Abstract
The chemotactic responses by starved cells of marine Vibrio sp. strain S14 differed from those elicited by cells that were not nutrient limited. The rate of chemotaxis at different concentrations of several attractants varied for starved and growing cells. Vibrio sp. strain S14 showed positive chemotaxis to leucine, valine, arginine, and glucose at the onset of energy and nutrient deprivation. A continued, though decreased, positive response was demonstrated fro leucine, arginine, and glucose at 10 h of starvation. Cells starved for 3 h displayed a stronger response to glucose than those starved for shorter or longer times. However, cells starved for 5 and 10 h responded more strongly to a lower concentration of glucose than did cells starved for 0 and 3 h. Starvation for 24 h elicited no measurable chemotaxis to leucine, arginine, or glucose. The motility decreased by over 95% in the cell population after 24 h of starvation, which resulted in a low sensitivity in the chemotaxis assay. A switch in the response to valine was observed by 3 h of starvation. The addition of nutrients of 22-h-starved cells elicited a temporary positive chemotactic response to leucine by 2 and 4 h of nutrient recovery, while cells at 1 and 6 h of recovery showed no response. At 2 h of recovery, the greatest response was recorded to 10−4 M leucine, whereas at 4 h it was to 10−2 M leucine. Ten to fifty percent of the 22-h-starved cell population regained their motility after 4 h of nutrient-aided recovery. It is possible that two types of chemosensory systems exist in marine bacteria. Starved and growing cells responded to different concentrations of the attractant, and growing cells displayed a saturated chemotactic system with leucine as the attractant, unlike the response during starvation.
Full text
PDF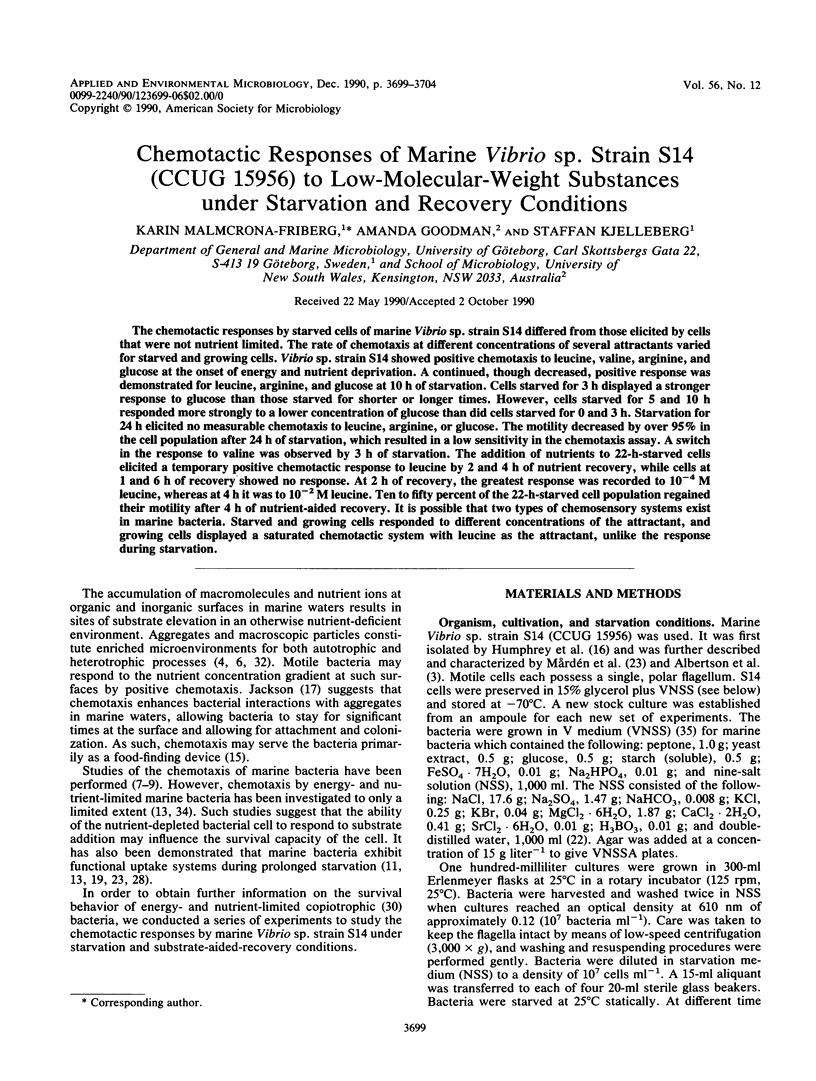
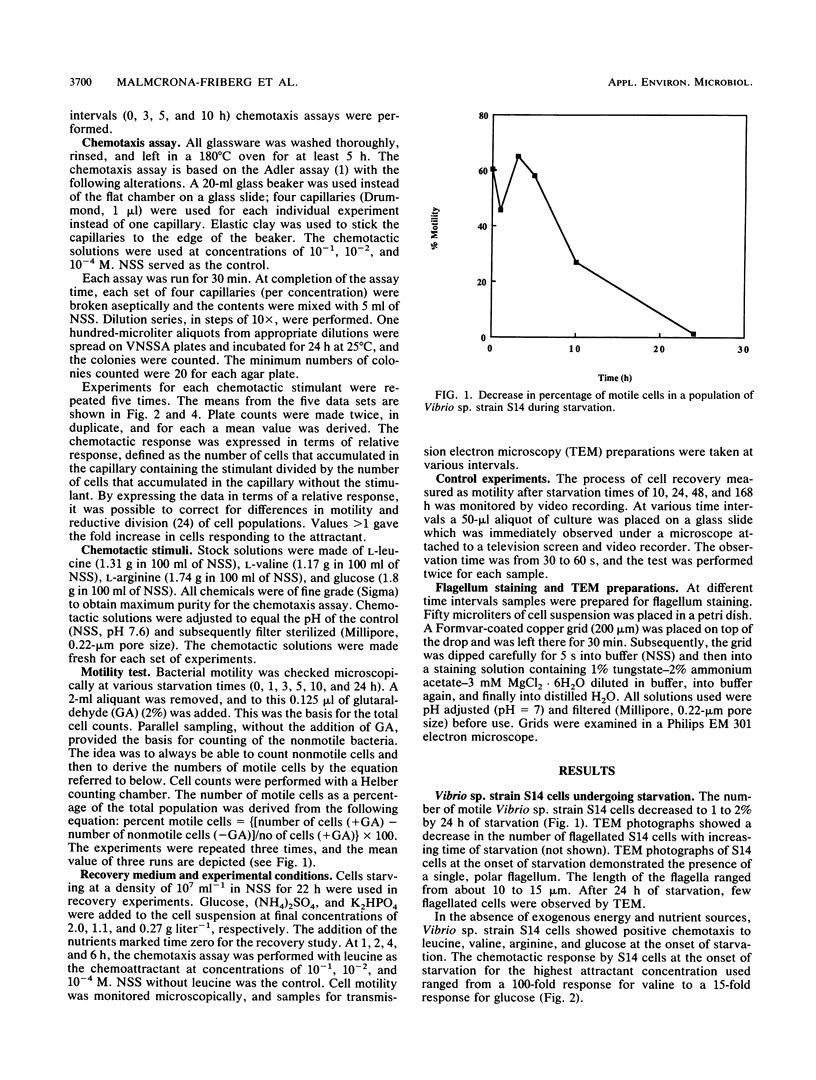
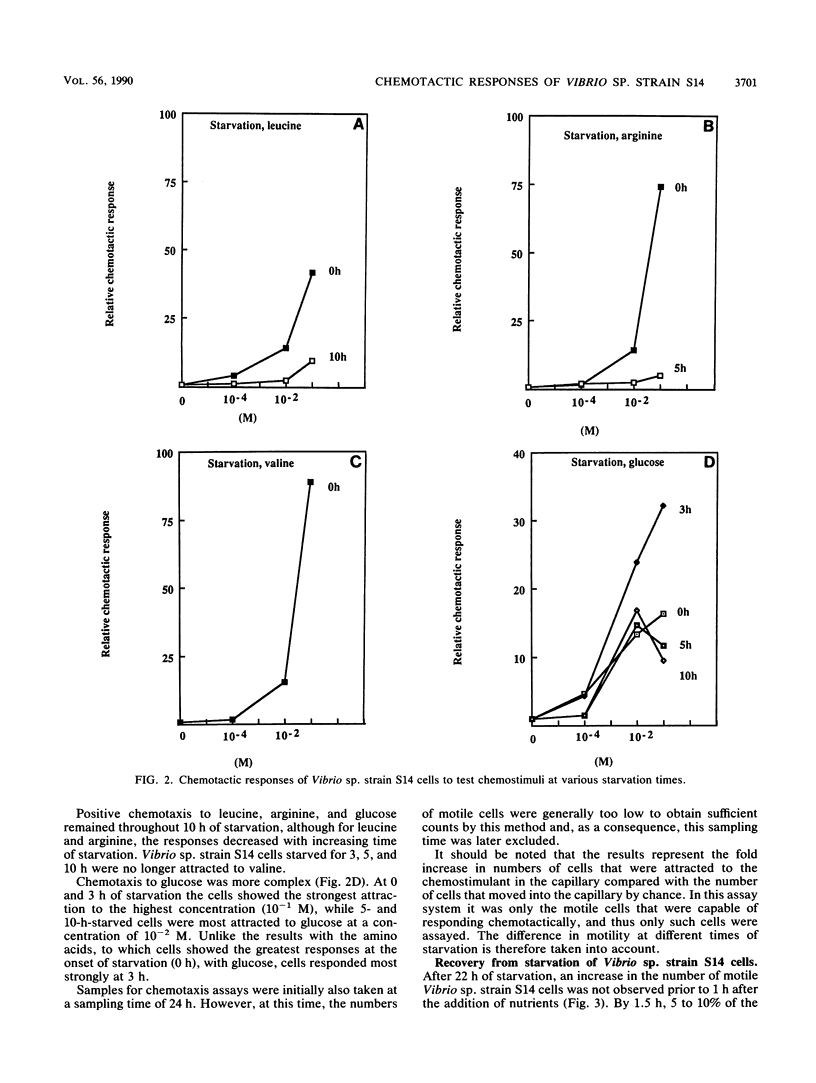
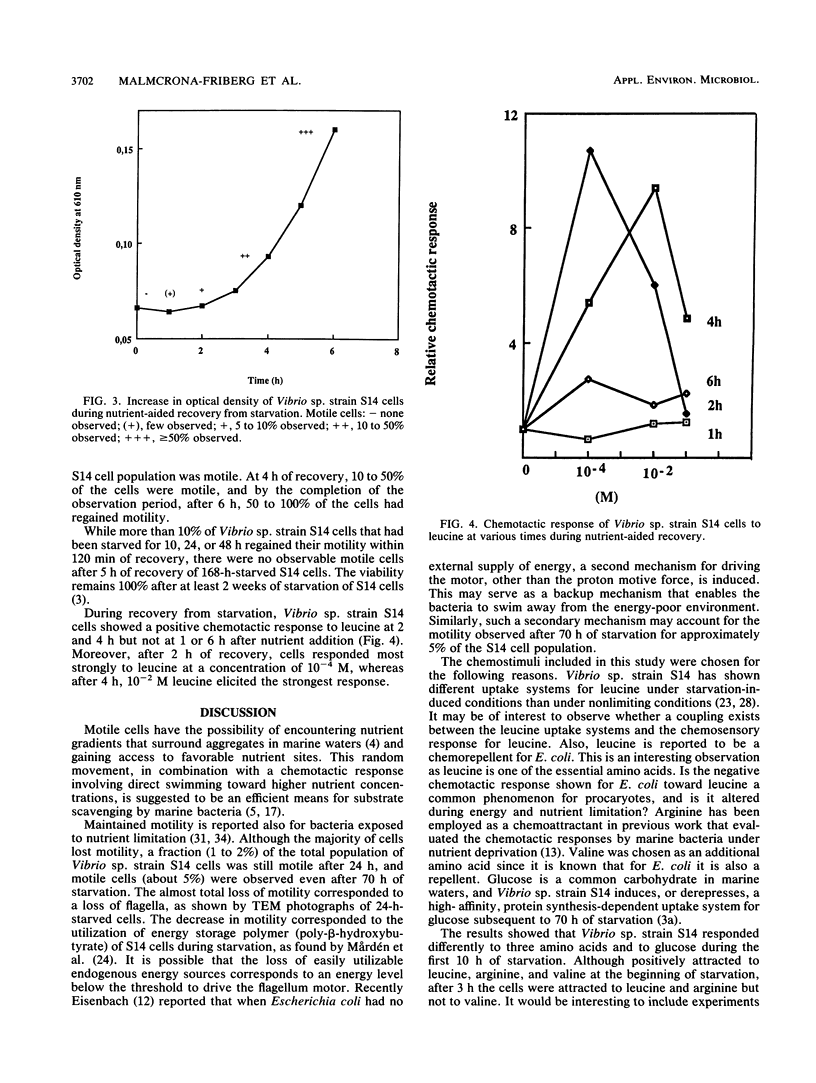
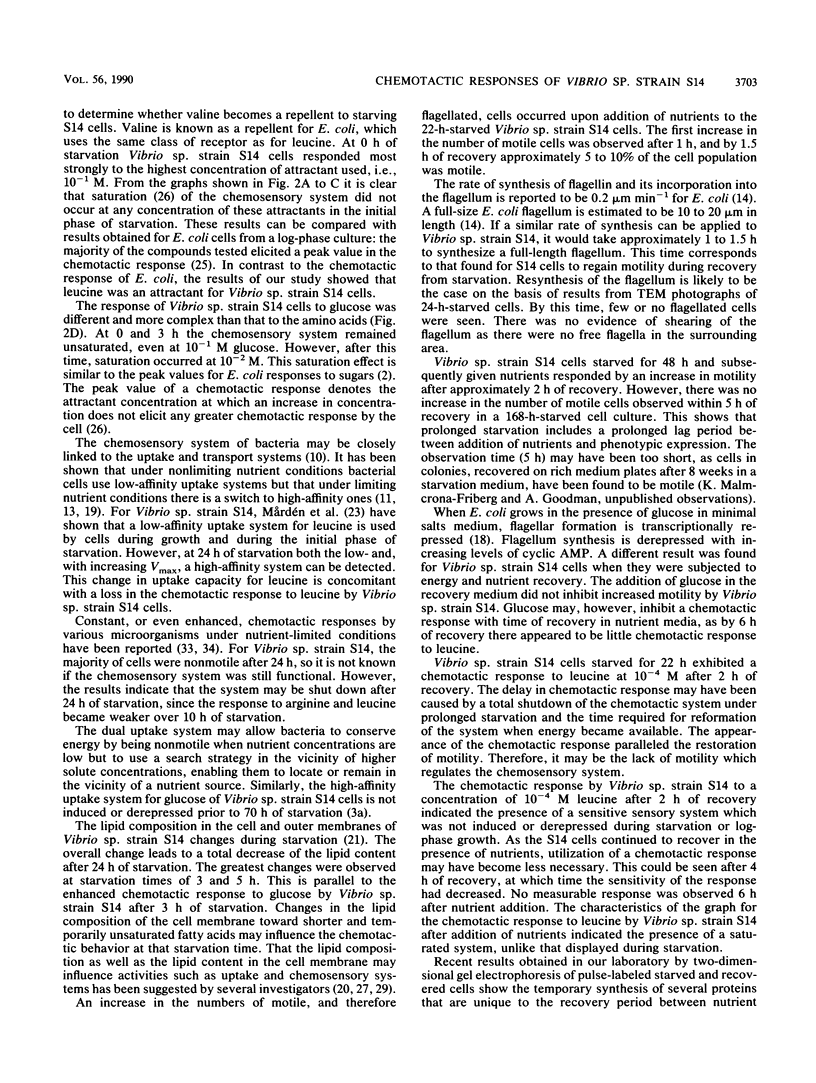
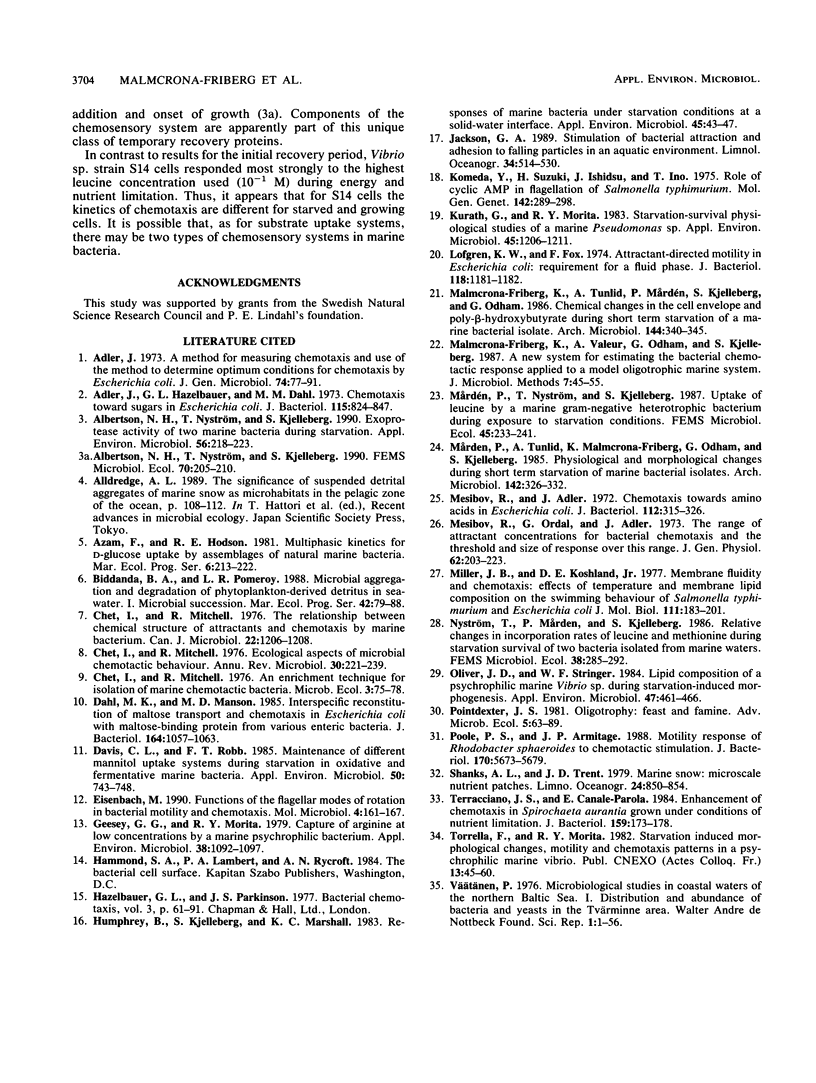
Selected References
These references are in PubMed. This may not be the complete list of references from this article.
- Adler J. A method for measuring chemotaxis and use of the method to determine optimum conditions for chemotaxis by Escherichia coli. J Gen Microbiol. 1973 Jan;74(1):77–91. doi: 10.1099/00221287-74-1-77. [DOI] [PubMed] [Google Scholar]
- Adler J., Hazelbauer G. L., Dahl M. M. Chemotaxis toward sugars in Escherichia coli. J Bacteriol. 1973 Sep;115(3):824–847. doi: 10.1128/jb.115.3.824-847.1973. [DOI] [PMC free article] [PubMed] [Google Scholar]
- Albertson N. H., Nyström T., Kjelleberg S. Exoprotease Activity of Two Marine Bacteria during Starvation. Appl Environ Microbiol. 1990 Jan;56(1):218–223. doi: 10.1128/aem.56.1.218-223.1990. [DOI] [PMC free article] [PubMed] [Google Scholar]
- Albertson N. H., Nyström T., Kjelleberg S. Starvation-induced modulations in binding protein-dependent glucose transport by the marine Vibrio sp. S14. FEMS Microbiol Lett. 1990 Jul;58(2):205–209. doi: 10.1111/j.1574-6968.1990.tb13979.x. [DOI] [PubMed] [Google Scholar]
- Chet I., Mitchell R. Ecological aspects of microbial chemotactic behavior. Annu Rev Microbiol. 1976;30:221–239. doi: 10.1146/annurev.mi.30.100176.001253. [DOI] [PubMed] [Google Scholar]
- Chet I., Mitchell R. The relationship between chemical structure of attractants and chemotaxis by a marine bacterium. Can J Microbiol. 1976 Aug;22(8):1206–1208. doi: 10.1139/m76-178. [DOI] [PubMed] [Google Scholar]
- Dahl M. K., Manson M. D. Interspecific reconstitution of maltose transport and chemotaxis in Escherichia coli with maltose-binding protein from various enteric bacteria. J Bacteriol. 1985 Dec;164(3):1057–1063. doi: 10.1128/jb.164.3.1057-1063.1985. [DOI] [PMC free article] [PubMed] [Google Scholar]
- Davis C. L., Robb F. T. Maintenance of Different Mannitol Uptake Systems during Starvation in Oxidative and Fermentative Marine Bacteria. Appl Environ Microbiol. 1985 Oct;50(4):743–748. doi: 10.1128/aem.50.4.743-748.1985. [DOI] [PMC free article] [PubMed] [Google Scholar]
- Eisenbach M. Functions of the flagellar modes of rotation in bacterial motility and chemotaxis. Mol Microbiol. 1990 Feb;4(2):161–167. doi: 10.1111/j.1365-2958.1990.tb00584.x. [DOI] [PubMed] [Google Scholar]
- Geesey G. G., Morita R. Y. Capture of arginine at low concentrations by a marine psychrophilic bacterium. Appl Environ Microbiol. 1979 Dec;38(6):1092–1097. doi: 10.1128/aem.38.6.1092-1097.1979. [DOI] [PMC free article] [PubMed] [Google Scholar]
- Humphrey B., Kjelleberg S., Marshall K. C. Responses of marine bacteria under starvation conditions at a solid-water interface. Appl Environ Microbiol. 1983 Jan;45(1):43–47. doi: 10.1128/aem.45.1.43-47.1983. [DOI] [PMC free article] [PubMed] [Google Scholar]
- Komeda Y., Suzuki H., Ishidsu J. I., Iino T. The role of cAMP in flagellation of Salmonella typhimurium. Mol Gen Genet. 1976 Dec 31;142(4):289–298. doi: 10.1007/BF00271253. [DOI] [PubMed] [Google Scholar]
- Kurath G., Morita R. Y. Starvation-Survival Physiological Studies of a Marine Pseudomonas sp. Appl Environ Microbiol. 1983 Apr;45(4):1206–1211. doi: 10.1128/aem.45.4.1206-1211.1983. [DOI] [PMC free article] [PubMed] [Google Scholar]
- Lofgren K. W., Fox C. F. Attractant-directed motility in Escherichia coli: requirement for a fluid lipid phase. J Bacteriol. 1974 Jun;118(3):1181–1182. doi: 10.1128/jb.118.3.1181-1182.1974. [DOI] [PMC free article] [PubMed] [Google Scholar]
- Mesibov R., Adler J. Chemotaxis toward amino acids in Escherichia coli. J Bacteriol. 1972 Oct;112(1):315–326. doi: 10.1128/jb.112.1.315-326.1972. [DOI] [PMC free article] [PubMed] [Google Scholar]
- Mesibov R., Ordal G. W., Adler J. The range of attractant concentrations for bacterial chemotaxis and the threshold and size of response over this range. Weber law and related phenomena. J Gen Physiol. 1973 Aug;62(2):203–223. doi: 10.1085/jgp.62.2.203. [DOI] [PMC free article] [PubMed] [Google Scholar]
- Miller J. B., Koshland D. E., Jr Membrane fluidity and chemotaxis: effects of temperature and membrane lipid composition on the swimming behavior of Salmonella typhimurium and Escherichia coli. J Mol Biol. 1977 Apr;111(2):183–201. doi: 10.1016/s0022-2836(77)80122-8. [DOI] [PubMed] [Google Scholar]
- Oliver J. D., Stringer W. F. Lipid Composition of a Psychrophilic Marine Vibrio sp. During Starvation-Induced Morphogenesis. Appl Environ Microbiol. 1984 Mar;47(3):461–466. doi: 10.1128/aem.47.3.461-466.1984. [DOI] [PMC free article] [PubMed] [Google Scholar]
- Poole P. S., Armitage J. P. Motility response of Rhodobacter sphaeroides to chemotactic stimulation. J Bacteriol. 1988 Dec;170(12):5673–5679. doi: 10.1128/jb.170.12.5673-5679.1988. [DOI] [PMC free article] [PubMed] [Google Scholar]
- Terracciano J. S., Canale-Parola E. Enhancement of chemotaxis in Spirochaeta aurantia grown under conditions of nutrient limitation. J Bacteriol. 1984 Jul;159(1):173–178. doi: 10.1128/jb.159.1.173-178.1984. [DOI] [PMC free article] [PubMed] [Google Scholar]