Abstract
The role of mer(Tn21) in the adaptation of aquatic microbial communities to Hg2+ was investigated. Elemental mercury was the sole product of Hg2+ volatilization by freshwater and saline water microbial communities. Bacterial activity was responsible for biotransformation because most microeucaryotes did not survive the exposure conditions, and removal of larger microbes (greater than 1 micromole) from adapted communities did not significantly (P greater than 0.01) reduce Hg2+ volatilization rates. DNA sequences homologous to mer(Tn21) were found in 50% of Hg2+-resistant bacterial strains representing two freshwater communities, but in only 12% of strains representing two saline communities (the difference was highly significant; P less than 0.001). Thus, mer(Tn21) played a significant role in Hg2+ resistance among strains isolated from fresh waters, in which microbial activity had a limited role in Hg2+ volatilization. In saline water environments in which microbially mediated volatilization was the major mechanism of Hg2+ loss, other bacterial genes coded for this biotransformation.
Full text
PDF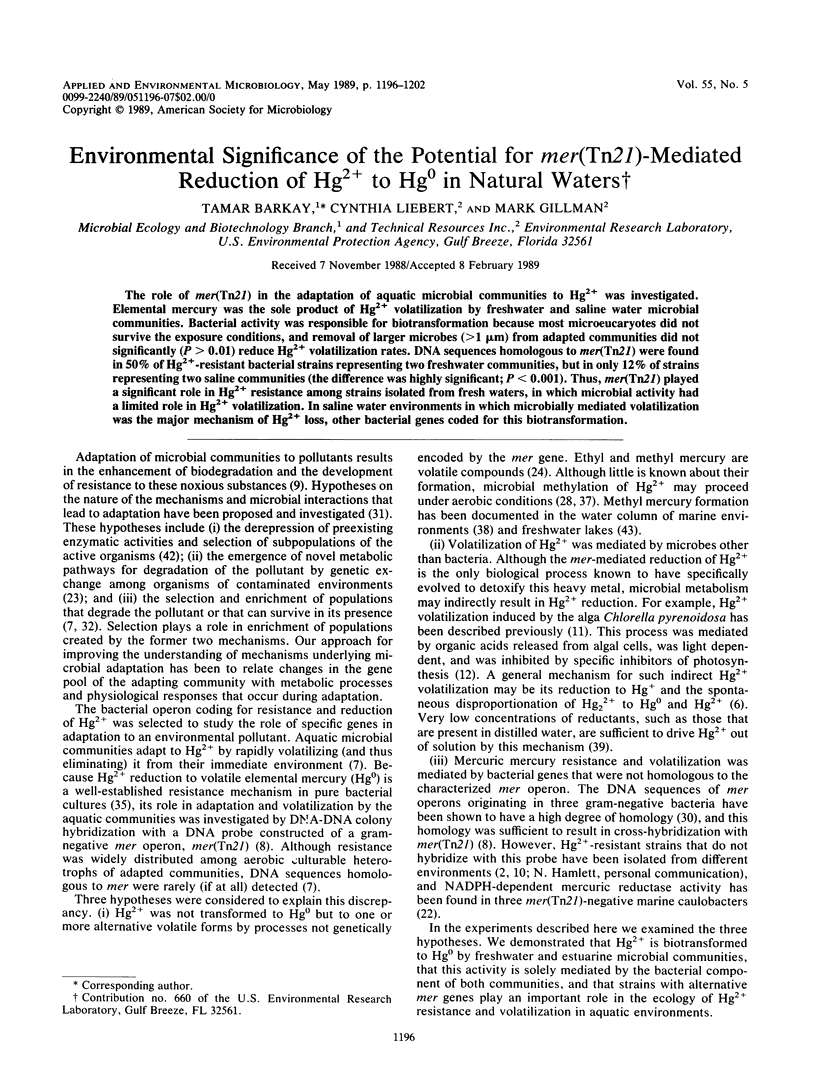
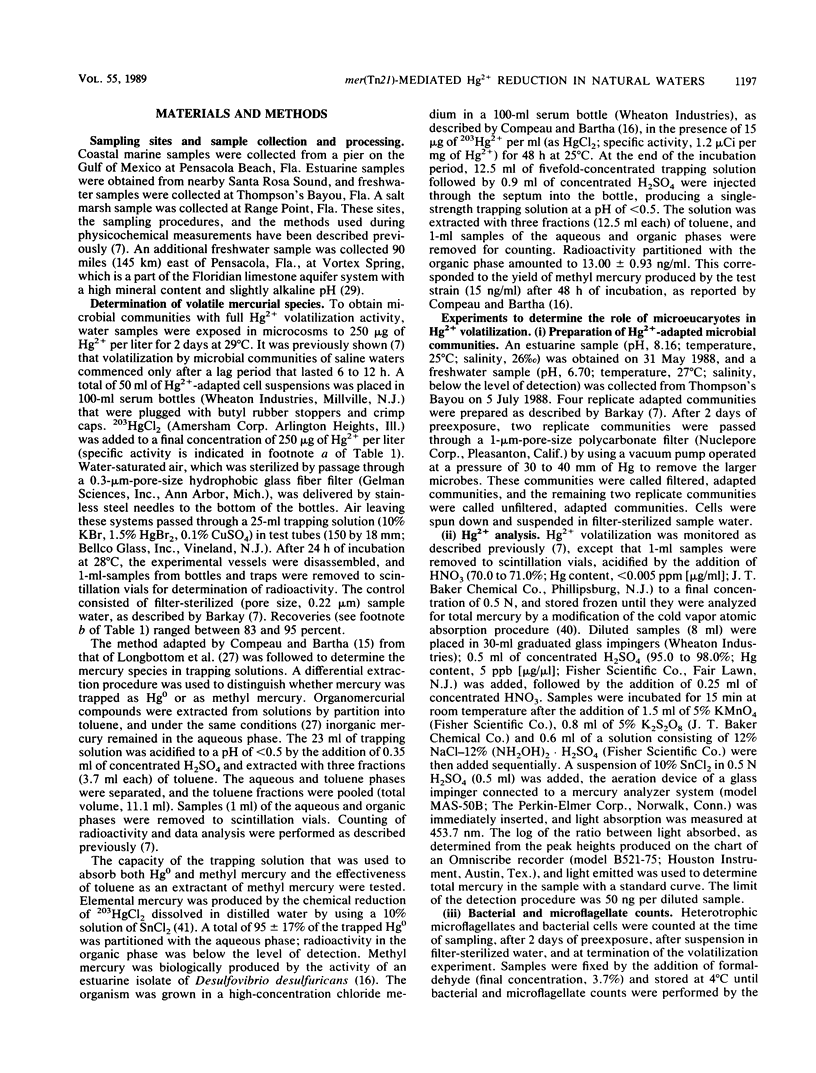
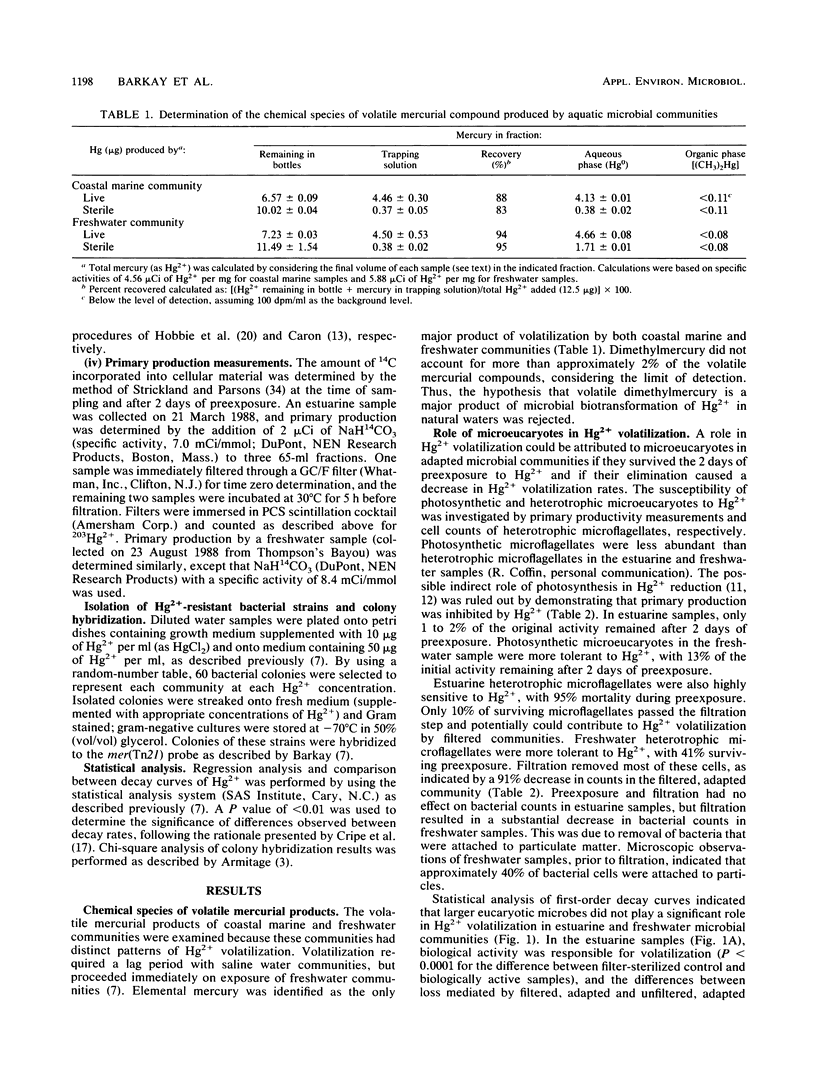
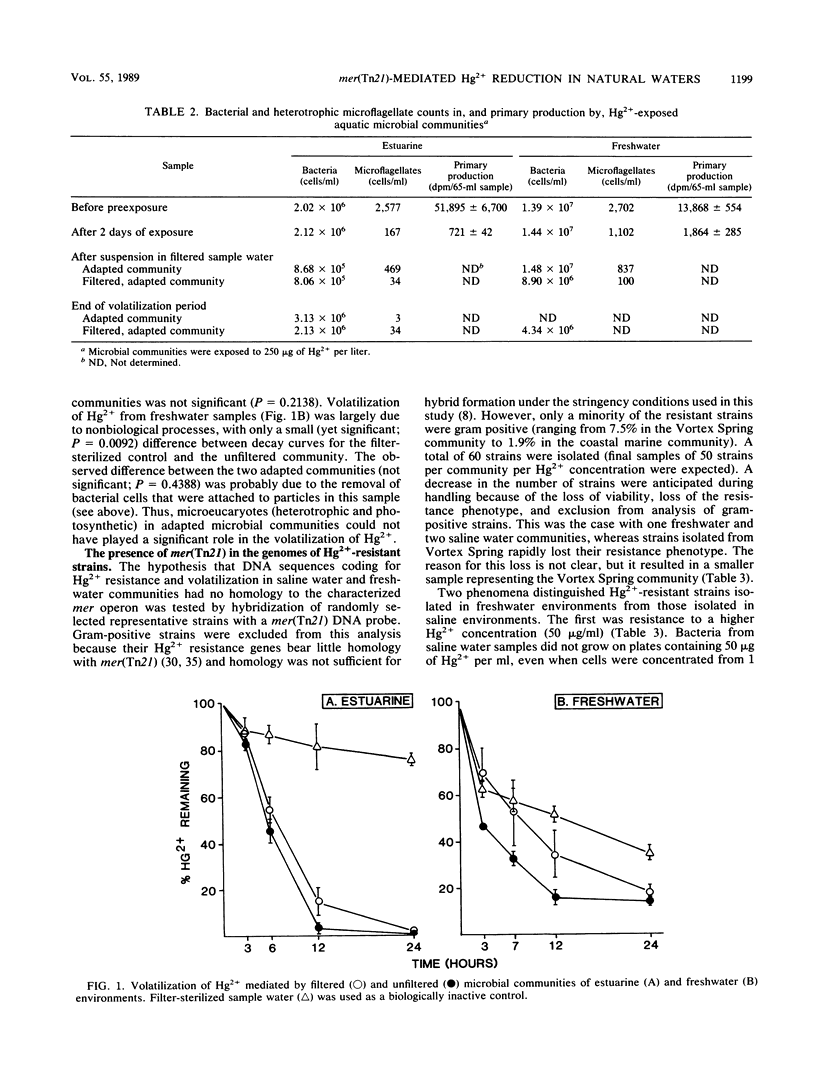
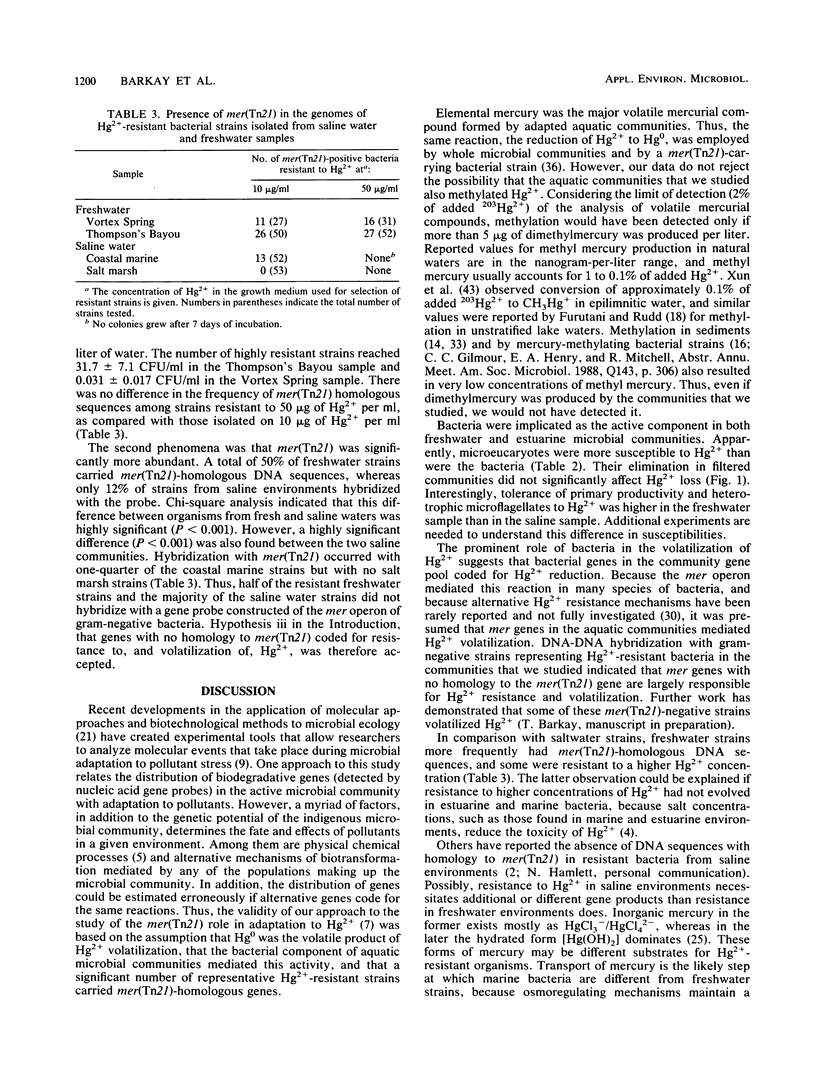
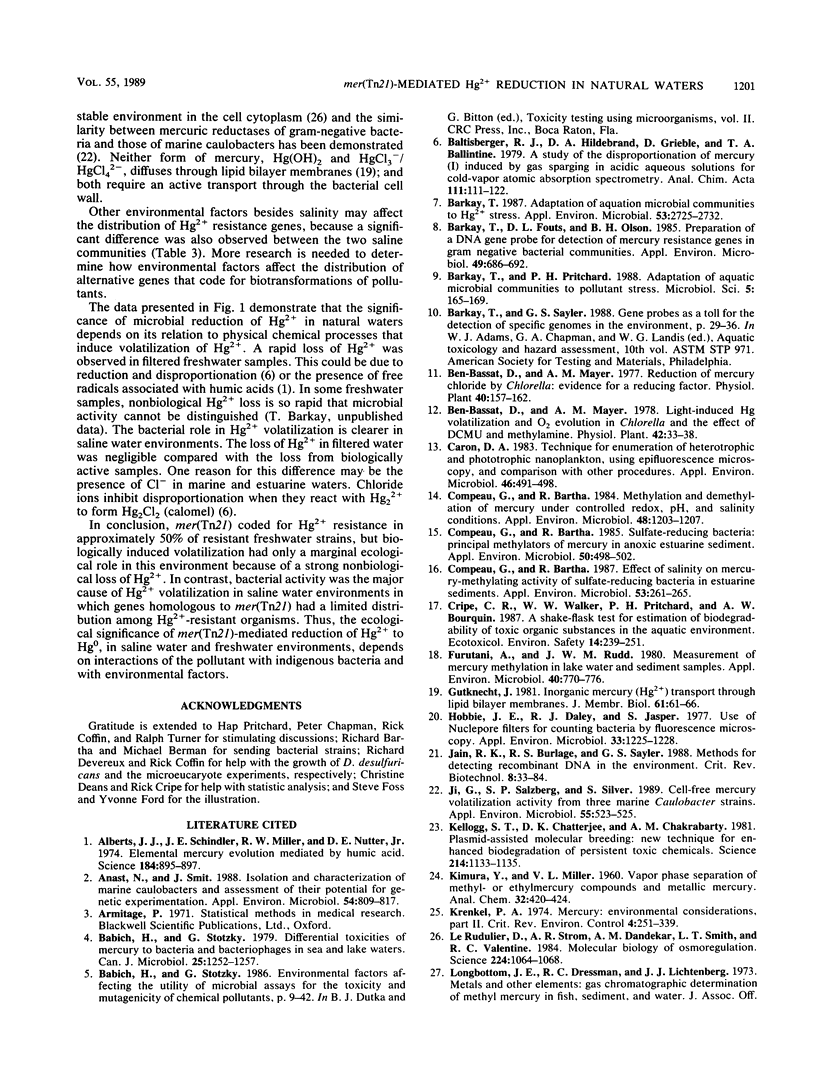
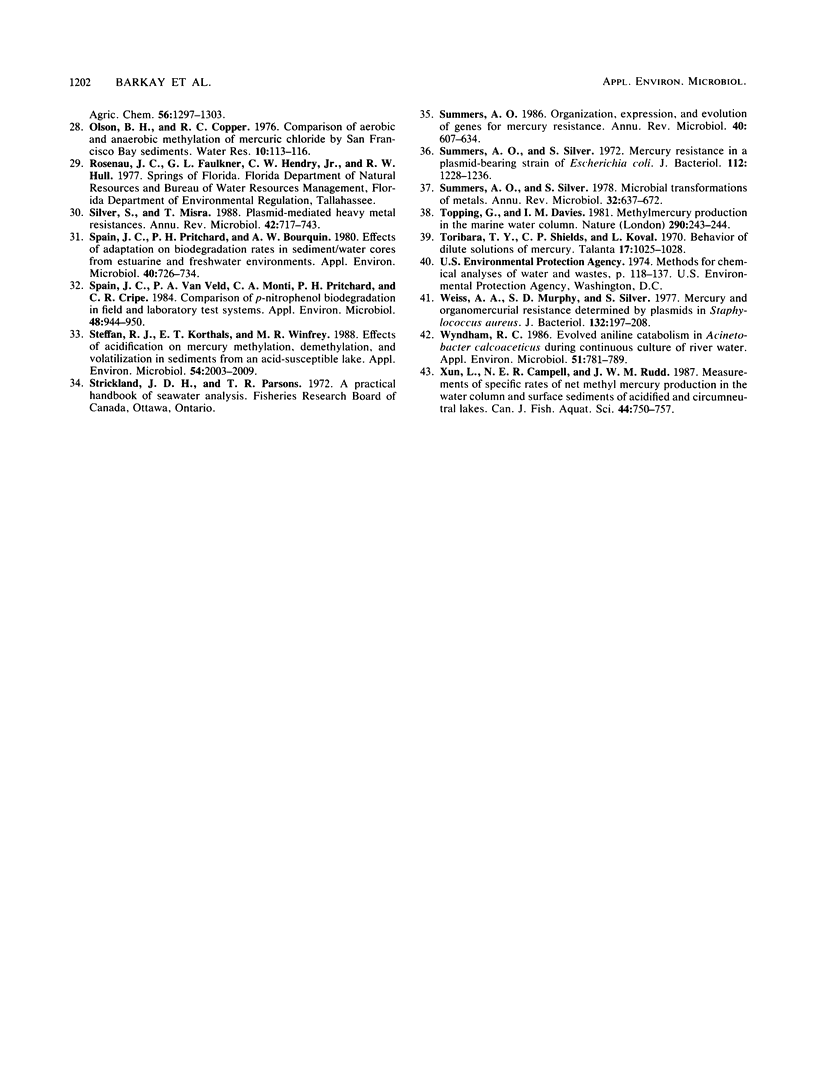
Selected References
These references are in PubMed. This may not be the complete list of references from this article.
- Alberts J. J., Schindler J. E., Miller R. W., Nutter D. E., Jr Elemental mercury evolution mediated by humic Acid. Science. 1974 May 24;184(4139):895–897. doi: 10.1126/science.184.4139.895. [DOI] [PubMed] [Google Scholar]
- Anast Nick, Smit John. Isolation and Characterization of Marine Caulobacters and Assessment of Their Potential for Genetic Experimentation. Appl Environ Microbiol. 1988 Mar;54(3):809–817. doi: 10.1128/aem.54.3.809-817.1988. [DOI] [PMC free article] [PubMed] [Google Scholar]
- Babich H., Stotzky G. Differential toxicities of mercury to bacteria and bacteriophages in sea and in lake water. Can J Microbiol. 1979 Nov;25(11):1252–1257. doi: 10.1139/m79-197. [DOI] [PubMed] [Google Scholar]
- Barkay T. Adaptation of aquatic microbial communities to hg stress. Appl Environ Microbiol. 1987 Dec;53(12):2725–2732. doi: 10.1128/aem.53.12.2725-2732.1987. [DOI] [PMC free article] [PubMed] [Google Scholar]
- Barkay T., Fouts D. L., Olson B. H. Preparation of a DNA gene probe for detection of mercury resistance genes in gram-negative bacterial communities. Appl Environ Microbiol. 1985 Mar;49(3):686–692. doi: 10.1128/aem.49.3.686-692.1985. [DOI] [PMC free article] [PubMed] [Google Scholar]
- Barkay T., Pritchard H. Adaptation of aquatic microbial communities to pollutant stress. Microbiol Sci. 1988 Jun;5(6):165–169. [PubMed] [Google Scholar]
- Caron D. A. Technique for enumeration of heterotrophic and phototrophic nanoplankton, using epifluorescence microscopy, and comparison with other procedures. Appl Environ Microbiol. 1983 Aug;46(2):491–498. doi: 10.1128/aem.46.2.491-498.1983. [DOI] [PMC free article] [PubMed] [Google Scholar]
- Compeau G. C., Bartha R. Effect of salinity on mercury-methylating activity of sulfate-reducing bacteria in estuarine sediments. Appl Environ Microbiol. 1987 Feb;53(2):261–265. doi: 10.1128/aem.53.2.261-265.1987. [DOI] [PMC free article] [PubMed] [Google Scholar]
- Compeau G. C., Bartha R. Sulfate-reducing bacteria: principal methylators of mercury in anoxic estuarine sediment. Appl Environ Microbiol. 1985 Aug;50(2):498–502. doi: 10.1128/aem.50.2.498-502.1985. [DOI] [PMC free article] [PubMed] [Google Scholar]
- Compeau G., Bartha R. Methylation and demethylation of mercury under controlled redox, pH and salinity conditions. Appl Environ Microbiol. 1984 Dec;48(6):1203–1207. doi: 10.1128/aem.48.6.1203-1207.1984. [DOI] [PMC free article] [PubMed] [Google Scholar]
- Cripe C. R., Walker W. W., Pritchard P. H., Bourquin A. W. A shake-flask test for estimation of biodegradability of toxic organic substances in the aquatic environment. Ecotoxicol Environ Saf. 1987 Dec;14(3):239–251. doi: 10.1016/0147-6513(87)90067-4. [DOI] [PubMed] [Google Scholar]
- Furutani A., Rudd J. W. Measurement of mercury methylation in lake water and sediment samples. Appl Environ Microbiol. 1980 Oct;40(4):770–776. doi: 10.1128/aem.40.4.770-776.1980. [DOI] [PMC free article] [PubMed] [Google Scholar]
- Hobbie J. E., Daley R. J., Jasper S. Use of nuclepore filters for counting bacteria by fluorescence microscopy. Appl Environ Microbiol. 1977 May;33(5):1225–1228. doi: 10.1128/aem.33.5.1225-1228.1977. [DOI] [PMC free article] [PubMed] [Google Scholar]
- Jain R. K., Burlage R. S., Sayler G. S. Methods for detecting recombinant DNA in the environment. Crit Rev Biotechnol. 1988;8(1):33–84. doi: 10.3109/07388558809150537. [DOI] [PubMed] [Google Scholar]
- Ji G. Y., Salzberg S. P., Silver S. Cell-free mercury volatilization activity from three marine caulobacter strains. Appl Environ Microbiol. 1989 Feb;55(2):523–525. doi: 10.1128/aem.55.2.523-525.1989. [DOI] [PMC free article] [PubMed] [Google Scholar]
- Kellogg S. T., Chatterjee D. K., Chakrabarty A. M. Plasmid-assisted molecular breeding: new technique for enhanced biodegradation of persistent toxic chemicals. Science. 1981 Dec 4;214(4525):1133–1135. doi: 10.1126/science.7302584. [DOI] [PubMed] [Google Scholar]
- Le Rudulier D., Strom A. R., Dandekar A. M., Smith L. T., Valentine R. C. Molecular biology of osmoregulation. Science. 1984 Jun 8;224(4653):1064–1068. doi: 10.1126/science.224.4653.1064. [DOI] [PubMed] [Google Scholar]
- Silver S., Misra T. K. Plasmid-mediated heavy metal resistances. Annu Rev Microbiol. 1988;42:717–743. doi: 10.1146/annurev.mi.42.100188.003441. [DOI] [PubMed] [Google Scholar]
- Spain J. C., Pritchard P. H., Bourquin A. W. Effects of adaptation on biodegradation rates in sediment/water cores from estuarine and freshwater environments. Appl Environ Microbiol. 1980 Oct;40(4):726–734. doi: 10.1128/aem.40.4.726-734.1980. [DOI] [PMC free article] [PubMed] [Google Scholar]
- Spain J. C., Van Veld P. A., Monti C. A., Pritchard P. H., Cripe C. R. Comparison of p-Nitrophenol Biodegradation in Field and Laboratory Test Systems. Appl Environ Microbiol. 1984 Nov;48(5):944–950. doi: 10.1128/aem.48.5.944-950.1984. [DOI] [PMC free article] [PubMed] [Google Scholar]
- Steffan R. J., Korthals E. T., Winfrey M. R. Effects of acidification on mercury methylation, demethylation, and volatilization in sediments from an acid-susceptible lake. Appl Environ Microbiol. 1988 Aug;54(8):2003–2009. doi: 10.1128/aem.54.8.2003-2009.1988. [DOI] [PMC free article] [PubMed] [Google Scholar]
- Summers A. O. Organization, expression, and evolution of genes for mercury resistance. Annu Rev Microbiol. 1986;40:607–634. doi: 10.1146/annurev.mi.40.100186.003135. [DOI] [PubMed] [Google Scholar]
- Summers A. O., Silver S. Mercury resistance in a plasmid-bearing strain of Escherichia coli. J Bacteriol. 1972 Dec;112(3):1228–1236. doi: 10.1128/jb.112.3.1228-1236.1972. [DOI] [PMC free article] [PubMed] [Google Scholar]
- Summers A. O., Silver S. Microbial transformations of metals. Annu Rev Microbiol. 1978;32:637–672. doi: 10.1146/annurev.mi.32.100178.003225. [DOI] [PubMed] [Google Scholar]
- Weiss A. A., Murphy S. D., Silver S. Mercury and organomercurial resistances determined by plasmids in Staphylococcus aureus. J Bacteriol. 1977 Oct;132(1):197–208. doi: 10.1128/jb.132.1.197-208.1977. [DOI] [PMC free article] [PubMed] [Google Scholar]
- Wyndham R. C. Evolved aniline catabolism in Acinetobacter calcoaceticus during continuous culture of river water. Appl Environ Microbiol. 1986 Apr;51(4):781–789. doi: 10.1128/aem.51.4.781-789.1986. [DOI] [PMC free article] [PubMed] [Google Scholar]