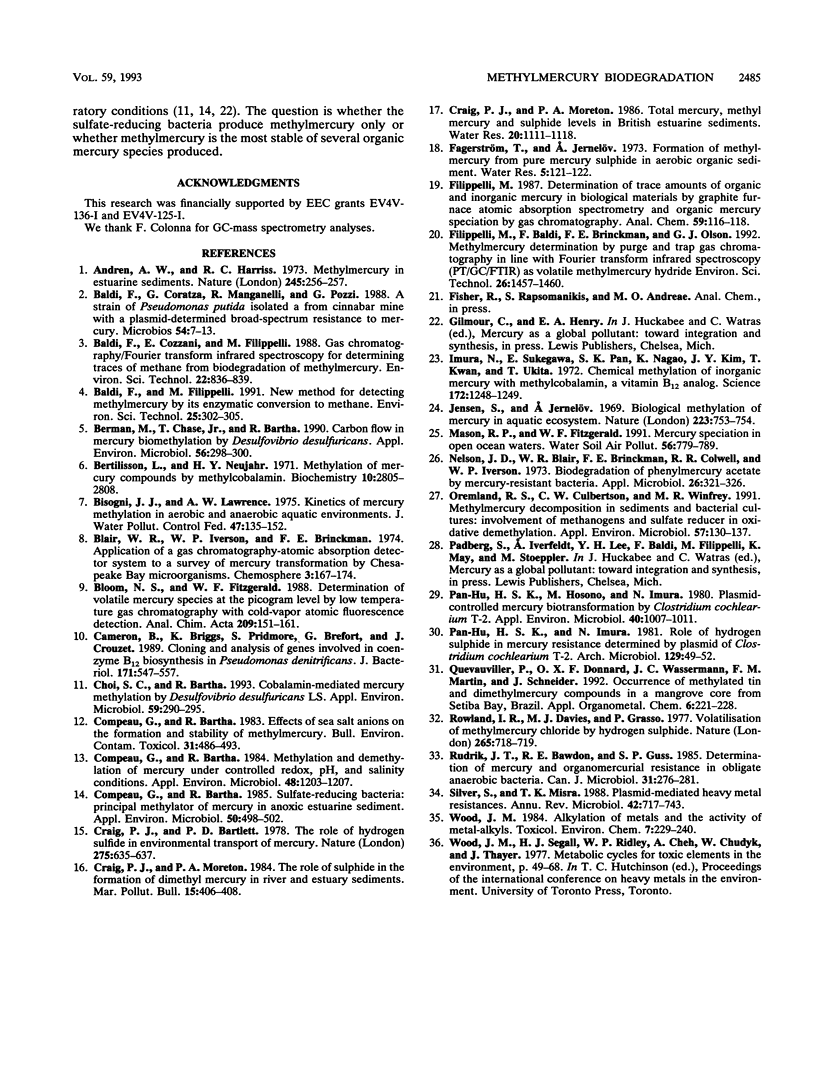
Selected References
These references are in PubMed. This may not be the complete list of references from this article.
- Berman M., Chase T., Bartha R. Carbon Flow in Mercury Biomethylation by Desulfovibrio desulfuricans. Appl Environ Microbiol. 1990 Jan;56(1):298–300. doi: 10.1128/aem.56.1.298-300.1990. [DOI] [PMC free article] [PubMed] [Google Scholar]
- Bertilsson L., Neujahr H. Y. Methylation of mercury compounds by methylcobalamin. Biochemistry. 1971 Jul 6;10(14):2805–2808. doi: 10.1021/bi00790a024. [DOI] [PubMed] [Google Scholar]
- Bisogni J. J., Jr, Lawrence A. W. Kinetics of mercury methylation in aerobic and anaerobic aquatic environments. J Water Pollut Control Fed. 1975 Jan;47(1):135–152. [PubMed] [Google Scholar]
- Cameron B., Briggs K., Pridmore S., Brefort G., Crouzet J. Cloning and analysis of genes involved in coenzyme B12 biosynthesis in Pseudomonas denitrificans. J Bacteriol. 1989 Jan;171(1):547–557. doi: 10.1128/jb.171.1.547-557.1989. [DOI] [PMC free article] [PubMed] [Google Scholar]
- Choi S. C., Bartha R. Cobalamin-mediated mercury methylation by Desulfovibrio desulfuricans LS. Appl Environ Microbiol. 1993 Jan;59(1):290–295. doi: 10.1128/aem.59.1.290-295.1993. [DOI] [PMC free article] [PubMed] [Google Scholar]
- Compeau G. C., Bartha R. Sulfate-reducing bacteria: principal methylators of mercury in anoxic estuarine sediment. Appl Environ Microbiol. 1985 Aug;50(2):498–502. doi: 10.1128/aem.50.2.498-502.1985. [DOI] [PMC free article] [PubMed] [Google Scholar]
- Compeau G., Bartha R. Effects of sea salt anions on the formation and stability of methylmercury. Bull Environ Contam Toxicol. 1983 Oct;31(4):486–493. doi: 10.1007/BF01622282. [DOI] [PubMed] [Google Scholar]
- Compeau G., Bartha R. Methylation and demethylation of mercury under controlled redox, pH and salinity conditions. Appl Environ Microbiol. 1984 Dec;48(6):1203–1207. doi: 10.1128/aem.48.6.1203-1207.1984. [DOI] [PMC free article] [PubMed] [Google Scholar]
- Filippelli M. Determination of trace amounts of organic and inorganic mercury in biological materials by graphite furnace atomic absorption spectrometry and organic mercury speciation by gas chromatography. Anal Chem. 1987 Jan 1;59(1):116–118. doi: 10.1021/ac00128a024. [DOI] [PubMed] [Google Scholar]
- Imura N., Sukegawa E., Pan S. K., Nagao K., Kim J. Y., Kwan T., Ukita T. Chemical methylation of inorganic mercury with methylcobalamin, a vitamin B12 analog. Science. 1971 Jun 18;172(3989):1248–1249. doi: 10.1126/science.172.3989.1248. [DOI] [PubMed] [Google Scholar]
- Jensen S., Jernelöv A. Biological methylation of mercury in aquatic organisms. Nature. 1969 Aug 16;223(5207):753–754. doi: 10.1038/223753a0. [DOI] [PubMed] [Google Scholar]
- Nelson J. D., Blair W., Brinckman F. E., Colwell R. R., Iverson W. P. Biodegradation of phenylmercuric acetate by mercury-resistant bacteria. Appl Microbiol. 1973 Sep;26(3):321–326. doi: 10.1128/am.26.3.321-326.1973. [DOI] [PMC free article] [PubMed] [Google Scholar]
- Oremland R. S., Culbertson C. W., Winfrey M. R. Methylmercury decomposition in sediments and bacterial cultures: involvement of methanogens and sulfate reducers in oxidative demethylation. Appl Environ Microbiol. 1991 Jan;57(1):130–137. doi: 10.1128/aem.57.1.130-137.1991. [DOI] [PMC free article] [PubMed] [Google Scholar]
- Pan-Hou H. S., Hosono M., Imura N. Plasmid-controlled mercury biotransformation by Clostridium cochlearium T-2. Appl Environ Microbiol. 1980 Dec;40(6):1007–1011. doi: 10.1128/aem.40.6.1007-1011.1980. [DOI] [PMC free article] [PubMed] [Google Scholar]
- Pan-Hou H. S., Imura N. Role of hydrogen sulfide in mercury resistance determined by plasmid of Clostridium cochlearium T-2. Arch Microbiol. 1981 Mar;129(1):49–52. doi: 10.1007/BF00417179. [DOI] [PubMed] [Google Scholar]
- Rowland I. R., Davies M. J., Grasso P. Volatilisation of methylmercuric chloride by hydrogen sulphide. Nature. 1977 Feb 24;265(5596):718–719. doi: 10.1038/265718a0. [DOI] [PubMed] [Google Scholar]
- Rudrik J. T., Bawdon R. E., Guss S. P. Determination of mercury and organomercurial resistance in obligate anaerobic bacteria. Can J Microbiol. 1985 Mar;31(3):276–281. doi: 10.1139/m85-051. [DOI] [PubMed] [Google Scholar]
- Silver S., Misra T. K. Plasmid-mediated heavy metal resistances. Annu Rev Microbiol. 1988;42:717–743. doi: 10.1146/annurev.mi.42.100188.003441. [DOI] [PubMed] [Google Scholar]