Abstract
The ability of Alteromonas putrefaciens to obtain energy for growth by coupling the oxidation of various electron donors to dissimilatory Fe(III) or Mn(IV) reduction was investigated. A. putrefaciens grew with hydrogen, formate, lactate, or pyruvate as the sole electron donor and Fe(III) as the sole electron acceptor. Lactate and pyruvate were oxidized to acetate, which was not metabolized further. With Fe(III) as the electron acceptor, A. putrefaciens had a high affinity for hydrogen and formate and metabolized hydrogen at partial pressures that were 25-fold lower than those of hydrogen that can be metabolized by pure cultures of sulfate reducers or methanogens. The electron donors for Fe(III) reduction also supported Mn(IV) reduction. The electron donors for Fe(III) and Mn(IV) reduction and the inability of A. putrefaciens to completely oxidize multicarbon substrates to carbon dioxide distinguish A. putrefaciens from GS-15, the only other organism that is known to obtain energy for growth by coupling the oxidation of organic compounds to the reduction of Fe(III) or Mn(IV). The ability of A. putrefaciens to reduce large quantities of Fe(III) and to grow in a defined medium distinguishes it from a Pseudomonas sp., which is the only other known hydrogen-oxidizing, Fe(III)-reducing microorganism. Furthermore, A. putrefaciens is the first organism that is known to grow with hydrogen as the electron donor and Mn(IV) as the electron acceptor and is the first organism that is known to couple the oxidation of formate to the reduction of Fe(III) or Mn(IV). Thus, A. putrefaciens provides a much needed microbial model for key reactions in the oxidation of sediment organic matter coupled to Fe(III) and Mn(IV) reduction.
Full text
PDF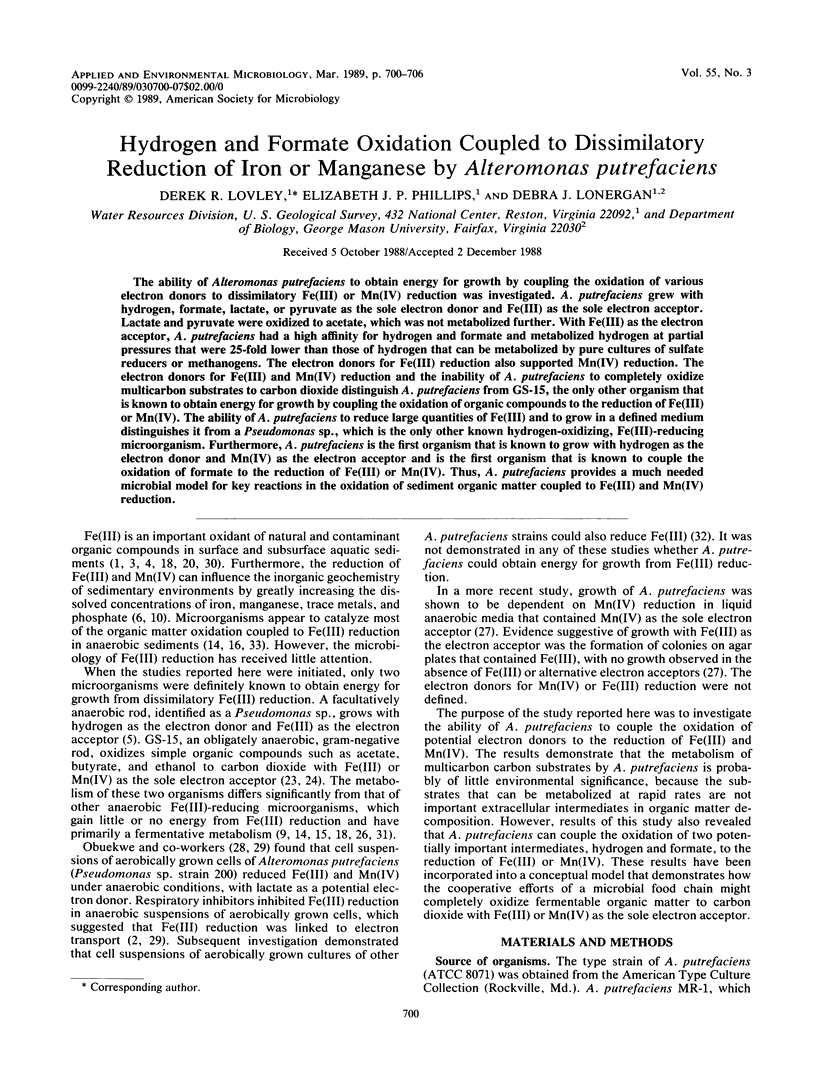
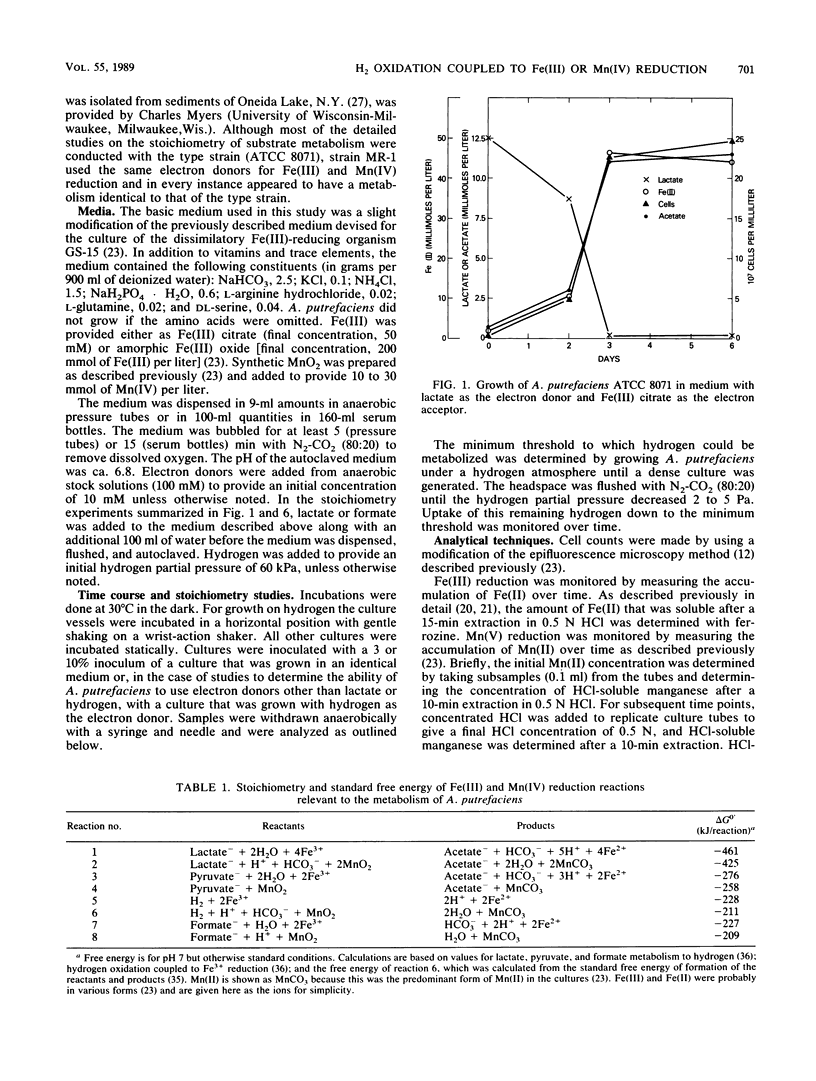
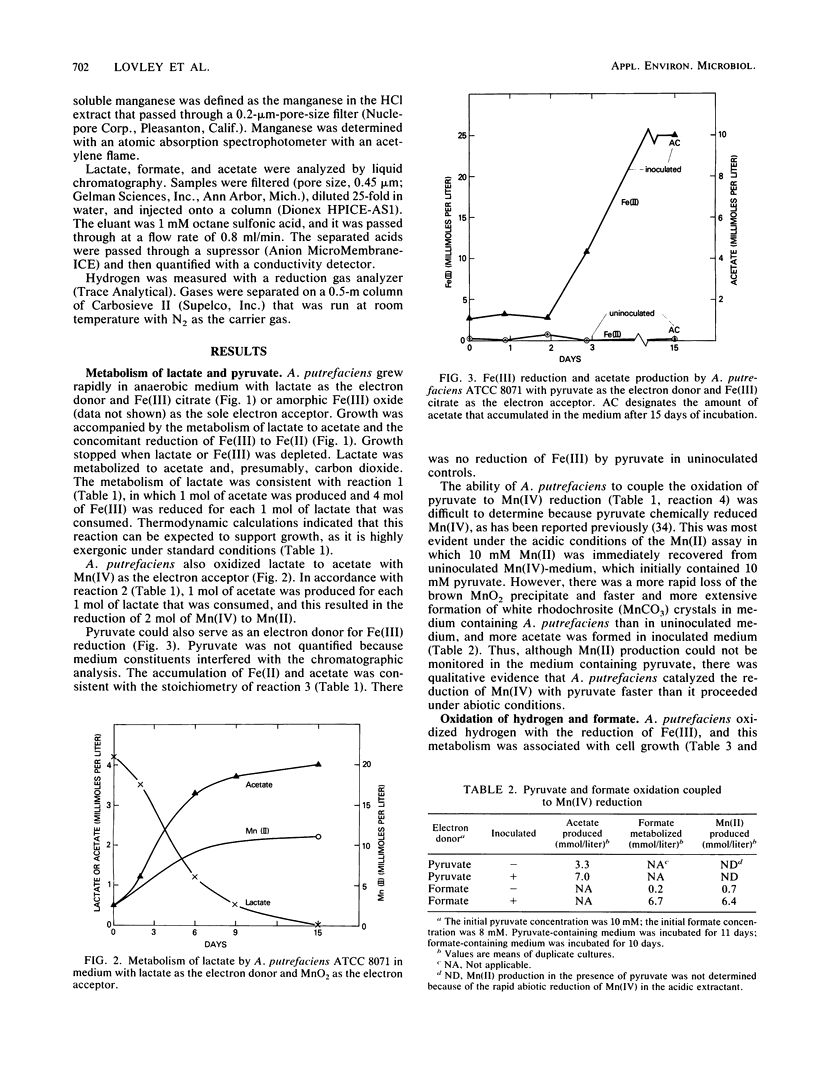
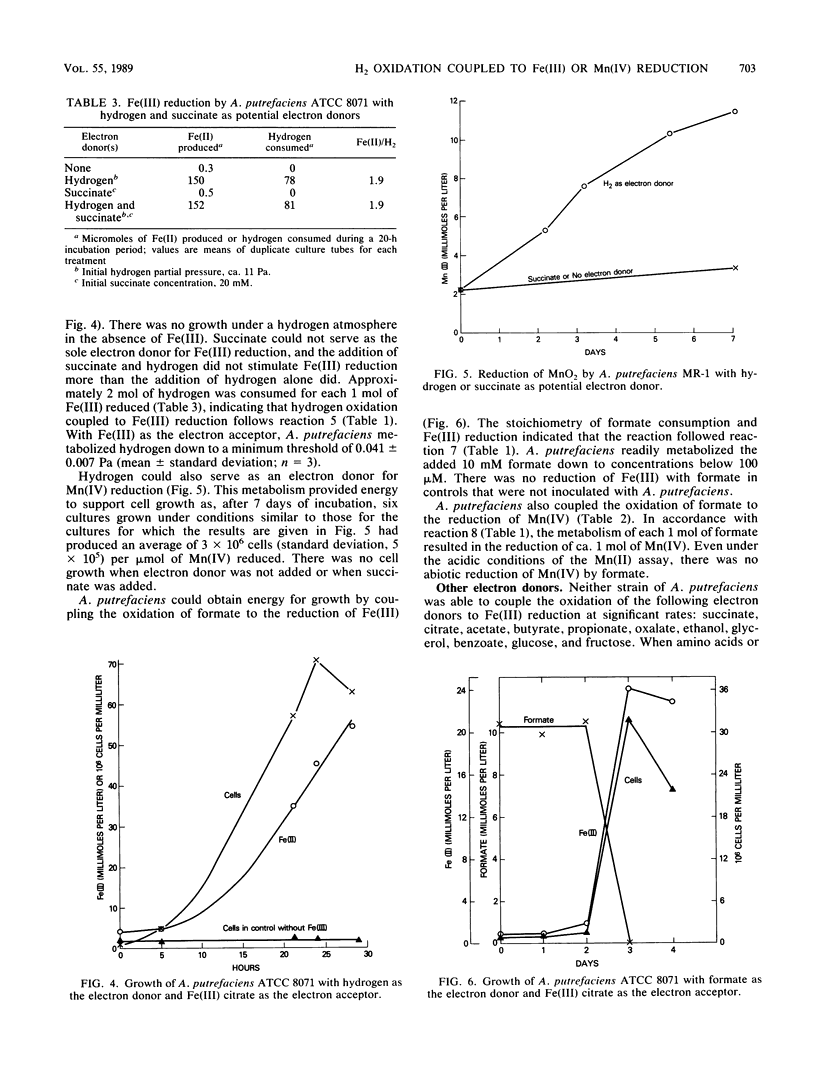
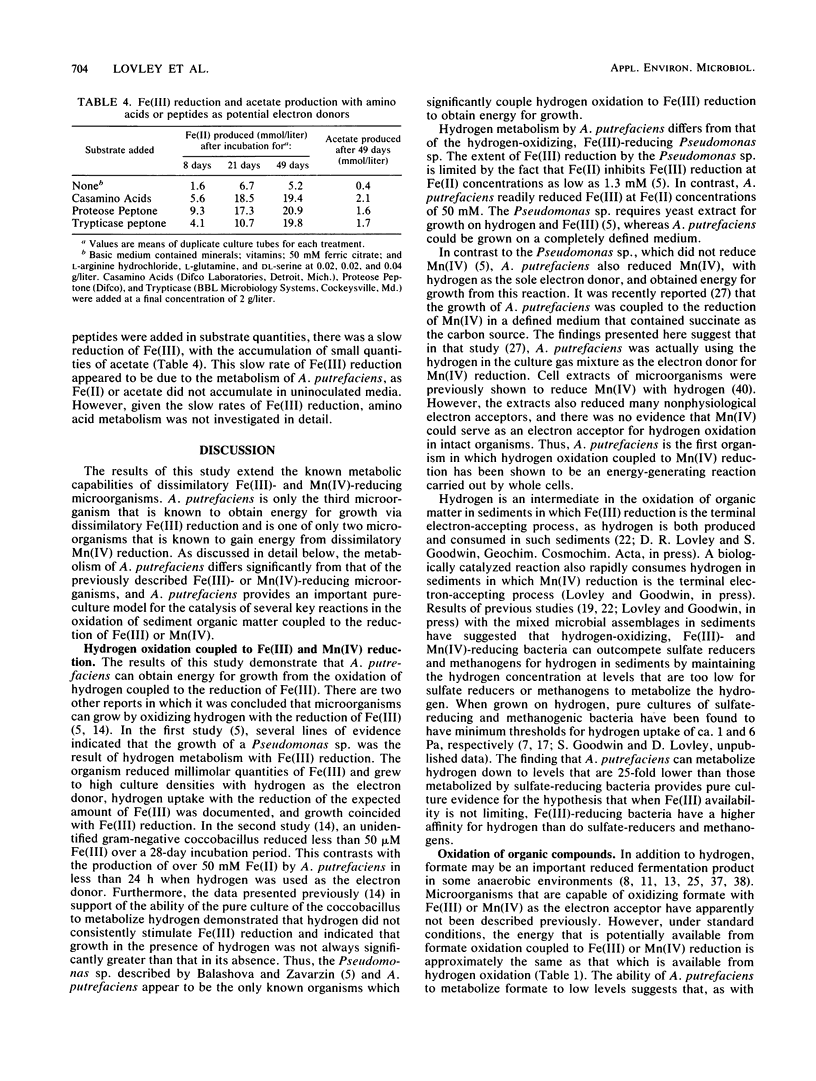
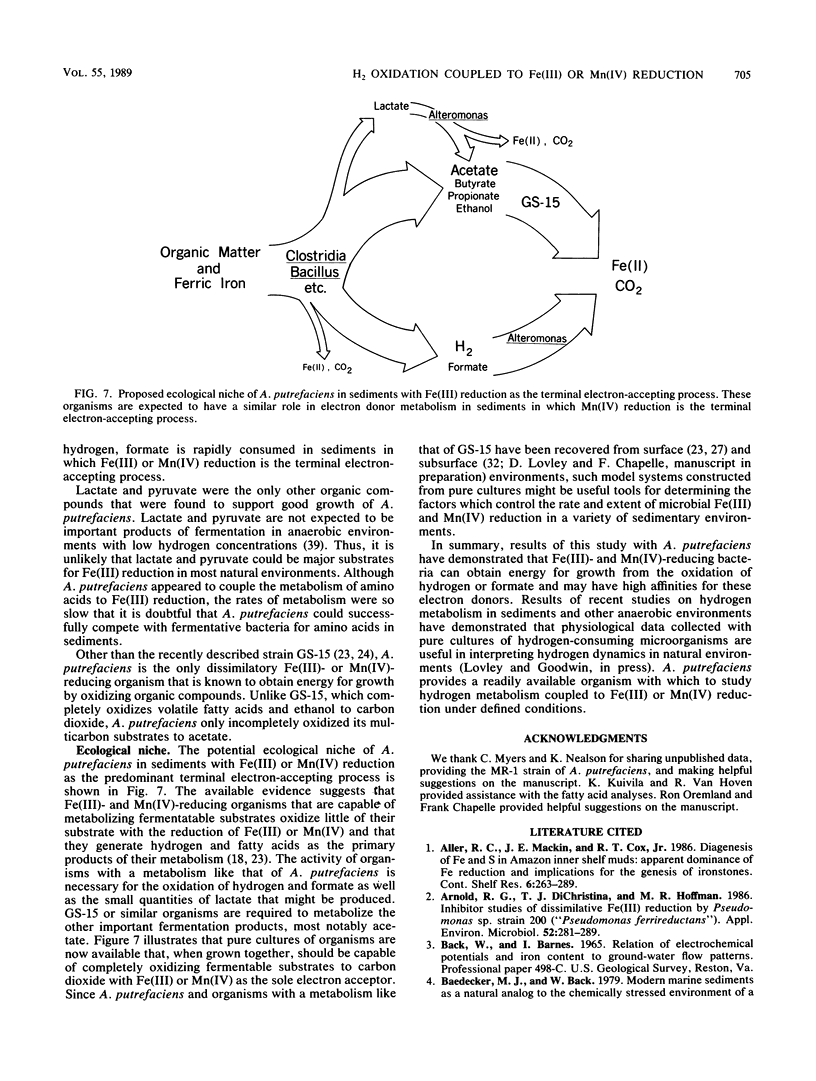
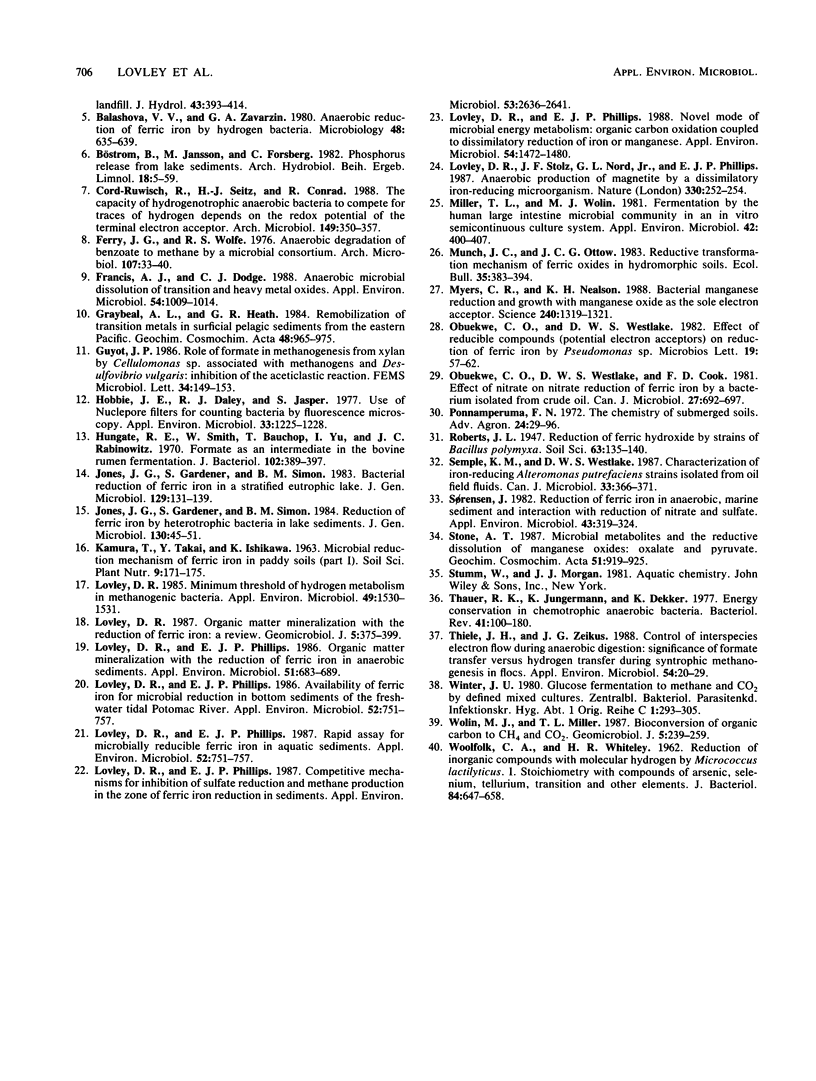
Selected References
These references are in PubMed. This may not be the complete list of references from this article.
- Arnold R. G., DiChristina T. J., Hoffmann M. R. Inhibitor studies of dissimilative Fe(III) reduction by Pseudomonas sp. strain 200 ("Pseudomonas ferrireductans") Appl Environ Microbiol. 1986 Aug;52(2):281–289. doi: 10.1128/aem.52.2.281-289.1986. [DOI] [PMC free article] [PubMed] [Google Scholar]
- Ferry J. G., Wolfe R. S. Anaerobic degradation of benzoate to methane by a microbial consortium. Arch Microbiol. 1976 Feb;107(1):33–40. doi: 10.1007/BF00427864. [DOI] [PubMed] [Google Scholar]
- Francis A. J., Dodge C. J. Anaerobic microbial dissolution of transition and heavy metal oxides. Appl Environ Microbiol. 1988 Apr;54(4):1009–1014. doi: 10.1128/aem.54.4.1009-1014.1988. [DOI] [PMC free article] [PubMed] [Google Scholar]
- Hobbie J. E., Daley R. J., Jasper S. Use of nuclepore filters for counting bacteria by fluorescence microscopy. Appl Environ Microbiol. 1977 May;33(5):1225–1228. doi: 10.1128/aem.33.5.1225-1228.1977. [DOI] [PMC free article] [PubMed] [Google Scholar]
- Hungate R. E., Smith W., Bauchop T., Yu I., Rabinowitz J. C. Formate as an intermediate in the bovine rumen fermentation. J Bacteriol. 1970 May;102(2):389–397. doi: 10.1128/jb.102.2.389-397.1970. [DOI] [PMC free article] [PubMed] [Google Scholar]
- Lovley D. R. Minimum threshold for hydrogen metabolism in methanogenic bacteria. Appl Environ Microbiol. 1985 Jun;49(6):1530–1531. doi: 10.1128/aem.49.6.1530-1531.1985. [DOI] [PMC free article] [PubMed] [Google Scholar]
- Lovley D. R., Phillips E. J. Availability of ferric iron for microbial reduction in bottom sediments of the freshwater tidal potomac river. Appl Environ Microbiol. 1986 Oct;52(4):751–757. doi: 10.1128/aem.52.4.751-757.1986. [DOI] [PMC free article] [PubMed] [Google Scholar]
- Lovley D. R., Phillips E. J. Availability of ferric iron for microbial reduction in bottom sediments of the freshwater tidal potomac river. Appl Environ Microbiol. 1986 Oct;52(4):751–757. doi: 10.1128/aem.52.4.751-757.1986. [DOI] [PMC free article] [PubMed] [Google Scholar]
- Lovley D. R., Phillips E. J. Competitive mechanisms for inhibition of sulfate reduction and methane production in the zone of ferric iron reduction in sediments. Appl Environ Microbiol. 1987 Nov;53(11):2636–2641. doi: 10.1128/aem.53.11.2636-2641.1987. [DOI] [PMC free article] [PubMed] [Google Scholar]
- Lovley D. R., Phillips E. J. Novel mode of microbial energy metabolism: organic carbon oxidation coupled to dissimilatory reduction of iron or manganese. Appl Environ Microbiol. 1988 Jun;54(6):1472–1480. doi: 10.1128/aem.54.6.1472-1480.1988. [DOI] [PMC free article] [PubMed] [Google Scholar]
- Lovley D. R., Phillips E. J. Organic matter mineralization with reduction of ferric iron in anaerobic sediments. Appl Environ Microbiol. 1986 Apr;51(4):683–689. doi: 10.1128/aem.51.4.683-689.1986. [DOI] [PMC free article] [PubMed] [Google Scholar]
- Miller T. L., Wolin M. J. Fermentation by the human large intestine microbial community in an in vitro semicontinuous culture system. Appl Environ Microbiol. 1981 Sep;42(3):400–407. doi: 10.1128/aem.42.3.400-407.1981. [DOI] [PMC free article] [PubMed] [Google Scholar]
- Myers C. R., Nealson K. H. Bacterial manganese reduction and growth with manganese oxide as the sole electron acceptor. Science. 1988 Jun 3;240(4857):1319–1321. doi: 10.1126/science.240.4857.1319. [DOI] [PubMed] [Google Scholar]
- Obuekwe C. O., Westlake D. W., Cook F. D. Effect of nitrate on reduction of ferric iron by a bacterium isolated from crude oil. Can J Microbiol. 1981 Jul;27(7):692–697. doi: 10.1139/m81-107. [DOI] [PubMed] [Google Scholar]
- Sørensen J. Reduction of ferric iron in anaerobic, marine sediment and interaction with reduction of nitrate and sulfate. Appl Environ Microbiol. 1982 Feb;43(2):319–324. doi: 10.1128/aem.43.2.319-324.1982. [DOI] [PMC free article] [PubMed] [Google Scholar]
- Thauer R. K., Jungermann K., Decker K. Energy conservation in chemotrophic anaerobic bacteria. Bacteriol Rev. 1977 Mar;41(1):100–180. doi: 10.1128/br.41.1.100-180.1977. [DOI] [PMC free article] [PubMed] [Google Scholar]
- Thiele Jurgen H., Zeikus J. Gregory. Control of Interspecies Electron Flow during Anaerobic Digestion: Significance of Formate Transfer versus Hydrogen Transfer during Syntrophic Methanogenesis in Flocs. Appl Environ Microbiol. 1988 Jan;54(1):20–29. doi: 10.1128/aem.54.1.20-29.1988. [DOI] [PMC free article] [PubMed] [Google Scholar]
- WOOLFOLK C. A., WHITELEY H. R. Reduction of inorganic compounds with molecular hydrogen by Micrococcus lactilyticus. I. Stoichiometry with compounds of arsenic, selenium, tellurium, transition and other elements. J Bacteriol. 1962 Oct;84:647–658. doi: 10.1128/jb.84.4.647-658.1962. [DOI] [PMC free article] [PubMed] [Google Scholar]