Abstract
The mesohaline portion of the Chesapeake Bay is subject to annual summertime hypoxia and anoxia in waters beneath the pycnocline. This dissolved oxygen deficit is directly related to salinity-based stratification of the water column in combination with high levels of autochthonously produced organic matter and a very high abundance of metabolically active bacteria. Throughout the water column in the lower, mesohaline part of the bay, between the Potomac and Rappahannock rivers, near the southern limit of the mainstem anoxia, bacterial abundance often exceeded 10 × 106 cells per ml and bacterial production exceeded 7 × 109 cells per liter per day during summer. Bacterial biomass averaged 34% (range, 16 to 126%) of the phytoplankton biomass in summer. These values are equal to or greater than those found farther north in the bay, where the oxygen deficit is more severe. Seasonal variations in bacterial abundance and production were correlated with phytoplankton biomass (lag time, 7 to 14 days), particulate organic carbon and nitrogen, and particulate biochemical oxygen demand in spring; but during summer, they were significantly correlated only with dissolved biochemical oxygen demand. During summer, dissolved biochemical oxygen demand can account for 50 to 60% of the total biochemical oxygen demand throughout the water column and 80% in the bottom waters. There is a clear spring-summer seasonal shift in the production of organic matter and in the coupling of bacteria and autochthonous organic matter. The measurement of dissolved, microbially labile organic matter concentrations is crucial in understanding the trophic dynamics of the lower mesohaline part of the bay. The absolute levels of organic matter in the water column and the bacterial-organic carbon relationships suggest that a lower bay source of organic matter fuels the upper mesohaline bay oxygen deficits.
Full text
PDF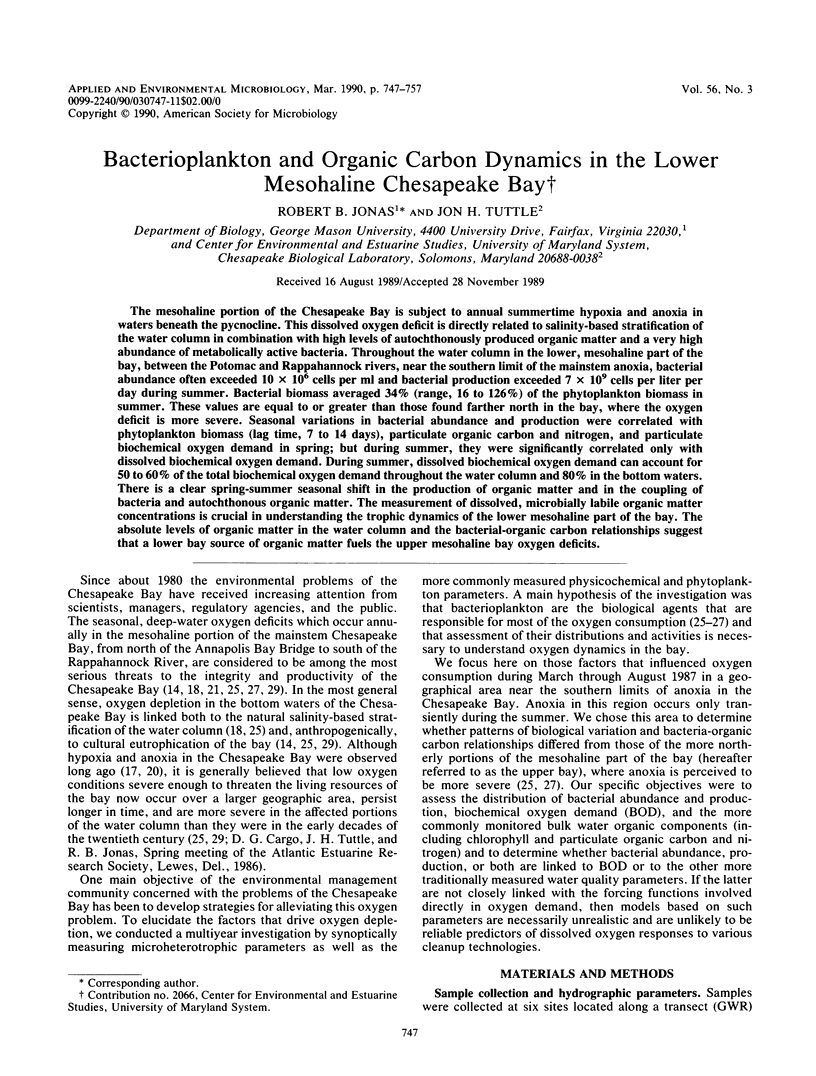
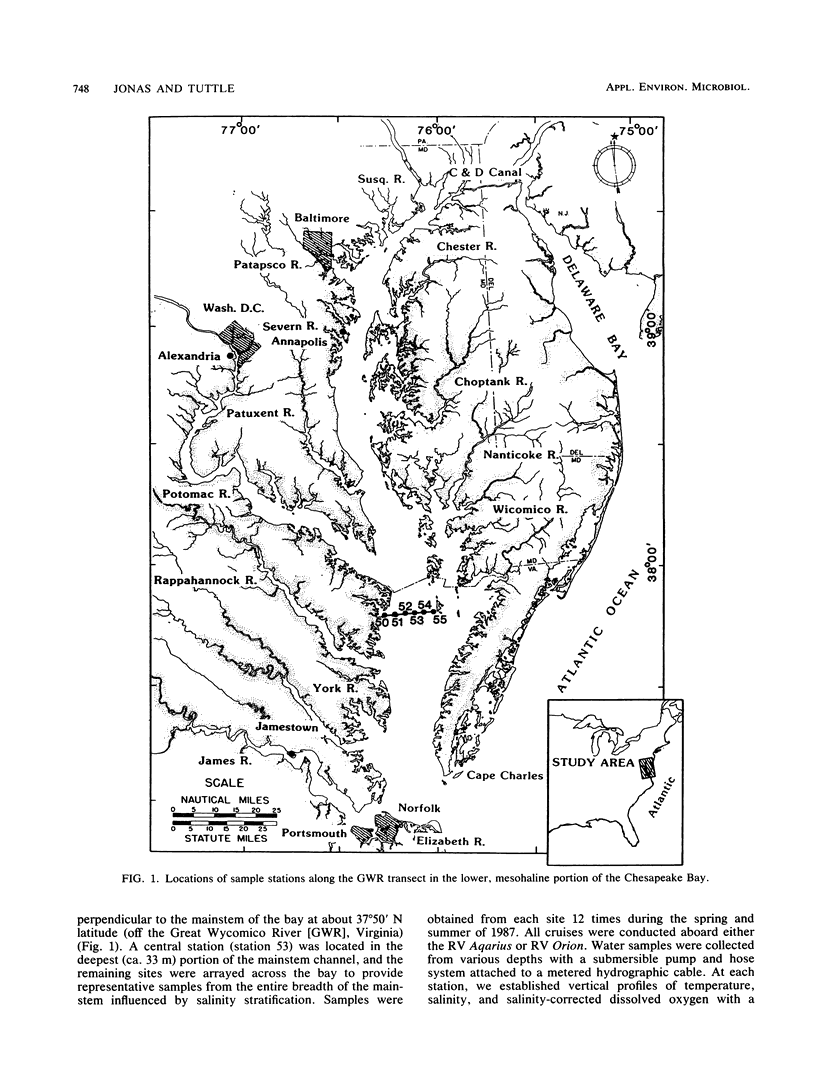
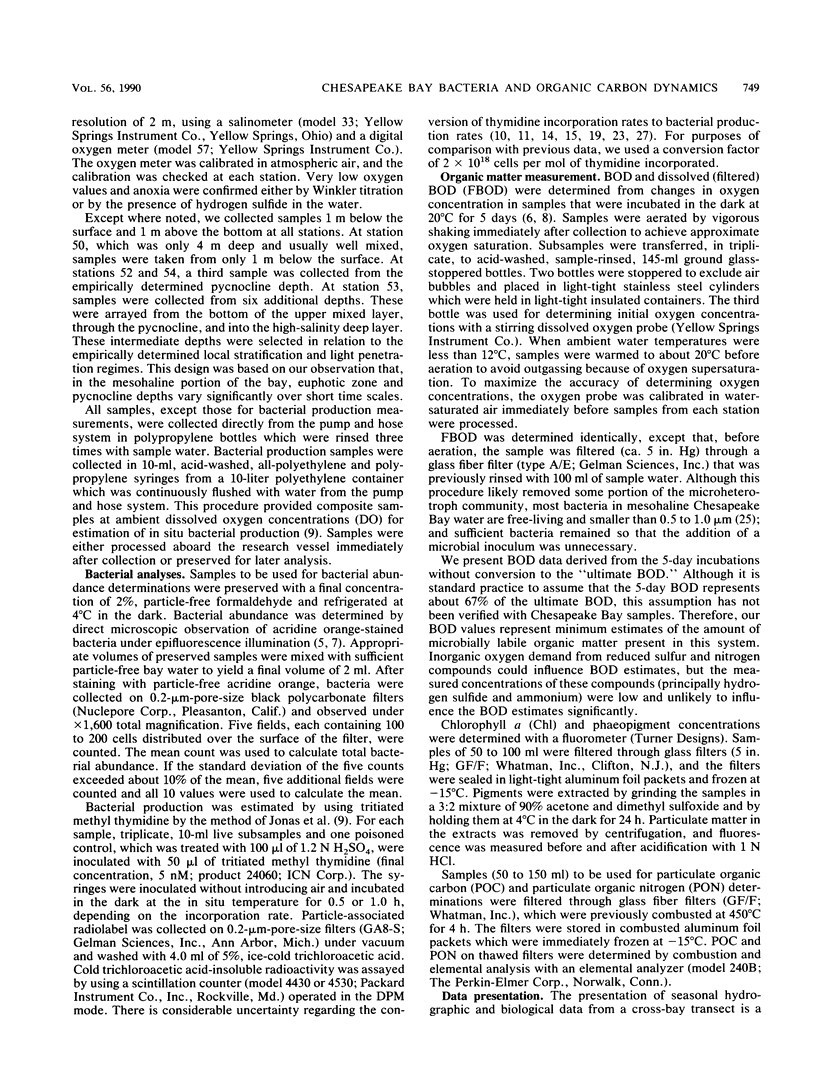
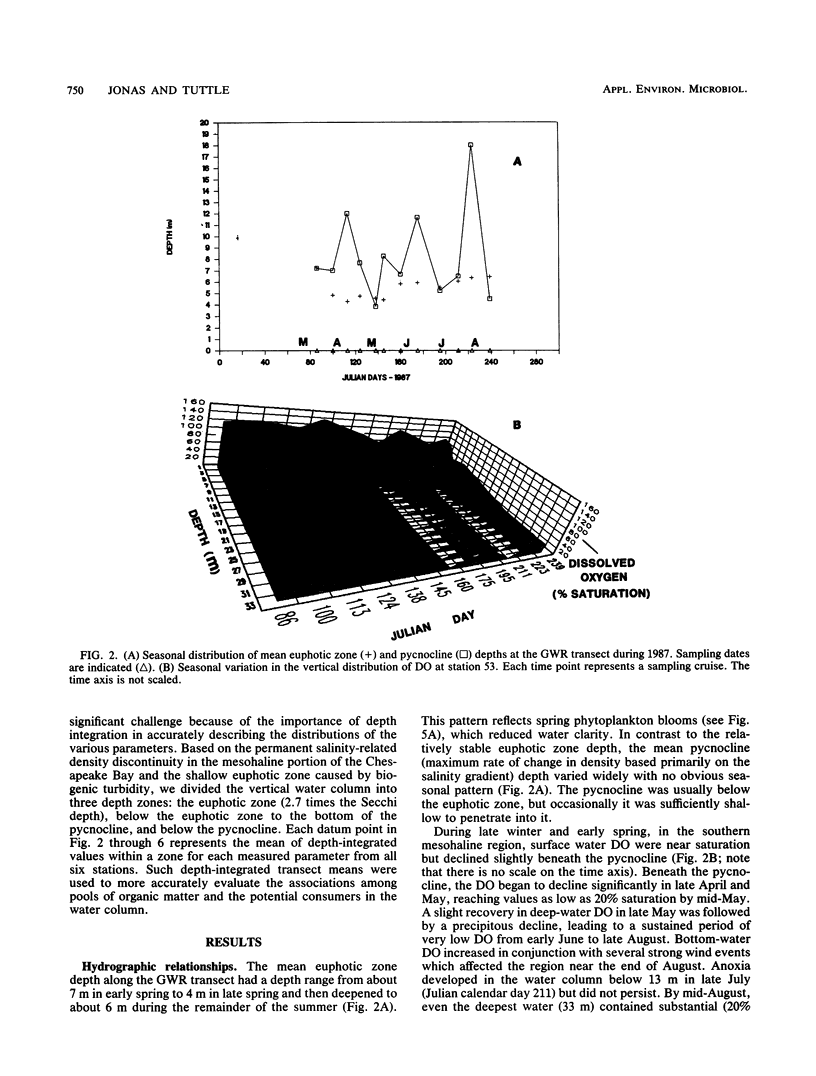
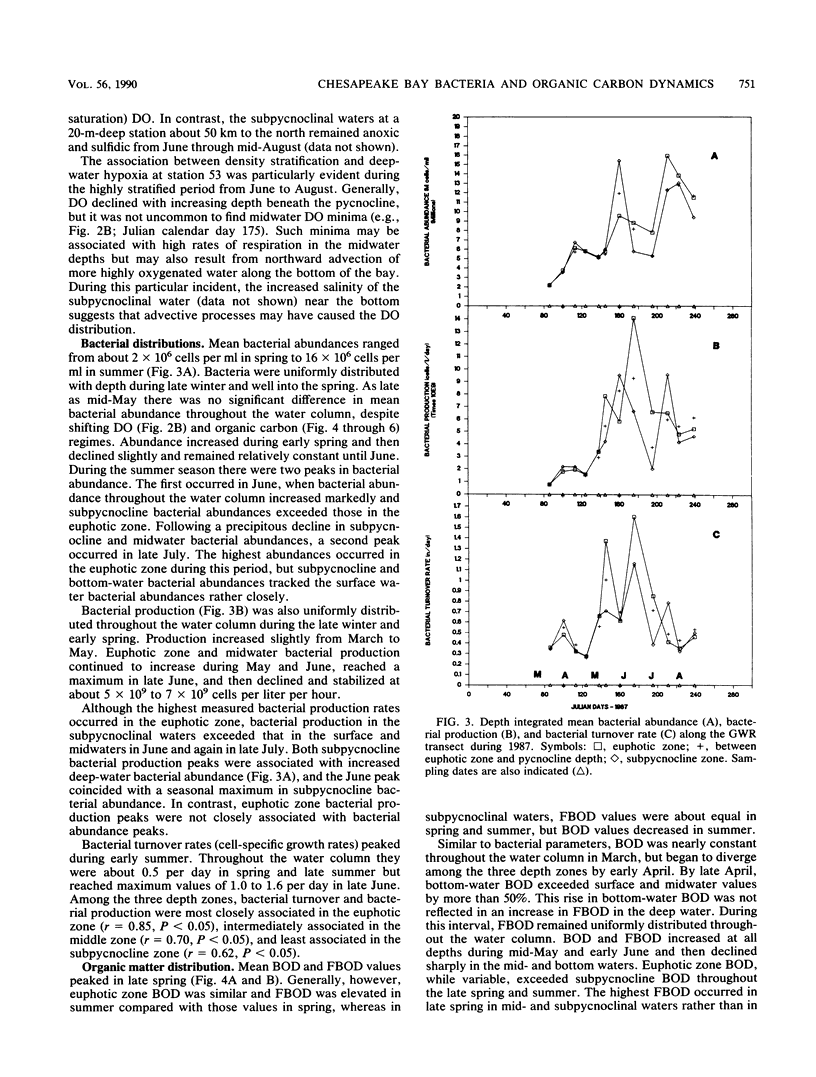
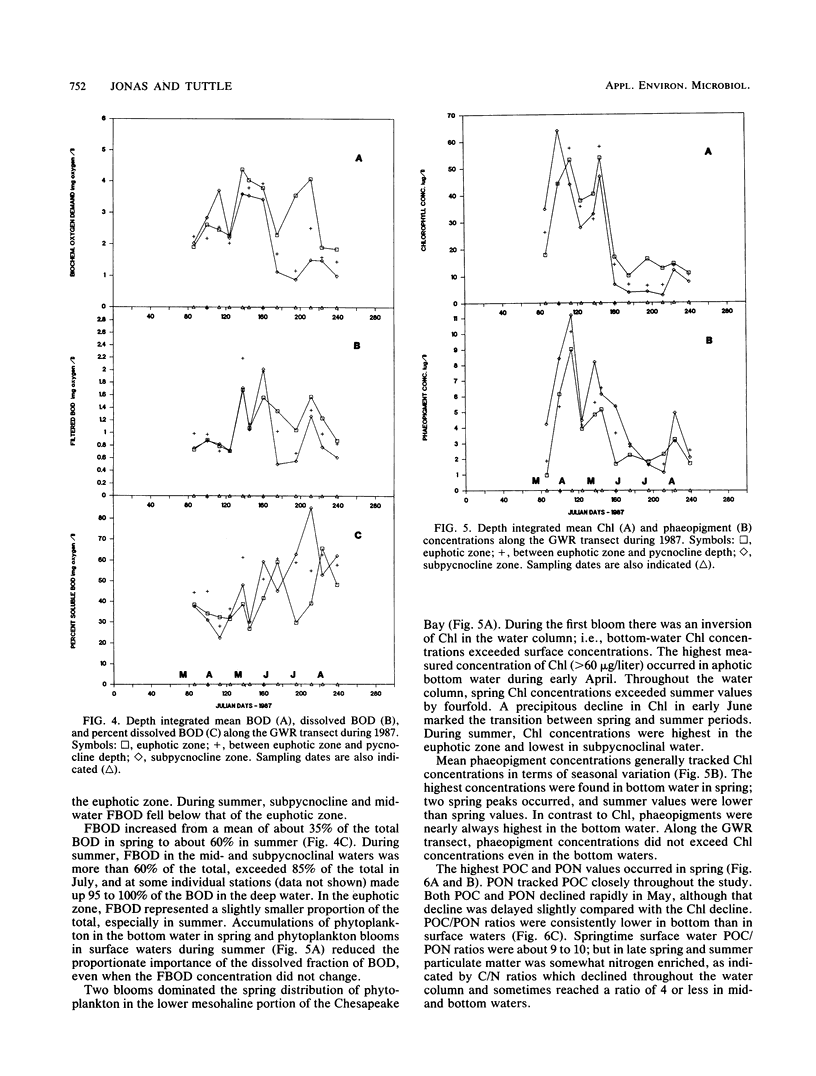
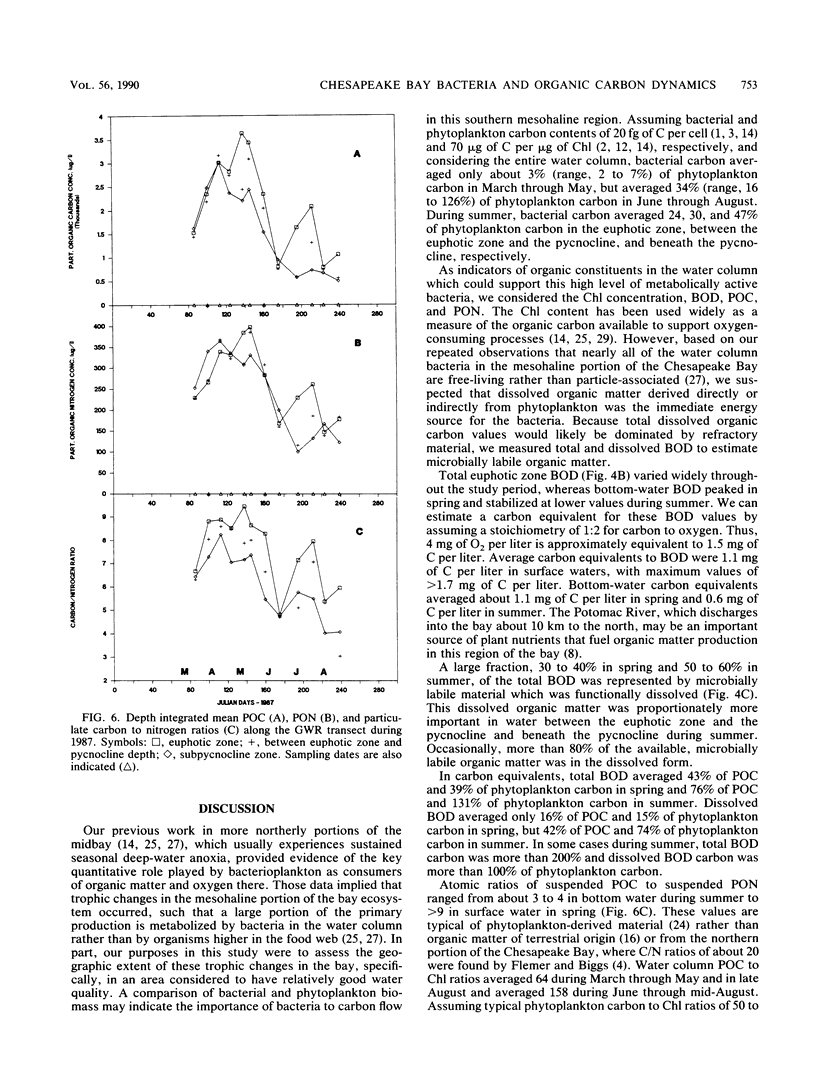
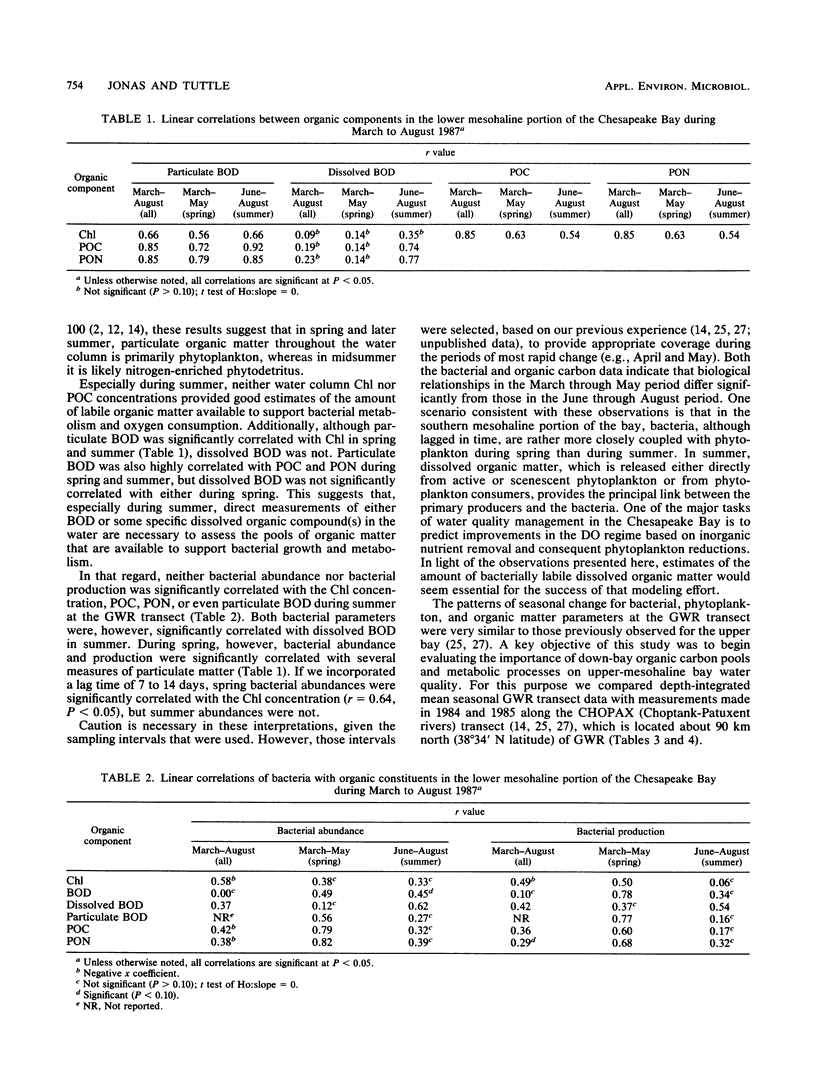
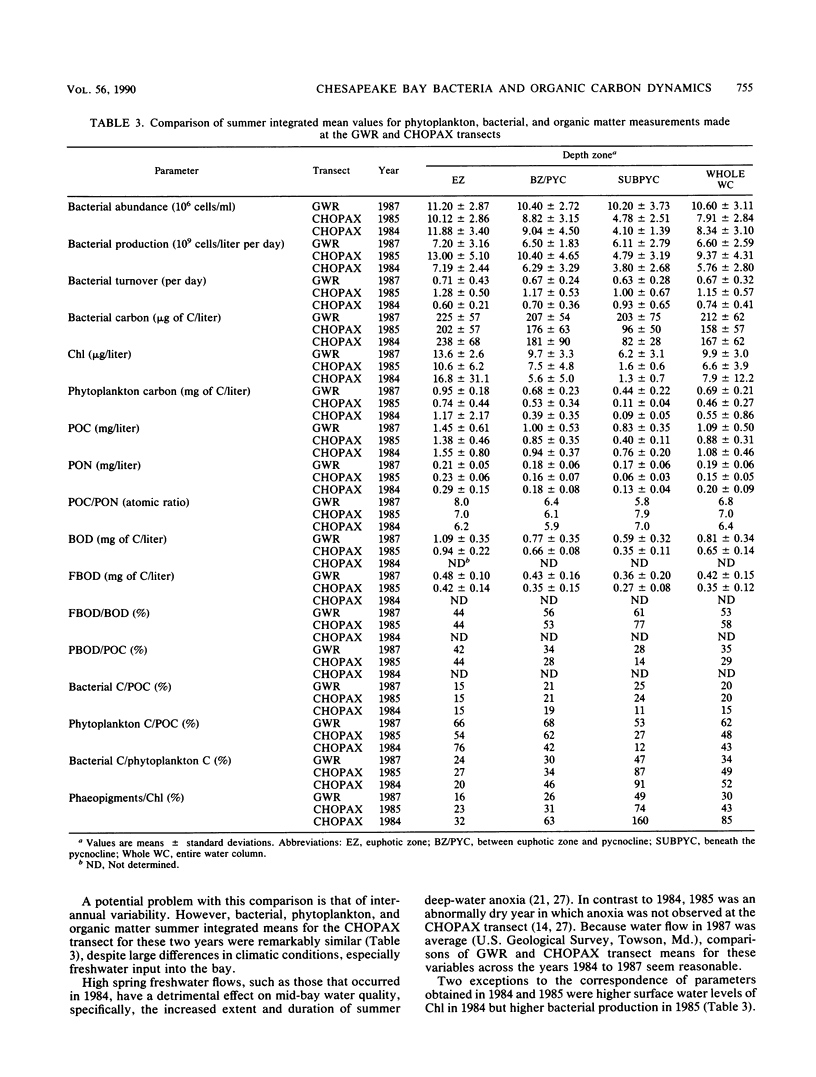
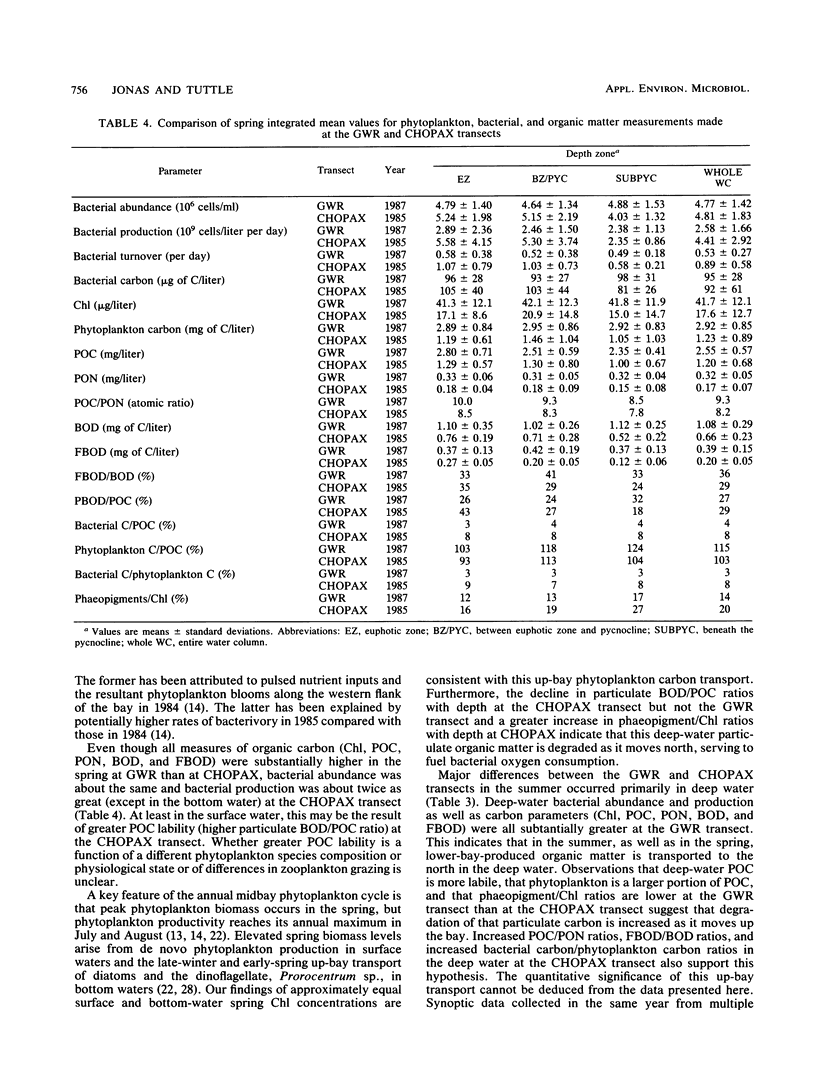
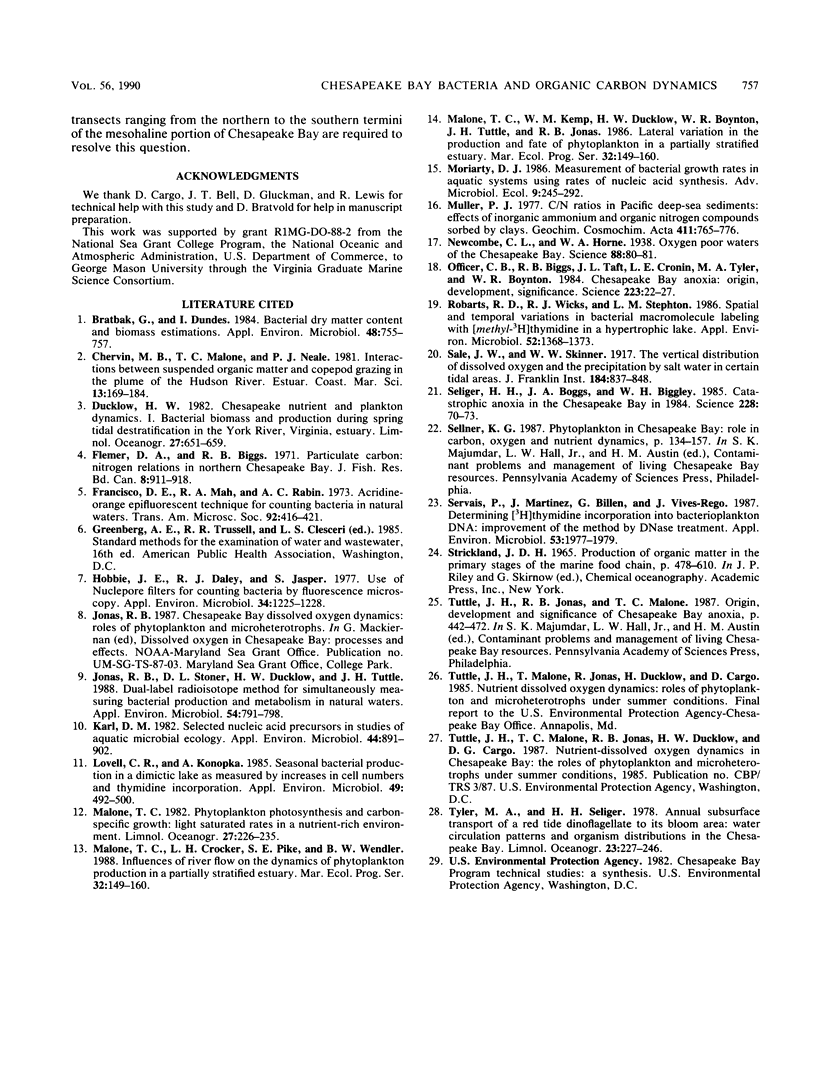
Selected References
These references are in PubMed. This may not be the complete list of references from this article.
- Bratbak G., Dundas I. Bacterial dry matter content and biomass estimations. Appl Environ Microbiol. 1984 Oct;48(4):755–757. doi: 10.1128/aem.48.4.755-757.1984. [DOI] [PMC free article] [PubMed] [Google Scholar]
- Francisco D. E., Mah R. A., Rabin A. C. Acridine orange-epifluorescence technique for counting bacteria in natural waters. Trans Am Microsc Soc. 1973 Jul;92(3):416–421. [PubMed] [Google Scholar]
- Hobbie J. E., Daley R. J., Jasper S. Use of nuclepore filters for counting bacteria by fluorescence microscopy. Appl Environ Microbiol. 1977 May;33(5):1225–1228. doi: 10.1128/aem.33.5.1225-1228.1977. [DOI] [PMC free article] [PubMed] [Google Scholar]
- Jonas Robert B., Tuttle Jon H., Stoner Daphne L., Ducklow Hugh W. Dual-Label Radioisotope Method for Simultaneously Measuring Bacterial Production and Metabolism in Natural Waters. Appl Environ Microbiol. 1988 Mar;54(3):791–798. doi: 10.1128/aem.54.3.791-798.1988. [DOI] [PMC free article] [PubMed] [Google Scholar]
- Karl D. M. Selected nucleic Acid precursors in studies of aquatic microbial ecology. Appl Environ Microbiol. 1982 Oct;44(4):891–902. doi: 10.1128/aem.44.4.891-902.1982. [DOI] [PMC free article] [PubMed] [Google Scholar]
- Lovell C. R., Konopka A. Seasonal bacterial production in a dimictic lake as measured by increases in cell numbers and thymidine incorporation. Appl Environ Microbiol. 1985 Mar;49(3):492–500. doi: 10.1128/aem.49.3.492-500.1985. [DOI] [PMC free article] [PubMed] [Google Scholar]
- Newcombe C. L., Horne W. A. OXYGEN-POOR WATERS OF THE CHESAPEAKE BAY. Science. 1938 Jul 22;88(2273):80–81. doi: 10.1126/science.88.2273.80. [DOI] [PubMed] [Google Scholar]
- Officer C. B., Biggs R. B., Taft J. L., Cronin L. E., Tyler M. A., Boynton W. R. Chesapeake bay anoxia: origin, development, and significance. Science. 1984 Jan 6;223(4631):22–27. doi: 10.1126/science.223.4631.22. [DOI] [PubMed] [Google Scholar]
- Robarts R. D., Wicks R. J., Sephton L. M. Spatial and Temporal Variations in Bacterial Macromolecule Labeling with [methyl-H]Thymidine in a Hypertrophic Lake. Appl Environ Microbiol. 1986 Dec;52(6):1368–1373. doi: 10.1128/aem.52.6.1368-1373.1986. [DOI] [PMC free article] [PubMed] [Google Scholar]
- Seliger H. H., Boggs J. A., Biggley W. H. Catastrophic anoxia in the chesapeake bay in 1984. Science. 1985 Apr 5;228(4695):70–73. doi: 10.1126/science.228.4695.70. [DOI] [PubMed] [Google Scholar]
- Servais P., Martinez J., Billen G., Vives-Rego J. Determining [H]Thymidine Incorporation into Bacterioplankton DNA: Improvement of the Method by DNase Treatment. Appl Environ Microbiol. 1987 Aug;53(8):1977–1979. doi: 10.1128/aem.53.8.1977-1979.1987. [DOI] [PMC free article] [PubMed] [Google Scholar]