Abstract
Significant quantities of Ag(I), Cu(II), and Cr(III) were bound to isolated Bacillus subtilis 168 walls, Escherichia coli K-12 envelopes, kaolinite and smectite clays, and the corresponding organic material-clay aggregates (1:1, wt/wt). These sorbed metals were leached with HNO3, Ca(NO3)2, EDTA, fulvic acid, and lysozyme at several concentrations over 48 h at room temperature. The remobilization of the sorbed metals depended on the physical properties of the organic and clay surfaces and on the character and concentration of the leaching agents. In general, the order of remobilization of metals was Cr much less than Ag less than Cu. Cr was very stable in the wall, clay, and composite systems; pH 3.0, 500 microM EDTA, 120-ppm [mg liter-1] fulvic acid, and 160-ppm Ca remobilized less than 32% (wt/wt) of sorbed Cr. Ag (45 to 87%) and Cu (up to 100%) were readily removed by these agents. Although each leaching agent was effective at mobilizing certain metals, elevated Ca or acidic pH produced the greatest overall mobility. The organic chelators were less effective. Lysozyme digestion of Bacillus walls remobilized Cu from walls and Cu-wall-kaolinite composites, but Ag, Cr, and smectite partially inhibited enzyme activity, and the metals remained insoluble. The extent of metal remobilization was not always dependent on increasing concentrations of leaching agents; for example, Ag mobility decreased with some clays and some composites treated with high fulvic acid, EDTA, and lysozyme concentrations. Sometimes the organic material-clay composites reacted in a manner distinctly different from that of their individual counterparts; e.g., 25% less Cu was remobilized from wall- and envelope-smectite composites than from walls, envelopes, or smectite individually in 500 microM EDTA. Alternatively, treatment with 160-ppm Ca removed 1.5 to 10 times more Ag from envelope-kaolinite composites than from the individual components. The particle size of the deposited metal may account for some of the stability changes; those metals that formed large, compact aggregates (Cr and Ag) as seen by transmission electron microscopy were less likely to be remobilized. In summary, it is apparent that remobilization of toxic heavy metals in sediments, soils, and the vadose zone is a complicated issue. Predictions based on single inorganic or organic component systems are too simplistic.
Full text
PDF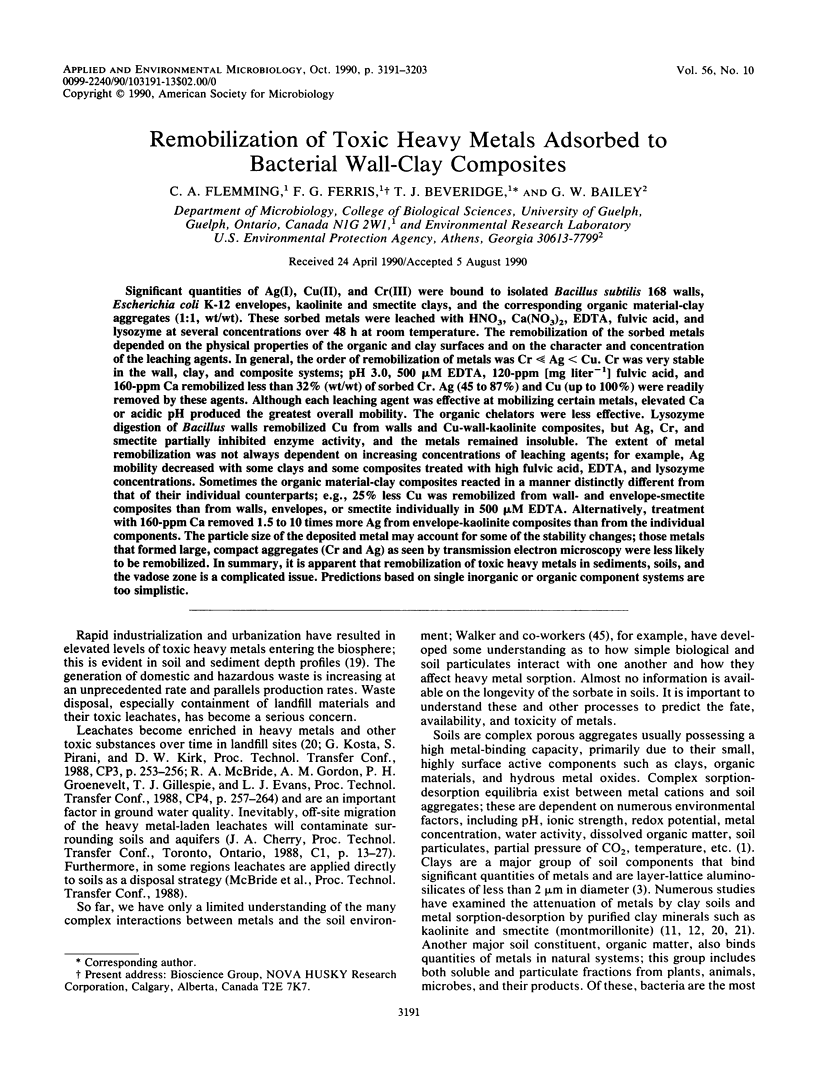
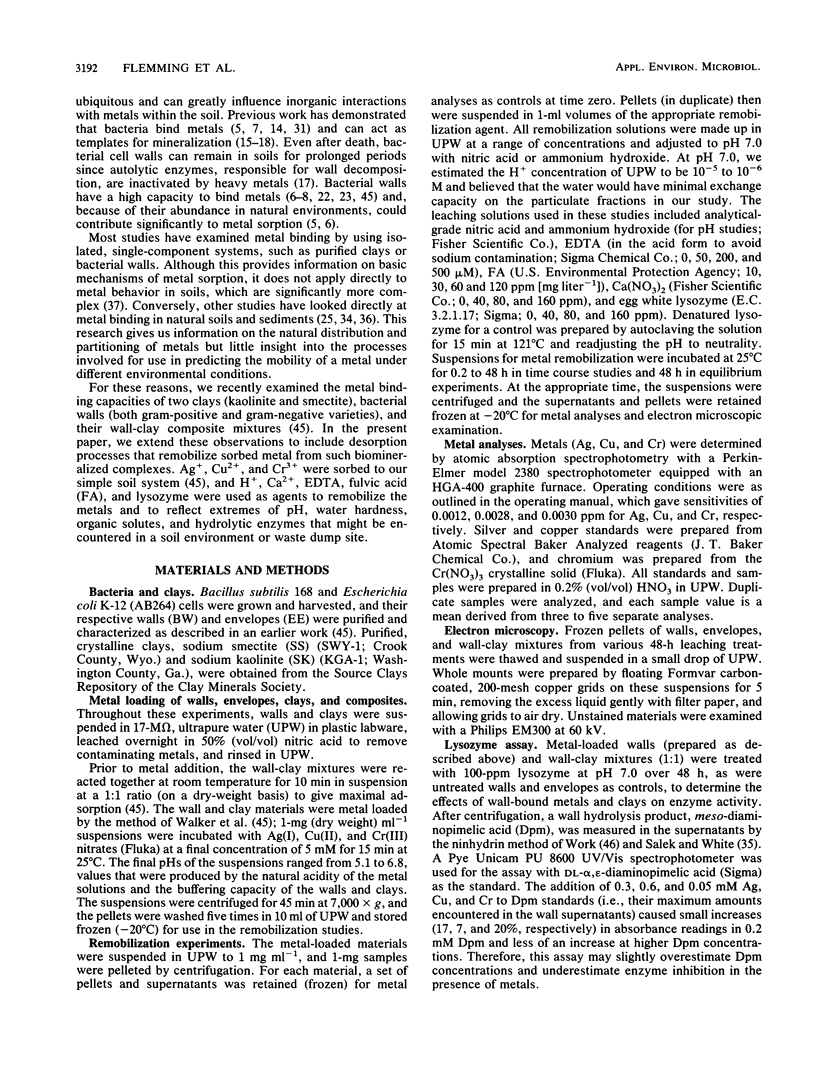
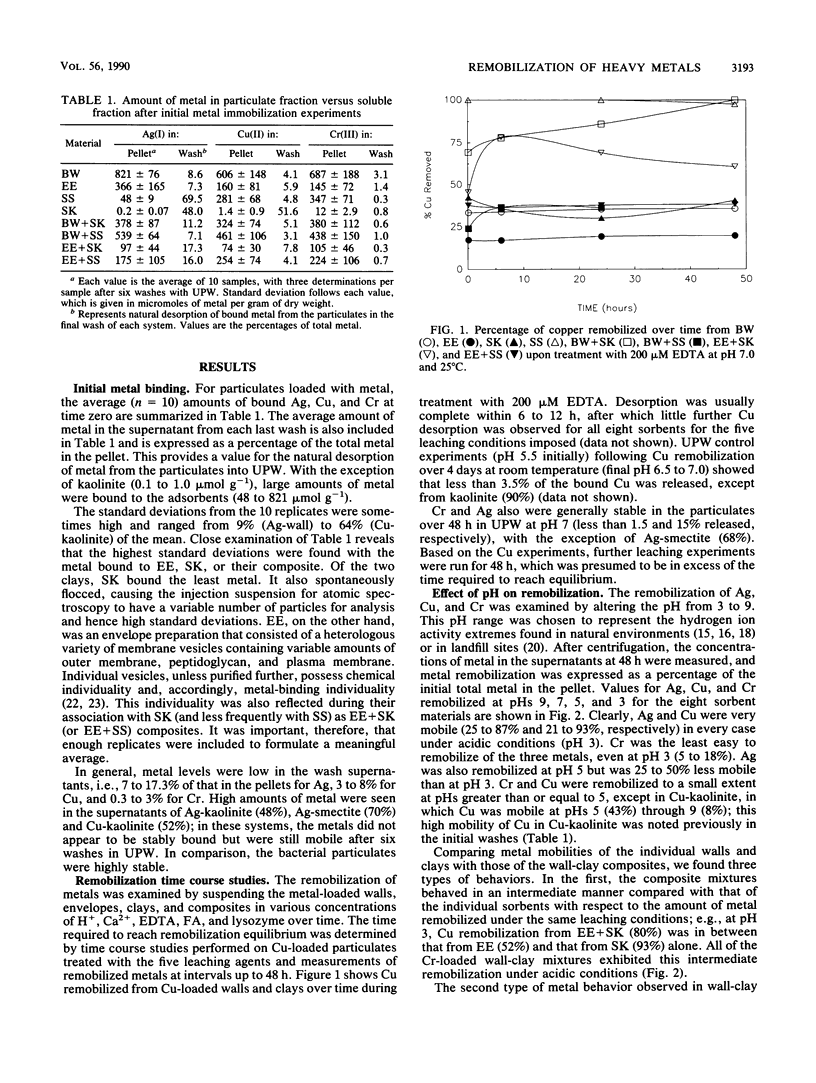
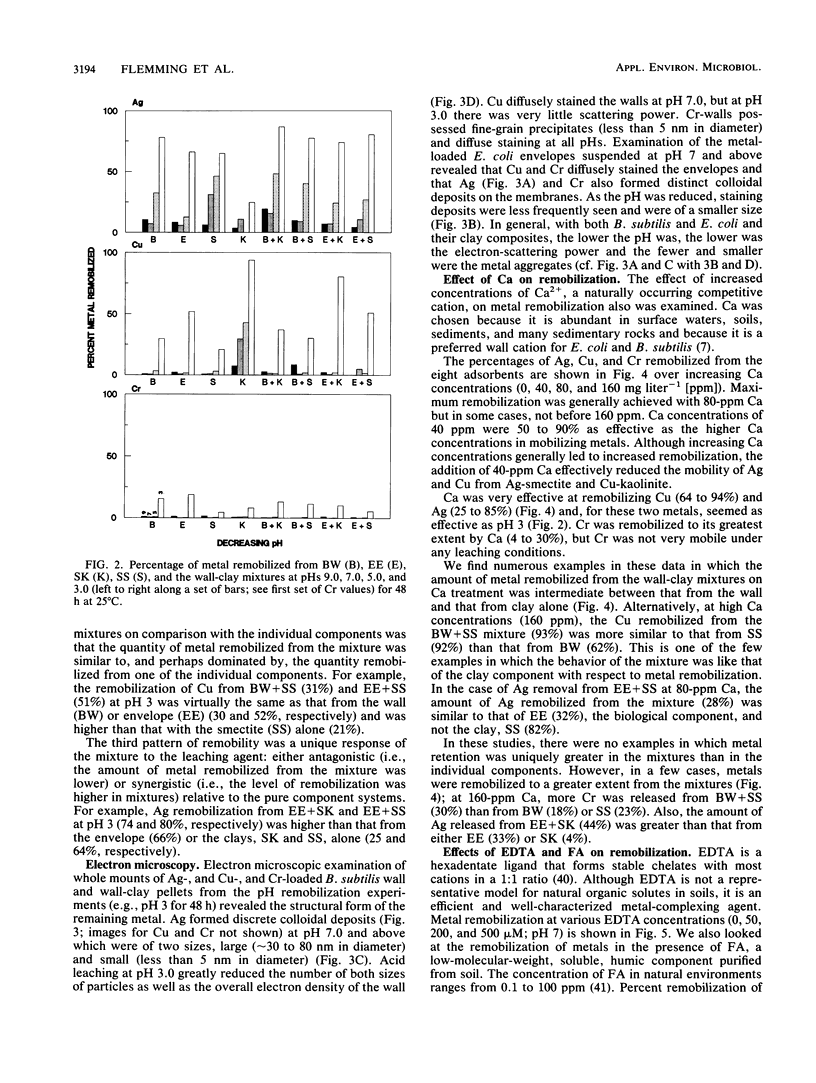
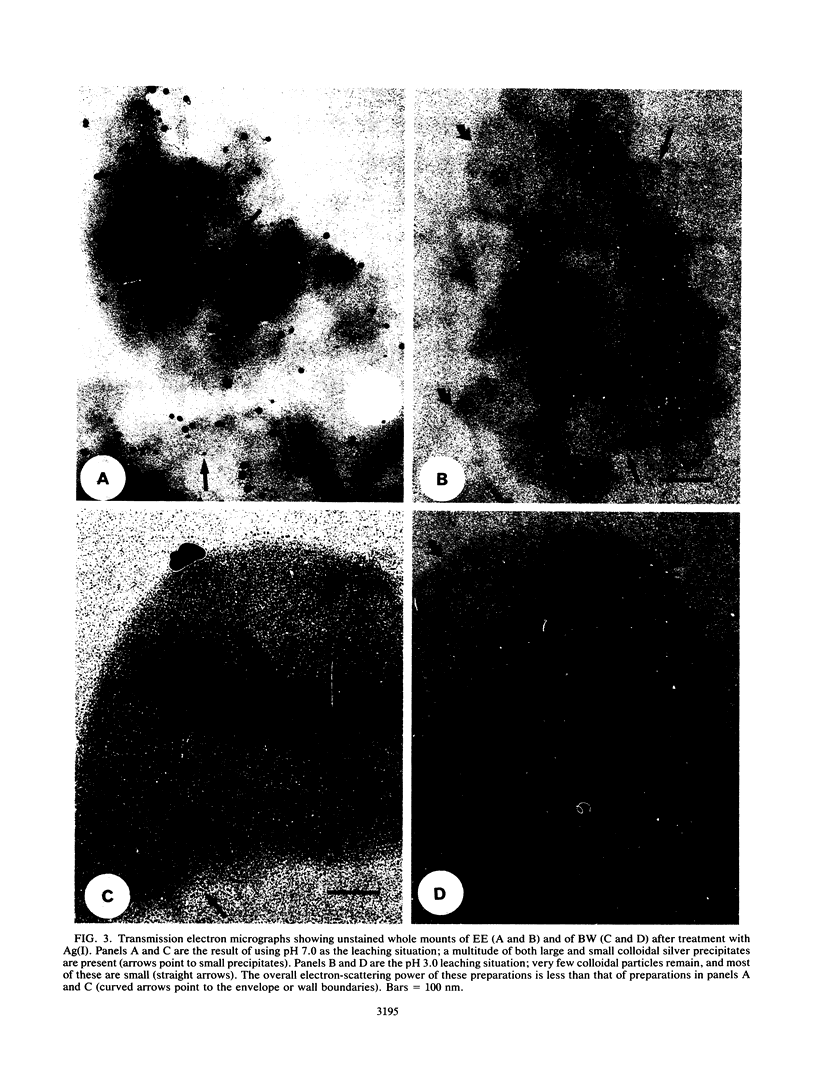
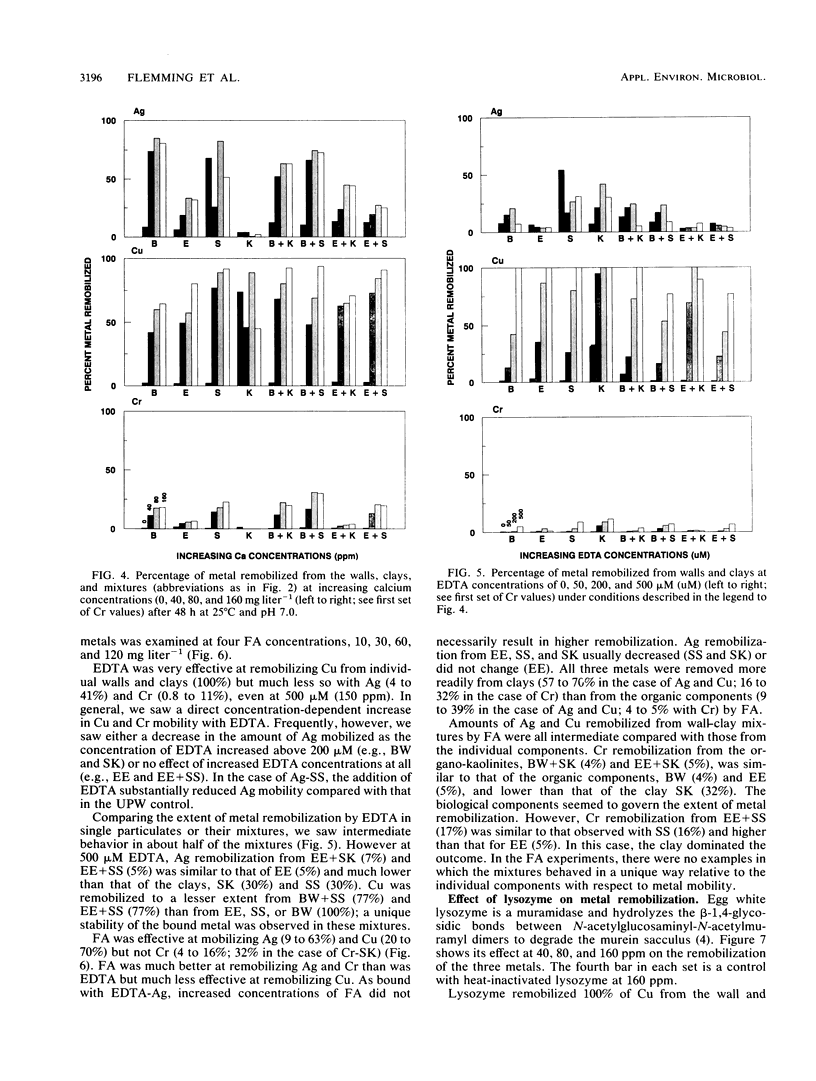
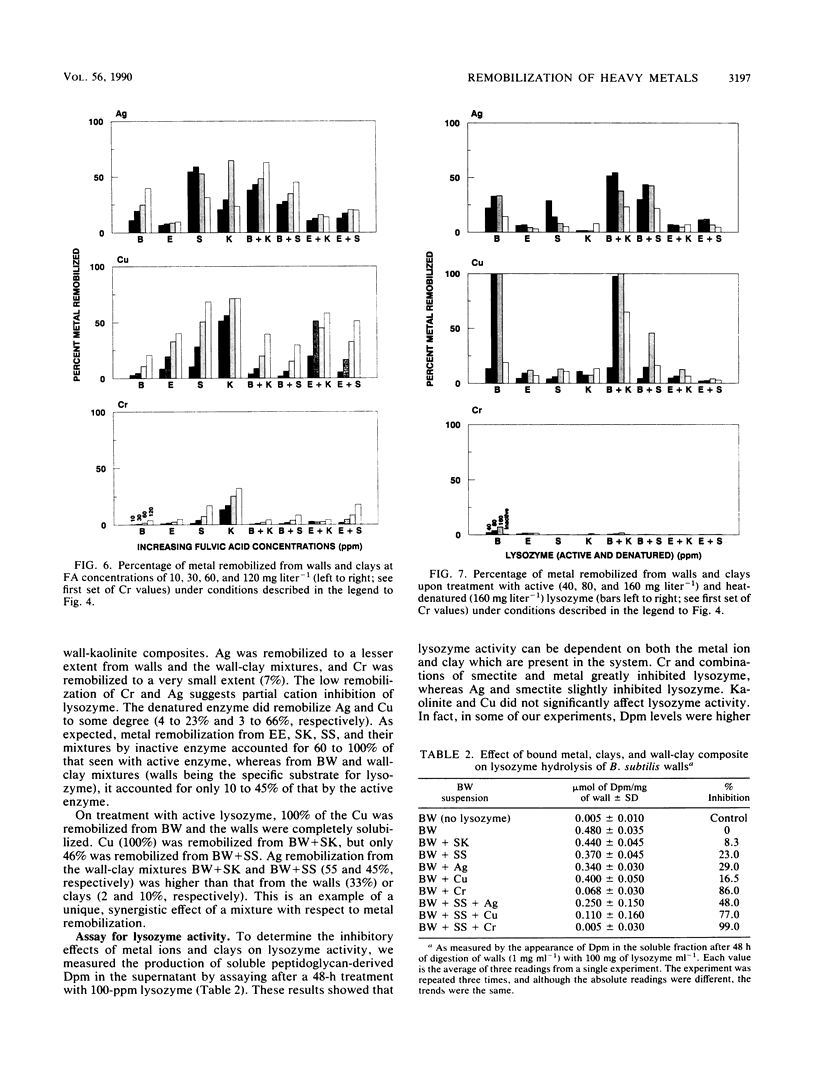
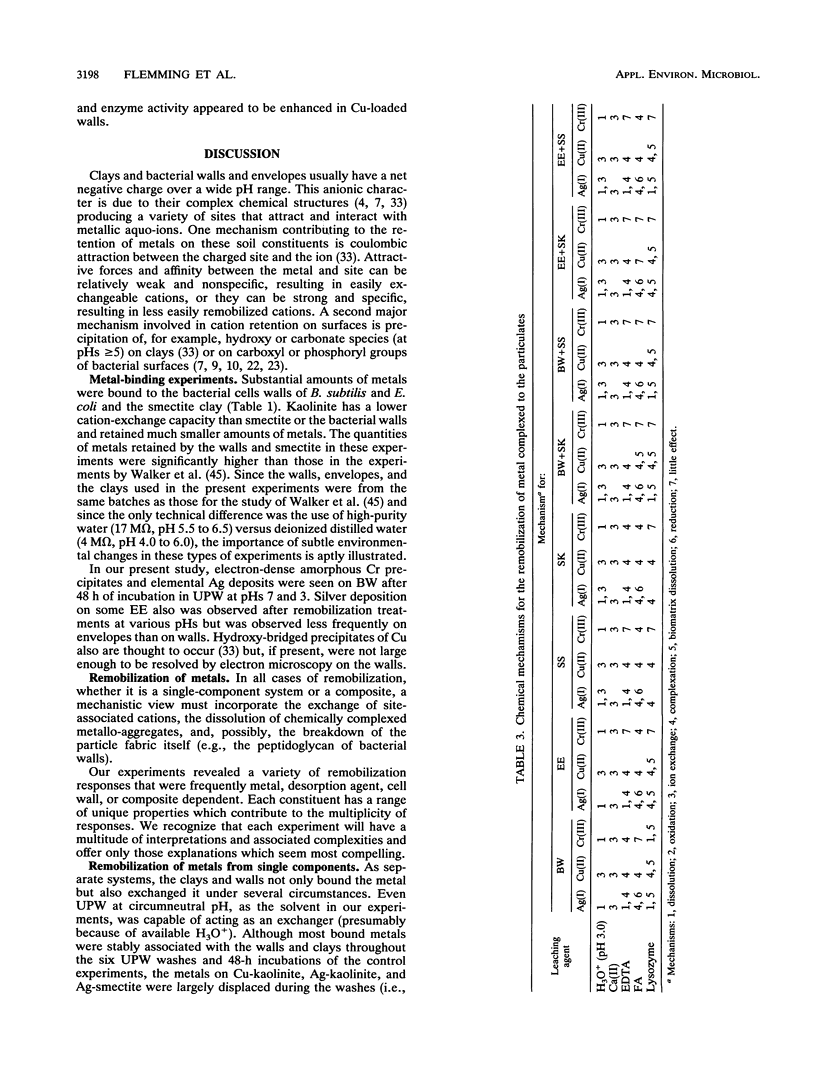
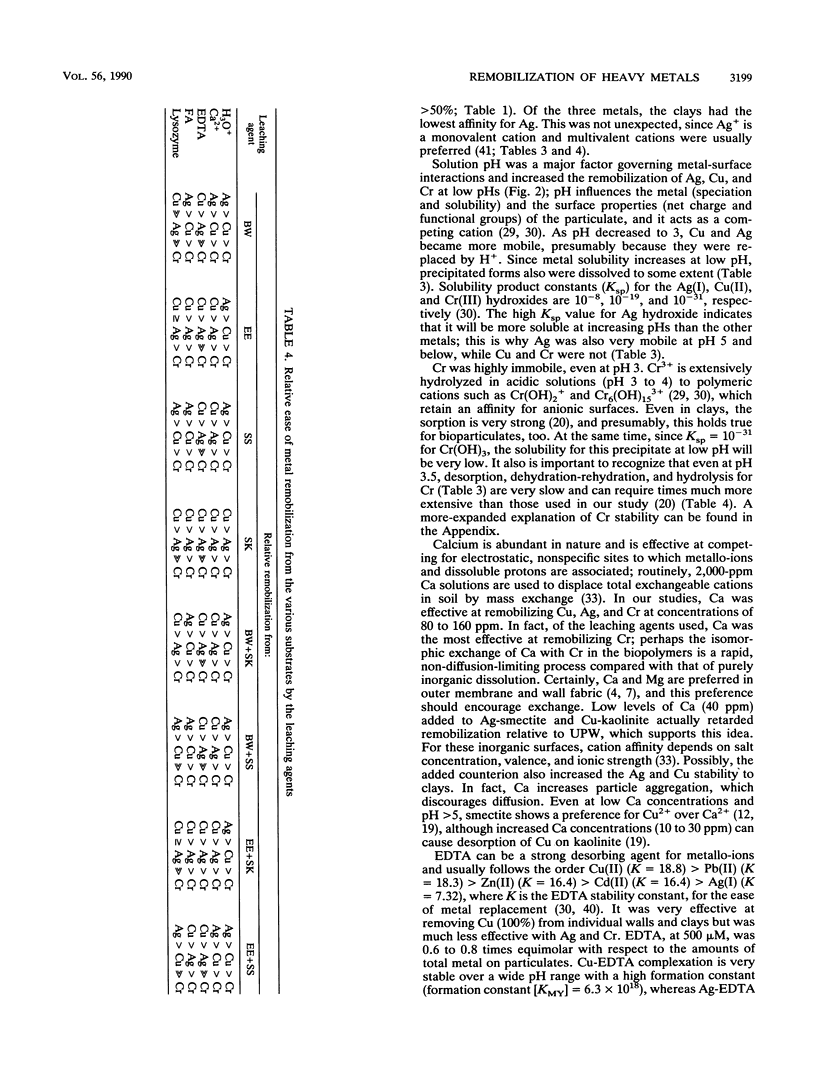
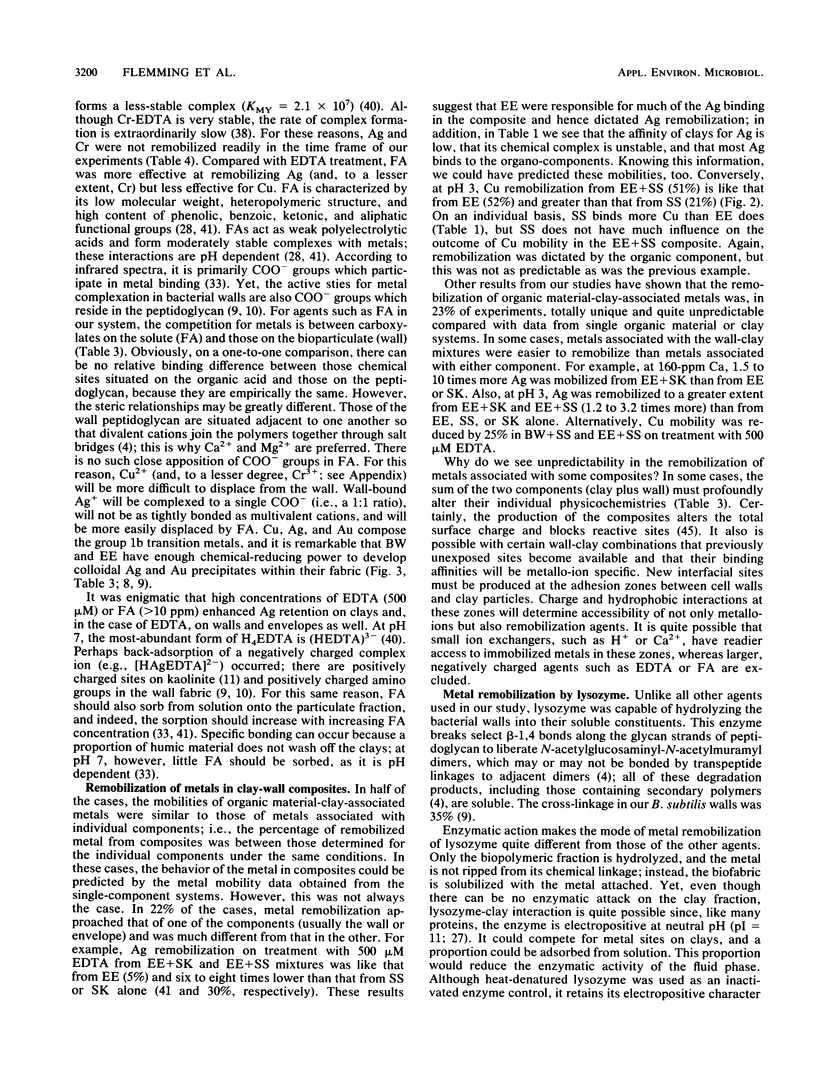
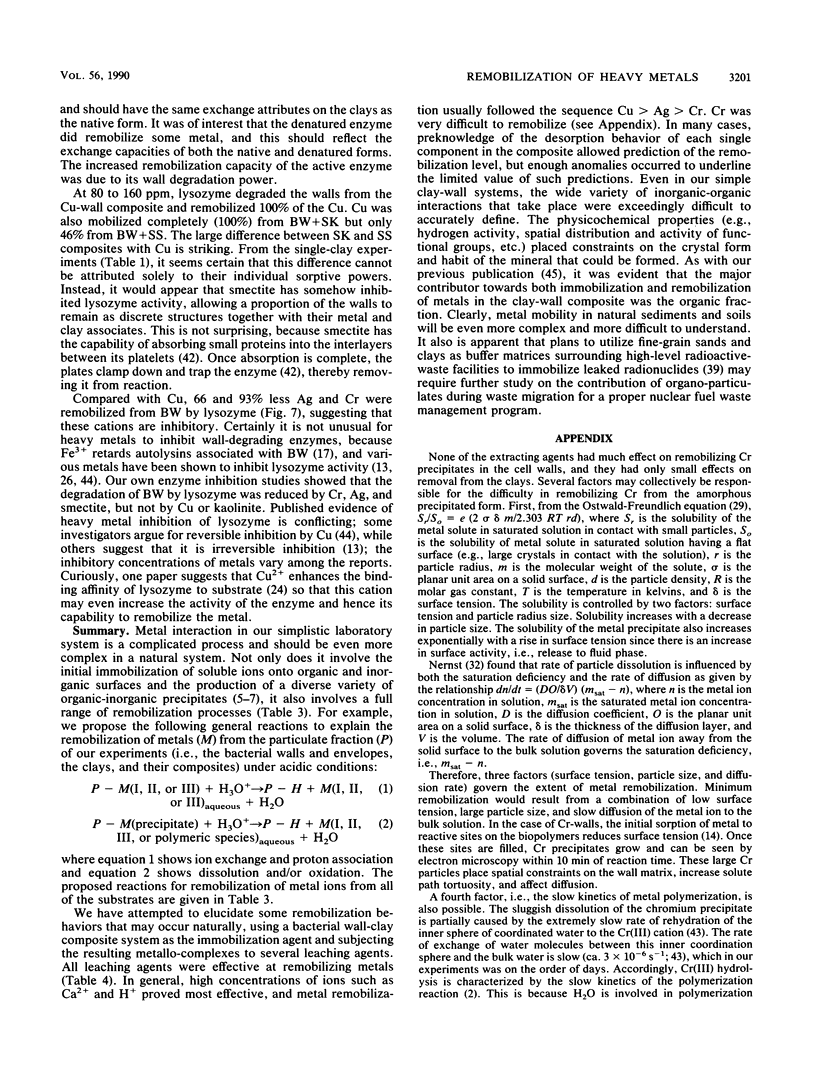
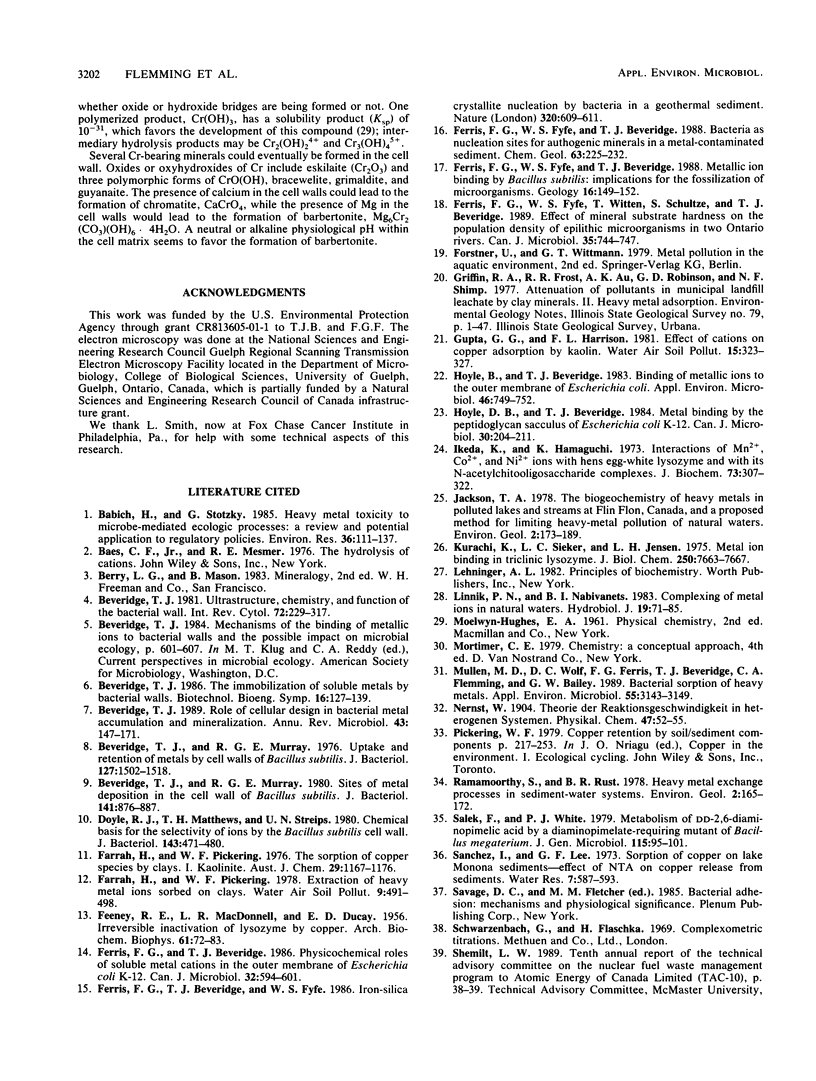
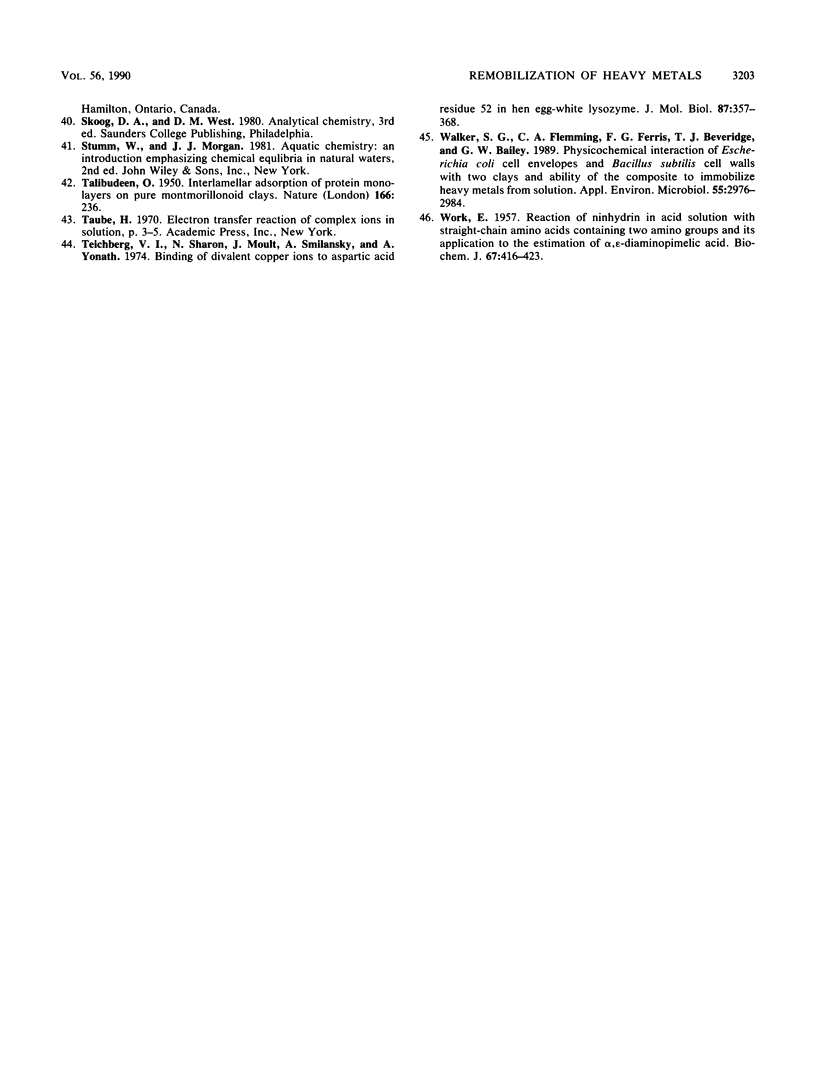
Images in this article
Selected References
These references are in PubMed. This may not be the complete list of references from this article.
- Babich H., Stotzky G. Heavy metal toxicity to microbe-mediated ecologic processes: a review and potential application to regulatory policies. Environ Res. 1985 Feb;36(1):111–137. doi: 10.1016/0013-9351(85)90011-8. [DOI] [PubMed] [Google Scholar]
- Beveridge T. J., Murray R. G. Sites of metal deposition in the cell wall of Bacillus subtilis. J Bacteriol. 1980 Feb;141(2):876–887. doi: 10.1128/jb.141.2.876-887.1980. [DOI] [PMC free article] [PubMed] [Google Scholar]
- Beveridge T. J., Murray R. G. Uptake and retention of metals by cell walls of Bacillus subtilis. J Bacteriol. 1976 Sep;127(3):1502–1518. doi: 10.1128/jb.127.3.1502-1518.1976. [DOI] [PMC free article] [PubMed] [Google Scholar]
- Beveridge T. J. Role of cellular design in bacterial metal accumulation and mineralization. Annu Rev Microbiol. 1989;43:147–171. doi: 10.1146/annurev.mi.43.100189.001051. [DOI] [PubMed] [Google Scholar]
- Beveridge T. J. Ultrastructure, chemistry, and function of the bacterial wall. Int Rev Cytol. 1981;72:229–317. doi: 10.1016/s0074-7696(08)61198-5. [DOI] [PubMed] [Google Scholar]
- Doyle R. J., Matthews T. H., Streips U. N. Chemical basis for selectivity of metal ions by the Bacillus subtilis cell wall. J Bacteriol. 1980 Jul;143(1):471–480. doi: 10.1128/jb.143.1.471-480.1980. [DOI] [PMC free article] [PubMed] [Google Scholar]
- FEENEY R. E., MACDONNELL L. R., DUCAY E. D. Irreversible inactivation of lysozyme by copper. Arch Biochem Biophys. 1956 Mar;61(1):72–83. doi: 10.1016/0003-9861(56)90317-4. [DOI] [PubMed] [Google Scholar]
- Ferris F. G., Beveridge T. J. Physicochemical roles of soluble metal cations in the outer membrane of Escherichia coli K-12. Can J Microbiol. 1986 Jul;32(7):594–601. doi: 10.1139/m86-110. [DOI] [PubMed] [Google Scholar]
- Hoyle B. D., Beveridge T. J. Metal binding by the peptidoglycan sacculus of Escherichia coli K-12. Can J Microbiol. 1984 Feb;30(2):204–211. doi: 10.1139/m84-031. [DOI] [PubMed] [Google Scholar]
- Hoyle B., Beveridge T. J. Binding of metallic ions to the outer membrane of Escherichia coli. Appl Environ Microbiol. 1983 Sep;46(3):749–752. doi: 10.1128/aem.46.3.749-752.1983. [DOI] [PMC free article] [PubMed] [Google Scholar]
- Ikeda K., Hamaguchi K. Interactions of Mn 2+, Co 2+, and Ni 2+ ions with hen egg-white lysozyme and with its N-acetylchitooligosaccharide complexes. J Biochem. 1973 Feb;73(2):307–322. [PubMed] [Google Scholar]
- Kurachi K., Sieker L. C., Jensen L. H. Metal ion binding in triclinic lysozyme. J Biol Chem. 1975 Oct 10;250(19):7663–7667. [PubMed] [Google Scholar]
- Mullen M. D., Wolf D. C., Ferris F. G., Beveridge T. J., Flemming C. A., Bailey G. W. Bacterial sorption of heavy metals. Appl Environ Microbiol. 1989 Dec;55(12):3143–3149. doi: 10.1128/aem.55.12.3143-3149.1989. [DOI] [PMC free article] [PubMed] [Google Scholar]
- TALIBUDEEN O. Interlamellar adsorption of protein monolayers on pure montmorillonoid clays. Nature. 1950 Aug 5;166(4214):236–236. doi: 10.1038/166236a0. [DOI] [PubMed] [Google Scholar]
- Teichberg V. I., Sharon N., Moult J., Smilansky A., Yonath A. Binding of divalent copper ions to aspartic acid residue 52 in hen egg-white lysozyme. J Mol Biol. 1974 Aug 5;87(2):357–368. doi: 10.1016/0022-2836(74)90155-7. [DOI] [PubMed] [Google Scholar]
- WORK E. Reaction of ninhydrin in acid solution with straight-chain amino acids containing two amino groups and its application to the estimation of alpha epsilon-diaminopimelic acid. Biochem J. 1957 Nov;67(3):416–423. doi: 10.1042/bj0670416. [DOI] [PMC free article] [PubMed] [Google Scholar]
- Walker S. G., Flemming C. A., Ferris F. G., Beveridge T. J., Bailey G. W. Physicochemical interaction of Escherichia coli cell envelopes and Bacillus subtilis cell walls with two clays and ability of the composite to immobilize heavy metals from solution. Appl Environ Microbiol. 1989 Nov;55(11):2976–2984. doi: 10.1128/aem.55.11.2976-2984.1989. [DOI] [PMC free article] [PubMed] [Google Scholar]