Abstract
In Saccharomyces cerevisiae, maltose is transported by a proton symport mechanism, whereas glucose transport occurs via facilitated diffusion. The energy requirement for maltose transport was evaluated with a metabolic model based on an experimental value of YATP for growth on glucose and an ATP requirement for maltose transport of 1 mol.mol-1. The predictions of the model were verified experimentally with anaerobic, sugar-limited chemostat cultures growing on a range of maltose-glucose mixtures at a fixed dilution rate of 0.1 h-1. The biomass yield (grams of cells.gram of sugar-1) decreased linearly with increasing amounts of maltose in the mixture. The yield was 25% lower during growth on maltose than during that on glucose, in agreement with the model predictions. During sugar-limited growth, the residual concentrations of maltose and glucose in the culture increased in proportion to their relative concentrations in the medium feed. From the residual maltose concentration, the in situ rates of maltose consumption by cultures, and the Km of the maltose carrier for maltose, it was calculated that the amount of this carrier was proportional to the in situ maltose consumption rate. This was also found for the amount of intracellular maltose. These two maltose-specific enzymes therefore exert high control over the maltose flux in S. cerevisiae in anaerobic, sugar-limited, steady-state cultures.
Full text
PDF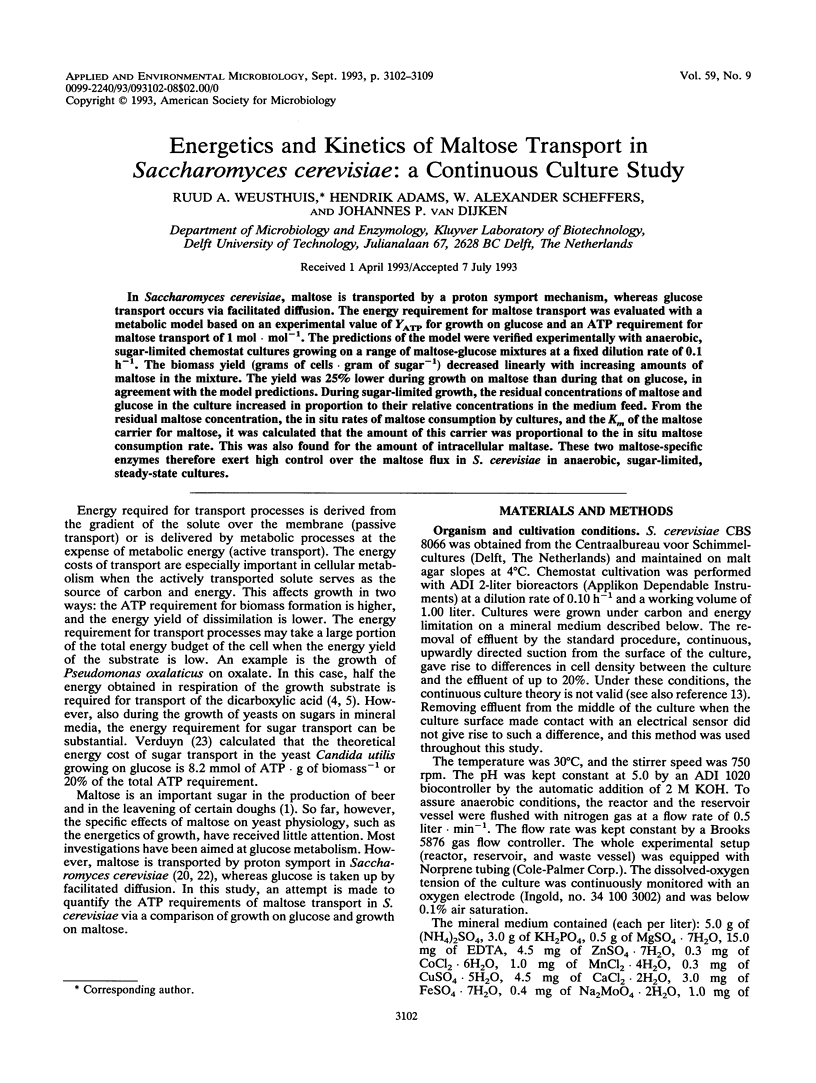
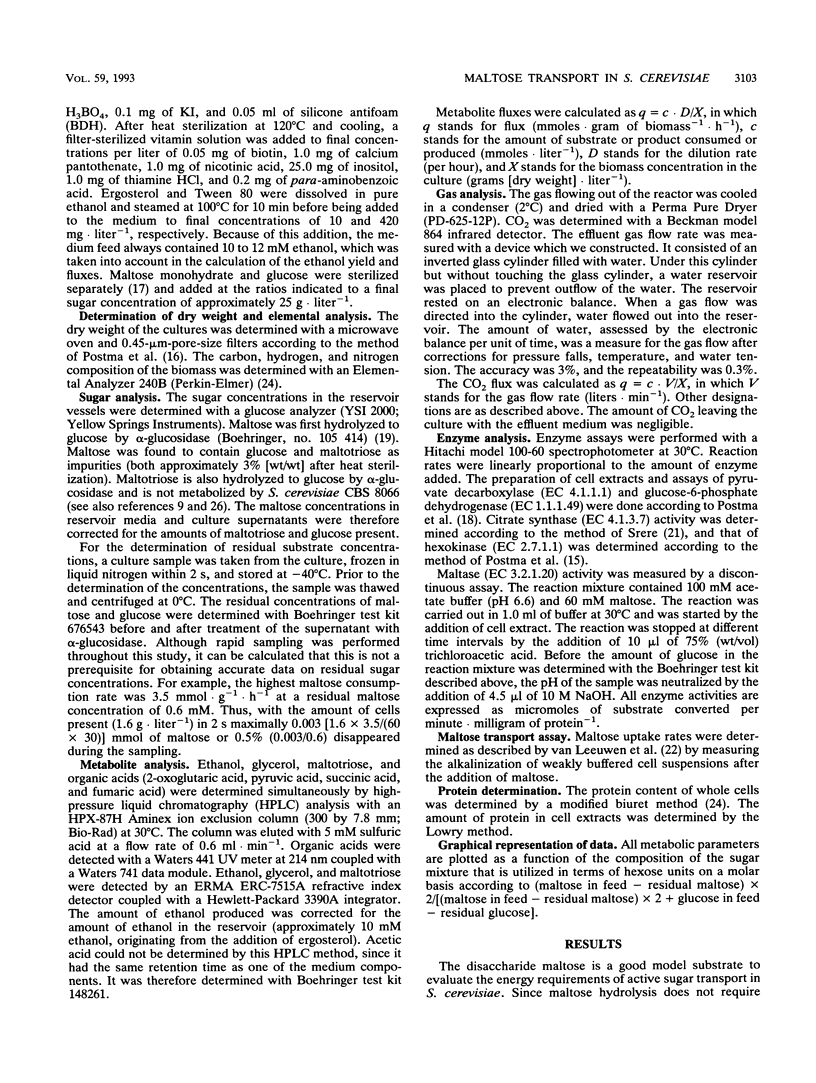
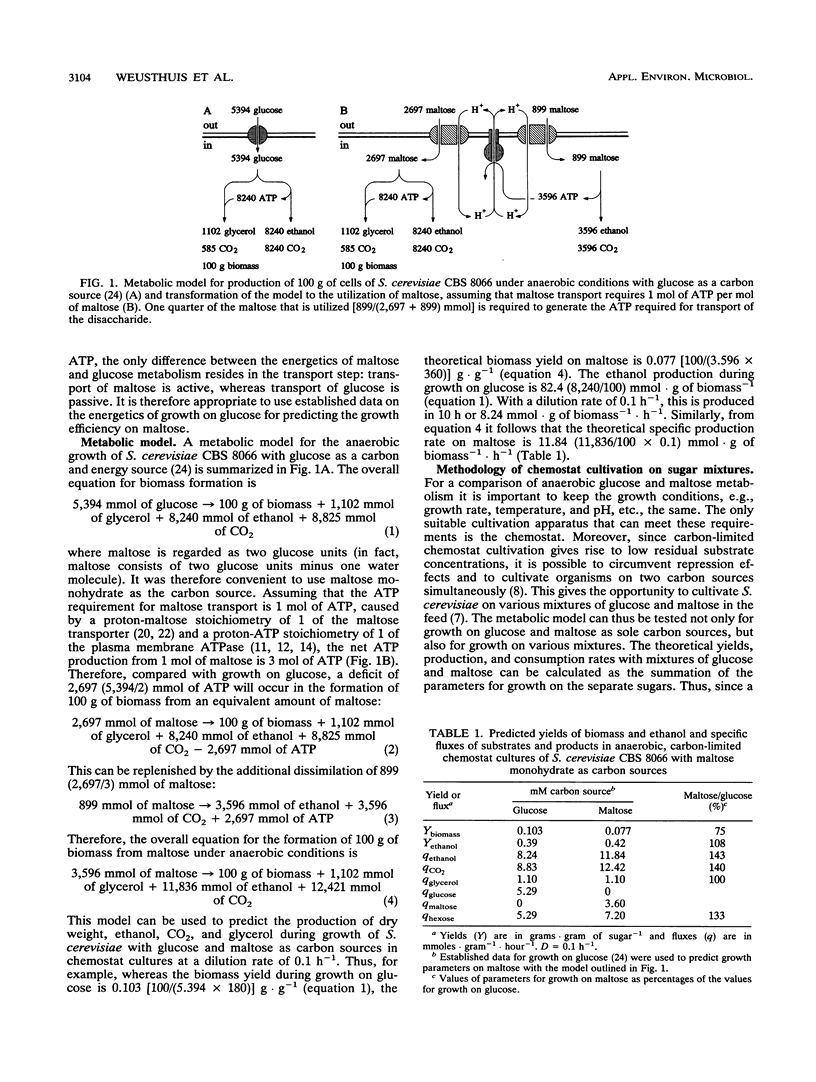
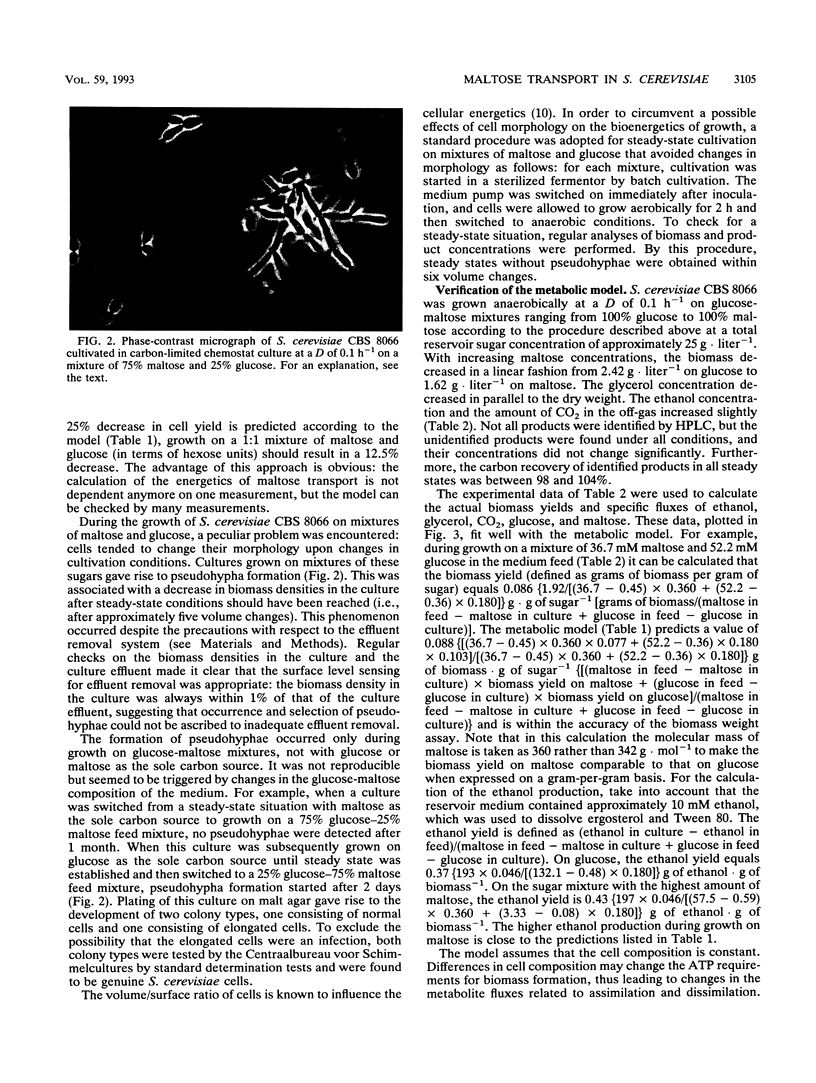
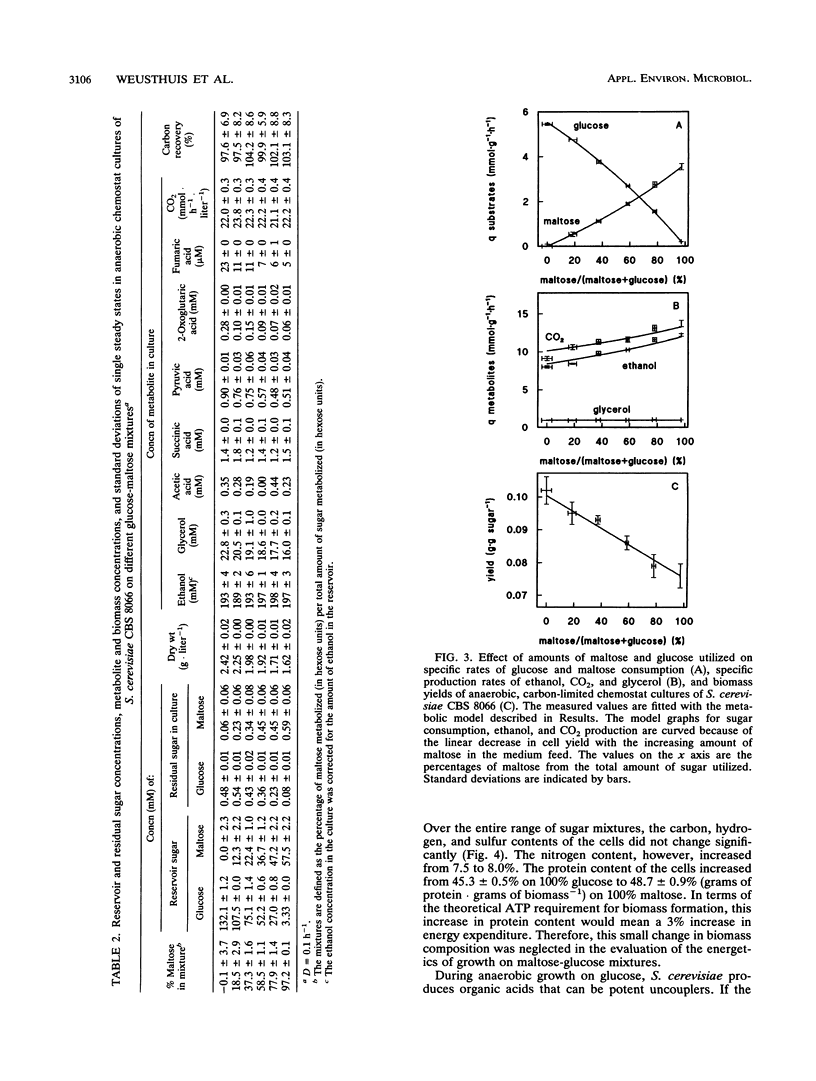
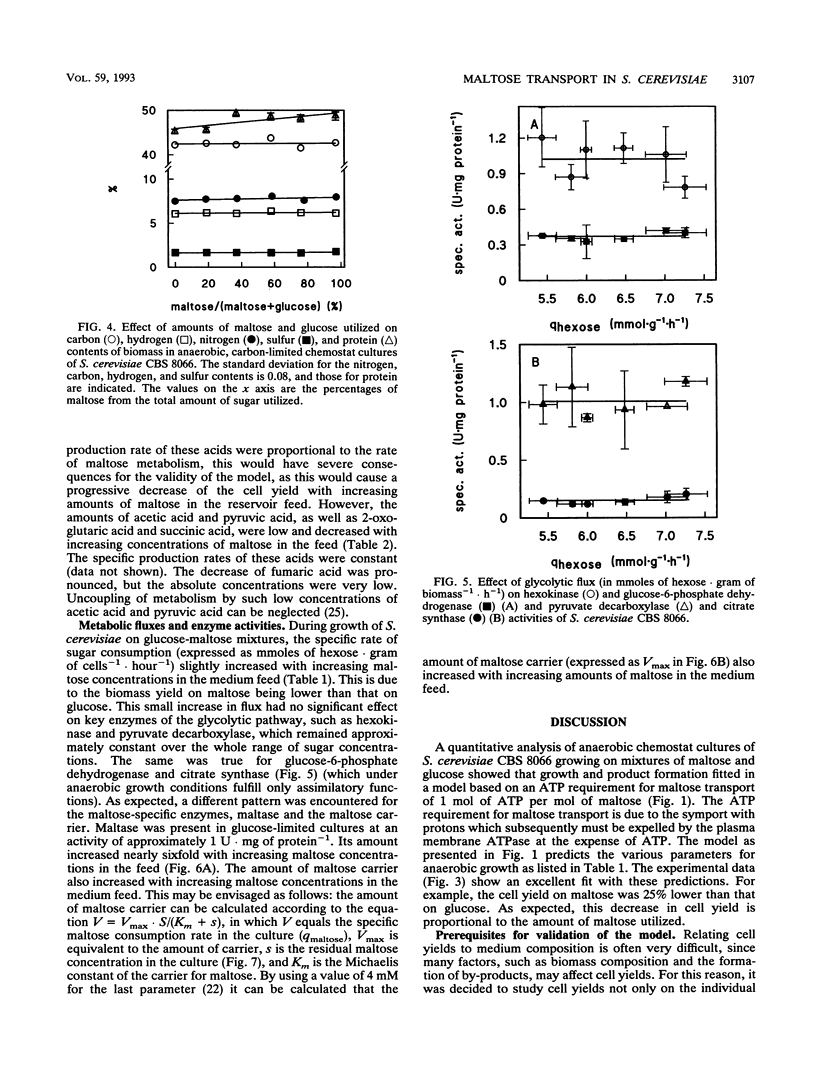
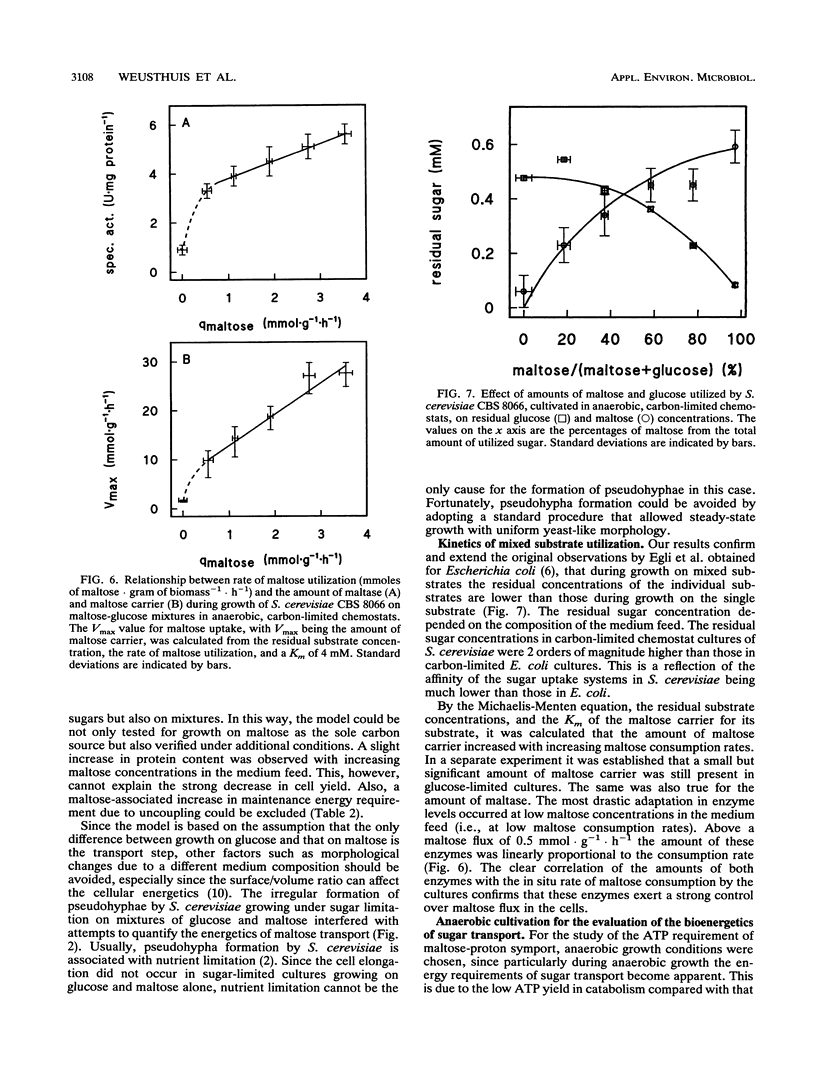
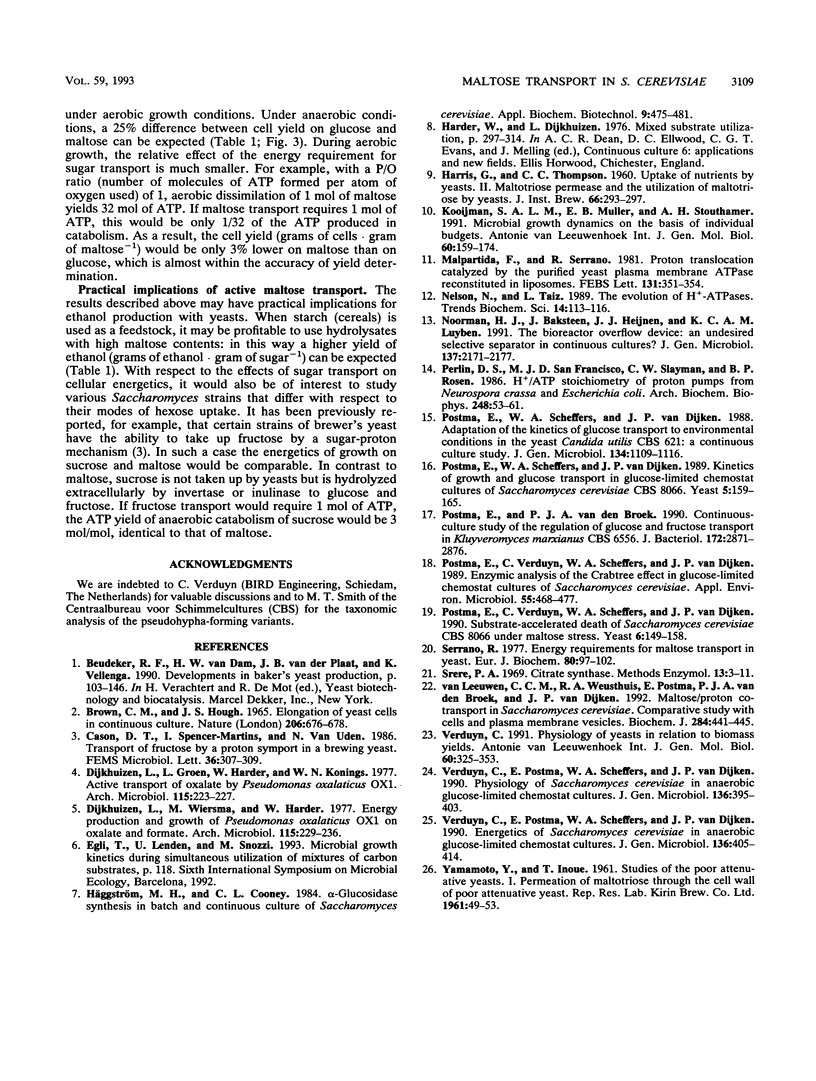
Images in this article
Selected References
These references are in PubMed. This may not be the complete list of references from this article.
- Brown C. M., Hough J. S. Elongation of yeast cells in continuous culture. Nature. 1965 May 15;206(985):676–678. doi: 10.1038/206676a0. [DOI] [PubMed] [Google Scholar]
- Dijkhuizen L., Groen L., Harder W., Konings W. N. Active transport of oxalate by Pseudomonas oxalaticus OX1. Arch Microbiol. 1977 Nov 18;115(2):223–227. doi: 10.1007/BF00406378. [DOI] [PubMed] [Google Scholar]
- Dijkhuizen L., Wiersma M., Harder W. Energy production and growth of Pseudomonas oxalaticus OX1 on oxalate and formate. Arch Microbiol. 1977 Nov 18;115(2):229–236. doi: 10.1007/BF00406379. [DOI] [PubMed] [Google Scholar]
- Kooijman S. A., Muller E. B., Stouthamer A. H. Microbial growth dynamics on the basis of individual budgets. Antonie Van Leeuwenhoek. 1991 Oct-Nov;60(3-4):159–174. doi: 10.1007/BF00430363. [DOI] [PubMed] [Google Scholar]
- Nelson N., Taiz L. The evolution of H+-ATPases. Trends Biochem Sci. 1989 Mar;14(3):113–116. doi: 10.1016/0968-0004(89)90134-5. [DOI] [PubMed] [Google Scholar]
- Perlin D. S., San Francisco M. J., Slayman C. W., Rosen B. P. H+/ATP stoichiometry of proton pumps from Neurospora crassa and Escherichia coli. Arch Biochem Biophys. 1986 Jul;248(1):53–61. doi: 10.1016/0003-9861(86)90400-5. [DOI] [PubMed] [Google Scholar]
- Postma E., Scheffers W. A., van Dijken J. P. Kinetics of growth and glucose transport in glucose-limited chemostat cultures of Saccharomyces cerevisiae CBS 8066. Yeast. 1989 May-Jun;5(3):159–165. doi: 10.1002/yea.320050305. [DOI] [PubMed] [Google Scholar]
- Postma E., Van den Broek P. J. Continuous-culture study of the regulation of glucose and fructose transport in Kluyveromyces marxianus CBS 6556. J Bacteriol. 1990 Jun;172(6):2871–2876. doi: 10.1128/jb.172.6.2871-2876.1990. [DOI] [PMC free article] [PubMed] [Google Scholar]
- Postma E., Verduyn C., Kuiper A., Scheffers W. A., van Dijken J. P. Substrate-accelerated death of Saccharomyces cerevisiae CBS 8066 under maltose stress. Yeast. 1990 Mar-Apr;6(2):149–158. doi: 10.1002/yea.320060209. [DOI] [PubMed] [Google Scholar]
- Postma E., Verduyn C., Scheffers W. A., Van Dijken J. P. Enzymic analysis of the crabtree effect in glucose-limited chemostat cultures of Saccharomyces cerevisiae. Appl Environ Microbiol. 1989 Feb;55(2):468–477. doi: 10.1128/aem.55.2.468-477.1989. [DOI] [PMC free article] [PubMed] [Google Scholar]
- Serrano R. Energy requirements for maltose transport in yeast. Eur J Biochem. 1977 Oct 17;80(1):97–102. doi: 10.1111/j.1432-1033.1977.tb11861.x. [DOI] [PubMed] [Google Scholar]
- Van Leeuwen C. C., Weusthuis R. A., Postma E., Van den Broek P. J., Van Dijken J. P. Maltose/proton co-transport in Saccharomyces cerevisiae. Comparative study with cells and plasma membrane vesicles. Biochem J. 1992 Jun 1;284(Pt 2):441–445. doi: 10.1042/bj2840441. [DOI] [PMC free article] [PubMed] [Google Scholar]
- Verduyn C. Physiology of yeasts in relation to biomass yields. Antonie Van Leeuwenhoek. 1991 Oct-Nov;60(3-4):325–353. doi: 10.1007/BF00430373. [DOI] [PubMed] [Google Scholar]
- Verduyn C., Postma E., Scheffers W. A., van Dijken J. P. Energetics of Saccharomyces cerevisiae in anaerobic glucose-limited chemostat cultures. J Gen Microbiol. 1990 Mar;136(3):405–412. doi: 10.1099/00221287-136-3-405. [DOI] [PubMed] [Google Scholar]
- Verduyn C., Postma E., Scheffers W. A., van Dijken J. P. Physiology of Saccharomyces cerevisiae in anaerobic glucose-limited chemostat cultures. J Gen Microbiol. 1990 Mar;136(3):395–403. doi: 10.1099/00221287-136-3-395. [DOI] [PubMed] [Google Scholar]